- Department of Archaeology and Art History, Seoul National University, Seoul, South Korea
There is great uncertainty over the timing and magnitude of the termination of the African Humid Period (AHP). Spanning from the early to middle Holocene, the AHP was a period of enhanced moisture over most of northern and eastern Africa. However, beginning 8000 years ago the moisture balance shifted due to changing orbital precession and vegetation feedbacks. Some proxy records indicate a rapid transition from wet to dry conditions, while others indicate a more gradual changeover. Heretofore, humans have been viewed as passive agents in the termination of the AHP, responding to changing climatic conditions by adopting animal husbandry and spreading an agricultural lifestyle across the African continent. This paper explores scenarios whereby humans could be viewed as active agents in landscape denudation. During the period when agriculture was adopted in northern Africa, the regions where it was occurring were at the precipice of ecological regime shifts. Pastoralism, in particular, is argued to enhance devegetation and regime shifts in unbalanced ecosystems. Threshold crossing events were documented in the historical records of New Zealand and western North America due to the introduction of livestock. In looking at temporally correlated archeological and paleoenvironmental records of northern Africa, similar landscape dynamics from the historical precedents are observed: reduction in net primary productivity, homogenization of the flora, transformation of the landscape into a shrub-dominated biozone, and increasing xerophylic vegetation overall. Although human agents are not seen as the only forces inducing regime change during the termination of the AHP, their potential role in inducing large-scale landscape change must be properly contextualized against other global occurrences of neolithization.
Introduction
The termination of the African Humid Period (AHP) induced the most significant, broad-scale landscape change that occurred on the African continent during the Holocene. Although variable in tempo and spatial distribution, rainfall profoundly decreased across most of the northern half of Africa and plant and animal communities reorganized into new ecological niches (Gasse, 2000; Hély et al., 2014; deMenocal, 2015). Human communities likewise changed their settlement and subsistence practices, shifting from foraging to agriculture-dependent economies (Marshall and Hildebrand, 2002; Blanchet et al., 2015). The termination of the AHP is hypothesized to have spurred ecological conditions favorable for the rise of complex social systems in northern Africa and southwestern Asia in which irrigation systems, agricultural resources and food redistribution networks were managed by hierarchical leaders (Kuper and Kröpelin, 2006; Gatto and Zerboni, 2015).
The orthodox view of the termination of the AHP, occurring variably across northern Africa between 8000 and 4500 years ago, is that orbital-induced weakening of monsoon advection over the Sahara caused desertification of inland regions, which enhanced albedo (the reflectance of the sun's rays off the earth's surface), accelerated dust entrainment and created a terrestrial-atmospheric feedback loop that further reduced the precipitation potential (Kutzbach et al., 1996; Gasse, 2000; Prentice and Jolly, 2000). The degree of albedo depends on vegetation cover, which retards sunlight penetrating to the ground. Simulations and proxy records show that an abrupt weakening of monsoon strength around 8200 years ago was not permanent, and there was a partial recovery to humid conditions (Liu et al., 2003; Adkins et al., 2006; Lézine et al., 2011a; Marshall et al., 2011). Later, a similar abrupt reduction in monsoon strength between 5500 and 4500 years ago occurred when the inland flow of monsoons weakened in response to SST variability, but there was no subsequent recovery, which may have been due to enhanced albedo (Liu et al., 2003; Adkins et al., 2006; Lézine et al., 2011b; Marshall et al., 2011). Computer simulation studies demonstrate that a minor increase in albedo in many portions of northern Africa can significantly weaken monsoon flow (Claussen and Gayler, 1997; Levis et al., 2004; Pausata et al., 2016; Skinner and Poulsen, 2016). However, the sheer size and inaccessibility of vast swaths of the Sahara has adversely constrained spatio-temporal understandings of this phenomenon. Thus, there is poor parameterization of boundary conditions and tipping events as reflected in contradictory hypotheses regarding Saharan climate change from this period with one side proposing an abrupt termination of the event (e.g., deMenocal et al., 2000; Salzmann and Hoelzmann, 2005) and the other arguing for a stepwise termination in sync with orbital precession (e.g., Neumann, 1989; Lézine et al., 2005; Kröpelin et al., 2008; Francus et al., 2013).
Given the uncertainty associated with the present state of knowledge, alternative frameworks are needed to construct hypotheses explaining the termination of the AHP. It is known that both effective moisture and terrestrial biomass reduced in the Sahara and Sahel between 8000 and 4500 years ago (e.g., Jolly et al., 1998). It is also known that there is variability in the spatial and temporal distribution of associated landscape change (Renssen et al., 2006; Lézine et al., 2011a; Blanchet et al., 2015). However, proposed synoptic-scale forcing mechanisms of the unevenly distributed trend from pluvial to arid conditions lack causality sufficient to explain the alacrity of the transition in some areas and slow pace in others (Jolly et al., 1998; Kiage and Liu, 2006; Braconnot et al., 2012; Hargreaves et al., 2013).
In this paper, the termination of the AHP is reviewed with the perspective that humans are potentially effective agents for inducing large-scale changes in vegetation, which can, in turn, force the crossing of ecological tipping points. Incipient pastoral economies are documented in prehistoric and historic contexts as reducing vegetal biomass. In the context of the termination of the AHP, a regime shift in vegetation was amplified by reduced inland monsoon flow and reduced precipitation. Thus, in this interpretation humans are not merely passive recipients of climatic variability, but could have been active agents in broad-scale landscape change.
Tipping Points and Regime Shifts
A tipping point, or threshold, is encountered in an ecological system when the biotic environment undergoes a transformation in state from one condition to another (Figure 1; Muradian, 2001; Andersen et al., 2009; Tylianakis and Coux, 2014). More specifically, regime shifts occur when trophic levels change in response to external dynamics (e.g., climate change, over-grazing, invasive species) or internal stimuli (e.g., over-population, biochemical, geomorphological; Folke et al., 2004; Kinzig et al., 2006; Andersen et al., 2009). Once a regime shift has occurred, a return to the previous state will not occur because there are too many variables associated with reproducing identical conditions to the previous regime.
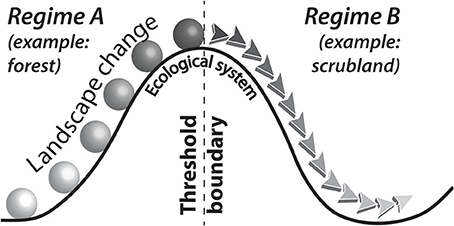
Figure 1. Conceptual model of ecological regime shifts. Forcing dynamics push landscapes to the precipice of the threshold boundary. Once the boundary is crossed, the ecosystem has irreversibly transitioned to a new regime.
Regime shifts occur in response to non-linear dynamics, often with multiple landscape pressures converging simultaneously to force the ecology of a region into a new condition (Scheffer and Carpenter, 2003; Andersen et al., 2009; Johnstone et al., 2016). In modern practice, detecting regime shifts often involves assembling multivariate, long-term ecological research (LTER) datasets, and statistically analyzing independent variables (e.g., Principal Component Analysis) to determine whether an abrupt change has occurred (Lindenmayer and Likens, 2009; Magurran et al., 2010; Müller et al., 2010). Monitoring and quantitative reporting of as many independent variables as possible—developing coherent models of ecological resilience using combinations of terrigenous, aquatic, and atmospheric datasets—has led to multifactorial and holistic indicator sets of where thresholds exist in present landscapes and the timeframes and degrees of pressure necessary to push them over the boundaries (Ellis, 2011; Fisher et al., 2015; Müller et al., 2016). The universal conclusion of LTER studies is that regime shifts that adversely impact biodiversity are never induced from single forcing events—they are always multi-causal, occur after some accumulated period of stress on the ecosystem and the ultimate tipping point does not have to be large scale (Foley et al., 2003; Scheffer and Carpenter, 2003; Pardini et al., 2010).
However, detecting regime shifts in the paleoecological record is fraught with uncertainty, especially in relation to developing concordant timeframes of different ecological variables (Rull, 2014). A classic example of such a misinterpretation can be found in Easter Island (Rapa Nui), where a regime shift was inferred among the pre-colonial inhabitants of the island due to competitive construction of ritual monuments (moai), over-exploitation of the land for agricultural pursuits and warfare (Flenley and King, 1984; Flenley et al., 1991). This scenario was later challenged based on new dates and paleoecological data that suggested contact with Europeans and landscape pressures wrought by the introduction of invasive species such as rats (Rattus exulans) pushed the island over an ecological threshold (Rainbird, 2002; Hunt and Lipo, 2006; Hunt, 2007). In the end, testable hypotheses proffered vis-à-vis incomplete paleoecological datasets provided the bases of more nuanced understandings of anthropogenic forcing of threshold crossing events were gleaned, both on Easter Island and more globally (Walker and Meyers, 2004).
In ecosystems geographically linked to continental systems, threshold crossing on local scales can trigger cascading effects that amplify across a landscape (Kinzig et al., 2006; Rietkerk et al., 2011; Pausas and Keeley, 2014). Localized land degradation in the form of diminishing biodiversity and enhancement of surface albedo has a tendency to spread outwards through dust entrainment, affecting regional atmospheric dynamics, which, in turn, increase the vulnerability of surrounding areas to arrive at their own thresholds (Lare and Nicholson, 1994; Dekker et al., 2007; Nicholson, 2015; Pausata et al., 2016). While investigating regional- and continental-scale regime shifts, the fundamental unit of analysis must be local because chains of events that precipitate threshold crossing normally involve local agents as one component in a complex web of landscape change.
The Scenario
In order for human agents to be considered potential drivers of landscape change sufficient to induce the crossing of an ecological threshold, three criteria must be met. The first is that the pre-threshold crossing condition of the landscape was at the precipice of a tipping point due to factors that weakened the resiliency of the overall system. The second is that a forcing event could have created circumstances plausibly severe enough to induce a regime shift. In the scenario of the termination of the AHP, internal dynamics of the system are unlikely given the scale of the transition both temporally and spatially—the Sahara and Sahel encompass 12.5 million km2 of highly diverse geographies, especially during the AHP. It is inconceivable that localized internal dynamics could have simultaneously (in geological timescales) induced a series of regime shifts across such a large region, although localized cascading effects have been hypothesized as impacting large geographic regions (Kinzig et al., 2006; Rietkerk et al., 2011; Pausas and Keeley, 2014). To make the case that human agency played a role in the transition, this paper will examine how landscapes with no previous exposure to grazing by domesticated animals have been documented as crossing ecological thresholds shortly after new grazing pressures were introduced. The third criterion is evidentiary: data must demonstrate that a transition between ecological regimes on a landscape occurred and that humans could have played a role in the process. If humans had agency in the termination of the AHP, the transition would have been localized and variable in scale. Furthermore, human activities would have induced a feedback loop altering regional ecologies that contributed to continental-scale changes.
First Criterion: The Precipice of Landscape Change?
In the context of the AHP, regime shifts in northern and eastern Africa were not uniformly distributed or uni-causal. Maximum summer insolation values across the Sahara and Sahel peaked between 10,000 and 9000 years BP (Berger and Loutre, 1991), which is generally regarded as the zenith of the AHP (Gasse, 2000; Prentice and Jolly, 2000; Wanner et al., 2008). It is difficult to generalize the vegetation for such a large and topographically diverse area, and there are currently no full-scale vegetation maps of the entire region based on proxy data, but coarse approximations can be made (Figure 2). Hély et al. (2014) provide a latitudinal reconstruction of vegetation based on proxy data, but the paucity of sampling sites has inhibited time-transgressive longitudinal studies. Paleoclimatic models often conflict in the details of the spatial distribution, but the overall consensus of both models and proxy data indicate that the present-day Sahara was significantly wetter and included much higher plant diversity than is found in the region today (Watrin et al., 2009; Hély et al., 2014; deMenocal, 2015).
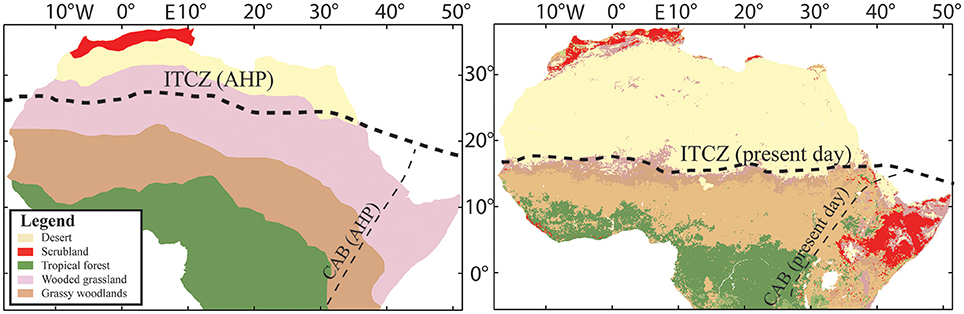
Figure 2. African biomes. Left: Reconstructed African Humid Period (AHP biomes based Larrasoaña et al., 2013). Average summer position of the Intertropical Convergence Zone (ITCZ) and Congo Air Boundary based on Junginger et al. (2014). Right: Present-day biomes created from data downloaded from the Atlas of the Biosphere (http://nelson.wisc.edu/) originally digitized from Ramankutty and Foley (1999).
The floristic composition of the Sahara during the AHP does not appear to have an analog in today's Sahara. During the zenith of the AHP, plant communities distinctly characterized today as “Guineo-Congolian” (moist tropical woodlands), “Sudanian” (grassy woodlands), “Sahelian” (wooded grassland), and “Saharan” (xeric-adapted grasses) lived side-by-side between 12° and 20°N (Watrin et al., 2009; Hély et al., 2014). Riparian areas in now-desiccated sections of the Sahara were comprised of woodlands (e.g., Alchornea sp., Piliostigma sp., Celtis sp.), and grasses (Calligonum sp., Ephedra sp.) were distributed up to 24°N by 4200 years BP (Watrin et al., 2009; Hély et al., 2014). Based on the composition of plant taxa alone, the maximum zone of precipitation during the AHP likely fell between 15° and 20°N (Hély et al., 2014).
However, weakening of the summer monsoons associated with reduced solar insolation (Figure 3A) following the Holocene Climatic Optimum (HCO) induced new atmospheric dynamics across the entire region, beginning in the northern Sahara and gradually extending southward. After 8200 years BP, landscape changes trending toward xeric conditions are documented in the northeastern Sahara (Hoelzmann et al., 2001) with periodic recharges in some lake basins until 4500 years BP, after which the region achieves the desert-like conditions that predominate today (Gasse, 2000). An abrupt regional transition from grassy to shrub-dominated vegetation is recorded in two sediment cores from the mouth of the Nile River at 8700 and after 8000 years BP, but there is no concurrent rapid reduction in river runoff or precipitation suggesting that monsoon activity was only slowly decreasing at that time (Figures 3B,C; Hennekam et al., 2014; Blanchet et al., 2015). There is consistent agreement in the proxy record that, beginning in northern Africa by ca. 8200 years BP and spreading south into what is now the Sahel and eastern Africa by 4500 years BP, the landscape transitioned from generally pluvial to arid or semi-arid ecological conditions, even though the timing, pace and magnitude of the transition was variable (Shanahan et al., 2015).
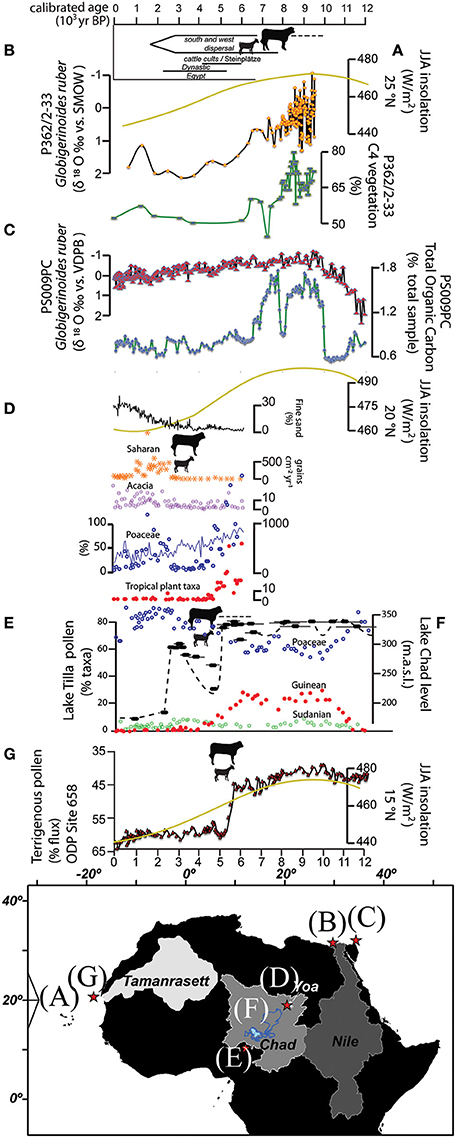
Figure 3. Paleoclimatic proxies and the introduction of livestock economies in Africa. Earliest occurrences of cattle and goats are represented by zoomorphic figures. (A) Summer insolation at 25°, 20°, and 15°N (Berger and Loutre, 1991); (B) Nile River hydrology and vegetation reconstruction (Blanchet et al., 2015); (C) Nile River hydrology and vegetation reconstruction (Hennekam et al., 2014); (D) Lake Yoa fine sand flux (dune activation) and pollen spectra (Kröpelin et al., 2008); (E) Lake Tilla pollen spectra in circles (Salzmann et al., 2002); (F) Black dashed line represents the Lake Chad level reconstruction. Elongated ovals are elevation-measured radiometric ages and associated statistical uncertainty (Armitage et al., 2015); (G) Ocean sediment core reflecting eolian activity near Cap Blanc, Mauretania (deMenocal et al., 2000). The Nile, Yoa, and Megalake Chad basins were created in ArcGIS 10.1 from the GTOPO30 DEM available from the USGS web data portal (http://earthexplorer.usgs.gov). The Tamanrasett paleodrainage was digitized from Skonieczny et al. (2015).
Climate models consistently predict that changes in orbital precession weakened inland monsoon flow over the northern Sahara (>20°N) by 8200 years BP, and this effect spread southward (to 12°N) over the next 3500 years (Claussen et al., 1999, 2013; de Noblet-Ducoure et al., 2000; Foley et al., 2003; Renssen et al., 2006, 2012; Liu et al., 2007; Hély et al., 2009; Watrin et al., 2009; Lézine et al., 2011b; Harrison et al., 2015). Ecological boundary conditions in regions affected by the termination of the HCO have been attributed to their position relative to the Intertropical Convergence Zone (ITCZ)—once the rain belt migrated southwards, their predominant source of moisture was substantially reduced (Liu et al., 2007; Castañeda et al., 2016). As will be detailed below, the speed with which the transition from high- to low-biodiversity landscapes occurs is variable according to both longitude and latitude.
Second Criterion: Crossing the Threshold Due to External Forcing?
In simulations of the AHP termination, it has been proposed that ecosystems that hosted diverse plant communities reduced the sensitivity of the Saharan ecosystem to climatic effects (Claussen et al., 2013). In a study of vegetation response to AHP termination from Lake Mbalang in northern Cameroon, the impacts of steadily decreasing precipitation on the floristic composition of the site were gradual, which is attributed to the pre-event “stability of vegetation” that preceded climate change (Vincens et al., 2010). Similar landscape dynamics have been documented from pollen records recovered from Lake Tilla (Salzmann et al., 2002), Lake Yoa (Lézine, 2009), Lake Mega-Chad (Amaral et al., 2013), portions of the eastern Sahara (Neumann, 1989), and from an offshore sediment core near Senegal (Niedermeyer et al., 2010; Schefuß et al., 2015) where the vegetation was slow to respond to changing rainfall patterns until clear human impacts are documented in the proxy record.
On the other hand, there are many reconstructions of hydrological cycles and vegetation indexes to indicate abrupt termination of the AHP. There is a rapid decrease in the abundance of C4 vegetation running off into the Nile River between 8500 and 7800 years BP and 6500 to 6000 years BP, which is out of phase with the more slowly decreasing quantities of river discharge (Blanchet et al., 2015). A similar abrupt changeover from C3 to C4 vegetation is recorded at I-n-Atei in southern Algeria at 7400 years BP (Lécuyer et al., 2016). A spike in terrigenous dust from collected offshore from the western Sahara (Figure 3G; deMenocal et al., 2000; Adkins et al., 2006), abrupt reductions in the distribution of Guineo-Congolian plant taxa after 4500 years BP north of 20°N (Hély et al., 2014) and lake level reconstructions from Lake Mega-Chad (Armitage et al., 2015) and Ethiopia (Gillespie et al., 1983; Gasse and Van Campo, 1994; Gasse, 2000) infer abrupt hydrological regime shifts to dry conditions (see also Tierney and deMenocal, 2013). Curiously, potassium content of sediments from the now-dry Lake Chew Bahir, located in southern Ethiopia indicates a slow, but steady transition to a xeric landscape in phase with orbital precession (Foerster et al., 2012), which is in contrast to nearby hydrological proxies in northern Kenya that show rapid changes in the hydrological cycle that culminated in an 80 m regression in the level of Lake Turkana at 4500 years BP (Garcin et al., 2009, 2012; Junginger et al., 2014; Bloszies et al., 2015). Such discordance in proxy data, even at scales of hundreds of kilometers, is typical for the terminal AHP.
Precipitation and vegetation dynamics were often out of sync with orbital precession, reflecting localized landscape pressures and feedbacks. In a synthetic review of AHP dynamics based on a complex, non-linear δDwax record from Lake Bosumtwi, Ghana, Shanahan et al. (2015) argue that the termination of the AHP was locally abrupt in the northern and eastern portions of Africa, but was buffered in the southern Atlantic regions by less variability in peak summer insolation values throughout the Holocene. This phenomenon is explained by two potential forcing mechanisms: either the summer monsoon belt (vis-à-vis the ITCZ) shifted south or there were synoptic-scale changes in Indian Ocean circulation patterns that reduced inland moisture flux over the continent (Shanahan et al., 2015). These mechanisms are not necessarily mutually exclusive. Atlantic Ocean sea surface temperatures also contributed to West African monsoon dynamics during the middle Holocene (Weldeab et al., 2005), but do not appear to have impacted local vegetation patterns as significantly as in northern Africa. Tierney et al. (2011) and Tierney and deMenocal (2013) attribute rapid termination of the AHP in northeastern Africa to constriction of the Congo Air Boundary (CAB) and ITCZ during the middle Holocene (see also Costa et al., 2014; Junginger et al., 2014; Bloszies et al., 2015; Castañeda et al., 2016). The CAB and ITCZ previously trapped tropical Atlantic moisture into a much wider area within the greater Sahara and Sahel region during the AHP, but with weakening summer insolation during the termination phase, the eastern and northern extents of these pressure convergence zones constricted between 5° and 7° relative to their maximum geographic extents (Figure 2; Gasse and Roberts, 2004).
Due to the spatially discordant nature of the evidence for the timing of the termination of the AHP, there is a need to consider alternative devegetation mechanisms beyond orbital parameters that could have amplified terrestrial-atmospheric feedbacks. The term “neolithization” is used to explain transformative landscape processes related to the transition of human societies from fully foraging to fully farming economies (Kuzmin and Orlova, 2000; Rispoli, 2007; Goring-Morris and Belfer-Cohen, 2011; Kim, 2014). Contrary to other places in the world, neolithization in northern Africa was not associated with the first use of ceramics and other forms of storage as so-called Aqualithic communities had developed pottery by 10,000 years ago (Stewart, 1989; McDonald, 2016). Instead, neolithization in the northern African context represented a shift in landscape tenure processes, which resulted in three primary changes to the human relationship with the environment: (A) transformation of the biotic environment from one that inherently produced resources for humans into one that must be cultivated to yield resources, (B) the net primary productivity (NPP) of the landscape diminished even as the anthrocentric productivity (ACP) initially increased, and (C) part and parcel of increasing the ACP resulted in a reduction in overall biodiversity and enhanced albedo. Increasing the ACP conforms to general theories of niche construction in which human communities manipulate the parameters of the ecosystem to improve their access to plant and animal resources (Smith, 2011). The tradeoff between NPP and ACP is an unintentional, yet repeated phenomenon in neolithization across the world. If NPP suffers to the detriment of the total ecology of a region, ACP may collapse, which ecologists typically call land denudation (Smil, 2000; Lal, 2012; Li et al., 2012).
Neolithization is used to argue in favor of the hypothesis of a “long Anthropocene” in which humans have been transformative agents on landscapes for many thousands of years, particularly following the introduction of agricultural techniques (Fuller et al., 2011; Ellis et al., 2013; Foley et al., 2013; He et al., 2014; Certini and Scalenghe, 2015; Lyons et al., 2016). In this view, humans have co-evolved as natural earth processes rather than acting as external forcing agents like orbital precession, and their influence on shaping the landscape can be traced deep into prehistory (Foley et al., 2013). As an apex species, humans have always played a significant role in the ecology of the landscapes they inhabit dating as far back as the Pleistocene (Smith, 2011; Ellis et al., 2013). In more recent times, large-scale land clearance resulted in significant deforestation and soil erosion during the early Neolithic periods of southwestern Asia (Yerkes et al., 2012), China (Zong et al., 2007), and northwestern Europe (Innes et al., 2013). A pollen study from the Tibetan Plateau documents the transformation from a Poaceae- to Kobresia-dominated landscape in intervals that correlate to changes in the monsoon system dating back to 6000 years ago (Schlütz and Lehmkuhl, 2009). Although such correlations can only provide a tentative basis for causation, apex species have been documented in numerous instances of profoundly altering regional vegetation and geomorphology (Terborgh and Estes, 2013).
This process is difficult to document in paleoecological records, so synchronic LTER conducted in historical settings provide the basis for understanding the processes involved with anthropogenic regime shifts. For example, in New Zealand climate change provided the backdrop for stress on rainforest ecosystems, but direct anthropogenic effects of deforestation, burning and the introduction of invasive species ultimately forced the system into a new regime following European settlement of the islands (Johnstone et al., 2016). Prior to the 1840s, the few European settlers in New Zealand were scattered around the coastal regions, but by the 1880s there were settlements throughout the interior of both North and South Islands, most of which were economically focused on sheep herding (Peden, 2011). Prior to European colonization, approximately two-thirds of North Island and one-fourth of South Island were covered in temperate rainforests (McGlone, 2009). By the end of the nineteenth century, forest cover in New Zealand had been reduced in half, wetlands were drained, and tussock grasslands were burned off to make way for sheep herding and cereal grain agriculture (Parsons and Nalau, 2016). The induced regime shifts within a 50-year period have proven to be irreversible even with aggressive efforts to restore the ecosystem to its pre-1840 condition (e.g., Whitehead et al., 2014; King et al., 2015). This phenomenon occurred with total accumulated population levels below 800,000 people on a landmass of 268,000 km2 (Easton, 2011). Climate stress alone cannot sufficiently explain such a rapid transformation of the New Zealand landscape from forest to grasslands, so obvious contributing factors must be considered.
More analogous to the African context, the introduction of domesticated livestock by Euroamericans into semi-arid and arid regions of the Americas profoundly altered the ecosystem, inducing regime shifts in many regions. Grazing and browsing ungulates evolved in the Americas during the Cenozoic and were a critical component of the ecological matrix (Grayson, 2011; Woodburne, 2012). Prior to Euroamerican settlement, vast prairie grasslands spanned the interior upland regions of both North and South America. However, with the exception of Highland South America, there were no domesticated grazers present before the arrival of European settlers. Cattle (Bos taurus) introduced a new pressure to the landscape that spatially and temporally correlates to a regime shift from grassland to scrubland (Van Auken, 2000).
Two studies conducted in climatically analogous ecosystems to the terminal AHP are instructive for understanding the relationship between the introduction of domesticated livestock and ecological regime shifts. Research in southern Texas, USA attributes the replacement of indigenous savannas by mesquite (Prosopis glandulosa)-dominated shrubland with a combination of overgrazing, fire suppression, and climate change dating back to early Euroamerican colonization of the region (Archer, 1989). A study of tree rings and fire history matched with 125 years of historical records of settlement in south-central Oregon, USA finds positive correlations between the introduction of livestock and growth of the western juniper (Juniperus occidentalis) (Miller and Jeffrey, 1999). More generally, a synthetic quantitative review of multiple diachronic studies of rangelands conducted throughout North America finds consistent correlations between the introduction of domesticated livestock to xeric ecosystems, commensurate decline in floral biodiversity and increase in shrub growth (Jones, 2000; see also Morris and Rowe, 2014). Once the threshold is crossed, experiments demonstrate that it will not return to its pre-disturbance state without significant human intervention (e.g., Laycock, 1991; Curtin, 2002; Loeser et al., 2007).
The potential of humans to transform landscapes and prompt threshold crossing has been extensively documented in both prehistoric (Boivin et al., 2016) and historic (Jones, 2000) contexts. Multiple factors lead ecosystems to the verge of regime change, but anthropogenic effects documented in historical contexts have been demonstrated as profoundly capable of reducing the biomass of a landscape. Such effects accelerate rates of devegetation and soil loss and can spatially cascade as albedo and dust entrainment are enhanced and a terrestrial-atmospheric feedback loop is created (Pausata et al., 2016). That the Sahara and eastern Africa stood at the precipice of a large-scale landscape transformation at the end of the AHP is not in dispute. The question of whether human agents were sufficiently capable of accelerating the termination of the AHP in some regions relies on evaluating diachronic evidence of landscape change coeval with the introduction of new land tenure systems.
Third Criterion: Threshold Crossing and the Arrival of Domesticated Livestock in Africa
When viewed through the lens of anthropogenically-induced regime change, humans are potential amplifying agents in reducing NPP, and in areas where there are coeval archeological and palynological records, local landscape change often occurred at profound scales. In a large-scale analysis, sediment runoff in the Nile River progressively increases from the early termination phase of the AHP (ca. 8000 years BP), which is inferred to be the result of intensified use of land associated with farming and animal pastoralism (Ehrmann et al., 2016). More localized studies demonstrate the mechanisms by which large-scale changes could have been induced. In the western Mediterranean, the arrival of domesticated taxa at Ifri Oudadane by 7300 years BP was concurrent to a significant reduction in native arboreal pollen (AP) and grasses with significant increases in shrubs, specifically maquis species (Figure 4A; Morales et al., 2013). The growth of maquis vegetation has been associated with livestock grazing and burning during the Neolithic colonization of the Mediterranean region. Maquis growth promoted evergreen holm oaks (Quercus ilex), strawberry trees (Arbutus unedo), and basswood (Phillyrea latifolia) at the expense of deciduous oaks (Quercus sp.), manna trees (Fraxinus sp.), terebinths (P. terebinthus), wild service trees (Sorbus sp.), and elms (Ulmus sp.) (Geddes, 1983; Naveh, 1987). There is a progressive decline in AP concurrent with an increase in NAP and cereal cultivars at the site of Ifri n'Etsedda, Morocco between 7400 and 6800 years BP (Figure 4B; Linstädter et al., 2016). At the site of Tin-a-Hanakaten, Algeria, a similar transformation of flora are documented along with evidence of significant eolian activity at 7200 years BP, which is stratigraphically associated with the first occurrences of substantial quantities of cattle remains in the archeological deposits (Figure 4C; Aumassip, 1984).
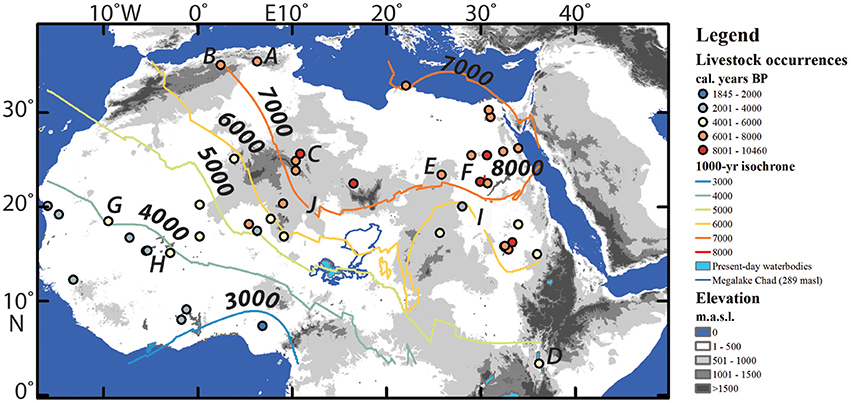
Figure 4. Locations of early occurrences of domesticated livestock in Africa. Data points were largely drawn from published archeological literature (e.g., Gifford-Gonzalez, 2005; Fuller et al., 2011; di Lernia, 2013; Linseele et al., 2014; Ozainne, 2014; Gatto and Zerboni, 2015). The 1000-year isochrone was digitized as contour lines from an ordinary kriged spherical semivariogram model (created in ArcGIS 10.1) of the distribution of early livestock occurrences. Archaeological sites mentioned in the text: (A) Ifri Oudadane, (B) Ifri n'Etsedda, (C) Tin-a-Hanakaten/Uan Afuda, (D) Lake Turkana, (E) Nabta Playa, (F) Fayum depression, (G) Hodh depression/Dhar Tichitt, (H) Ounjougou, (I) Selima Oasis, (J) Segedim depression.
Further south, a sediment core from Lake Chad provides evidence for a gradual increase in non-arboreal pollen (NAP) and shrub vegetation at the expense of AP and non-wetland grasses between 6700 and 5000 years BP (Amaral et al., 2013) with an abrupt switch to arid conditions after 5000 years BP (Figure 3F; Armitage et al., 2015). This time period has been posited, though not yet archeologically proven, to be when domesticated plants and animals arrived in the region (MacEachern, 2012). Nearby, a curiously abrupt reduction in Guinean and Sudanian plant taxa by 3300 years BP in favor of Sahelian shrublands is recorded in the Manga Plateau west of Lake Chad (Figure 3E; Salzmann and Waller, 1998; Salzmann et al., 2002). However, the hypothesis that human agency played a role in the transformation is questioned by the latter study's authors. The pollen record at Lake Yoa in the northern catchment of the Megalake Chad basin, shows the transition to a semidesert plant community on disturbed dunes after 4600 years BP and true desert communities are found after 2700 years BP (Figure 3D; Kröpelin et al., 2008; Francus et al., 2013). This corresponds to the time when archeology indicates that pastoralism replaced hunting and gathering as the primary subsistence economy (Van Neer, 2002).
In eastern Africa, the arrival of domesticated animals is difficult to precisely ascertain because the point of first entry likely occurs in the Lake Turkana region when lake levels were approaching their nadir (Figure 4D; Wright et al., 2015). However, by 4500 years BP, domesticated cattle and an accompanying Neolithic complex, including megalithic burial features, had taken root in the region (Grillo and Hildebrand, 2013) and had nominally spread southward into the equatorial regions at approximately the same time (Wright, 2007; Prendergast, 2010; Lane, 2013). Initial pastoral settlement of the Ethiopian Highlands also occurs at ~4500 years BP (Lesur et al., 2014). Coincidentally, there is a near-simultaneous monotonic regression in lake levels across the region recorded in central Ethiopian lakes (Gasse, 2000) and Lake Turkana (Bloszies et al., 2015), whereas lake levels and vegetation changes in the equatorial regions of Lake Victoria (Berke et al., 2012) and closer to the Indian Ocean coast (Tierney et al., 2011) were more attenuated. Although abrupt changes in hydrological systems were common in lakes on the Ethiopian Plateau drainage basin prior to 4500 years BP, the final regression is linked to the change in position of the CAB with vegetation-feedback factors considered as unlikely contributors (Tierney and deMenocal, 2013; Nutz and Schuster, 2016).
Changing climatic conditions progressively trending toward more arid conditions presented the backdrop for a radical transformation of the northern African landscape. However, the variable tempo and intensity of the termination of the AHP is spatially correlated to local transitions to shrubland environments and accelerated rates of soil erosion (Kutzbach et al., 1996; Braconnot et al., 1999; Foley et al., 2003), which, critically, also correspond to increasing human populations (Manning and Timpson, 2014) and the spread of the domesticated animal economy in the same locations at the same times (Supplementary Material). In tropical western Africa where pastoralism took root later and was a minor component of the subsistence economy relative to plant cultivation (Ozainne, 2014), the termination of the AHP was significantly later, progressed at a slower pace, occurred with reduced magnitude relative to the subtropical regions to the north and took place in a region with a robust ambient floral biodiversity (Shanahan et al., 2015). On the Ethiopian Plateau, pre-colonial endemic floral species biodiversity was likewise high in locations where pollen records exist (Lamb et al., 2004; Umer et al., 2007), which may have attenuated the impact of vegetation feedbacks on the hydrological cycle even though the speed of the AHP termination was high.
Discussion: Could African Neolithic Populations have Induced Regime Changes?
In every case presented above, there is spatio-temporal correlation between the introduction of domesticated animal economies, the reduction of AP and/or grasslands, an increase in shrub species and the eventual (or concurrent) transition from wet to dry conditions. Such correlations produce a “chicken and egg” dilemma—which came first? Correlation does not demonstrate causation, and it is possible that domesticated animal economies moved into shrub-transitioning environments in response to ecological shifts rather than causing such shifts. Indeed, this is the orthodox view of the relationship between climate changes and the spread of the Neolithic throughout sub-Saharan Africa (Smith, 1992; Marshall and Hildebrand, 2002; Kuper and Kröpelin, 2006; Ozainne, 2014; Wright, 2014; Gatto and Zerboni, 2015).
It should be recognized that there was tremendous diversity in early African pastoral economies and in many ways, pastoral economies co-evolved with the landscapes they inhabited. Domesticated cattle from Nabta Playa may be among the earliest in the world and may have been tamed by humans beginning 11,000 years ago (Figure 4E; Wendorf and Schild, 1998), although this claim is controversial. There is also evidence for penning of Barbary sheep (Ammotragus lervia) at Uan Afuda in southwestern Libya by the 9th millennium BP as part of a delayed return foraging strategy (Figure 4C; di Lernia, 2001). More secure contexts for domesticated animals in the Fayum Depression date to 7350 years BP and are associated with fish remains and a diverse array of mobility and settlement practices (Figure 4F; Linseele et al., 2014). Further up the Nile River, low mobility pastoral economies inhabit the alluvial plain beginning 7300 years BP (Honegger and Williams, 2015). In the Acacus Mountains of southwestern Libya, strontium isotopes from burials are used to argue for a possible increase in mobility patterns through the Holocene as the climate became drier (Tafuri et al., 2006). The introduction of domesticated livestock in the Hodh depression of south-central Mauritania began 4500 years BP and is associated with the formation of Neolithic villages, such as Dhar Tichitt (Figure 4G; Holl, 2012). Given the diversity of human-animal-landscape relationships across northern and eastern Africa during the early to middle Holocene, there should not be an expected one-size-fits-all model for how ecological systems (which are themselves diverse) respond to new anthropogenic pressures.
However, there are common features that underpin the introduction of domestic economies into previously undomesticated landscapes. Unlike New Zealand, North America and Africa had large populations of endemic mammalian herbivores prior to the arrival of livestock economies. In herbivore-rich, semi-arid regions of the earth, fire is a natural feature of the landscape (Schüle, 1990; Marlon et al., 2013). The fire record in the Sahara is sparse, but Neolithic archeological sites in Ounjougou in southern Mali (Figure 4H; Huysecom et al., 2004), Selima Oasis in Sudan (Figure 4I; Haynes et al., 1989) and the Segedim depression in northern Niger (Figure 4J; Schulz, 1994), among many, yield evidence for common occurrences of fires during the Holocene, which is interpreted as a landscape management tool, particularly after the introduction of domesticated animals (Kershaw et al., 1997). Based on a charcoal record from Lake Tilla, northeastern Nigeria (Figure 3E), Salzmann et al. (2002) argue that burning was a continuous feature of landscape maintenance throughout the Holocene, regardless of the presence of a foraging or domesticated subsistence economy (see also Marlon et al., 2013).
The presence of a natural fire-adapted ecology presented an opportunity for pastoralists that gave them an advantage over wild bovids. Cattle and caprines are selective grazers and browsers, and their feeding habits can significantly enhance the range of shrub vegetation (Grover and Musick, 1990; Moleele et al., 2002). Following a fire, grasses will be the first pioneers on the landscape, which will be consumed by grazing animals. On the other hand, shrubs are lower-ranked fodder for livestock and also have limited transpiration and retention of surface moisture within soils because of deep root systems. Whereas wild ungulates are likely to avoid fire-disturbed landscapes for a significant amount of time following a fire since they are high-risk/low-reward ecosystems, domesticated animals will go where they are directed (Schüle, 1990; Gil-Romera et al., 2011). Such landscape practices represent disruptions in the natural “ecology of fear” (Terborgh and Estes, 2013)—whereby wild animals do not overtax an ecological system due to predator avoidance, herded livestock respond to artificial constraints. Therefore, the ecological impacts that fire can have on the landscape are amplified by the effects brought by livestock trampling/soil compaction and foddering following burning.
As outlined above, the key ingredients to anthropogenically-induced ecological regime change involve natural or artificial processes and population growth or increasing landscape pressures sufficient to push the system into a new state. In the case of the termination of the AHP, all of these conditions were satisfied simultaneously. Vegetation feedbacks are inferred as inducing rapid changes in the hydrological cycle over the Sahara between 6000 and 4000 years BP (Claussen et al., 1999), which is the period in which animal pastoralism manifests as the dominant mode of subsistence throughout much of the region (Marshall and Hildebrand, 2002; Kuper and Kröpelin, 2006). Various measures of population growth include a significant increase of radiocarbon ages for the Neolithic (Manning and Timpson, 2014), and increased production of fire-cracked rock features (hearths) called Steinplätze between 5800 and 5000 years BP in the central Sahara are interpreted as proxies for population growth (Gabriel, 1987).
Conclusion
The spatial and temporal hetereogenity of the termination of the AHP points to potential anthropogenic influences inducing local ecological threshold crossing events, which catalyzed devegetation and negative feedbacks between terrestrial-atmospheric domains. Local regime shifts have the potential to spread outward as humans guide livestock to new pastures. Middle Holocene human population growth and natural (orbital-induced) climate change underpinned the landscape pressures, but the particular grazing habits of domesticated relative to wild ungulates on fire-adapted, xeric landscapes are argued here to be the decisive factor in irreversibly reducing the NPP of ecosystems that existed in a threshold state. Numerous historical examples from the European colonization of the Americas and Pacific Rim demonstrate the plausibility of this scenario—as humans alter ecosystems to construct high ACP niches, they induce changes that adversely impact NPP. The deep time histories of domesticated bovids in Eurasia and Africa preclude a synchronic view of the effects of the incipient spread of livestock, but the available diachronic evidence from the Sahara, in particular, finds correlative patterns between the arrival of domesticated stock and the changeover of the landscape from high NPP to desert.
Where available, the evidence suggests that there is systematic homogenization of the floral composition of terminal AHP landscapes commensurate with the spread of shrubbery and reduced precipitation. Subsistence choices were predicated on ecological conditions, and early pastoral economies took root against the backdrop of a progressively drying climate. Because humans have been documented as exerting significant pressures on the NPP of prehistoric and historic landscapes elsewhere in the world, it is conceivable that they were also catalysts in accelerating the pace of devegetation in the Sahara at the end of the AHP. This, in turn, would have enhanced albedo, dust entrainment and retarded inland monsoon convection (Kutzbach et al., 1996; Braconnot et al., 1999; Foley et al., 2003; Pausata et al., 2016), pushing pastoralists into new territories to begin the cycle again. The Neolithic quest to maximize ACP may have pounded the final nails into the NPP coffin, and desertification of the Sahara was the end result of the cumulative process.
Scientists have coined the term “Anthropocene” to refer to the modern period in which our species has apparently tipped the planet across a threshold of landscape change that is profound and irreversible (Crutzen and Stoermer, 2000). Many prefer the term “the Great Acceleration” in order to conceptually distinguish human impacts to ecosystems wrought in the pre-Industrial era from the post-Industrial era (Braje and Erlandson, 2013; Steffen et al., 2015). However, the Great Acceleration does not imply the “Great Start.” Human-induced landscape pressures are as old as humanity itself. Although there is little doubt that post-Industrial anthropogenic activities have placed more global stress on the environment than for the millions of preceding years, human impacts are not concisely restricted to the post-Industrial world.
Author Contributions
The author confirms being the sole contributor of this work and approved it for publication.
Conflict of Interest Statement
The author declares that the research was conducted in the absence of any commercial or financial relationships that could be construed as a potential conflict of interest.
Acknowledgments
Research that contributed to the creation of this manuscript was funded by the National Research Foundation of Korea Grant funded by the Korean Government (NRF-2013S1A5B6043901). Many thanks to Upinder Sharanjit and Jino Kim, whose stimulating conversations about the Anthropocene provided much of the inspirational juice that fueled the creation of this manuscript. Kristina Dziedzic Wright, Steve Forman, and Jangsuk Kim graciously offered critical comments to an early draft of the manuscript. Valentí Rull and three reviewers provided invaluable constructive and stimulating feedback that greatly improved the quality of this paper and I offer heartfelt thanks for their time.
Supplementary Material
The Supplementary Material for this article can be found online at: http://journal.frontiersin.org/article/10.3389/feart.2017.00004/full#supplementary-material
References
Adkins, J., deMenocal, P., and Eshel, G. (2006). The “African humid period” and the record of marine upwelling from excess 230Th in Ocean Drilling Program Hole 658C. Paleoceanography 21:PA4203. doi: 10.1029/2005PA001200
Amaral, P. G. C., Vincens, A., Guiot, J., Buchet, G., Deschamps, P., Doumnang, J. C., et al. (2013). Palynological evidence for gradual vegetation and climate changes during the African Humid Period termination at 13°N from a Mega-Lake Chad sedimentary sequence. Clim. Past 9, 223–241. doi: 10.5194/cp-9-223-2013
Andersen, T., Carstensen, J., Hernández-García, E., and Duarte, C. M. (2009). Ecological thresholds and regime shifts: approaches to identification. Trends Ecol. Evol. 24, 49–57. doi: 10.1016/j.tree.2008.07.014
Archer, S. (1989). Have southern Texas savannas been converted to woodlands in recent history? Am. Nat. 134, 545–561. doi: 10.1086/284996
Armitage, S. J., Bristow, C. S., and Drake, N. A. (2015). West African monsoon dynamics inferred from abrupt fluctuations of Lake Mega-Chad. Proc. Natl. Acad. Sci. U.S.A. 112, 8543–8548. doi: 10.1073/pnas.1417655112
Aumassip, G. (1984). Le site de Ti-n-Hanakaten et la néolithisation sur les marges orientales du Sahara central. La Néolithisation Sahara 14, 201–203.
Berger, A., and Loutre, M. F. (1991). Insolation values for the climate of the last 10 million years. Quat. Sci. Rev. 10, 297–318. doi: 10.1016/0277-3791(91)90033-Q
Berke, M. A., Johnson, T. C., Werne, J. P., Grice, K., Schouten, S., and Sinninghe Damsté, J. S. (2012). Molecular records of climate variability and vegetation response since the Late Pleistocene in the Lake Victoria basin, East Africa. Quat. Sci. Rev. 55, 59–74. doi: 10.1016/j.quascirev.2012.08.014
Blanchet, C. L., Frank, M., and Schouten, S. (2015). Asynchronous changes in vegetation, runoff and erosion in the Nile River watershed during the Holocene. PLoS ONE 9:e115958. doi: 10.1371/journal.pone.0115958
Bloszies, C., Forman, S. L., and Wright, D. K. (2015). Water level history for Lake Turkana, Kenya in the past 15,000 years and a variable transition from the African Humid Period to Holocene aridity. Glob. Planet. Change 132, 64–76. doi: 10.1016/j.gloplacha.2015.06.006
Boivin, N. L., Zeder, M. A., Fuller, D. Q., Crowther, A., Larson, G., Erlandson, J. M., et al. (2016). Ecological consequences of human niche construction: examining long-term anthropogenic shaping of global species distributions. Proc. Natl. Acad. Sci. U.S.A. 113, 6388–6396. doi: 10.1073/pnas.1525200113
Braconnot, P., Harrison, S. P., Kageyama, M., Bartlein, P. J., Masson-Delmotte, V., Abe-Ouchi, A., et al. (2012). Evaluation of climate models using palaeoclimatic data. Nat. Clim. Change 2, 417–424. doi: 10.1038/nclimate1456
Braconnot, P., Joussaume, S., Marti, O., and de Noblet, N. (1999). Synergistic feedbacks from ocean and vegetation on the African monsoon response to mid-Holocene insolation. Geophys. Res. Lett. 26, 2481–2484. doi: 10.1029/1999GL006047
Braje, T. J., and Erlandson, J. M. (2013). Human acceleration of animal and plant extinctions: a late Pleistocene, Holocene, and Anthropocene continuum. Anthropocene 4, 14–23. doi: 10.1016/j.ancene.2013.08.003
Castañeda, I. S., Schouten, S., Pätzold, J., Lucassen, F., Kasemann, S., Kuhlmann, H., et al. (2016). Hydroclimate variability in the Nile River Basin during the past 28,000 years. Earth Planet. Sci. Lett. 438, 47–56. doi: 10.1016/j.epsl.2015.12.014
Certini, G., and Scalenghe, R. (2015). Holocene as Anthropocene. Science 349:246. doi: 10.1126/science.349.6245.246-a
Claussen, M., and Gayler, V. (1997). The greening of the Sahara during the mid-Holocene: results of an interactive atmosphere-biome model. Glob. Ecol. Biogeogr. Lett. 6, 369–377. doi: 10.2307/2997337
Claussen, M., Bathiany, S., Brovkin, V., and Kleinen, T. (2013). Simulated climate-vegetation interaction in semi-arid regions affected by plant diversity. Nat. Geosci. 6, 954–958. doi: 10.1038/ngeo1962
Claussen, M., Kubatzki, C., Brovkin, V., Ganopolski, A., Hoelzmann, P., and Pachur, H. J. (1999). Simulation of an abrupt change in Saharan vegetation in the mid-Holocene. Geophys. Res. Lett. 26, 2037–2040. doi: 10.1029/1999GL900494
Costa, K., Russell, J., Konecky, B., and Lamb, H. (2014). Isotopic reconstruction of the African Humid Period and Congo Air Boundary migration at Lake Tana, Ethiopia. Quat. Sci. Rev. 83, 58–67. doi: 10.1016/j.quascirev.2013.10.031
Crutzen, P. J., and Stoermer, E. F. (2000). The “Anthropocene.” IGBP Newsl. 41, 17–18. Available online at: http://www.igbp.net/download/18.316f18321323470177580001401/1376383088452/NL41.pdf
Curtin, C. G. (2002). Livestock grazing, rest, and restoration in arid landscapes. Conserv. Biol. 16, 840–842. doi: 10.1046/j.1523-1739.2002.01212.x
de Noblet-Ducoure, N., Claussen, R., and Prentice, C. (2000). Mid-Holocene greening of the Sahara: first results of the GAIM 6000 year BP Experiment with two asynchronously coupled atmosphere/biome models. Clim. Dyn. 16, 643–659. doi: 10.1007/s003820000074
Dekker, S. C., Rietkerk, M. A. X., and Bierkens, M. F. P. (2007). Coupling microscale vegetation–soil water and macroscale vegetation–precipitation feedbacks in semiarid ecosystems. Glob. Chang. Biol. 13, 671–678. doi: 10.1111/j.1365-2486.2007.01327.x
di Lernia, S. (2001). Dismantling dung: delayed use of food resources among early Holocene foragers of the Libyan Sahara. J. Anthropol. Archaeol. 20, 408–441. doi: 10.1006/jaar.2000.0384
di Lernia, S. (2013). “The emergence and spread of herding in northern Africa: critical reappraisal,” in The Oxford Handbook of African Archaeology, eds P. Mitchell and P. J. Lane (Oxford: Oxford University Press), 523–536.
deMenocal, P. B. (2015). Palaeoclimate: end of the African humid period. Nat. Geosci. 8, 86–87. doi: 10.1038/ngeo2355
deMenocal, P., Ortiz, J., Guilderson, T., Adkins, J., Sarnthein, M., Baker, L., et al. (2000). Abrupt onset and termination of the African Humid Period: rapid climate responses to gradual insolation forcing. Quat. Sci. Rev. 19, 347–361. doi: 10.1016/S0277-3791(99)00081-5
Easton, B. (2011). Exercises in New Zealand's demography and economic history. N. Z. Popul. Rev. 37, 173–182. Available online at: http://www.population.org.nz/wp-content/uploads/2012/10/NZPR-Vol-37-Easton.pdf
Ehrmann, W., Schmiedl, G., Seidel, M., Krüger, S., and Schulz, H. (2016). A distal 140 kyr sediment record of Nile discharge and East African monsoon variability. Clim. Past 12, 713–727. doi: 10.5194/cp-12-713-2016
Ellis, E. C. (2011). Anthropogenic transformation of the terrestrial biosphere. Philos. Trans. R. Soc. Lond. A Math. Phys. Eng. Sci. 369, 1010–1035. doi: 10.1098/rsta.2010.0331
Ellis, E. C., Kaplan, J. O., Fuller, D. Q., Vavrus, S., Klein Goldewijk, K., and Verburg, P. H. (2013). Used planet: a global history. Proc. Natl. Acad. Sci. U.S.A. 110, 7978–7985. doi: 10.1073/pnas.1217241110
Fisher, S., Dinshaw, A., McGray, H., Rai, N., and Schaar, J. (2015). Evaluating climate change adaptation: learning from methods in International development. New Dir. Eval. 2015, 13–35. doi: 10.1002/ev.20128
Flenley, J. R., and King, S. M. (1984). Late Quaternary pollen records from Easter Island. Nature 307, 47–50. doi: 10.1038/307047a0
Flenley, J. R., King, A. S. M., Jackson, J., Chew, C., Teller, J. T., and Prentice, M. E. (1991). The Late Quaternary vegetational and climatic history of Easter Island. J. Quat. Sci. 6, 85–115. doi: 10.1002/jqs.3390060202
Foerster, V., Junginger, A., Langkamp, O., Gebru, T., Asrat, A., Umer, M., et al. (2012). Climatic change recorded in the sediments of the Chew Bahir basin, southern Ethiopia, during the last 45,000 years. Quat. Int. 274, 25–37. doi: 10.1016/j.quaint.2012.06.028
Foley, A. J., Coe, T. M., Scheffer, M., and Wang, G. (2003). Regime shifts in the Sahara and Sahel: interactions between ecological and climatic systems in northern Africa. Ecosystems 6, 524–532. doi: 10.1007/s10021-002-0227-0
Foley, S. F., Gronenborn, D., Andreae, M. O., Kadereit, J. W., Esper, J., Scholz, D., et al. (2013). The Palaeoanthropocene – The beginnings of anthropogenic environmental change. Anthropocene 3, 83–88. doi: 10.1016/j.ancene.2013.11.002
Folke, C., Carpenter, S., Walker, B., Scheffer, M., Elmqvist, T., Gunderson, L., et al. (2004). Regime shifts, resilience, and biodiversity in ecosystem management. Annu. Rev. Ecol. Evol. Syst. 35, 557–581. doi: 10.1146/annurev.ecolsys.35.021103.105711
Francus, P., von Suchodoletz, H., Dietze, M., Donner, R. V., Bouchard, F., Roy, A.-J., et al. (2013). Varved sediments of Lake Yoa (Ounianga Kebir, Chad) reveal progressive drying of the Sahara during the last 6100 years. Sedimentology 60, 911–934. doi: 10.1111/j.1365-3091.2012.01370.x
Fuller, D. Q., van Etten, J., Manning, K., Castillo, C., Kingwell-Banham, E., Weisskopf, A., et al. (2011). The contribution of rice agriculture and livestock pastoralism to prehistoric methane levels: an archaeological assessment. Holocene 21, 743–759. doi: 10.1177/0959683611398052
Gabriel, B. (1987). Palaeoecological evidence from Neolithic fireplaces in the Sahara. Afr. Archaeol. Rev. 5, 93–103. doi: 10.1007/BF01117085
Garcin, Y., Junginger, A., Melnick, D., Olago, D. O., Strecker, M. R., and Trauth, M. H. (2009). Late Pleistocene–Holocene rise and collapse of Lake Suguta, northern Kenya Rift. Quat. Sci. Rev. 28, 911–925. doi: 10.1016/j.quascirev.2008.12.006
Garcin, Y., Melnick, D., Strecker, M. R., Olago, D., and Tiercelin, J.-J. (2012). East African mid-Holocene wet-dry transition recorded in palaeo-shorelines of Lake Turkana, northern Kenya Rift. Earth Planet. Sci. Lett. 331–332, 322–334. doi: 10.1016/j.epsl.2012.03.016
Gasse, F. (2000). Hydrological changes in the African tropics since the Last Glacial Maximum. Quat. Sci. Rev. 19, 189–211. doi: 10.1016/S0277-3791(99)00061-X
Gasse, F., and Roberts, C. N. (2004). “Late quaternary hydrologic changes in the arid and semiarid belt of Northern Africa,” in The Hadley Circulation: Present, Past and Future, eds H. F. Diaz and R. S. Bradley (Dordrecht: Springer Netherlands), 313–345.
Gasse, F., and Van Campo, E. (1994). Abrupt post-glacial climate events in West Asia and North Africa monsoon domains. Earth Planet. Sci. Lett. 126, 435–456. doi: 10.1016/0012-821X(94)90123-6
Gatto, M. C., and Zerboni, A. (2015). Holocene supra-regional environmental changes as trigger for major socio-cultural processes in northeastern Africa and the Sahara. Afr. Archaeol. Rev. 32, 301–333. doi: 10.1007/s10437-015-9191-x
Geddes, D. S. (1983). Neolithic transhumance in the Mediterranean Pyrenees. World Archaeol. 15, 51–66. doi: 10.1080/00438243.1983.9979884
Gifford-Gonzalez, D. (2005). “Pastoralism and its consequences,” in African Archaeology: A Critical Introduction, ed A. B. Stahl (Oxford: Blackwell), 187–224.
Gillespie, R., Street-Perrott, F. A., and Switsur, R. (1983). Post-glacial arid episodes in Ethiopia have implications for climate prediction. Nature 306, 680–682. doi: 10.1038/306680a0
Gil-Romera, G., Turton, D., and Sevilla-Callejo, M. (2011). Landscape change in the lower Omo valley, southwestern Ethiopia: burning patterns and woody encroachment in the savanna. J. East. Afr. Stud. 5, 108–128. doi: 10.1080/17531055.2011.544550
Goring-Morris, A. N., and Belfer-Cohen, A. (2011). Neolithization processes in the Levant: the outer envelope. Curr. Anthropol. 52, S195–S208. doi: 10.1086/658860
Grayson, D. K. (2011). The Great Basin: A Natural Prehistory. Berkeley, CA: University of California Press.
Grillo, K. M., and Hildebrand, E. A. (2013). The context of early megalithic architecture in eastern Africa: the Turkana Basin c. 5000-4000 BP. Azania Archaeol. Res. Afr. 48, 193–217. doi: 10.1080/0067270X.2013.789188
Grover, H. D., and Musick, H. B. (1990). Shrubland encroachment in southern New Mexico, U.S.A.: an analysis of desertification processes in the American southwest. Clim. Change 17, 305–330. doi: 10.1007/BF00138373
Hargreaves, J. C., Annan, J. D., Ohgaito, R., Paul, A., and Abe-Ouchi, A. (2013). Skill and reliability of climate model ensembles at the Last Glacial Maximum and mid-Holocene. Clim. Past 9, 811–823. doi: 10.5194/cp-9-811-2013
Harrison, S. P., Bartlein, P. J., Izumi, K., Li, G., Annan, J., Hargreaves, J., et al. (2015). Evaluation of CMIP5 palaeo-simulations to improve climate projections. Nat. Clim. Change 5, 735–743. doi: 10.1038/nclimate2649
Haynes, C. V., Eyles, C. H., Pavlish, L. A., Ritchie, J. C., and Rybak, M. (1989). Holocene palaeoecology of the eastern Sahara; Selima Oasis. Quat. Sci. Rev. 8, 109–136. doi: 10.1016/0277-3791(89)90001-2
He, F., Vavrus, S. J., Kutzbach, J. E., Ruddiman, W. F., Kaplan, J. O., and Krumhardt, K. M. (2014). Simulating global and local surface temperature changes due to Holocene anthropogenic land cover change. Geophys. Res. Lett. 41, 623–631. doi: 10.1002/2013GL058085
Hély, C., Braconnot, P., Watrin, J., and Zheng, W. (2009). Climate and vegetation: simulating the African humid period. C. R. Geosci. 341, 671–688. doi: 10.1016/j.crte.2009.07.002
Hély, C., Lézine, A. M., and APD contributors (2014). Holocene changes in African vegetation: tradeoff between climate and water availability. Clim. Past 10, 681–686. doi: 10.5194/cp-10-681-2014
Hennekam, R., Jilbert, T., Schnetger, B., and de Lange, G. J. (2014). Solar forcing of Nile discharge and sapropel S1 formation in the early to middle Holocene eastern Mediterranean. Paleoceanography 29, 343–356. doi: 10.1002/2013PA002553
Hoelzmann, P., Keding, B., Berke, H., Kropelin, S., and Kruse, H. J. (2001). Environmental change and archaeology: lake evolution and human occupation in the eastern Sahara during the Holocene. Palaeogeogr. Palaeoclimatol. Palaeoecol. 169, 193–217. doi: 10.1016/S0031-0182(01)00211-5
Holl, A. F. C. (2012). Dhar Tichitt, Walata et Nema. Les Nouv. l'Archéol. 127, 35–39. doi: 10.4000/nda.1584
Honegger, M., and Williams, M. (2015). Human occupations and environmental changes in the Nile valley during the Holocene: the case of Kerma in Upper Nubia (northern Sudan). Quat. Sci. Rev. 130, 141–154. doi: 10.1016/j.quascirev.2015.06.031
Hunt, T. L. (2007). Rethinking Easter Island's ecological catastrophe. J. Archaeol. Sci. 34, 485–502. doi: 10.1016/j.jas.2006.10.003
Hunt, T. L., and Lipo, C. P. (2006). Late colonization of Easter Island. Science 311, 1603–1606. doi: 10.1126/science.1121879
Huysecom, E., Ozainne, S., Raeli, F., Ballouche, A., Rasse, M., and Stokes, S. (2004). Ounjougou (Mali): a history of Holocene settlement at the southern edge of the Sahara. Antiquity 78, 579–593. doi: 10.1017/S0003598X00113237
Innes, J. B., Blackford, J. J., and Rowley-Conwy, P. A. (2013). Late Mesolithic and early Neolithic forest disturbance: a high resolution palaeoecological test of human impact hypotheses. Quat. Sci. Rev. 77, 80–100. doi: 10.1016/j.quascirev.2013.07.012
Johnstone, J. F., Allen, C. D., Franklin, J. F., Frelich, L. E., Harvey, B. J., Higuera, P. E., et al. (2016). Changing disturbance regimes, ecological memory, and forest resilience. Front. Ecol. Environ. 14, 369–378. doi: 10.1002/fee.1311
Jolly, D., Harrison, S. P., Damnati, B., and Bonnefille, R. (1998). Simulated climate and biomes of Africa during the late quaternary: comparison with pollen and lake status data. Quat. Sci. Rev. 17, 629–657. doi: 10.1016/S0277-3791(98)00015-8
Jones, A. (2000). Effects of cattle grazing on North American arid ecosystems: a quantitative review. West. North Am. Nat. 60, 155–164. Available online at: http://scholarsarchive.byu.edu/wnan/vol60/iss2/5
Junginger, A., Roller, S., Olaka, L. A., and Trauth, M. H. (2014). The effects of solar irradiation changes on the migration of the Congo Air Boundary and water levels of paleo-Lake Suguta, Northern Kenya Rift, during the African Humid Period (15–5 ka BP). Palaeogeogr. Palaeoclimatol. Palaeoecol. 396, 1–16. doi: 10.1016/j.palaeo.2013.12.007
Kershaw, A. P., Bush, M. B., Hope, G. S., Weiss, K.-F., Goldammer, J. G., and Sanford, R. (1997). “The contribution of humans to past biomass burning in the tropics,” in Sediment Records of Biomass Burning and Global Change, eds J. S. Clark, H. Cachier, J. G. Goldammer, and B. Stocks (Berlin; Heidelberg: Springer), 413–442.
Kiage, L. M., and Liu, K.-B. (2006). Late Quaternary paleoenvironmental changes in East Africa: a review of multiproxy evidence from palynology, lake sediments, and associated records. Prog. Phys. Geogr. 30, 633–658. doi: 10.1177/0309133306071146
Kim, J. (2014). From labour control to surplus appropriation: landscape changes in the Neolithization of southwestern Korea. J. World Prehist. 27, 263–275. doi: 10.1007/s10963-014-9076-y
King, C. M., Innes, J. G., Smale, M. C., and Nugent, G. (2015). “Protecting the forest from introduced herbivores,” in The Drama of Conservation: The History of Pureora Forest, New Zealand, eds M. C. King, J. D. Gaukrodger, and A. N. Ritchie (Cham: Springer International Publishing), 245–273.
Kinzig, A. P., Ryan, P. A., Etienne, M., Allison, H. E., Elmqvist, T., and Walker, B. H. (2006). Resilience and regime shifts: assessing cascading effects. Ecol. Soc. 11, 20. doi: 10.5751/ES-01678-110120
Kröpelin, S., Verschuren, D., Lézine, A.-M., Eggermont, H., Cocquyt, C., Francus, P., et al. (2008). Climate-driven ecosystem succession in the Sahara: the past 6000 years. Science 320, 765–768. doi: 10.1126/science.1154913
Kuper, R., and Kröpelin, S. (2006). Climate-controlled Holocene occupation in the Sahara: motor of Africa's evolution. Science 313, 803–807. doi: 10.1126/science.1130989
Kutzbach, J. E., Bartlein, P. J., Foley, J. A., Harrison, S. P., Hostetler, S. W., Liu, Z., et al. (1996). Potential role of vegetation feedback in the climate sensitivity of high-latitude regions: a case study at 6000 years BP. Glob. Biogeochem. Cycles 10, 727–736. doi: 10.1029/96GB02690
Kuzmin, Y. V., and Orlova, L. A. (2000). The Neolithization of Siberia and the Russian Far East: radiocarbon evidence. Antiquity 74, 356–364. doi: 10.1017/S0003598X00059433
Lal, R. (2012). Climate change and soil degradation mitigation by sustainable management of soils and other natural resources. Agric. Res. 1, 199–212. doi: 10.1007/s40003-012-0031-9
Lamb, A. L., Leng, M. J., Mohammed, M. U., and Lamb, H. F. (2004). Holocene climate and vegetation change in the Main Ethiopian Rift Valley, inferred from the composition (C/N and delta C-13) of lacustrine organic matter. Quat. Sci. Rev. 23, 881–891. doi: 10.1016/j.quascirev.2003.06.010
Lane, P. (2013). “Trajectories of pastoralism in northern and central Kenya: an overview of the archaeological and environmental evidence,” in Pastoralism in Afirca: Past, Present, and Future, eds M. Bollig, M. Schnegg, and H.-P. Wotzka (New York, NY: Berghahn Books), 104–144.
Lare, A. R., and Nicholson, S. E. (1994). Contrasting conditions of surface water balance in wet years and dry years as a possible land surface-atmosphere feedback mechanism in the West African Sahel. J. Clim. 7, 653–668. doi: 10.1175/1520-0442(1994)007<0653:CCOSWB>2.0.CO;2
Larrasoaña, J. C., Roberts, A. P., and Rohling, E. J. (2013). Dynamics of green Sahara periods and their role in hominin evolution. PLoS ONE 8:e76514. doi: 10.1371/journal.pone.0076514
Laycock, W. A. (1991). Stable states and thresholds of range condition on North American rangelands: a viewpoint. J. Range Manag. 44, 427–433. doi: 10.2307/4002738
Lécuyer, C., Lézine, A.-M., Fourel, F., Gasse, F., Sylvestre, F., Pailles, C., et al. (2016). I-n-Atei palaeolake documents past environmental changes in central Sahara at the time of the “Green Sahara”: Charcoal, carbon isotope and diatom records. Palaeogeogr. Palaeoclimatol. Palaeoecol. 441(Pt 4), 834–844. doi: 10.1016/j.palaeo.2015.10.032
Lesur, J., Hildebrand, E. A., Abawa, G., and Gutherz, X. (2014). The advent of herding in the Horn of Africa: new data from Ethiopia, Djibouti and Somaliland. Quat. Int. 343, 148–158. doi: 10.1016/j.quaint.2013.11.024
Levis, S., Bonan, G. B., and Bonfils, C. (2004). Soil feedback drives the mid-Holocene North African monsoon northward in fully coupled CCSM2 simulations with a dynamic vegetation model. Clim. Dyn. 23, 791–802. doi: 10.1007/s00382-004-0477-y
Lézine, A. M., Zheng, W., Braconnot, P., and Krinner, G. (2011b). Late Holocene plant and climate evolution at Lake Yoa, northern Chad: pollen data and climate simulations. Clim. Past 7, 1351–1362. doi: 10.5194/cp-7-1351-2011
Lézine, A.-M. (2009). Timing of vegetation changes at the end of the Holocene Humid Period in desert areas at the northern edge of the Atlantic and Indian monsoon systems. C. R. Geosci. 341, 750–759. doi: 10.1016/j.crte.2009.01.001
Lézine, A.-M., Duplessy, J.-C., and Cazet, J.-P. (2005). West African monsoon variability during the last deglaciation and the Holocene: evidence from fresh water algae, pollen and isotope data from core KW31, Gulf of Guinea. Palaeogeogr. Palaeoclimatol. Palaeoecol. 219, 225–237. doi: 10.1016/j.palaeo.2004.12.027
Lézine, A.-M., Hély, C., Grenier, C., Braconnot, P., and Krinner, G. (2011a). Sahara and Sahel vulnerability to climate changes, lessons from Holocene hydrological data. Quat. Sci. Rev. 30, 3001–3012. doi: 10.1016/j.quascirev.2011.07.006
Li, A., Wu, J., and Huang, J. (2012). Distinguishing between human-induced and climate-driven vegetation changes: a critical application of RESTREND in inner Mongolia. Landsc. Ecol. 27, 969–982. doi: 10.1007/s10980-012-9751-2
Lindenmayer, D. B., and Likens, G. E. (2009). Adaptive monitoring: a new paradigm for long-term research and monitoring. Trends Ecol. Evol. 24, 482–486. doi: 10.1016/j.tree.2009.03.005
Linseele, V., Van Neer, W., Thys, S., Phillipps, R., Cappers, R., Wendrich, W., et al. (2014). New archaeozoological data from the fayum “Neolithic” with a critical assessment of the evidence for early stock keeping in Egypt. PLoS ONE 9:e108517. doi: 10.1371/journal.pone.0108517
Linstädter, J., Kehl, M., Broich, M., and López-Sáez, J. A. (2016). Chronostratigraphy, site formation processes and pollen record of Ifri n'Etsedda, NE Morocco. Quat. Int. 410(Pt A), 6–29. doi: 10.1016/j.quaint.2015.11.017
Liu, Z., Otto-Bliesner, B., Kutzbach, J., Li, L., and Shields, C. (2003). Coupled Climate Simulation of the evolution of global monsoons in the Holocene. J. Clim. 16, 2472–2490. doi: 10.1175/1520-0442(2003)016<2472:CCSOTE>2.0.CO;2
Liu, Z., Wang, Y., Gallimore, R., Gasse, F., Johnson, T. C., deMenocal, P., et al. (2007). Simulating the transient evolution and abrupt change of northern Africa atmosphere-ocean-terrestrial ecosystem in the Holocene. Quat. Sci. Rev. 26, 1818–1837. doi: 10.1016/j.quascirev.2007.03.002
Loeser, M. R. R., Sisk, T. D., and Crews, T. E. (2007). Impact of grazing intensity during drought in an Arizona grassland. Cons. Biol. 21, 87–97. doi: 10.1111/j.1523-1739.2006.00606.x
Lyons, S. K., Amatangelo, K. L., Behrensmeyer, A. K., Bercovici, A., Blois, J. L., Davis, M., et al. (2016). Holocene shifts in the assembly of plant and animal communities implicate human impacts. Nature 529, 80–83. doi: 10.1038/nature16447
MacEachern, S. (2012). The Holocene history of the southern Lake Chad Basin: archaeological, linguistic and genetic evidence. Afr. Archaeol. Rev. 29, 253–271. doi: 10.1007/s10437-012-9110-3
Magurran, A. E., Baillie, S. R., Buckland, S. T., Dick, J. M., Elston, D. A., Scott, E. M., et al. (2010). Long-term datasets in biodiversity research and monitoring: assessing change in ecological communities through time. Trends Ecol. Evol. 25, 574–582. doi: 10.1016/j.tree.2010.06.016
Manning, K., and Timpson, A. (2014). The demographic response to Holocene climate change in the Sahara. Quat. Sci. Rev. 101, 28–35. doi: 10.1016/j.quascirev.2014.07.003
Marlon, J. R., Bartlein, P. J., Daniau, A.-L., Harrison, S. P., Maezumi, S. Y., Power, M. J., et al. (2013). Global biomass burning: a synthesis and review of Holocene paleofire records and their controls. Quat. Sci. Rev. 65, 5–25. doi: 10.1016/j.quascirev.2012.11.029
Marshall, F., and Hildebrand, E. (2002). Cattle before crops: the beginnings of food production in Africa. J. World Prehist. 16, 99–143. doi: 10.1023/A:1019954903395
Marshall, M. H., Lamb, H. F., Huws, D., Davies, S. J., Bates, R., Bloemendal, J., et al. (2011). Late Pleistocene and Holocene drought events at Lake Tana, the source of the Blue Nile. Glob. Planet. Change 78, 147–161. doi: 10.1016/j.gloplacha.2011.06.004
McDonald, M. M. A. (2016). The pattern of Neolithization in Dakhleh Oasis in the Eastern Sahara. Quat. Int. 410, 181–197. doi: 10.1016/j.quaint.2015.10.100
McGlone, M. S. (2009). Postglacial history of New Zealand wetlands and implications for their conservation. N. Z. J. Ecol. 33, 1–23. Available online at: http://www.jstor.org/stable/24060858
Miller, R. F., and Jeffrey, A. R. (1999). Fire history and western juniper encroachment in sagebrush steppe. J. Range Manag. 52, 550–559. doi: 10.2307/4003623
Moleele, N. M., Ringrose, S., Matheson, W., and Vanderpost, C. (2002). More woody plants? the status of bush encroachment in Botswana's grazing areas. J. Environ. Manag. 64, 3–11. doi: 10.1006/jema.2001.0486
Morales, J., Pérez-Jordà, G., Peña-Chocarro, L., Zapata, L., Ruíz-Alonso, M., López-Sáez, J. A., et al. (2013). The origins of agriculture in North-West Africa: macro-botanical remains from Epipalaeolithic and Early Neolithic levels of Ifri Oudadane (Morocco). J. Archaeol. Sci. 40, 2659–2669. doi: 10.1016/j.jas.2013.01.026
Morris, L. R., and Rowe, R. J. (2014). Historical land use and altered habitats in the Great Basin. J. Mammal. 95, 1144–1156. doi: 10.1644/13-MAMM-S-169
Müller, F., Baessler, C., Schubert, H., and Klotz, S. (2010). Long-Term Ecological Research. Berlin: Springer.
Müller, F., Bergmann, M., Dannowski, R., Dippner, J. W., Gnauck, A., Haase, P., et al. (2016). Assessing resilience in long-term ecological data sets. Ecol. Indic. 65, 10–43. doi: 10.1016/j.ecolind.2015.10.066
Muradian, R. (2001). Ecological thresholds: a survey. Ecol. Econ. 38, 7–24. doi: 10.1016/S0921-8009(01)00146-X
Naveh, Z. (1987). “Landscape ecology, management and conservation of European and Levant Mediterranean uplands,” in Plant Response to Stress: Functional Analysis in Mediterranean Ecosystems, eds J. D. Tenhunen, F. M. Catarino, O. L. Lange, and W. C. Oechel (Berlin; Heidelberg: Springer), 641–657.
Neumann, K. (1989). Holocene vegetation of the Eastern Sahara: charcoal from prehistoric sites. Afr. Archaeol. Rev. 7, 97–116. doi: 10.1007/BF01116839
Nicholson, S. E. (2015). Evolution and current state of our understanding of the role played in the climate system by land surface processes in semi-arid regions. Glob. Planet. Change 133, 201–222. doi: 10.1016/j.gloplacha.2015.08.010
Niedermeyer, E. M., Schefuß, E., Sessions, A. L., Mulitza, S., Mollenhauer, G., Schulz, M., et al. (2010). Orbital- and millennial-scale changes in the hydrologic cycle and vegetation in the western African Sahel: insights from individual plant wax δD and δ13C. Quat. Sci. Rev. 29, 2996–3005. doi: 10.1016/j.quascirev.2010.06.039
Nutz, A., and Schuster, M. (2016). Stepwise drying of Lake Turkana at the end of the African Humid Period: a forced regression modulated by solar activity variations? Solid Earth 7, 1609–1618. doi: 10.5194/se-7-1609-2016
Ozainne, S. (2014). “West and Central African Neolithic: geography and overview,” in Encyclopedia of Global Archaeology, ed C. Smith (New York, NY: Springer), 7744–7759.
Pardini, R., Bueno, A. d. A., Gardner, T. A., Prado, P. I., and Metzger, J. P. (2010). Beyond the fragmentation threshold hypothesis: regime shifts in biodiversity across fragmented landscapes. PLoS ONE 5:e13666. doi: 10.1371/journal.pone.0013666
Parsons, M., and Nalau, J. (2016). Historical analogies as tools in understanding transformation. Glob. Environ. Change 38, 82–96. doi: 10.1016/j.gloenvcha.2016.01.010
Pausas, J. G., and Keeley, J. E. (2014). Abrupt climate-independent fire regime changes. Ecosystems 17, 1109–1120. doi: 10.1007/s10021-014-9773-5
Pausata, F. S. R., Messori, G., and Zhang, Q. (2016). Impacts of dust reduction on the northward expansion of the African monsoon during the Green Sahara period. Earth Planet. Sci. Lett. 434, 298–307. doi: 10.1016/j.epsl.2015.11.049
Peden, R. (2011). “Pastoralism and the transformation of the open grassland,” in Seeds of Empire: The Environmental Transformation of New Zealand, eds T. Brooking, P. Star, and E. Pawson (London: I.B.Tauris), 73–93.
Prendergast, M. E. (2010). Diversity in East African foraging and food producing communities. Azania Archaeol. Res. Afr. 45, 1–5. doi: 10.1080/00672700903291674
Prentice, I. C., and Jolly, D. (2000). Mid-Holocene and glacial-maximum vegetation geography of the northern continents and Africa. J. Biogeogr. 27, 507–519. doi: 10.1046/j.1365-2699.2000.00425.x
Rainbird, P. (2002). A message for our future? The Rapa Nui (Easter Island) ecodisaster and Pacific island environments. World Archaeol. 33, 436–451. doi: 10.1080/00438240120107468
Ramankutty, N., and Foley, J. A. (1999). Estimating historical changes in land cover:North American croplands from 1850 to 1992. Glob. Ecol. Biogeogr. 8, 381–396. doi: 10.1046/j.1365-2699.1999.00141.x
Renssen, H., Brovkin, V., Fichefet, T., and Goosse, H. (2006). Simulation of the Holocene climate evolution in Northern Africa: the termination of the African humid period. Quat. Int. 150, 95–102. doi: 10.1016/j.quaint.2005.01.001
Renssen, H., Seppä, H., Crosta, X., Goosse, H., and Roche, D. M. (2012). Global characterization of the Holocene thermal maximum. Quat. Sci. Rev. 48, 7–19. doi: 10.1016/j.quascirev.2012.05.022
Rietkerk, M., Brovkin, V., van Bodegom, P. M., Claussen, M., Dekker, S. C., Dijkstra, H. A., et al. (2011). Local ecosystem feedbacks and critical transitions in the climate. Ecol. Complexity 8, 223–228. doi: 10.1016/j.ecocom.2011.03.001
Rispoli, F. (2007). The incised & impressed pottery style of mainland Southeast Asia: following the paths of Neolithization. East West 57, 235–304. Available online at: http://www.jstor.org/stable/29757730
Rull, V. (2014). Time continuum and true long-term ecology: from theory to practice. Front. Ecol. Evol. 2:75. doi: 10.3389/fevo.2014.00075
Salzmann, U., and Hoelzmann, P. (2005). The Dahomey Gap: an abrupt climatically induced rain forest fragmentation in West Africa during the late Holocene. Holocene 15, 190–199. doi: 10.1191/0959683605hl799rp
Salzmann, U., and Waller, M. (1998). The Holocene vegetational history of the Nigerian Sahel based on multiple pollen profiles. Rev. Palaeobot. Palynol. 100, 39–72. doi: 10.1016/S0034-6667(97)00053-5
Salzmann, U., Hoelzmann, P., and Morczinek, I. (2002). Late Quaternary climate and vegetation of the Sudanian zone of northeast Nigeria. Quat. Res. 58, 73–83. doi: 10.1006/qres.2002.2356
Scheffer, M., and Carpenter, S. R. (2003). Catastrophic regime shifts in ecosystems: linking theory to observation. Trends Ecol. Evol. 18, 648–656. doi: 10.1016/j.tree.2003.09.002
Schefuß, E., Werner, M., Beckmann, B., Haese, B., and Lohmann, G. (2015). “North-West African hydrologic changes in the Holocene: a combined isotopic data and model approach,” in Integrated Analysis of Interglacial Climate Dynamics (INTERDYNAMIC), eds M. Schulz and A. Paul (Cham, Switzerland: Springer International Publishing), 109–114.
Schlütz, F., and Lehmkuhl, F. (2009). Holocene climatic change and the nomadic Anthropocene in Eastern Tibet: palynological and geomorphological results from the Nianbaoyeze Mountains. Quat. Sci. Rev. 28, 1449–1471. doi: 10.1016/j.quascirev.2009.01.009
Schüle, W. (1990). “Landscapes and climate in prehistory: interactions of wildlife, man, and fire,” in Fire in the Tropical Biota: Ecosystem Processes and Global Challenges, ed J. G. Goldammer (Berlin; Heidelberg: Springer), 273–318.
Schulz, E. (1994). The southern limit of the Mediterranean vegetation in the Sahara during the Holocene. Hist. Biol. 9, 137–156. doi: 10.1080/10292389409380495
Shanahan, T. M., McKay, N. P., Hughen, K. A., Overpeck, J. T., Otto-Bliesner, B., Heil, C. W., et al. (2015). The time-transgressive termination of the African Humid Period. Nat. Geosci. 8, 140–144. doi: 10.1038/ngeo2329
Skinner, C. B., and Poulsen, C. J. (2016). The role of fall season tropical plumes in enhancing Saharan rainfall during the African Humid Period. Geophys. Res. Lett. 43, 349–358. doi: 10.1002/2015GL066318
Skonieczny, C., Paillou, P., Bory, A., Bayon, G., Biscara, L., Crosta, X., et al. (2015). African humid periods triggered the reactivation of a large river system in Western Sahara. Nat. Commun. 6:8751. doi: 10.1038/ncomms9751
Smil, V. (2000). Phosporus in the environment: natural flows and human interferences. Ann. Rev. Ener. Environ. 25, 53–88. doi: 10.1146/annurev.energy.25.1.53
Smith, A. B. (1992). Pastoralism in Africa: Origins and Development Ecology. London: Hurst & Company.
Smith, B. D. (2011). General patterns of niche construction and the management of ‘wild’ plant and animal resources by small-scale pre-industrial societies. Philos. Trans. R. Soc. B Biol. Sci. 366, 836–848. doi: 10.1098/rstb.2010.0253
Steffen, W., Broadgate, W., Deutsch, L., Gaffney, O., and Ludwig, C. (2015). The trajectory of the Anthropocene: the Great Acceleration. Anthropocene Rev. 2, 81–98. doi: 10.1177/2053019614564785
Stewart, K. M. (1989). Fishing Sites of North and East African in the Late Pleistocene and Holocene: Environmental Change and Adaptation. Oxford: BAR International Series 521.
Tafuri, M. A., Bentley, R. A., Manzi, G., and di Lernia, S. (2006). Mobility and kinship in the prehistoric Sahara: strontium isotope analysis of Holocene human skeletons from the Acacus Mts. (southwestern Libya). J. Anthropol. Archaeol. 25, 390–402. doi: 10.1016/j.jaa.2006.01.002
Terborgh, J., and Estes, J. A. (2013). Trophic Cascades: Predators, Prey, and the Changing Dynamics of Nature. Washington, DC: Island Press.
Tierney, J. E., and deMenocal, P. B. (2013). Abrupt shifts in Horn of Africa hydroclimate since the Last Glacial Maximum. Science 342, 843–846. doi: 10.1126/science.1240411
Tierney, J. E., Lewis, S. C., Cook, B. I., LeGrande, A. N., and Schmidt, G. A. (2011). Model, proxy and isotopic perspectives on the East African Humid Period. Earth Planet. Sci. Lett. 307, 103–112. doi: 10.1016/j.epsl.2011.04.038
Tylianakis, J. M., and Coux, C. (2014). Tipping points in ecological networks. Trends Plant Sci. 19, 281–283. doi: 10.1016/j.tplants.2014.03.006
Umer, M., Lamb, H. F., Bonnefille, R., Lézine, A. M., Tiercelin, J. J., Gibert, E., et al. (2007). Late Pleistocene and Holocene vegetation history of the Bale Mountains, Ethiopia. Quat. Sci. Rev. 26, 2229–2246. doi: 10.1016/j.quascirev.2007.05.004
Van Auken, O. W. (2000). Shrub invasions of North American semiarid grasslands. Annu. Rev. Ecol. Syst. 31, 197–215. doi: 10.1146/annurev.ecolsys.31.1.197
Van Neer, W. (2002). “Food security in western and central Africa during the Late Holocene: the role of domestic stock keeping, hunting and fishing,” in Droughts, Food and Culture: Ecological Change and Food Security in Africa's Later Prehistory, ed F. A. Hassan (New York, NY: Kluwer), 251–274.
Vincens, A., Buchet, G., and Servant, M. (2010). Vegetation response to the “African Humid Period” termination in Central Cameroon (7° N)–new pollen insight from Lake Mbalang. Clim. Past 6, 281–294. doi: 10.5194/cp-6-281-2010
Walker, B., and Meyers, J. A. (2004). Thresholds in ecological and socialecological systems: a developing database. Ecol. Soc. 9, 3. doi: 10.5751/ES-00664-090203
Wanner, H., Beer, J., Bütikofer, J., Crowley, T. J., Cubasch, U., Flückiger, J., et al. (2008). Mid- to Late Holocene climate change: an overview. Quat. Sci. Rev. 27, 1791–1828. doi: 10.1016/j.quascirev.2008.06.013
Watrin, J., Lézine, A.-M., and Hély, C. (2009). Plant migration and plant communities at the time of the “green Sahara.” C. R. Geosci. 341, 656–670. doi: 10.1016/j.crte.2009.06.007
Weldeab, S., Schneider, R. R., Kölling, M., and Wefer, G. (2005). Holocene African droughts relate to eastern equatorial Atlantic cooling. Geology 33, 981–984. doi: 10.1130/G21874.1
Wendorf, F., and Schild, R. (1998). Nabta Playa and its role in northeastern African prehistory. J. Anthropol. Archaeol. 17, 97–123. doi: 10.1006/jaar.1998.0319
Whitehead, A. L., Byrom, A. E., Clayton, R. I., and Pech, R. P. (2014). Removal of livestock alters native plant and invasive mammal communities in a dry grassland–shrubland ecosystem. Biol. Invasions 16, 1105–1118. doi: 10.1007/s10530-013-0565-1
Woodburne, M. O. (2012). Late Cretaceous and Cenozoic Mammals of North America: Biostratigraphy and Geochronology. New York, NY: Columbia University Press.
Wright, D. K. (2007). Tethered mobility and riparian resource exploitation among Neolithic hunters and herders in the Galana River Basin, Kenyan Coastal Lowlands. Environ. Archaeol. 12, 25–47. doi: 10.1179/174963107x172732
Wright, D. K. (2014). “East and Southern African Neolithic: geography and overview,” in Encyclopedia of Global Archaeology, ed C. Smith (New York, NY: Springer), 2281–2298.
Wright, D. K., Forman, S. L., Kiura, P., Bloszies, C., and Beyin, A. (2015). Lakeside view: Sociocultural responses to changing water levels of Lake Turkana, Kenya. Afr. Archaeol. Rev. 32, 335–367. doi: 10.1007/s10437-015-9185-8
Yerkes, R. W., Khalaily, H., and Barkai, R. (2012). Form and function of early Neolithic bifacial stone tools reflects changes in land use practices during the Neolithization process in the Levant. PLoS ONE 7:e42442. doi: 10.1371/journal.pone.0042442
Keywords: African humid period, neolithization, regime shifts, land-atmospheric interactions, human-environmental systems, animal husbandry, long anthropocene
Citation: Wright DK (2017) Humans as Agents in the Termination of the African Humid Period. Front. Earth Sci. 5:4. doi: 10.3389/feart.2017.00004
Received: 17 October 2016; Accepted: 11 January 2017;
Published: 26 January 2017.
Edited by:
Valentí Rull, Institute of Earth Sciences Jaume Almera-CSIC, SpainReviewed by:
Richard ‘Bert’ Roberts, University of Wollongong, AustraliaRob Marchannt, University of York, UK
Graciela Gil-Romera, Instituto Pirenaico de Ecología, Spain
Copyright © 2017 Wright. This is an open-access article distributed under the terms of the Creative Commons Attribution License (CC BY). The use, distribution or reproduction in other forums is permitted, provided the original author(s) or licensor are credited and that the original publication in this journal is cited, in accordance with accepted academic practice. No use, distribution or reproduction is permitted which does not comply with these terms.
*Correspondence: David K. Wright, msafiri@snu.ac.kr