- 1Department of Earth Sciences, ETH Zurich, Zurich, Switzerland
- 2Department of Biological, Geological and Environmental Sciences, Bologna University, Bologna, Italy
- 3Department of Geological Sciences, Jackson School of Geosciences, The University of Texas at Austin, Austin, TX, USA
- 4Comité Espeleológico de Matanzas, Sociedad Espeleológica de Cuba, Matanzas, Cuba
- 5Associazione di Esplorazioni Geografiche la Venta, Treviso, Italy
Unusual speleothems resembling giant mushrooms occur in Cueva Grande de Santa Catalina, Cuba. Although these mineral buildups are considered a natural heritage, their composition and formation mechanism remain poorly understood. Here we characterize their morphology and mineralogy and present a model for their genesis. We propose that the mushrooms, which are mainly comprised of calcite and aragonite, formed during four different phases within an evolving cave environment. The stipe of the mushroom is an assemblage of three well-known speleothems: a stalagmite surrounded by calcite rafts that were subsequently encrusted by cave clouds (mammillaries). More peculiar is the cap of the mushroom, which is morphologically similar to cerebroid stromatolites and thrombolites of microbial origin occurring in marine environments. Scanning electron microscopy (SEM) investigations of this last unit revealed the presence of fossilized extracellular polymeric substances (EPS)—the constituents of biofilms and microbial mats. These organic microstructures are mineralized with Ca-carbonate, suggesting that the mushroom cap formed through a microbially-influenced mineralization process. The existence of cerebroid Ca-carbonate buildups forming in dark caves (i.e., in the absence of phototrophs) has interesting implications for the study of fossil microbialites preserved in ancient rocks, which are today considered as one of the earliest evidence for life on Earth.
Introduction
Santa Catalina Cave (Cueva Grande de Santa Catalina) in Cuba hosts unusual mineral structures whose morphology is similar to that of giant mushrooms (Figures 1A,B). These curious and unique speleothems have also been found in another Cuban cave on the Cayo Caguanes island (Nuñez Jimenez, 1962, 1973), but in Santa Catalina Cave they reach dimensions and numbers by far more important. Although these speleothems have been well-known by speleologists for decades, and Santa Catalina has been declared “National Monument” in 1996 (Watson et al., 1997; D'Angeli et al., 2015), the exact mechanism of their formation, as well as their petrography and mineralogy, remain virtually unstudied. Besides representing a yet uncharacterized type of speleothem, they are of utmost interest due to their uppermost unit (i.e., the mushroom cap), which is similar to stromatolitic and thrombolitic microbialites that form in the presence of microbial mats (Figures 1A,B; Jahnert and Collins, 2012; Bosak et al., 2013). Microbialites occur in a large variety of distinctive morphologies and are found in the geological record from the early Archean onwards (Grotzinger and Knoll, 1999). Today, they are considered as one of the oldest macroscopic evidence for the existence of life on Earth (Allwood et al., 2006). The mechanisms controlling their genesis have been extensively studied, trying to shed light on the evolution of early metabolisms (Allwood et al., 2010; Bontognali et al., 2012). Although most microbialite studies focus on processes occurring in the photic zone of marine and lacustrine environments (Bosak et al., 2013), a growing number of works also deals with biomineralization processes that take place in subsurface environments (Jones, 2001, 2011; Melim et al., 2001; Olivier et al., 2003; Baskar et al., 2007, 2011, 2014; Blyth and Frisia, 2008; Lisker et al., 2009; Rossi et al., 2010; Florea et al., 2011; Lundberg and McFarlane, 2011; Gradzíński et al., 2012; Lozano and Rossi, 2012; Léveillé et al., 2000; Pacton et al., 2013; Tisato et al., 2015). It has often been hypothesized that life originated in the dark, protected from the strong UV radiation that characterized the surface environments of the early Earth and other planets (Homann et al., 2016). For this reason, caves—such as Santa Catalina—represent ideal locations for the study of primitive microbe-mineral interactions.
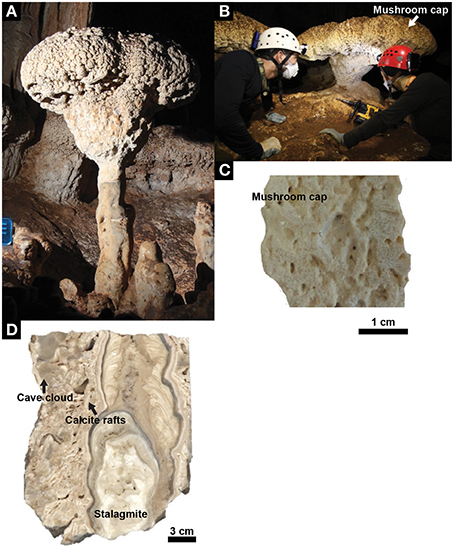
Figure 1. Mushroom speleothem. (A) A representative mushroom in Santa Catalina Cave, Cuba. (B) Mushroom speleothem from where the core samples used for laboratory analyses were drilled. (C) Cross section showing the internal structure of the mushroom cap. (D) Cross section showing the internal structure of the different units that, together with the mushroom cap, constitute the speleothem (i.e., stalagmite, calcite rafts, cave cloud).
Here, we propose a model that reconstructs the paleo-environments and the processes that led to the formation of the Santa Catalina mushroom-shaped speleothems. As we found evidence suggesting that microbes took part in their formation, we discuss the implications that our study has for interpreting ancient microbialites.
Geological Setting, Sampling and Methods
Santa Catalina Cave is located on the northern coast of Cuba, ~20 km east from Matanzas. The regional geology surrounding the cave is comprised of a series of marine terraces composed of eogenetic limestones dated from Pliocene to present, covering pre-Cretaceous ophiolites (locally rich in chalcopyrite) forming the basement rocks (Ducloz, 1963). The cave opens on the Yucayo terrace (lower Pleistocene age) at around 20 m a.s.l. and <1 km from the present coastline. The cave is carved in the fossiliferous reef limestones of the Vedado Formation of Late Pliocene-Early Pleistocene age (Cabrera and Penãlver, 2001) and comprises more than 10 km of galleries that develop on three dry sub-horizontal levels. The uppermost level, which can be classified as a flank margin cave (Mylroie and Carew, 1990), hosts more than 100 mushroom-shaped speleothems (D'Angeli et al., 2015), located ~16 m above present-day sea- and water-table level. Due to the presence of some military infrastructures in broadly the same region of the studied area, we are not authorized to report the exact location and topographic survey of the cave. The distribution and macroscopic morphology of the mushrooms were documented during several expeditions that took place in December 2012. Laboratory analyses were performed on three short (~6 cm) cores drilled from a representative mushroom speleothem (e.g., Figure 1C), an additional sample of a mushroom cap, and a naturally broken piece of a mushroom stipe (Figure 1D). Thin sections were prepared for petrographic investigations. Scanning electron microscopy (SEM) analysis were performed on freshly broken, ethanol cleaned subsamples with a Zeiss Supra 50 VP equipped with an energy dispersive X-ray detector for element analysis (EDX). SEM images and EDX analysis were obtained with a secondary electron detector, applying an accelerating voltage of 10 kV, and a working distance of 9 mm. Powders for stable isotope analyses were produced with a dental drill. The isotopic composition of carbonate was measured with a Gasbench II coupled with a Delta V mass spectrometer (both ThermoFisher Scientific) using the method described in Breitenbach and Bernasconi (2011).
Results
The mushroom speleothems present in Santa Catalina Cave can reach heights up to 250 cm. The typical diameter of their stipe and cap ranges from 20 to 70 cm, and from 80 to 90 cm, respectively, and the cap thickness ranges from 30 to 45 cm. A representative mushroom is constituted by an assemblage of 4 different subunits (Figure 1). Its stipe consists of (1) a stalagmite covered by (2) centimeter-size mineral flat-flakes, well-known as “calcite rafts,” and forming a cone, which are surrounded by (3) a dense mineral coating with a bulb-like morphology called “cave clouds” or “calcite mammillaries.” The mushroom cap is constituted by (4) a mineral structure with a porous cerebroid-morphology.
The stalagmite is composed of submillimetric layers of calcite crystals characterized by two main fabrics; the external part has a high crystalline porosity and a scaffold-like arrangement, typical of dendritic fabric (Frisia, 2015; Figure 2A), while the innermost part has a laminated microcrystalline fabric with micritic layers (Figure 2B). The mineral flat-flakes are constituted of microsparite crystals bordered by aragonite crystals (Figure 2C). The flakes create a very porous deposit and its voids are locally filled with gypsum, authigenic silicate minerals, azurite and oxides. The external dense crust growing on the cone surface is made of acicular aragonite crystals that developed toward the external surface forming a fibro-radial pattern (Figure 2D).
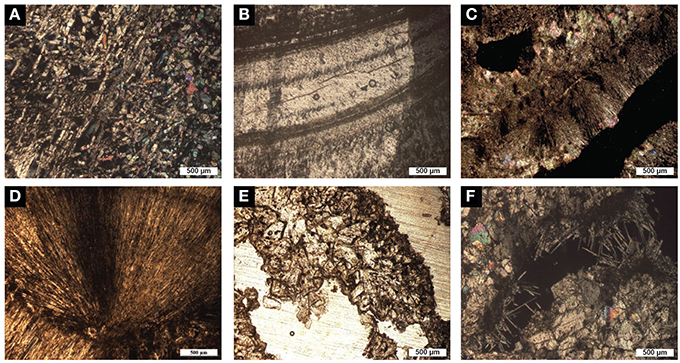
Figure 2. Optical microscope images of the different units forming the mushroom speleothem. (A) The stalagmite unit, which is composed of calcite crystals, is locally characterized by a dendritic fabric showing a scaffold-like arrangement and a high porosity (NX). (B) Microcrystalline fabric showing the laminae (likely annual growth) that characterize the inner stalagmite (NX). (C) Calcite raft composed of sparry-calcite crystals surrounded by aragonite needles (NX). (D) Aragonite crystals that constitute the cave cloud unit with acicular fabric (NX). (E) Sparry-calcite aggregates that constitute the mushroom cap (N//). (F) Fenestrae within the mushroom cap filled with rhombohedral calcite crystals and pyramidal fibers of calcite (NX).
The mushroom cap has an external granular, cerebroid morphology, while the internal structure has a porous texture characterized by irregular, elongated fenestrae visible to the naked eye (Figure 1C). Continuous laminae with a thickness ranging from 1 to 12 mm can be observed in the studied sample. However, the heterogeneous arrangement of the fenestrae, which is mainly parallel but locally also perpendicular to the laminations, confers a generally irregular internal structure to the mushroom cap. The average size of the fenestrae (32 fenestrae were measured in an area of 1120 mm2) is 4 × 2 mm. Under the microscope, it is possible to observe that the porous laminated texture is composed of sparry-calcite aggregates (Figure 2E) connected by calcite branches. The average size of the calcite aggregates and of the single crystals is 70 and 0.08 mm2, respectively. The orientation of the c-axis suggests that the growth direction of these crystals is toward the external surface of the speleothem. The fenestrae are locally filled with rhombohedral calcite crystals and needle-fiber calcite (Cailleau et al., 2009; Curry et al., 2009; Jones and Peng, 2014; Figure 2F). SEM investigations revealed the widespread presence of filamentous, oval and sheet–like microstructures that are locally encrusted by nanometric globules (Figure 3). Such microstructures have an elemental composition that is richer in C compared to the surrounding Ca-carbonate crystals, and also includes Mg and Si (Figures 3B,D–F). SEM investigations were performed on freshly broken fragments of the sample, which makes it difficult to evaluate whether these microstructures preferentially occur within the sparry-calcite aggregates or at the interface with the fenestrae. These microstructures were exclusively identified within the mushroom cap and were not observed in any of the freshly broken samples from the other units of the speleothem. The mineralogy of the different subunits of the speleothem was confirmed by X-ray diffraction.
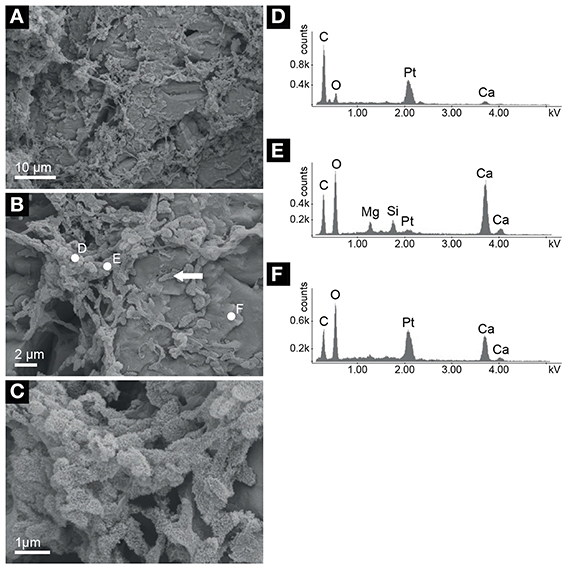
Figure 3. Scanning electron microscopy (SEM) photomicrographs of the mushroom cap. (A) Overview showing the presence of a matrix made of filamentous microstructures associated with the calcite crystals. (B) Detail of (A) showing filaments that we interpret as fossilized extracellular polymeric substances (EPS). Oval structures (arrow) may be microbial cells or EPS aggregates. (C) Detail of (B) showing nanoglobules forming on the EPS/putative cells microstructures. (D–F) Energy dispersive X-ray spectra relative to measurements performed on the sites indicated in (B), which show different stages of mineralization of the putative cells and EPS filaments from mainly organic features (D) to calcium carbonate (F). The intermediate stage of mineral growth on the organic microstructures (E) is characterized by a phase that also includes Mg and Si. Pt peaks correspond to coating applied during sample preparation.
δ13C and δ18O measured from the different units of the speleothems are shown in Figure 4. The stalagmite unit shows a larger isotopic variability with respect to that characterizing the other units of the mushroom speleothem, with δ13C ranging from −10.56 to −1.14‰; and δ18O from −4.97 to −1.09‰. Part of such variability can be attributed to climatic changes that influenced the isotopic composition of the water percolating from the surface. However, the fairly good correlation between δ18O and δ13C values (Figure 4) suggests that kinetic effects may have had a significant influence in producing the observed isotopic variability (e.g., Mickler et al., 2006). The calcite rafts, cave cloud and mushroom cap units show limited δ18O variability (i.e., <2‰) and only the cave cloud unit is characterized by distinctive, slightly more negative δ13C values, which may indicate formation during a more humid climate that caused a larger amount of organic carbon to be incorporated in the carbonate minerals. It is important to note that all the isotopic values measured from the various subunits of the mushroom speleothem are encompassed by the variability recorded in the stalagmite unit. As a consequence, isotopic data cannot be used to confidently identify specific paleoenvironments or mineralization mechanisms that occurred inside the cave. As it is the case for the stalagmite, the isotopic signals recorded in the different units may be fully controlled by kinetic processes and processes occurring outside the cave and not by differences in evaporative regimes or microbial processes occurring within the cave room/cave pool in which the mushroom speleothem formed.
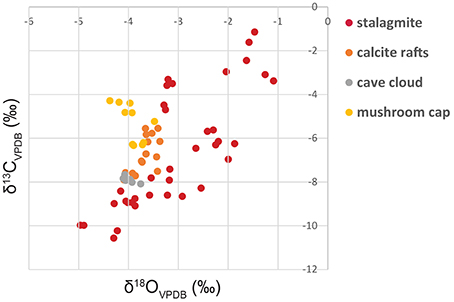
Figure 4. δ13C and δ18O values measured from the different units of the mushroom speleothem. Analytical reproducibility of the measurements was better than ±0.1‰; for both δ13C and δ18O. Isotope ratios are measured against Vienna Pee Dee Belemnite (VPDB).
Discussion
Model for Mushroom Speleothem Formation
Our observations and analyses suggest that the mushroom speleothem is a composite structure that formed during 4 distinctive phases, within an evolving cave environment (Figure 5):
Phase 1 (Figure 5A): The innermost component of the speleothem is comprised of a common stalagmite. Its mineralogy, petrography and isotopic composition are similar to those measured in other stalagmites forming in tropical environments through dripping water from the cave ceiling (Hill and Forti, 1997; Mickler et al., 2006; Fensterer et al., 2012). During this period the cave was dry, suggesting a colder and drier climate with low sea level.
Phase 2 (Figure 5B): The conical deposit, which covers the stalagmite, formed in a period during which the cave was invaded by water submerging the stalagmite. This would correspond to a wetter and warmer climate, such as an interglacial, with a sea level high-stand that caused the water table to be higher than today (Fornos et al., 2009; van Hengstum et al., 2011). Similar concretions have been described from other caves worldwide and are referred to as “raft cones.” A “raft” comprises calcite crystals that form at the surface of quiescent water bodies due to degassing or evaporation (White, 1976; Jones, 1989). Usually, floating rafts sink when hit by a falling water drop or due to their own weight. In Santa Catalina Cave, the floating rafts probably sunk below dripping points on the ceiling, which also formed the stalagmites. The aragonitic rim that surrounds the cone, as well as the other identified minerals (gypsum and authigenic quartz), likely formed during and after the sinking of the rafts. The Azurite observed in few samples probably derives from oxidation of Cu-containing minerals transported downstream by surface waters flowing from the higher lying ophiolitic rocks (Ducloz, 1963). δ18O and δ13C values of the raft cone are consistent with calcite precipitation from a cave-pool filled with tropical rain-water that percolated through the soil overlaying the cave (Mickler et al., 2006).
Phase 3 (Figure 5C): The aragonite crust growing on the cone surface is also interpreted as a subaqueous facies. Speleothems with this morphology are not uncommon and are known as “cave clouds” or “calcite mammillaries” (Hill and Forti, 1997; Polyak et al., 2013). They usually form slowly below but close to the water level, in large water bodies with limited renewal of the solution, low oversaturation with respect to CaCO3 and limited airflows. The cave was probably almost entirely flooded, as suggested by the vertical distribution of these speleothems occurring also close to the roof, thus inhibiting airflow between adjacent entrances (which might also not have existed at all). This would also be consistent with a period characterized by a warm and wet climate with a high sea level stand, causing the water table to be much higher than today. Such interpretation is consistent with the isotopic values measured from this part of the speleothem. δ18O and δ13C show less total variability indicating an environment that was chemically more stable than those related to phase 1, 2, and 4 (Figure 4).
Phase 4 (Figure 5D): The cap of the mushroom is the most unusual subunit of the speleothem. To our knowledge, this type of rock facies has never been described in detail from any other cave (one of the few comparable occurrences is probably that described by Tuccimei et al. (2010)). The cap facies occurs in a well-defined horizontal band of the cave rooms (i.e., on the top of the mushroom stipe and on the walls surrounding the water pool). Their largest parts are all located at exactly the same height, suggesting a water level control. Most probably, this indicates that the precipitation of minerals occurred close to the water surface within a vertical oscillation zone that was likely caused by variations of the water table influenced by tidal fluctuations (Mylroie and Carew, 1990; Boop et al., 2014). Alternatively, this facies may have formed just below the water table, constantly bathed in stagnant water, in a zone where chemical gradients existed, for example, caused by degassing of CO2. The water level at this stage was certainly lower than during phase 3, likely with a lower sea level and under a colder and less humid climate. δ18O and δ13C values of cap samples are not dissimilar to those characterizing the other subunits of the mushroom, suggesting that unusual evaporative regimes or unusual carbonate sources were not the key factor controlling its formation. The porous cerebroid-like morphology of the cap is similar to that of stromatolites and thrombolites that occur in the photic zone of marine embayments and lagoons (Jahnert and Collins, 2012; Bosak et al., 2013). The analogies with microbialites are even more evident at the microscopic scale. Indeed, filamentous microstructures made of partially mineralized organic material—that we interpret as fossilized extracellular polymeric substances (EPS)—and oval bodies that may be relic cells or EPS aggregates are widespread within the mushroom cap (Figure 3). Similar organic microstructures are common in living biofilms (e.g., Baum et al., 2009; Figure 14a in Jahnert and Collins, 2012) and are sometimes preserved in ancient microbialites (Perry et al., 2007). In living biofilms, EPS microstructures form an organic matrix that plays an important role in microbially influenced mineralization processes, leading to the formation of mineral concretions characterized by internal laminations, fenestrae, cavities, as well as crenulated and irregular surfaces (Bontognali et al., 2008; Dupraz et al., 2009; Vasconcelos et al., 2014). Besides acting as a nucleation site, the EPS matrix concentrates specific cations creating local micro-environments that favor supersaturation and precipitation of carbonate minerals (Dupraz et al., 2009). The early stage of mineral nucleation within EPS is often characterized by the formation of nanoglobules (Riding, 2000; Benzerara et al., 2006; Bontognali et al., 2008; Sánchez-Román et al., 2014) and by the presence of an amorphous Mg-silicate (Léveillé et al., 2000; Arp et al., 2003; Bontognali et al., 2010, 2014; Burne et al., 2014; Zeyen et al., 2015). Both features are observable in the mushroom cap in combination with the partially mineralized EPS microstructures (Figure 3B), suggesting that the cap facies may have formed through a microbially-influenced mineralization process similar to that occurring in many microbialite-producing microbial mats (Dupraz et al., 2009). Because no “mushroom-cap facies” is actively forming today in any of the explored areas of Santa Catalina Cave, it has not been possible to conduct a molecular biology study and obtain information on the diversity and physiology of the microbial community that was associated with the speleothem at the time of its formation.
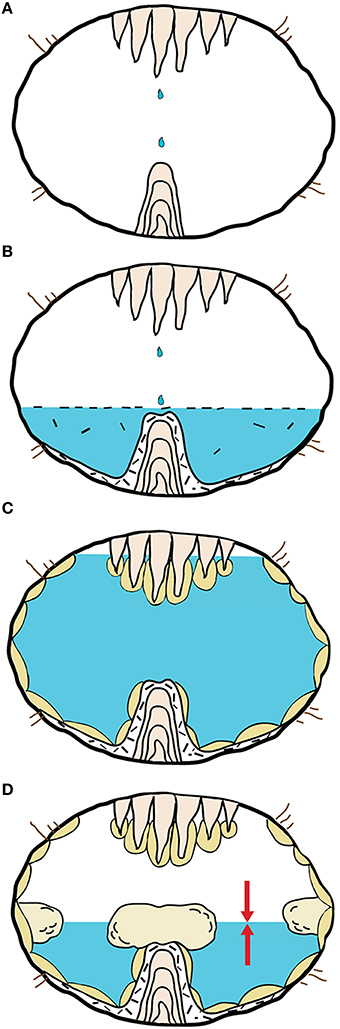
Figure 5. Genesis of the Mushroom speleothem.(A) A stalagmite forms in a subaerial environment. (B) Calcite rafts precipitate at the water table of a cave pool and sink forming a raft cone that surrounds the stalagmite. (C) The raft cone is encrusted by aragonitic cave clouds that form in a subaqueous environment. (D) The mushroom cap forms close to the water table through a microbially-influenced mineralization process.
Implications for the Interpretations of Ancient Stromatolites
A debated question in the field of geobiology is whether some microbialite morphotypes can be used not simply as “generic” evidence for the existence of past life but as an indication that photosynthetic microorganisms—and in particular oxygenic ones—were part of the primitive mat community (Bosak et al., 2013). In the context of this debate, the mushroom speleothems represent an interesting case. Although we cannot quantify the importance of biological vs. abiotic factors in determining their morphology, there is no doubt that the studied speleothems formed in the complete darkness and, thus, in the absence of any photosynthetic microorganism. We can conclude that the cerebroid morphology of the mushroom cap, as well as its internal texture including elongated fenestrae are not due to biological growth driven by competition for light. Moreover, in Santa Catalina Cave, the mushroom caps occur exclusively in a limited vertical band, which was likely defined by a fluctuating water table at the time of their formation. In previous studies, the occurrence of stromatolites in well-defined intervals of Archean sedimentary sequences has been interpreted as evidence that the microbialites formed at a certain water depth with a specific seafloor illumination, leading to the conclusion that phototrophs were present in the early microbial community (e.g., Allwood et al., 2009). Our study shows that other factors, which do not depend on illumination (e.g., fluctuating water-table), may control and limit the distribution of stromatolites to specific areas of a sedimentary basin, not exclusively in cave pools but potentially also in restricted basins at the surface. Although recent cave environments are surely significantly different in terms of water chemistry and microbial ecology than Archean paleoenvironments, further investigations of Santa Catalina Cave and similar subterranean sites could lead towards a better and more comprehensive understanding of the mineralization processes that may have been common on the early Earth, prior to the advent of photosynthetic microorganisms.
Author Contributions
TB: conceived research, participated in the expedition to collect the samples, performed analyses in the laboratory, interpreted the data, and wrote the manuscript. ID: participated in the expedition to collect the samples, performed most of the analyses in the laboratory, interpreted the data, helped writing the manuscript and prepared most of the figures. NT: conceived research, participated in the expedition to collect the samples, interpreted the data, edited the manuscript. CV: conceived research, provided most analytical facilities, interpreted the data, edited the manuscript. SB: performed stable isotopes measurements, provided analytical facility, interpreted the data, edited the manuscript. EG: conceived research, organized the expedition to the cave, participated in the expedition to collect the samples, interpreted the data. JD: conceived research, participated in the expedition to collect the samples, performed analyses in the laboratory, interpreted the data, and helped writing the manuscript.
Conflict of Interest Statement
The authors declare that the research was conducted in the absence of any commercial or financial relationships that could be construed as a potential conflict of interest.
Acknowledgments
We thank Stefano Torriani and Osmany Ceballo Melendres for taking part to the sampling campaign, La Salle 3D for the help in organizing the expedition, Ercilio Vento Canosa for helping us to obtain the necessary permissions for sampling, Emily Rose Ciscato for the help in writing the manuscript, and Michał Gradziński for the comprehensive and constructive review. Scurion GmbH provided the high performance lamps that were used during the exploration of the cave. This study was supported by PETHROS, a collaboration project between Petrobras and ETH Zurich.
References
Allwood, A. C., Grotzinger, J. P., Knoll, A. H., Burch, I. W., Anderson, M. S., Coleman, M.-L., et al. (2009). Controls on development and diversity of early archean stromatolites. Proc. Natl. Acad. Sci. U.S.A. 106, 9548–9555. doi: 10.1073/pnas.0903323106
Allwood, A. C., Kamber, B. S., Walter, M. R., Burch, I. W., and Kanik, I. (2010). Trace elements record depositional history of an early archean stromatolitic carbonate platform. Chem. Geol. 270, 148–163. doi: 10.1016/j.chemgeo.2009.11.013
Allwood, A. C., Walter, M. R., Kamber, B. S., Marshall, C. P., and Burch, I. W. (2006). Stromatolite reef from the early archaean era of Australia. Nature 441, 714–718. doi: 10.1038/nature04764
Arp, G., Reimer, A., and Reitner, J. (2003). Microbialite formation in seawater of increased alkalinity, Satonda Crater lake, Indonesia. J. Sediment. Res. 73, 105–127. doi: 10.1306/071002730105
Baskar, S., Baskar, R., and Kaushik, A. (2007). Evidence for microbial involvement in the genesis of speleothem carbonates, Borra caves, Visakhapatnam, India. Curr. Sci. 92, 350–355.
Baskar, S., Baskar, R., and Routh, J. (2011). Biogenic evidences of moonmilk deposition in the Mawmluh cave, Meghalaya, India. Geomicrobiol. J. 28, 252–265. doi: 10.1080/01490451.2010.494096
Baskar, S., Baskar, R., and Routh, J. (2014). Speleothems from Sahastradhara caves in Siwalik Himalaya, India: possible biogenic inputs. Geomicrobiol. J. 31, 664–681. doi: 10.1080/01490451.2013.871087
Baum, M. M., Kainovic, A., O'Keeffe, T., Pandita, R., McDonald, K., Wu, S., et al. (2009). Characterization of structures in biofilms formed by a Pseudomonas fluorescens isolated from soil. BMC Microbiol. 9:103. doi: 10.1186/1471-2180-9-103
Benzerara, K., Menguy, N., López-García, P., Yoon, T.-H., Kazmierczak, J., Tyliszczak, T., et al. (2006). Nanoscale detection of organic signatures in carbonate microbialites. Proc. Natl. Acad. Sci. U.S.A. 103, 9440–9445. doi: 10.1073/pnas.0603255103
Blyth, A. J., and Frisia, S. (2008). Molecular evidence for bacterial mediation of calcite formation in cold high-altitude caves. Geomicrobiol. J. 25, 101–111. doi: 10.1080/01490450801934938
Bontognali, T. R., Martinez-Ruiz, F., McKenzie, J. A., Bahniuk, A., Anjos, S., and Vasconcelos, C. (2014). Smectite synthesis at low temperature and neutral pH in the presence of succinic acid. Appl. Clay Sci. 101. 553–557. doi: 10.1016/j.clay.2014.09.018
Bontognali, T. R. R., Sessions, A. L., Allwood, A. C., Fischer, W. W., Grotzinger, J. P., Summons, R. E., et al. (2012). Sulfur isotopes of organic matter preserved in 3.45-billion-year-old stromatolites reveal microbial metabolism. Proc. Natl. Acad. Sci. U.S.A. 109, 15146–15151. doi: 10.1073/pnas.1207491109
Bontognali, T. R. R., Vasconcelos, C., Warthmann, R. J., Bernasconi, S. M., Dupraz, C., Strohmenger, C. J., et al. (2010). Dolomite formation within microbial mats in the coastal sabkha of Abu Dhabi (United Arab Emirates). Sedimentology 57, 824–844. doi: 10.1111/j.1365-3091.2009.01121.x
Bontognali, T. R. R., Vasconcelos, C., Warthmann, R. J., Dupraz, C., Bernasconi, S. M., and McKenzie, J. A. (2008). Microbes produce nanobacteria-like structures, avoiding cell entombment. Geology 36, 663–666. doi: 10.1130/G24755A.1
Boop, L. M., Onac, B. P., Wynn, J. G., Fornós, J. J., Rodríguez-Homar, M., and Merino, A. (2014). Groundwater geochemistry observations in littoral caves of Mallorca (western Mediterranean): implications for deposition of phreatic overgrowths on speleothems. Int. J. Speleol. 43, 193–203. doi: 10.5038/1827-806X.43.2.7
Bosak, T., Knoll, A. H., and Petroff, P. (2013). The meaning of Stromatolites. Annu. Rev. Earth Planet. Sci. 41, 21–44. doi: 10.1146/annurev-earth-042711-105327
Breitenbach, S. F. M., and Bernasconi, S. M. (2011). Carbon and oxygen isotope analysis of small carbonate samples (20 to 100 μg) with a GasBench II preparation device. RCM 25, 1910–1914. doi: 10.1002/rcm.5052
Burne, R. V., Moore, L. S., Christy, A. G., Troitzsch, U., King, P. L., Carnerup, A. M., et al. (2014). Stevensite in the modern thrombolites of Lake Clifton, Western Australia: a missing link in microbialite mineralization? Geology 42, 575–578. doi: 10.1130/G35484.1
Cabrera, M., and Penãlver, L. L. (2001). Contribución a la estratigrafia de los depósitos Cuaternarios de Cuba. Rev. C. G. 15, 37–49.
Cailleau, G., Verrecchia, E. P., Braissant, O., and Emmanuel, L. (2009). The biogenic origin of needle fiber calcite. Sedimentology 56, 1858–1875. doi: 10.1111/j.1365-3091.2009.01060.x
Curry, M. D., Boston, P. J., Spilde, M. N., Baichtal, J. F., and Campbell, A. R. (2009). Cottonballs, a unique subaqueous moonmilk, and abundant subaerial moonmilk in Cataract cave, Tongass National Forest, Alaska. Int. J. Spel. 38, 111–128. doi: 10.5038/1827-806X.38.2.3
D'Angeli, I. M., De Waele, J., Ceballo Melendres, O., Tisato, N., Sauro, F., Grau Gonzalez, E. R., et al. (2015). Genesis of folia in a non-thermal epigenic cave (Matanzas, Cuba), Geomorphology 228, 526–535. doi: 10.1016/j.geomorph.2014.09.006
Ducloz, C. (1963). Etude géomorphologiue de la région de Matanzas, Cuba. Arch. Sci. Génève 16, 351–422.
Dupraz, C., Reid, R. P., Braissant, O., Decho, A. W., Norman, R. S., and Visscher, P. T. (2009). Processes of carbonate precipitation in modern microbial mats. Earth Sci. Rev. 96, 141–162. doi: 10.1016/j.earscirev.2008.10.005
Fensterer, C., Scholz, D., Hoffmann, D., Spötl, C., Pajón, J. M., and Mangini, A. (2012). Cuban stalagmite suggests relationship between Caribbean precipitation and the Atlantic multidecadal oscillation during the past 1.3 ka. Holocene 22, 1405–1412. doi: 10.1177/0959683612449759
Florea, L. J., Noe-Stinson, C. L., Brewer, J., Fowler, R., Kearns, B. J., and Greco, A. M. (2011). Iron oxide and calcite associated with Leptothrix sp. biofilms within an estavelle in the upper floridan aquifer. Int. J. Speleol. 40, 205–219. doi: 10.5038/1827-806X.40.2.12
Fornos, J. J., Gines, J., and Gracia, F. (2009). Present-day sedimentary facies in the coastal karst caves of Mallorca island (western Mediterranean). J. Caves Karst Stud. 71, 86–99.
Frisia, S. (2015). Microstratigraphic logging of calcite fabrics in speleothems as tool for palaeoclimate studies. Int. J. Speleol. 44, 1–16. doi: 10.5038/1827-806X.44.1.1
Gradzíński, M., Chmiel, M. J., and Motyka, J. (2012). Formation of calcite by chemolithoautotrophic bacteria - a new hypothesis, based on microcrystalline cave pisoids. Ann. Soc. Geol. Pol. 82, 361–369.
Grotzinger, J. P., and Knoll, A. H. (1999). Stromatolites in Precambrian carbonmates: evolutionary mileposts or environmental dipsticks? Annu. Rev. Earth Planet. Sci. 27, 313–358. doi: 10.1146/annurev.earth.27.1.313
Hill, C. A., and Forti, P. (1997). Cave minerals of the World, 2nd Edn. Huntsville, AL: National Speleological Society.
Homann, M., Heubeck, C., Bontognali, T. R., Bouvier, A. S., Baumgartner, L. P., and Airo, A. (2016). Evidence for cavity-dwelling microbial life in 3.22 Ga tidal deposits. Geology 44, 51–54. doi: 10.1130/G37272.1
Jahnert, R. J., and Collins, L. B. (2012). Characteristics, distribution and morphogenesis of subtidal microbial system in Shark Bay, Australia. Mar. Geol. 303–306, 115–136. doi: 10.1016/j.margeo.2012.02.009
Jones, B. (1989). Calcite rafts, peloids, and micrite in cave deposits from Cayman Brac, British West Indies. Can. J. Earth Sci. 26, 654–664. doi: 10.1139/e89-056
Jones, B. (2001). Microbial activity in caves—a geological perspective. Geomicrobiol. J. 18, 345–357. doi: 10.1080/01490450152467831
Jones, B. (2011). Stalactite growth mediated by biofilms: example from Nani cave, Cayman Brac, British West Indies. J. Sediment. Res. 81, 322–338. doi: 10.2110/jsr.2011.28
Jones, B., and Peng, X. (2014). Hot spring deposits on a cliff face: a case study from Jifei, Yunnan Province, China. Sediment. Geol. 302, 1–28. doi: 10.1016/j.sedgeo.2013.12.009
Léveillé, R. J., Fyfe, W. S., and Longstaffe, F. J. (2000). Geomicrobiology of carbonate-silicate microbialites from Hawaiian basaltic sea caves. Chem. Geol. 169, 339–355. doi: 10.1016/S0009-2541(00)00213-8
Lisker, S., Vask, A., Bar-Matthews, M., Porat, R., and Frumkin, A. (2009). Stromatolites in caves of Dead Sea Fault Escarpment: implications to latest Pleistocene lake levels and tectonic subsidence. Q. Sci. Rev. 28, 80–92. doi: 10.1016/j.quascirev.2008.10.015
Lozano, R. P., and Rossi, C. (2012). Exceptional preservation of Mn-oxiding microbes in cave stromatolites (El Soplao, Spain). Sediment. Geol. 255–256, 42–55. doi: 10.1016/j.sedgeo.2012.02.003
Lundberg, J., and McFarlane, D. A. (2011). A note on the occurrence of a crayback stalagmite at Niah Caves, Borneo. Int. J. Speleol. 40, 39–43. doi: 10.5038/1827-806X.40.1.5
Melim, L. A., Shinglman, K. M., Boston, P. J., Northup, D. E., Spilde, M. J., and Queen, M. J. (2001). Evidence for microbial involvement in pool finger precipitation, Hidden Cave, New Mexico. Geomicrobiol. J. 18, 311–329. doi: 10.1080/01490450152467813
Mickler, P. J., Stern, L. A., and Banner, J. L. (2006). Large kinetic isotope effects in modern speleothems. Geol. Soc. Am. Bull. 118, 65–81. doi: 10.1130/B25698.1
Mylroie, J. E., and Carew, J. L. (1990). The flank margin model for dissolution cave development in carbonate platforms. Earth Surf. Process. Land. 15, 413–424. doi: 10.1002/esp.3290150505
Nuñez Jimenez, A. (1962). “Una nueva formación secundaria en la espeleologia,” in 2nd International Congress on Speleology. Istituto Italiano di Speleologia (Castellana Grotte), 496–499.
Nuñez Jimenez, A. (1973). “Las formaciones fungiformes y su importancia para conocer las fluctuaciones del mar,” in VIth International Congress on Speleology (Olomouc: Czech Speleological Society), 519–527.
Olivier, N., Hantzpergue, P., Gaillard, C., Pittet, B., Leinfelder, R. R., Schmid, D. U., et al. (2003). Microbialite morphology, structure and growth: a model of the upper Jurassic reefs of the Chay Peninsula (western France). Palaeo3, 193, 383–404. doi: 10.1016/S0031-0182(03)00236-0
Pacton, M., Breitenbach, S. F. M., Lechleitner, F. A., Vaks, A., Rollion-Bard, C., Gutareva, O. S., et al. (2013). The role of microorganisms in the formation of a stalactite in Botovskaya Cave, Siberia – paleoenvironmental implications. Biogeosciences 10, 6115–6130. doi: 10.5194/bg-10-6115-2013
Perry, R. S., McLoughlin, N., Lynne, B. Y., Sephton, M. A., Oliver, J. D., Perry, C. C., et al. (2007). Defining biominerals and organominerals: direct and indirect indicators of life. Sediment. Geol. 201, 157–179. doi: 10.1016/j.sedgeo.2007.05.014
Polyak, V. J., DuChene, H. R., Davis, D. G., Palmer, A. N., Palmer, M. V., and Asmerom, Y. (2013). Incision history of Glenwood Canyon, Colorado, USA, from the uranium-series analyses of water-table speleothems. Int. J. Speleol. 42, 193–202. doi: 10.5038/1827-806X.42.3.3
Riding, R. (2000). Microbial carbonates: the geological record of calcified bacterial–algal mats and biofilms. Sedimentology 47, 179–214. doi: 10.1046/j.1365-3091.2000.00003.x
Rossi, C., Lozano, R. P., Isanta, N., and Hellstrom, J. (2010). Manganese stromatolites in caves: El Soplao (Cantabria, Spain). Geology 38, 1119–1122. doi: 10.1130/G31283.1
Sánchez-Román, M., Fernández-Remolar, D., Amils, R., Sánchez-Navas, A., Schmid, T., San Martin-Uriz, P., et al. (2014). Microbial mediated formation of Fe-carbonate minerals under extreme acidic conditions. Sci. Rep. 4:4767. doi: 10.1038/srep04767
Tisato, N., Torriano, S. F. F., Monteux, S., Sauro, F., De Waele, J., Lavagna, M. L., et al. (2015). Microbial mediation of complex subterranean mineral structures. Sci. Rep. 5, 10. doi: 10.1038/srep15525
Tuccimei, P., Soligo, M., Ginés, J., Ginés, A., Fornós, J., Kramers, J., et al. (2010). Constraining Holocene sea levels using U-Th ages of phreatic overgrowths on speleothems from coastal caves in Mallorca (western Mediterranean). ESPL 35, 782–790. doi: 10.1002/esp.1955
van Hengstum, P. J., Scott, D. B., Gröcke, D. R., and Charette, M. A. (2011). Sea level controls sedimentation and environments in coastal caves and sinkholes. Mar. Geol. 286, 35–50. doi: 10.1016/j.margeo.2011.05.004
Vasconcelos, C., Dittrich, M., and McKenzie, J. A. (2014). Evidence of microbiocoenosis in the formation of laminae in modern stromatolites. Facies 60, 3–13. doi: 10.1007/s10347-013-0371-3
Watson, J., Hamilton-Smith, E., Gillieson, D., and Kiernan, K. (eds.). (1997). Guidelines for Cave and Karst Protection. Gland; Cambridge: IUCN.
White, W. (1976). “Cave minerals and speleothems,” in The Science Of Speleology eds T. D. Ford and C. H. D. Cullingford (New York, NY: Academic Press), 267–327.
Keywords: biogenic speleothem, microbialites, stromatolites, EPS, tropical cave, Cuba, early life
Citation: Bontognali TRR, D'Angeli IM, Tisato N, Vasconcelos C, Bernasconi SM, Gonzales ERG and De Waele J (2016) Mushroom Speleothems: Stromatolites That Formed in the Absence of Phototrophs. Front. Earth Sci. 4:49. doi: 10.3389/feart.2016.00049
Received: 16 December 2015; Accepted: 11 April 2016;
Published: 28 April 2016.
Edited by:
Karim Benzerara, Centre National de la Recherche Scientifique, FranceReviewed by:
Jakob Zopfi, University of Basel, SwitzerlandMichal Gradzinski, Jagiellonian University, Poland
Copyright © 2016 Bontognali, D'Angeli, Tisato, Vasconcelos, Bernasconi, Gonzales and De Waele. This is an open-access article distributed under the terms of the Creative Commons Attribution License (CC BY). The use, distribution or reproduction in other forums is permitted, provided the original author(s) or licensor are credited and that the original publication in this journal is cited, in accordance with accepted academic practice. No use, distribution or reproduction is permitted which does not comply with these terms.
*Correspondence: Tomaso R. R. Bontognali, tomaso.bontognali@erdw.ethz.ch;
Ilenia M. D'Angeli, ilenia.dangeli@alice.it