- 1ETH-Zürich, Geological Institute, Zürich, Switzerland
- 2Department of Earth Sciences, Utrecht University, Utrecht, Netherlands
We analyzed the abundance and distribution of branched glycerol dialkyl glycerol tetraethers (brGDGTs) in grain size fractions of 7 globally distributed river flank sediments and catchment soils in order to determine if and how the initial soil-brGDGT signature is influenced by hydrodynamic sorting upon entering a river and during subsequent transport from land to sea. BrGDGTs are hypothesized to form associations with high-surface-area fine-grained minerals in soils. Such associations, if maintained during transport, may impart resistance to degradation and promote downstream transport, reducing potential interferences by aquatic brGDGTs. We find that brGDGTs are indeed primarily associated with organic carbon (OC) bound to the clay-silt fraction (<63 μm) in both soils and river sediments, and that these associations appear to be maintained during river transport. However, the relative distribution of individual brGDGTs among size fractions is relatively uniform, suggesting that brGDGTs are well mixed in river sediments and that OC-mineral associations are continuously renewed. Consequently, the brGDGT signature finally stored in continental margin sediments appears insensitive to differential particle transport processes. Nevertheless, the lower (upstream) temperature signal generally reflected by brGDGTs in river sediments may also be explained by a contribution of in situ produced brGDGTs leading to an underestimation of reconstructed air temperatures.
Introduction
Rivers form an important connection between terrestrial and marine carbon cycles. A major part of the organic carbon (OC) that rivers export from land to sea originates from soils (e.g., Schlesinger and Melack, 1981). When buried and preserved in marine sediments, (fluvially-transported) soil OC serves as a long-term sink for atmospheric CO2 fixed through photosynthesis (Berner, 1982). In addition, soils are a messenger of terrestrial paleoclimate signals to the marine sedimentary archive. However, uncertainties remain concerning the flux of soil OC to the marine environment, as well as the processes affecting soil OC during mobilization and transit. Prior studies have mostly focused on bulk OC properties, carbon isotopic compositions, or higher plant biomarker (lignin, plant waxes) compositions to assess terrestrial OC contributions to marine sediments (e.g., Hedges et al., 1986; Prahl et al., 1994). However, the heterogeneous nature of terrestrial OC complicates the quantification of this OC pool in marine sediments, and does not specifically account for soil contributions (Gordon and Goñi, 2004). Investigating how soil OC makes its way from land to sea has been hampered by the lack of a suitable tracer for this OC pool.
The discovery of branched glycerol dialkyl glycerol tetraether lipids (brGDGTs) followed by subsequent work that demonstrated their near-ubiquitous presence in peats and soils (Sinninghe Damsté et al., 2000; Weijers et al., 2006) has led to the proposition of their use as tracer for soil OC in carbon cycle and paleoenvironmental studies (Hopmans et al., 2004). Specifically, the abundance of brGDGTs in coastal marine sediments relative to that of crenarchaeol, an isoprenoid GDGT produced by marine plankton, parameterized as the Branched and Isoprenoid Tetraethers (BIT) index (Hopmans et al., 2004; Kim et al., 2006), has been proposed as a proxy for fluvial supply of soil OC to marine systems. Moreover, variations in the relative abundance of different brGDGTs have been found to relate with the mean air temperature (MAT) and pH of the soil in which they are produced (Weijers et al., 2007b). Specifically, estimates of MAT and soil pH can be derived from the proportion of methyl branches and/or cyclopentane moieties within individual brGDGTs. This environmental information encoded in their molecular structures has led to the development of the MBT-CBT (methylation of branched tetraethers-cyclisation of branched tetraethers) paleotemperature proxy (Weijers et al., 2007b). Analysis of down-core changes in brGDGT distributions in fluvially-influenced continental margin sediments may thus carry a continental climate record of the adjacent river basin (Weijers et al., 2007a; Bendle et al., 2010), whilst the application of the BIT index can reveal past changes in the relative contribution of soil OC to continental margin sediments (e.g., Weijers et al., 2009). Recent improvements in chromatographic separation that resolve previously co-eluting brGDGT isomers has helped to disentangle the separate influences of temperature and pH on brGDGT distributional changes, and hence improve their utility as proxies of terrestrial (paleo)environmental conditions (De Jonge et al., 2014a).
The use of brGDGTs as universal tracers of soil OC, and in particular as a continental paleothermometer, is based on the assumption that river-deposited brGDGTs reflect, and faithfully record drainage basin-wide processes (e.g., Weijers et al., 2007a). However, variations in brGDGT distributions discharged by rivers may be caused by factors other than climatic changes within the river basin. Processes of hydrodynamic sorting, microbial alteration, or degradation may affect biomarker signatures during transport. For example, n-alkanes and lignin phenols appear to be associated with different particle size fractions, and may experience different mobilization pathways and transport efficiencies (Prahl, 1985; Prahl et al., 1994; Goñi et al., 1997; Feng et al., 2013). Compound-specific isotope analysis of lignin phenols in sediments from the Gulf of Mexico revealed that C4 plant material derived from the Mississippi River basin was deposited further offshore than material derived from C3 vegetation in the delta, likely due to the association of OC to fine-grained minerals in the river basin, promoting their transport (Goñi et al., 1997). Additionally, recent studies suggest that brGDGTs in river suspended material (SPM) and sediments are not only derived from soils, but may also have an aquatic origin (e.g., Zhu et al., 2011; Kim et al., 2012; Zell et al., 2013, 2014a,b; De Jonge et al., 2014b). These aquatic brGDGTs may consequently modify the original soil-derived brGDGT signature transported and discharged by the river. Thus, to assess the suitability of brGDGTs as tracers of soil OC and proxies of terrestrial paleoclimate in the sedimentary archive it is important to not only understand the processes that they experience during transport, but also to distinguish between the contributions of brGDGTs produced in soils and those in rivers. We hypothesize that brGDGTs form intimate associations with high-surface-area clay minerals in soils. Assuming these associations are maintained both upon erosion and delivery to a river network and during subsequent transport, hydrodynamic sorting would be anticipated to result in efficient transmission of fine-grained particulate matter carrying the soil signal compared to large(r) size fractions (e.g., McLaren and Bowles, 1985). Hydrodynamic sorting processes may therefore be expected to result in distinct brGDGT distributions among particle size fractions in river sediments. This, in turn, might imply that brGDGTs associated with fine-grained sediment fractions may serve as a soil-specific tracer, obviating possible interferences from aquatic brGDGTs.
In order to evaluate potential influences of transport and hydrodynamic sorting processes on brGDGT signals and the use of these compounds as tracers of soil OC or proxy for continental paleoclimate, we here analyze the distribution of brGDGTs among different particle size fractions of sediments from seven rivers spanning different continents and climate zones. When available, riverbank brGDGTs signatures are compared with those in nearby soils in an attempt to determine the source of the brGDGTs associated to the different grain size fractions.
Materials and Methods
Sample Collection and Preparation
A selection of recently deposited sediments from the flanks of seven rivers was made from the sample collection of the Biogeoscience group at ETH Zürich (Figure 1; Table 1). The rivers were selected to cover a large range in latitudes, climate zones, lengths, or catchment area in order to enable linking potentially emerging patterns to environmental parameters, and include the east channel of the Mackenzie River delta (NT, Canada), Fraser River (BC, Canada), Connecticut River (CT, USA), Waimea River (HI, USA), Tempisque River (Costa Rica), upper Rhône River (Switzerland), and the Rakaia River (New Zealand). For each river, material from the site closest to the river mouth was chosen to yield a brGDGT signature that best represents the upstream catchment, as well as the signal that is discharged to the marine environment.
For the Mackenzie, Rhône, and Rakaia Rivers, surface soils (0–10 cm, without litter layer) from the catchment areas were also available. All materials were collected between 2005 and 2011 during different expeditions of the Biogeoscience group, and have been stored frozen after sampling. Details about each river catchment are listed in Table 1. Annual mean air temperatures for each site are from the Global Historical Climatology Network (GHCN; http://www.noaa.gov).
The river flank sediments and soils were freeze-dried, after which macroscopic root material and plant fragments were removed. The sediments and soils were subsampled three times (10–35 g) and separated into a silt/clay fraction (<63 μm), a medium/fine sand fraction (63–200 μm), and a coarse sand fraction (200–1,000 μm) by dry-sieving on an automated shaker. Due to the relatively coarse distribution of the Waimea River sediments, the silt/clay and fine sand fractions were combined into one fraction (<200 μm), and the coarse sand fraction was split into two separate fractions of 200–500 μm and 500–1,000 μm, respectively. The workup in triplicate is indented to average out potential variability or incomplete separation by dry-sieving. All reported values for the size fractions are the average of single measurements on each of the triplicate samples and the standard deviation of these three measurements.
TOC and δ13C Measurements
All available grain size fractions were homogenized and decalcified by acidifying with concentrated HCl vapors at 60–65°C for 60–72 h. The total organic carbon (TOC) and δ13C value of each decalcified sample was determined using a ThermoFisher Delta V Plus isotope ration mass spectrometer (IRMS) coupled with a ThermoFisher Flash elemental analyzer 1112 at ETH Zürich.
GDGT Analysis
One to five grams of each size fraction was extracted using a MARS Xpress microwave extraction system, using 9:1 (v/v) dichloromethane (DCM):MeOH at 100°C for 20 min after 10 min of ramping time. The samples were centrifuged for 5 min at 400 rpm in the extraction vessels, after which the extract was pipetted off and collected in a glass vial. The residues were rinsed with 9:1 (v/v) DCM:MeOH (2x), and all solvents were combined into a total lipid extract (TLE). Following addition of a C46 GDGT standard the TLEs were dried down under N2 before passing over a silica column (deactivated with Mili-Q water, 5% dry weight) to separate the extract into an apolar and a polar fraction, using 9:1 (v/v) hexane:DCM and 1:1 (v/v) DCM:MeOH, respectively. The polar fraction, containing the GDGTs, was dried under N2, dissolved in 99:1 hexane:isopropanol, and filtered over a 0.45 μm PTFE filter prior to analysis with high performance liquid chromatography/atmospheric pressure chemical ionization-mass spectrometry (HPLC/APCI-MS) on an Agilent 1260 Infinity series LC/MS according to Schouten et al. (2007). Separation of the GDGTs was achieved with a Grace Prevail Cyano column (3 μm, 150 × 2.1 mm) after passing through a Prevail Cyano Guard column (5 μm, 7.5 × 2.1 mm) with hexane:isopropanol (99:1, v/v) as eluent. The flow rate was 0.2 ml/min. Each GDGT-fraction was eluted isocratically with 90% A and 10% B for 5 min, and then with a linear gradient to 18%B for 34 min, where A = hexane and B = hexane:isopropanol 9:1 (v/v). Selective ion monitoring of the [M+H]+ was used to detect and quantify the different GDGTs, according to Huguet et al. (2006), except for that a similar response factor was assumed for the GDGTs and the internal standard.
Results and Discussion
TOC Concentration and Composition
The TOC concentrations in river sediments and soils range between 0.1 wt% in the Waimea River (Hi, USA), and 6.0 wt% in the 63–200 μm fraction of the Connecticut River (CT, USA). Although the TOC concentrations show no clear trend with latitude (and thus temperature and/or precipitation) or basin size, highest concentrations of TOC are mostly found in the 63–200 μm fraction in the river sediments, followed by the <63 μm fraction. This latter fraction also contains most TOC in soils (Figure 2A), where OC tends to form aggregates or associations with mineral surfaces, and the smallest particle size fraction (i.e., clay) with the largest surface area exhibit the highest OC concentrations (e.g., Mayer, 1994; Kögel-Knabner et al., 2008; Keil and Mayer, 2014; Doetterl et al., 2016). Although chemical and physical protection of OC by minerals has been assessed in soils and during lateral transport (e.g., hill to valley), the stability of organo-mineral associations upon entering a river and subsequent transport remains poorly studied (Doetterl et al., 2016 and references therein). However, Keil et al. (1997) reported that the relation between OC content and mineral surface area of SPM and river sediments is relatively invariant for several rivers worldwide, but is altered upon entering the marine environment. Notably, in this dataset only the Fraser River sediments have the highest TOC concentration in the <63 μm fraction, whereas the other rivers carry more TOC associated with the 63–200 μm fraction. This may reflect the presence of fragments of undecomposed plant debris carried by the river that reside in these coarser size fractions.
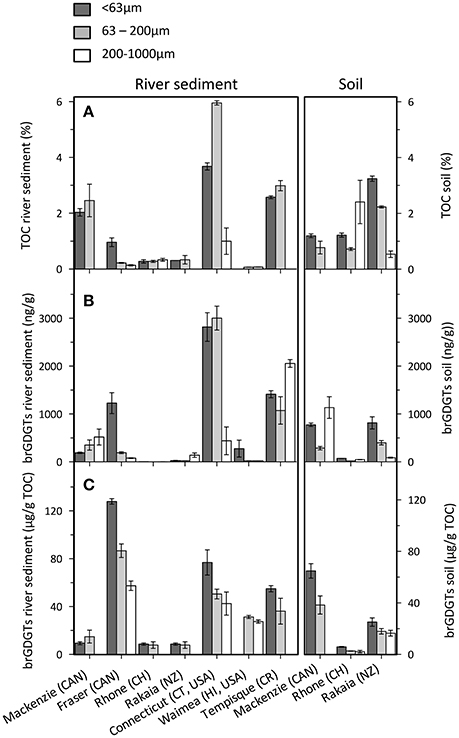
Figure 2. Concentration of (A) TOC, (B) brGDGTs, (C) brGDGTs normalized to TOC in the particle size fractions of riverbank sediments (left panels) and soils (right panels). Error bars represent the variation of triplicate sample workup.
The δ13C values of OC in the river sediments and soils vary between −24 and −30‰ (Figure 3), consistent with a primary origin from higher plant sources. Nevertheless, freshwater phytoplankton or fossil carbon carried by the river may also exhibit δ13C values within this range, so that their contribution to the OC pool cannot be excluded. Published δ13C values for carbonate bedrock (−5 to 0‰; Hitchon and Krouse, 1972) and OC in river SPM (−27.7 to −26.5‰; Vonk et al., 2016) in the Mackenzie River basin suggest that the enriched OC associated with the fine fraction of the Mackenzie River sediment (−12‰; Figure 3) could be caused by the incomplete removal of inorganic carbon.
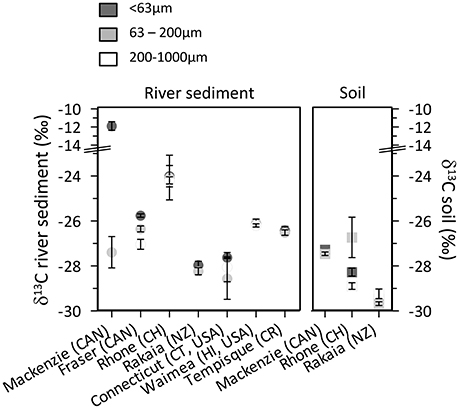
Figure 3. Bulk δ13C values of particle size fractions of riverbank sediments (left panel) and soils (right panel). Error bars represent the variation of triplicate sample workup.
brGDGT Concentrations
The concentration of brGDGTs varies substantially among the river sediments and soils, as well as among the grain size fractions (See datasheet in supplementary information). There is no specific fraction that contains a systematically different concentration of brGDGTs among all rivers (Figure 2B). However, brGDGTs concentrations in the river sediments are significantly correlated with TOC content (r2 = 0.79), suggesting that brGDGTs and OC associated with each fraction have a common preservation mechanism. Subsequent normalization of brGDGT concentrations to TOC reveals that the OC associated with the fine fraction contains greatest proportion of brGDGTs in soils and sediments (Figure 2C). This observation would seem to support the notion that organo-mineral associations formed in soils are maintained during river transport. Furthermore, the <63 μm fraction is most likely to reflect the suspended load of sediments, which is the material that is discharged and reaches the ocean. Thus, simply based on concentrations, this suggests that brGDGTs in river sediments associated with the clay/silt-sized river sediments fraction show potential as specific tracers for soil OC.
brGDGT Distribution and Source
Information on brGDGT distributions in this study was obtained using a Prevail Cyano column for the separation of brGDGTs. A new method that has recently been proposed uses 2 silica columns in tandem, which improves the separation of brGDGTs and any occurring isomers with methyl branches attached on positions 6/6′ rather than 5/5′ (De Jonge et al., 2013; Hopmans et al., 2016). A subsequent re-investigation of the global surface soil calibration set indicated that 5-methyl brGDGTs relate to MAT, and 6-methyl brGDGTs to soil pH (De Jonge et al., 2014a). This explains the pH sensitivity of the MBT' index in the previous calibrations, where 5-methyl and 6-methyl brGDGTs were not fully chromatographically resolved (Weijers et al., 2007b; Peterse et al., 2012; De Jonge et al., 2014a). Furthermore, 6-methyl brGDGTs were among the dominant brGDGTs in SPM from the Yenisei River, suggesting an aquatic source for these isomers (De Jonge et al., 2014b). Even though the “classic” separation method has been used in this study, brGDGT index values vary only marginally among different size fractions from the same site (Figures 4A,B). This indicates that there are no marked differences in contributions of 5-methyl and 6-methyl brGDGTs as a function of grain size, as this would have influenced the total peak areas and thus the index values of the different fractions.
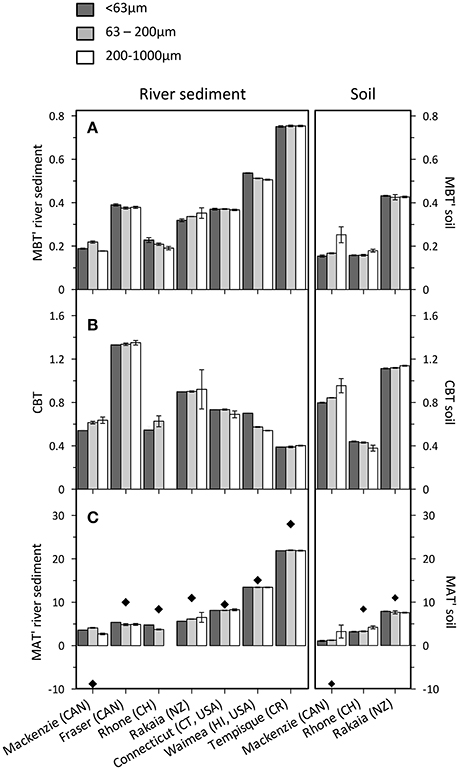
Figure 4. Distributions of brGDGTs in the size fractions of riverbank sediments (left panels) and soils (right panels) expressed in the (A) MBT' index, (B) CBT index, and (C) MBT'-CBT-based MAT. Diamonds indicate actual MAT at the river mouth. Error bars represent the variation of triplicate sample workup.
MBT' and CBT indices are calculated in order to study distributional differences of brGDGTs among grain size fractions in soils (cf. Weijers et al., 2007b; Peterse et al., 2012). Index values show that distributional differences are very minor (Figures 4A,B, and datasheet in supplementary information), varying on average by 0.03 for both the MBT' index (max. 0.10) and the CBT index (max. 0.16). This indicates that although brGDGTs are preferentially associated with the fine grain size fractions (as indicated by the concentration data), brGDGT distributions remain relatively invariant among size fractions from a given site. Hence, upon entering a river, all size fractions should initially carry the same brGDGT signals. However, small particles are generally transported fastest due to their hydrodynamic properties (e.g., McLaren and Bowles, 1985). This could result in an increasing deviation of brGDGT distributions among size fractions downstream, where brGDGTs associated with the fast traveling smallest particles would transport the initial soil signal, whereas brGDGTs associated with the more slowly moving coarser particles would be subject to more intense alteration, and have a greater opportunity for dilution by an aquatic contribution. However, MBT' and CBT index values for brGDGTs in particle size fractions of the river sediments are similar, with average variations of 0.01 (max. 0.04) and 0.06 (max. 0.16) for the MBT' and CBT indices, respectively. This minor variation in brGDGT parameters among river grain size fractions implies either that hydrodynamic sorting processes do not exert strong control on brGDGT distributions in any of the studied rivers, or that the organo-mineral associations change during river transport. Given the high TOC-normalized brGDGT concentrations in the <63 μm fraction of the river sediments, the uniformity in brGDGT distributions across grain sizes suggests that the organo-mineral associations must evolve during transport. Indeed, recent studies on the Yangtze and Danube Rivers showed that the brGDGT signature of river sediments resembles local soils rather than a basin wide signal, suggesting that upstream brGDGTs are continuously prone to degradation and subsequent replacement by more proximal sources during transport, even when associated with minerals (Li et al., 2015 and Freymond et al., 2017, respectively).
Implications for brGDGTs As Tracers for Soil OC and brGDGT-Based Climate Proxies
A consequence of this scenario of continuous replacement is that newly formed associations may also include brGDGTs with an aquatic source. Previous studies have identified the occurrence of in situ brGDGT production based on clear distributional offsets between river sediments and SPM and catchment soils, generally resulting in an underestimation of reconstructed MAT (e.g., Tierney and Russell, 2009; Zell et al., 2013, 2014a,b; De Jonge et al., 2014b). Discrepancies in brGDGT distributions between soils and river sediments are also evident in the present dataset, as manifested in differing MBT' and CBT index values for soils and sediments derived from the same location (Figures 4A,B). However, this observation is based on a single soil sample along the river, which is almost certainly unrepresentative of the spectrum of soil types within the respective catchments. Moreover, soil-derived brGDGTs will have entered the river upstream of the sample location, and can thus not be directly compared to the soils collected at the same site. They should carry an upstream (higher elevation) signal, and evidence for this can be found in derived temperatures from the MBT' and CBT index values which, with the exception of the Mackenzie River, are slightly lower than measured MAT at the sample location (Figure 4C). The higher MAT reflected by the Mackenzie River sediment may be explained by the South-to-North flow direction of the river. Additionally, the strong seasonality and related short growth period at this latitude likely causes a bias in brGDGT distributions toward summer temperatures in both soils and river sediments (Peterse et al., 2014).
Nevertheless, regardless of the actual source of the brGDGTs in river sediments, application of the MBT'-CBT proxy generates MATs that relate well with measured MATs at the sampling location (r2 = 0.71), especially when the South-to-North flowing Mackenzie River sample is omitted (r2 = 0.93). This observation, albeit on a very limited dataset, suggests that rivers worldwide transport a temperature-sensitive signal from land to sea, and that down-core changes in brGDGT distributions in near-river mouth sediments will reflect a record that is primarily forced by temperature. The uniform molecular distributions of brGDGTs among grain size fractions furthermore implies that their signature in river sediments will unlikely be altered by hydrological sorting, although these processes still could influence the overall flux of fluvially transported brGDGTs as a consequence of differential transport of particles with different brGDGT concentrations. Consequently, the BIT index may be most prone to changing hydrodynamic conditions, such as those associated with seasonal or shorter-term (e.g., storm events) variations in fluvial discharge (cf. Kim et al., 2007).
Conclusions
The concentration of TOC-normalized brGDGTs within the <63 μm fraction of soils and river sediments suggests that these brGDGTs develop close associations with high-surface-area, fine-grained mineral phases in soils. However, the remarkably uniform brGDGT distributions among different size fractions of river sediments suggests that hydrodynamic sorting does not play an important role in modifying corresponding climate proxy signals, although the latter may influence the overall flux of brGDGTs and hence observed BIT index values. Instead, brGDGT-mineral associations appear to be dynamic, and evolve during mobilization and transport from soil to river. While the brGDGT signals discharged by those rivers investigated here generally correspond well with mean air temperature, it remains unclear whether the system underestimation of local MATs is caused by the incorporation of in-situ produced brGDGTs upon renewal of organo-mineral associations during transport or by upstream signals.
Author Contributions
FP and TE designed the research, FP performed the analysis and took lead in paper writing, TE provided input and comments.
Conflict of Interest Statement
The authors declare that the research was conducted in the absence of any commercial or financial relationships that could be construed as a potential conflict of interest.
The reviewer HN and handling Editor declared their shared affiliation and the handling Editor states that the process nevertheless met the standards of a fair and objective review.
Acknowledgments
Britta Voss, David Griffith, Camilo Ponton, Lukas Jonkers, Jorien Vonk, Daniel Montluçon, and the Biogeoscience group at ETH Zürich for collecting and providing river sediments. This work was financially supported by ESF-MOLTER grant 3695, ETH Fellowship FEL-36 11-1, and NWO-Veni grant 863.13.016 to FP. The comments of two reviewers contributed to improvement in this manuscript.
Supplementary Material
The Supplementary Material for this article can be found online at: http://journal.frontiersin.org/article/10.3389/feart.2017.00049/full#supplementary-material
References
Bendle, J. A., Weijers, J. W. H., Maslin, M. A., Sinninghe Damsté, J. S., Schouten, S., Hopmans, E. C., et al. (2010). Major changes in glacial and Holocene terrestrial temperatures and sources of organic carbon recorded in the Amazon fan by tetraether lipids. Geochem. Geophys. 11, 1–13. doi: 10.1029/2010GC003308
Berner, R. A. (1982). Burial of organic carbon and pyrite sulfur in the modern ocean: its geochemical and environmental significance. Am. J. Sci. 282, 451–473. doi: 10.2475/ajs.282.4.451
De Jonge, C., Hopmans, E. C., Stadnitskaia, A., Rijpstra, W. I. C., Hofland, R., Sinninghe Damsté, E., et al. (2013). Identification of novel penta- and hexamethylated branched glycerol dialkyl glycerol tetraethers in peat using HPLC-MS2, GC-MS and GC-SMB-MS. Org. Geochem. 54, 78–82. doi: 10.1016/j.orggeochem.2012.10.004
De Jonge, C., Hopmans, E. C., Zell, C. I., Kim, J.-H., Schouten, S., and Sinninghe Damsté, J. S. (2014a). Occurrence and abundance of 6-methyl branched glycerol dialkyl glycerol tetraethers in soils: implications for paleoclimate reconstructions. Geochim. Cosmochim. Acta 141, 97–112. doi: 10.1016/j.gca.2014.06.013
De Jonge, C., Stadnitskaia, A., Hopmans, E. C., Cherkashov, G., Fedotov, A., and Sinninghe Damsté, J. S. (2014b). In-situ produced branched glycerol dialkyl glycerol tetraethers in suspended particulate matter from the Yenisei River, Eastern Siberia. Geochim. Cosmochim. Acta 125, 476–491. doi: 10.1016/j.gca.2013.10.031
Doetterl, S., Berhe, A. A., Nadeu, E., Wang, Z., Sommer, M., and Fiener, P. (2016). Erosion, deposition and soil carbon: a review of process-level controls, experimental tools, and models to address C cycling in dynamic landscapes. Earth Sci. Rev. 154, 102–122. doi: 10.1016/j.earscirev.2015.12.005
Feng, X., Vonk, J. E., van Dongen, B. E., Gustafsson, Ö., Semiletov, I. P., Eglinton, O. V., et al. (2013). Differential mobilization of terrestrial carbon pools in Eurasian Arctic river basins. Proc. Natl. Acad. Sci. U.S.A. 110, 14168–14173. doi: 10.1073/pnas.1307031110
Freymond, C. V., Peterse, F., Fischer, L. V., Filip, F., Giosan, L., and Eglinton, T. I. (2017). Branched GDGT signals in fluvial sediments of the danube river basin: method comparison and longitudinal evolution. Org. Geochem. 103, 88–96. doi: 10.1016/j.orggeochem.2016.11.002
Goñi, M. A., Ruttenberg, K. C., and Eglinton, T. I. (1997). Sources and contribution of terrigenous organic carbon to surface sediments in the Gulf of Mexico. Nature 389, 275–278.
Gordon, E. S., and Goñi, M. A. (2004). Controls on the distribution and accumulation of terrigenous organic matter in sediments from the Mississippi and Atchafalaya river margin. Mar. Chem. 92, 331–352. doi: 10.1016/j.marchem.2004.06.035
Hedges, J. I., Clark, W. A., Quay, P. D., Richey, J. E., Devol, A. H., and Santos, M. (1986). Compositions and fluxes of particulate organic material in the Amazon River. Limnol. Oceanogr. 31, 717–738. doi: 10.4319/lo.1986.31.4.0717
Hitchon, B., and Krouse, H. R. (1972). Hydrogeochemistry of the surface waters of the Mackenzie River drainage basin, Canada – III. Stable isotopes of oxygen, carbon and sulphur. Geochim. Cosmochim. Acta 36, 1337–1357. doi: 10.1016/0016-7037(72)90066-X
Hopmans, E. C., Schouten, S., and Sinninghe Damsté, J. S. (2016). The effect of improved chromatography on GDGT-based paleoproxies. Org. Geochem. 93, 1–6. doi: 10.1016/j.orggeochem.2015.12.006
Hopmans, E. C., Weijers, J. W. H., Schefuss, E., Herfort, L., Sinninghe Damsté, J. S., and Schouten, S. (2004). A novel proxy for terrestrial organic matter in sediments based on branched and isoprenoid tetraether lipids. Earth Planet Sci. Lett. 224, 107–116. doi: 10.1016/j.epsl.2004.05.012
Huguet, C., Hopmans, E. C., Febo-Ayala, W., Thompson, D. H., Sinninghe Damsté, J. S., and Schouten, S. (2006). An improved method to determine the absolute abundance of glycerol dibiphytanyl glycerol tetraether lipids. Org. Geochem. 37, 1036–1041. doi: 10.1016/j.orggeochem.2006.05.008
Keil, R. G., and Mayer, L. M. (2014). “Mineral matrices and organic matter,” in Treatise on Geochemistry. 2nd Edn., Vol. 12, eds H. D. Holland, and K. K. Turekian (Oxford: Elsevier), 337–359.
Keil, R. G., Mayer, L. M., Quay, P. D., Richey, J. E., and Hedges, J. I. (1997). Loss of organic matter from riverine particles in deltas. Geochim. Cosmochim. Acta 61, 1507–1511. doi: 10.1016/S0016-7037(97)00044-6
Kim, J.-H. C., Zell, P., Moreira-Turcq, M. A. P., Pérez, G., Abril, J.-M., Martillaro, J. W. H., et al. (2012). Tracing soil organic carbon in the lower Amazon River and its tributaries using GDGT distributions and bulk organic matter properties. Geochim. Cosmochim. Acta 90, 163–180. doi: 10.1016/j.gca.2012.05.014
Kim, J.-H., Schouten, S., Buscail, R., Ludwig, W., Bonnin, J., and Sinninghe Damsté, J. S. (2006). Origin and distribution of terrestrial organic matter in the NW Mediterranean (Gulf of Lions): Exploring the newly developed BIT index. Geochem Geophys 7. doi: 10.1029/2006GC001306
Kim, J.-H. W., Ludwig, S., Schouten, P., Kerhervé, L., Herfort, J., Bonnin, J. S., et al. (2007). Impact of flood events on the transport of terrestrial organic matter to the ocean: a study of the Têt River (SW France) using the BIT index. Org. Geochem. 38, 1592–1606. doi: 10.1016/j.orggeochem.2007.06.010
Kögel-Knabner, I., Guggenberger, G., Kleber, M., Kandeler, E., Kalbitz, K., Leinweber, P., et al. (2008). Organo-mineral associations in temperature soils: integrating biology, mineralogy, and organic matter chemistry. J. Plant Nutr. 171, 61–82. doi: 10.1002/jpln.200700048
Li, Z., Peterse, F., Wu, Y., Bao, H., Eglinton, T. I., and Zhang, J. (2015). Sources of organic matter in Changjiang (Yangtze River) bed sediments: preliminary insights from organic geochemical proxies. Org. Geochem. 85, 11–21. doi: 10.1016/j.orggeochem.2015.04.006
Mayer, L. M. (1994). Surface area control of organic carbon accumulation in continental shelf sediments. Geochim. Cosmochim. Acta 58, 1271–1284. doi: 10.1016/0016-7037(94)90381-6
McLaren, P., and Bowles, D. (1985). The effects of sediment transport on grain-size distributions. J. Sediment Res. 55, 457–470.
Peterse, F. J. E., Vonk, R. M., Holmes, L., Giosan, N., and Zimov, T. I., Eglinton (2014). Branched glycerol dialkyl glycerol tetraethers in Arctic lake sediments: sources and implications for paleothermometery at high altitudes. J. Geophys. Res. 119, 1738–1754. doi: 10.1002/2014JG002639
Peterse, F., van der Meer, J., Schouten, S., Weijers, J. W. H., Fierer, N., Jackson, R. B., et al. (2012). Revised calibration of the MBT-CBT paleotemperature proxy based on branched tetraether membrane lipids in surface soils. Geochim. Cosmochim. Acta 96, 215–229. doi: 10.1016/j.gca.2012.08.011
Prahl, F. G. (1985). Chemical evidence of differential particle dispersal in the southern Washington coastal environment. Geochim. Cosmochim. Acta 91, 2533–2539. doi: 10.1016/0016-7037(85)90121-8
Prahl, F. G., Ertel, J. R., Goñi, M. A., Sparrow, M. A., and Eversmeyer, B. (1994). Terrestrial organic carbon contributions to sediments on the Washington Margin. Geochim. Cosmochim. Acta 58, 3035–3048. doi: 10.1016/0016-7037(94)90177-5
Schlesinger, W. H., and Melack, J. M. (1981). Transport of organic carbon in the world's rivers. Tellus 33, 172–187. doi: 10.3402/tellusa.v33i4.10730
Schouten, S., Huguet, C., Hopmans, E. C., Kienhuis, M. V. M., and Sinninghe Damsté, J. S. (2007). Analytical methodology for TEX86 paleothermometry by high-performance liquid chromatography/atmospheric pressure chemical ionization-mass spectrometry. Anal. Chem. 79, 2940–2944. doi: 10.1021/ac062339v
Sinninghe Damsté, J. S., Hopmans, E. C., Pancost, R. D., Schouten, S., and Geenevasen, J. A. J. (2000). Newly discovered nonisoprenoid glycerol dialkyl glycerol tetraether lipids in sediments. Chem. Comm. 17, 1683–1684. doi: 10.1039/b004517i
Tierney, J. E., and Russell, J. M. (2009). Distributions of branched GDGTs in a tropical lake system: implications for lacustrine application of the MBT/CBT paleoproxy. Org. Geochem. 40, 1032–1036. doi: 10.1016/j.orggeochem.2009.04.014
Vonk, J. E., Dickens, A. F., Giosan, L., Hussain, Z. A., Kim, B., Zipper, S. C., et al. (2016). Arctic deltaic lake sediments as recorders of fluvial organic matter deposition. Front. Earth Sci. 4:77. doi: 10.3389/feart.2016.00077
Weijers, J. W. H., Schefuss, E., Schouten, S., and Sinninghe Damsté, J. S. (2007a). Coupled thermal and hydrological evolution of tropical Africa over the last deglaciation. Science 315, 1701–1704. doi: 10.1126/science.1138131
Weijers, J. W. H., Schouten, S., Spaargaren, O. C., and Sinninghe Damsté, J. S. (2006). Occurrence and distribution of tetraether membrane lipids in soils: implications for the use of the TEX86 proxy and the BIT index. Org. Geochem. 37, 1680–1693. doi: 10.1016/j.orggeochem.2006.07.018
Weijers, J. W. H., Schouten, S., van den Donker, J. C., Hopmans, E. C., and Sinninghe Damsté, J. S. (2007b). Environmental controls on bacterial tetraether membrane lipid distribution in soils. Geochim. Cosmochim. Acta 71, 703–713. doi: 10.1016/j.gca.2006.10.003
Weijers, J. W. H., Schouten, S., Schefuss, E., Schneider, R. R., and Sinninghe Damsté, J. S. (2009). Disentangling marine, soil and plant organic carbon contributions to continental margin sediments: a multi-proxy approach in a 20,000 year sediment record from the Congo deep-sea fan. Geochim. Cosmochim. Acta 73, 119–132. doi: 10.1016/j.gca.2008.10.016
Zell, C., Kim, J.-H., Balsinha, M., Dorhout, D., Fernandes, C., Sinninghe Damsté, J. S., et al. (2014a). Transport of branched tetraether lipids from the Tagus River basin to the coastal ocean of the Portuguese margin: consequences for the interpretation of the MBT'/CBT paleothermometer. Biogeosciences 11, 2637–5655. doi: 10.5194/bg-11-5637-2014
Zell, C., Kim, J.-H., Hollander, D., Lorenzoni, L., Baker, P., Sinninghe Damsté, J. S., et al. (2014b). Sources and distributions of branched and isoprenoid tetraether lipids on the Amazon shelf and fan: implications for the use of GDGT-based proxies in marine sediments. Geochim. Cosmochim. Acta 139, 293–312. doi: 10.1016/j.gca.2014.04.038
Zell, C., Kim, J.-H., Moreira-Turcq, P., Abril, G., Hopmans, E. C., Sinninghe Damsté, J. S., et al. (2013). Disentangling the origin of branched tetraether lipids and crenarchaeol in the lower Amazon River: implications for GDGT-based proxies. Limnol. Oceanogr. 58, 343–353. doi: 10.4319/lo.2013.58.1.0343
Keywords: GDGT, soil organic carbon, river, tracer, particle size fraction
Citation: Peterse F and Eglinton TI (2017) Grain Size Associations of Branched Tetraether Lipids in Soils and Riverbank Sediments: Influence of Hydrodynamic Sorting Processes. Front. Earth Sci. 5:49. doi: 10.3389/feart.2017.00049
Received: 30 November 2016; Accepted: 29 May 2017;
Published: 13 June 2017.
Edited by:
Moritz Felix Lehmann, University of Basel, SwitzerlandReviewed by:
Helge Niemann, University of Basel, SwitzerlandGesine Mollenhauer, Alfred-Wegener-Institute, Germany
Copyright © 2017 Peterse and Eglinton. This is an open-access article distributed under the terms of the Creative Commons Attribution License (CC BY). The use, distribution or reproduction in other forums is permitted, provided the original author(s) or licensor are credited and that the original publication in this journal is cited, in accordance with accepted academic practice. No use, distribution or reproduction is permitted which does not comply with these terms.
*Correspondence: Francien Peterse, f.peterse@uu.nl