- 1Department of Ecology, Evolution, and Behavior, University of Minnesota, Saint Paul, MN, USA
- 2Department of Plant Biology, University of Minnesota, Saint Paul, MN, USA
- 3College of Biological Science, University of Minnesota, Saint Paul, MN, USA
Humans have more than doubled inputs of reactive nitrogen globally and greatly accelerated the biogeochemical cycles of phosphorus and metals. However, the impacts of increased element mobility on tropical ecosystems remain poorly quantified, particularly for the vast tropical dry forest biome. Tropical dry forests are characterized by marked seasonality, relatively little precipitation, and high heterogeneity in plant functional diversity and soil chemistry. For these reasons, increased nutrient deposition may affect tropical dry forests differently than wet tropical or temperate forests. Here, we review studies that investigated how nutrient availability affects ecosystem and community processes from the microsite to ecosystem scales in tropical dry forests. The effects of N and P addition on ecosystem carbon cycling and plant and microbial dynamics depend on forest successional stage, soil parent material, and rainfall regime. Responses may depend on whether overall productivity is N- vs. P-limited, although data to test this hypothesis are limited. These results highlight the many important gaps in our understanding of tropical dry forest responses to global change. Large-scale experiments are required to resolve these uncertainties.
Introduction
Not only have humans more than doubled the amount of reactive nitrogen (N) entering the global N cycle through industry, energy use, and agriculture (Vitousek, 1994; Galloway et al., 2004), we have also greatly accelerated the biogeochemical cycles of phosphorus (P) and metals (Smil, 2000; Rauch and Pacyna, 2009). While ecosystem processes in the temperate zone are thought to be primarily limited by the availability of N, a wide array of elements including N, P, and sodium (Na) can control tropical forest productivity, decomposition, and tree species distributions (Kaspari et al., 2009; Cleveland et al., 2011; Condit et al., 2013). The high biogeochemical heterogeneity of tropical forests (Townsend et al., 2008) challenges our ability to model ecosystem carbon (C) cycling and forest dynamics under rapidly changing climate and nutrient deposition regimes, and suggests that research from temperate forests may not apply in the tropics.
Ecosystem state factors including climate, parent material, topography, biotic organisms, and weathering affect the absolute and relative availabilities of different elements (Jenny, 1941). In general, ecosystem processes (e.g., net primary productivity, NPP) are limited by N on geologically young soil substrates, such as recently glaciated soils in the temperate zone or recent lava flows in the tropics (Vitousek, 1999; Reich and Oleksyn, 2004). By contrast, older geologic substrates are depleted of rock-derived elements such as P and cations [e.g., calcium (Ca), potassium (K)]. As a consequence, it is generally thought that tropical forests, which tend to have more highly weathered soils, are primarily limited by P (Walker and Syers, 1976; Chadwick et al., 1999; Cleveland et al., 2011). Indirect evidence tends to support this generalization for tropical wet forests (Cleveland et al., 2011), although stand-level fertilization experiments demonstrate that N, P, and K additions all may influence tropical NPP (Wright et al., 2011; Alvarez-Clare et al., 2013). Additionally, previous land-use practices such as deforestation and burning may lead to N limitation of young secondary forests (Kauffman et al., 1993; Campo and Vázquez-Yanes, 2004; Davidson et al., 2007).
Tropical dry forests (TDFs) comprise more than 40% of the potentially forested area in tropical latitudes (Murphy and Lugo, 1986). In general, these forests have lower annual rainfall compared to moist or wet tropical forests, and experience a 3+ month dry season with little or no rainfall. As a consequence, many dry forest species have unique adaptations to seasonal drought such as deciduous leaf habits and deep roots (Eamus, 1999). Despite their large area, nutrient limitation and biogeochemical cycling in TDFs are relatively understudied (Gei and Powers, 2014). Further, there are reasons to expect that nutrient constraints and responses to anthropogenic nutrient deposition may differ in dry vs. wet tropical forests because of the strong water limitation and seasonally pulsed soil water availability in TDFs (Lambert et al., 1980; Read and Lawrence, 2006).
Here, we review the few studies that investigate how nutrient availability affects ecological processes in TDFs. Because of rapidly increasing rates of N deposition across the tropics (Hietz et al., 2011; Sullivan et al., 2014a), most of the studies included in our review focus on effects of N addition. We develop a conceptual model of how added N affects TDFs; because TDFs may be N- or P-limited, our model considers how responses to N addition may vary between forests with low or high P availability. We compare these hypotheses with what is known about these interactions in TDFs, integrate key lessons from temperate-zone research and draw contrasts with tropical wet forests. Further, when available, we point to studies that measure TDF ecosystem responses to the addition of P or other elements. We conclude by highlighting research gaps and priorities for future research.
Conceptual Model of Nutrients and Ecological Processes in Tropical Dry Forest
Identifying which elements limit ecological processes in TDFs is a high priority for several reasons. First, ecosystem responses to nutrient enrichment and rising atmospheric CO2 depend upon the identity of the limiting nutrient (Hall and Matson, 1999). For example, N deposition may increase productivity in N-limited forests, but have no effect in a P-limited forest. Second, because rainfall is highly seasonal in TDFs, documenting interactions between water and nutrient availability may be crucial to accurately predict ecosystem responses to anthropogenic change. Understanding the relationship between precipitation pulses and element cycling poses a significant challenge for ecosystem modelers (Manzoni et al., 2014), and therefore responses of tropical wet forests to fertilization may not generalize to more seasonal ecosystems. Last, TDFs typically contain trees with diverse phenological strategies, from evergreen to drought-deciduous trees (Eamus, 1999), and these may respond differently to changing nutrient availability.
Our conceptual model of how increasing N deposition may affect key ecosystem processes in TDFs includes multiple levels of organization (Figure 1). This model summarizes hypotheses about how N deposition interacts with underlying nutrient limitation to generate a diversity of ecosystem responses. We emphasize here that different plant functional or phenological groups may vary in their responses to N deposition. Furthermore, TDFs are notable for the high abundances and diversity of legume trees that fix atmospheric nitrogen, and theory suggests legumes will be less affected by added N (Hedin et al., 2009). Because available evidence on TDF responses to water and nutrient availability is so limited, we treat Figure 1 as a set of hypotheses, and throughout the review we evaluate the extent to which current literature for all biomes, and TDFs in particular, supports our conceptual model (Table 1).
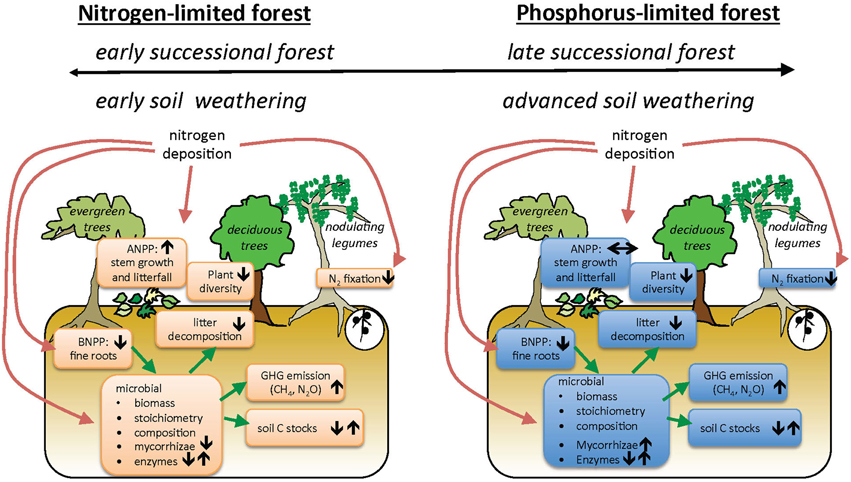
Figure 1. Conceptual model of how nitrogen addition affects community dynamics and ecosystem processes in tropical dry forest ecosystems initially limited by nitrogen (N) (A) or phosphorus (P) (B). Our model includes the possibilities that there are multiple mechanisms that account for N- or P-limitation (top bars). Arrows represent hypothesized direction of change in pool or process under N deposition.

Table 1. List of processes, hypothesized changes under nitrogen deposition in tropical dry forests, and whether this change is supported by the literature, along with key references.
Communities: Microbial Composition and Function
Because bacteria and fungi mediate soil element cycling, microbial responses to N and P inputs may drive ecosystem-scale responses to nutrient enrichment. For example, resource stoichiometry influences microbial growth rates (Rousk and Baath, 2007), substrate use efficiency (Sinsabaugh et al., 2013), and the production of extracellular enzymes that degrade soil organic matter (Mooshammer et al., 2012). In turn, these microbial traits and processes affect soil C storage (Cotrufo et al., 2013) and the availability of nutrients to plants. Across the globe, the C:N:P ratio of microbial biomass is fairly well-constrained despite substantial variation in the environmental availability of these elements (Cleveland and Liptzin, 2007). Therefore, background nutrient limitation will determine microbial responses to nutrient enrichment in TDF. The addition of limiting nutrients should augment the size and/or growth efficiency of the microbial biomass (Schimel and Weintraub, 2003; Geisseler and Scow, 2014), which in turn may alter soil C storage (Bradford et al., 2013; Frey et al., 2014).
Limited evidence suggests that microbial biomass tends to be more P-limited in tropical soils (Cleveland et al., 2002, 2003; Waring et al., 2013; Turner and Wright, 2014; Warren et al., 2015). Consistent with this hypothesis, P but not N addition increased microbial biomass in a TDF in southern China (Li et al., 2015a). However, because diffusion and osmotic stress constrain microbial growth under dry conditions (Manzoni et al. 2014), nutrient uptake is strongly mediated by water availability. The handful of studies that have examined microbial nutrient limitation in TDF soils report that microbial responses to labile C (Montano et al., 2007), N, and P (Galicia and Garcia-Oliva, 2004) are dependent upon season and the intensity of plant-microbe competition.
Mycorrhizal fungi deserve special mention. Mycorrhizae live in close symbiosis with plants and their responses to nutrient addition depend partially upon plant nutrient status as well as background nutrient limitation (Treseder, 2004). N deposition decreases arbuscular mycorrhizal biomass in both temperate and tropical forests (Johnson et al., 2003; Treseder, 2004; Camenzind et al., 2014; Krashevska et al., 2014; Wurzburger and Wright, 2015), but responses to N and P addition are context-dependent. Ecological relationships between plants and their mycorrhizae depend both upon soil C:N:P stoichiometry (Johnson et al., 2015) and mycorrhizal type (e.g., arbuscular, ectomycorrhizal, etc). Theory predicts that N deposition may lead to increased mycorrhizal biomass and more parasitic phenotypes under N-limitation and decreased biomass/more mutualistic phenotypes under P-limitation (Johnson et al., 2015). Finally, because mycorrhizae may enhance plant water uptake to different degrees depending on nutrient availability and mycorrhizal type (Auge, 2001, 2004; Lehto and Zwiazek, 2011), any change in mycorrhizal colonization or composition related to nutrient deposition may affect plant water relations during drought, potentially leading to differential feedbacks on evergreen and deciduous species in TDFs.
Communities: Plants
N deposition may reduce plant diversity in a number of ecosystems (Bobbink et al., 2010). While large-scale studies addressing this in TDFs are rare, a number of seedling pot experiments have tested how particular functional groups respond to increased nutrient availability (Lawrence, 2003). In general, higher N availability enhances biomass and relative growth rates and decreases root: shoot ratios (Huante et al., 1995a; Mendieta-Araica et al., 2013). The response to elevated nutrient availability is higher in light-demanding species compared to shade-tolerants (Huante et al., 1995a), in small-seeded species compared to those with larger seeds (Huante et al., 1995b), in slow-growing compared to fast-growing species (Khurana and Singh, 2004; Tripathi and Raghubanshi, 2014), and in non-legumes compared to legumes (Tripathi and Raghubanshi, 2014). However, not all of these generalizations are consistent among studies.
In field fertilization experiments in TDFs, seedling growth and survivorship in fertilized plots was higher than controls overall, but this effect was species- and site-specific (Salinas-Peba et al., 2014). Additionally, seedling responses tended to be higher for N vs. P addition (Salinas-Peba et al., 2014), suggesting that ANPP may be N-limited, at least for young plants. Fertilization of regenerating TDFs in Mexico led to higher seedling recruitment, survival and growth (Ceccon et al., 2003, 2004), increases in herbivory, and increased leaf P or N content in trees (in young and old sites, respectively) (Campo and Dirzo, 2003). In this experiment, responses to fertilization were higher in young forests with high light availability, but again, these depended on particular species (Ceccon et al., 2003).
Altered nutrient availability may also impact the intensity of interspecific competition, generating shifts in community composition over time. Six years of fertilizing an abandoned pasture with N and P in a highly seasonal region in Amazonia favored the growth of a few nutrient-responsive early-successional tree species, decreasing tree assemblage evenness (Siddique et al., 2010). We hypothesize that higher nutrient availability could impact species composition through similar mechanisms in regenerating TDFs (Figure 1), though there is a dearth of long-term experimental data that can clarify community-wide impacts (Table 1).
Ecosystem Processes: Net Primary Production
Responses of NPP to added N or P indicate whether primary productivity is limited by N, P, or both (Figure 1). Fertilization experiments can also offer mechanistic insight by demonstrating how tree stem diameter growth, litterfall, and fine root production each respond to increases in nutrient availability. Fertilization experiments that measured ANPP in TDFs after nutrient addition have yielded varied results. A fertilization study in a plantation forest in Venezuela showed no effect of P on wood production (Torres and Franco, 1994), while other studies have demonstrated strong co-limitation of ANPP by N and P (Campo and Vázquez-Yanes, 2004). In the latter study, responses of litterfall to nutrient addition depended upon soil type, underscoring that leaf and wood production may respond differently to element availability. Complicating matters further, the experiment in Mexico also showed that diameter growth responses to added nutrients depended on species identity (Campo et al., 2012).
Belowground NPP results are similarly heterogeneous. A meta-analysis of fine root production across temperate and wet tropical ecosystems showed decreases in fine root production with increases in N deposition, coupled with an increase in coarse root stocks (Li et al., 2015b). By contrast, a savannah fertilization experiment showed increased fine root production only in plots fertilized with P, and not with N (Barger et al., 2002). While these studies have done much to elucidate how N and P influence NPP, more detailed fertilization experiments in TDF are needed that address interactions between nutrient addition and factors that modify forest responses including succession, edaphic gradients, management, and water availability.
Ecosystem Processes: Decomposition
In general, decomposition rates are mediated by interactions among climate, litter quality, and decomposer communities (Meentemeyer, 1978; Kwabiah et al., 1999). Nutrient addition could affect decomposition via changes in litter chemistry or alterations to microbial community composition or function (Hobbie and Vitousek, 2000; Campo et al., 2012). However, within TDFs litterfall and soil nutrient availability are regulated by intra-annual precipitation events (Anaya et al., 2012), and as a result, decomposition may be primarily limited by water availability (Lambert et al., 1980; Read and Lawrence, 2006).
Plot-scale fertilization with N and/or P changed both litter nutrient concentrations and quantities in a TDF in Mexico, although the magnitude of change depended on forest successional age (Campo et al., 2007) and soil N availability (Campo et al., 2012). This is consistent with evidence that N addition effects depend on the form of added N (Lv et al., 2013), litter chemistry or quality (Kwabiah et al., 1999), and site-specific characteristics such as annual rainfall and soil nutrient availability (Bejarano et al., 2014a). In another fertilization experiment in a seasonal tropical forest in China, added P increased decomposition rates while the addition of N inhibited decomposition (Chen et al., 2013). Lab experiments also find that P and micronutrients accelerate leaf litter decay in TDF, while added N retards it (Powers and Salute, 2011). The mechanisms for why added N decreases decomposition, even in N-limited systems include both biotic and abiotic explanations, and this pattern appears robust across ecosystems (Treseder, 2008).
Ecosystem Processes: Soil Carbon Dynamics
Soils contain the largest terrestrial carbon stock, thus changes to the inputs, outputs, or turnover times of soil organic carbon (SOC) may affect atmospheric CO2 concentrations and climate (Schlesinger, 1997). Much research has been devoted to understanding how N deposition affects SOC storage in temperate ecosystems (Liu and Greaver, 2010; Lu et al., 2011), and the mechanisms that mediate such changes (Li et al., 2015b). If increased N deposition stimulates plant productivity, inputs to the soil from leaf or root litter may increase. Furthermore, N deposition may also repress lignolytic enzyme activity, causing decreases in organic matter decay rates and enhancing SOC storage (Eisenlord et al., 2013). Alternatively, changes in nutrient availability may increase root exudation, “priming” the decomposition of organic matter and decreasing soil C storage (Phillips et al., 2011). Understanding the net effects of these processes is further complicated by the fact that SOC is composed of diverse compounds or fractions that vary in turnover times and mechanisms of stabilization (Sollins et al., 1996), and labile, slow, and stable SOC fractions may respond differently to N or P addition (Cusack et al., 2010; Nottingham et al., 2015).
In an experiment in a wet tropical forest, long-term N addition reduced labile SOC pools, corresponding to increased hydrolytic enzyme activity (Cusack et al., 2010, 2011). However, overall slow SOC pools (and thus total SOC) increased, as a function of reductions in oxidative enzymes (Cusack et al., 2010, 2011). By contrast, in a large-scale experiment in 10 and 60-year old regenerating TDF in Mexico, 3 years of P fertilization decreased total SOC in the young, N-limited forest, but increased it in the P-limited older forest, with no significant effects of N or N+P addition (Gamboa et al., 2010). Together with laboratory incubation experiments (Bejarano et al., 2014b), these studies emphasize that the response of SOC in TDFs to N deposition may be complex, and vary according to the nutrient limitation status of plants and soil microbial biomass.
Ecosystem Processes: Nitrogen Cycling
N2 fixation, N mineralization, and (de)nitrification are all regulated by the availability of N. Evidence from wet forests suggests that legumes down-regulate symbiotic N2 fixation as soil N becomes more available through secondary succession (Batterman et al., 2013; Sullivan et al., 2014a). Similarly, rates of free-living N2 fixation in soils and the forest floor may decrease with increasing N availability, as seen in wet forests of Puerto Rico (Cusack et al., 2009). However, water availability may be a more important control on legume nodulation in TDFs (Gei and Powers, 2015), in which case N2 fixation rates may change little with nutrient addition. A fertilization study in a TDF in India showed that N pools and mineralization rates increased with added N (Verma et al., 2013); however, both nitrogen cycling (Saynes et al., 2005) and nutrient addition effects on N cycling in another TDF in Mexico depended on season and forest successional status (Solís and Campo, 2004). Last, nitric and nitrous oxide emissions should increase under N deposition, particularly during the wet season (Erickson et al., 2002; Wang et al., 2014), and especially from N-saturated or P-limited forests (Hall and Matson, 1999).
Conclusions and Directions for Future Research
Taken together, the evidence on tropical dry forest responses to nutrient availability suggests several robust generalizations (Table 1). First, tree species respond individually to amendments with different nutrients, which likely reflects differences in life history strategies and functional traits. Second, TDF ecosystem responses to increased N or P are context-dependent and are mediated by ecosystem state factors such as soil parent material, prior land-use history, species composition, and both intra- and interannual variation in water availability.
Our analysis also revealed large knowledge gaps that represent critical directions for future research (Table 1). A major uncertainty in TDF ecology is understanding the extent to which N, P, or other elements most limit productivity, the consequences of nutrient limitation under altered nutrient deposition regimes, and how belowground communities and processes respond (Table 1). Although many methods have been proposed to accomplish this (Sullivan et al., 2014b), the “gold standard” of ecosystem ecology remains large-scale fertilization experiments. Such experiments provide the opportunity to resolve nutrient addition effects and mechanisms across a hierarchy of scales from microbial to trees. Ideally, such experiments could be established along gradients of ecosystem state factors such as annual rainfall (Bejarano et al., 2014a) or forest age (Campo and Vázquez-Yanes, 2004), and in a greater diversity of dry forests including those in India and Africa, which are poorly represented in the literature. Most of the handful of field-scale fertilization experiments in TDF occurred in Mexico. Although these studies have provided valuable insights, establishing similar studies in a range of dry forests is critical if we are to advance our knowledge of TDF responses to global change (Fahey et al., 2015). Moreover, we need to look beyond N and P to the other elements that affect forest processes (Kaspari et al., 2009; Powers and Salute, 2011). Such knowledge is necessary for both accurately representing TDF dynamics in ecosystem simulation models and managing TDF under anthropogenic nutrient deposition.
Conflict of Interest Statement
The authors declare that the research was conducted in the absence of any commercial or financial relationships that could be construed as a potential conflict of interest.
Acknowledgments
JP gratefully acknowledges funding from the US National Science Foundation CAREER grant DEB 1053237.
References
Alvarez-Clare, S., Mack, M. C., and Brooks, M. (2013). A direct test of nitrogen and phosphorus limitation to net primary productivity in a lowland tropical wet forest. Ecology 94, 1540–1551. doi: 10.1890/12-2128.1
Anaya, C. A., Jaramillo, V. J., Martinez-Yrizar, A., and Garcia-Oliva, F. (2012). Large rainfall pulses control litter decomposition in a tropical dry forest: evidence from an 8-Year Study. Ecosystems 15, 652–663. doi: 10.1007/s10021-012-9537-z
Auge, R. M. (2001). Water relations, drought and vesicular-arbuscular mycorrhizal symbiosis. Mycorrhiza 11, 3–42. doi: 10.1007/s005720100097
Auge, R. M. (2004). Arbuscular mycorrhizae and soil/plant water relations. Can. J. Soil Sci. 84, 373–381. doi: 10.4141/S04-002
Barger, N. N., D'Antonio, C. M., Ghneim, T., Brink, K., and Cuevas, E. (2002). Nutrient limitation to primary productivity in a secondary savanna in Venezuela. Biotropica 34, 493–501. doi: 10.1111/j.1744-7429.2002.tb00569.x
Batterman, S. A., Hedin, L. O., Van Breugel, M., Ransijn, J., Craven, D. J., and Hall, J. S. (2013). Key role of symbiotic dinitrogen fixation in tropical forest secondary succession. Nature 502, 224–227. doi: 10.1038/nature12525
Bejarano, M., Crosby, M. M., Parra, V., Etchevers, J. D., and Campo, J. (2014a). Precipitation regime and nitrogen addition effects on leaf litter decomposition in tropical dry forests. Biotropica 46, 415–424. doi: 10.1111/btp.12127
Bejarano, M., Etchevers, J. D., Ruiz-Suarez, G., and Campo, J. (2014b). The effects of increased N input on soil C and N dynamics in seasonally dry tropical forests: an experimental approach. Appl. Soil Ecol. 73, 105–115. doi: 10.1016/j.apsoil.2013.08.015
Bobbink, R., K., Hicks, J., Galloway, T., Spranger, R., Alkemade, M., et al. (2010). Global assessment of nitrogen deposition effects on terrestrial plant diversity: a synthesis. Ecol. Appl. 20, 30–59. doi: 10.1890/08-1140.1
Bradford, M. A., Keiser, A. D., Davies, C. A., Mersmann, C. A., and Strickland, M. S. (2013). Empirical evidence that soil carbon formation from plant inputs is positively related to microbial growth. Biogeochemistry 113, 271–281. doi: 10.1007/s10533-012-9822-0
Camenzind, T., Hempel, S., Homeier, J., Horn, S., Velescu, A., Wilcke, W., et al. (2014). Nitrogen and phosphorus additions impact arbuscular mycorrhizal abundance and molecular diversity in a tropical montane forest. Glob. Change Biology 20, 3646–3659. doi: 10.1111/gcb.12618
Campo, J., and Dirzo, R. (2003). Leaf quality and herbivory responses to soil nutrient addition in secondary tropical dry forests of Yucatan, Mexico. J. Trop. Ecol. 19, 525–530. doi: 10.1017/s0266467403003572
Campo, J., Solis, E., and Gallardo, J. F. (2012). Effects of fertilisation on soil nutrient characteristics and the growth of tree stand in secondary seasonally dry tropical forests in Mexico. J. Trop.For. Sci. 24, 408–415.
Campo, J., Solís, E., and Valencia, M. G. (2007). Litter N and P dynamics in two secondary tropical dry forests after relaxation of nutrient availability constraints. For. Ecol. Manage. 252, 33–40. doi: 10.1016/j.foreco.2007.06.022
Campo, J., and Vázquez-Yanes, C. (2004). Effects of nutrient limitation on aboveground carbon dynamics during tropical dry forest regeneration in Yucatan, Mexico. Ecosystems 7, 311–319. doi: 10.1007/s10021-003-0249-2
Ceccon, E., Huante, P., and Campo, J. (2003). Effects of nitrogen and phosphorus fertilization on the survival and recruitment of seedlings of dominant tree species in two abandoned tropical dry forests in Yucatán, Mexico. For. Ecol. Manage. 182, 387–402. doi: 10.1016/S0378-1127(03)00085-9
Ceccon, E., Sánchez, S., and Campo, J. (2004). Tree seedling dynamics in two abandoned tropical dry forests of differing successional status in Yucatán, Mexico: a field experiment with N and P fertilization Plant. Ecology 170, 277–285. doi: 10.1023/B:VEGE.0000021699.63151.47
Chadwick, O. A., Derry, L. A., Vitousek, P. M., Huebert, B. J., and Hedin, L. O. (1999). Changing sources of nutrients during four million years of ecosystem development. Nature 397, 491–497.
Chen, H., Dong, S. F., Liu, L., Ma, C. A., Zhang, T., Zhu, X. M., et al. (2013). Effects of Experimental nitrogen and phosphorus addition on litter decomposition in an old-growth tropical forest. PLoS ONE 8:e84101. doi: 10.1371/journal.pone.0084101
Cleveland, C. C., and Liptzin, D. (2007). C: N: P stoichiometry in soil: is there a “Redfield ratio” for the microbial biomass? Biogeochemistry 85, 235–252. doi: 10.1007/s10533-007-9132-0
Cleveland, C. C., Townsend, A. R., and Schmidt, S. K. (2002). Phosphorus limitation of microbial processes in moist tropical forests: evidence from short-term laboratory incubations and field studies. Ecosystems 5, 680–691. doi: 10.1007/s10021-002-0202-9
Cleveland, C. C., Townsend, A. R., Schmidt, S. K., and Constance, B. C. (2003). Soil microbial dynamics and biogeochemistry in tropical forests and pastures, southwestern Costa Rica. Ecol. Appl. 13, 314–326. doi: 10.1890/1051-0761(2003)013[0314:SMDABI]2.0.CO;2
Cleveland, C. C., Townsend, A. R., Taylor, P., Alvarez-Clare, S., Bustamante, M. M. C., Chuyong, G., et al. (2011). Relationships among net primary productivity, nutrients and climate in tropical rain forest: a pan-tropical analysis. Ecol. Lett. 14, 939–947. doi: 10.1111/j.1461-0248.2011.01658.x
Condit, R., Engelbrecht, B. M. J., Pino, D., Perez, R., and Turner, B. L. (2013). Species distributions in response to individual soil nutrients and seasonal drought across a community of tropical trees. Proc. Natl. Acad. Sci. U.S.A. 110, 5064–5068. doi: 10.1073/pnas.1218042110
Cotrufo, M. F., Wallenstein, M. D., Boot, C. M., Denef, K., and Paul, E. (2013). The Microbial Efficiency-Matrix Stabilization (MEMS) framework integrates plant litter decomposition with soil organic matter stabilization: do labile plant inputs form stable soil organic matter? Glob. Change Biology 19, 988–995. doi: 10.1111/gcb.12113
Cusack, D. F., Silver, W. L., and McDowell, W. H. (2009) Biological nitrogen fixation in two tropical forests: ecosystem-level patterns and effects of nitrogen fertilization. Ecosystems 12, 1299–1315. doi: 10.1007/s10021-009-9290-0
Cusack, D. F., Silver, W. L., Torn, M. S., Burton, S. D., and Firestone, M. K. (2011). Changes in microbial community characteristics and soil organic matter with nitrogen additions in two tropical forests. Ecology 92, 621–632. doi: 10.1890/10-0459.1
Cusack, D. F., Torn, M. S., Mcdowell, W. H., and Silver, W. L. (2010). The response of heterotrophic activity and carbon cycling to nitrogen additions and warming in two tropical soils. Glob. Change Biol. 16, 2555–2572. doi: 10.1111/j.1365-2486.2009.02131.x
Davidson, E. A., Reisdecarvalho, C. J., Figueira, A. F., Ishida, F. Y., Ometto, J. P. H. B., Nardoto, G. B., et al. (2007). Recuperation of nitrogen cycling in Amazonian forests following agricultural abandonment. Nature 447, 995–998. doi: 10.1038/nature05900
Eamus, D. (1999). Ecophysiological traits of deciduous and evergreen woody species in the seasonally dry tropics. Trends Ecol. Evol. 14, 11–16.
Eisenlord, S. D., Freedman, Z., Zak, D. R., Xue, K., He, Z. L., and Zhou, J. Z. (2013). Microbial mechanisms mediating increased soil C storage under elevated atmospheric N deposition. Appl. Environ. Microbiol. 79, 1191–1199. doi: 10.1128/aem.03156-12
Erickson, H., Davidson, E. A., and Keller, M. (2002). Former land-use and tree species affect nitrogen oxide emissions from a tropical dry forest. Oecologia 130, 297–308. doi: 10.1007/s004420100801
Fahey, T. J., Templer, P. H., Anderson, B. T., Battles, J. J., Campbell, J. L., Driscoll, C. T., et al. (2015). The promise and peril of intensive-site-based ecological research: insights from the Hubbard Brook ecosystem study. Ecology 96, 885–901. doi: 10.1016/j.soilbio.2015.01.001
Frey, S. D., Ollinger, S., Nadelhoffer, K., Bowden, R., Brzostek, E., Burton, A., et al. (2014). Chronic nitrogen additions suppress decomposition and sequester soil carbon in temperate forests. Biogeochemistry 121, 305–316. doi: 10.1007/s10533-014-0004-0
Galicia, L., and Garcia-Oliva, F. (2004). The effects of C, N and P additions on soil microbial activity under two remnant tree species in a tropical seasonal pasture. Appl. Soil Ecol. 26, 31–39. doi: 10.1016/j.apsoil.2003.10.006
Galloway, J. N., Dentener, F. J., Capone, D. G., Boyer, E. W., Howarth, R. W., Seitzinger, S. P., et al. (2004). Nitrogen cycles: past, present, and future. Biogeochemistry 70, 153–226. doi: 10.1007/s10533-004-0370-0
Gamboa, A. M., Hidalgo, C., De Leon, F., Etchevers, J. D., Gallardo, J. F., and Campo, J. (2010). Nutrient addition differentially affects soil carbon sequestration in secondary tropical dry forests: early- versus late-succession stages. Restor. Ecol. 18, 252–260. doi: 10.1111/j.1526-100X.2008.00432.x
Gei, M. G., and Powers, J. S. (2014). “Nutrient cycling in tropical dry forests,” in Tropical Dry Forests in the Americas: Ecology, Conservation, and Management, eds G. A. Sanchez-Azofeifa, J. S. Powers, G. W. Fernandes and M. Quesada (Boca Raton, FL: CRC Press), 141–155.
Gei, M. G., and Powers, J. S. (2015). The influence of seasonality and species effects on surface fine roots and nodulation in tropical legume tree plantations. Plant Soil 388, 187–196. doi: 10.1007/s11104-014-2324-1
Geisseler, D., and Scow, K. M. (2014). Long-term effects of mineral fertilizers on soil microorganisms - a review. Soil Biol. Biochem. 75, 54–63. doi: 10.1016/j.soilbio.2014.03.023
Hall, S. J., and Matson, P. A. (1999). Nitrogen oxide emissions after nitrogen additions in tropical forests. Nature 400, 152–155.
Hedin, L. O., Brookshire, E. N. J., Menge, D. N. L., and Barron, A. R. (2009). The nitrogen paradox in tropical forest ecosystems. Annu. Rev. Ecol. Evol. Syst. 40, 613–635. doi: 10.1146/annurev.ecolsys.37.091305.110246
Hietz, H., Turner, B. L., Wanek, W., Richter, A., Nock, C. A., and Wright, S. J. (2011). Long-term change in the nitrogen cycle of tropical forests. Science 334, 664–666. doi: 10.1126/science.1211979
Hobbie, S. E., and Vitousek, P. M. (2000). Nutrient limitation of decomposition in Hawaiian forests. Ecology 81, 1867–1877. doi: 10.1890/0012-9658(2000)081[1867:NLODIH]2.0.CO;2
Huante, P., Rincon, E., and Acosta, I. (1995a). Nutrient availability and growth rate of 34 woody species from a tropical deciduous forest in Mexico. Funct. Ecol. 9, 849–858
Huante, P., Rincon, E., and Chapin, F. S. (1995b). Responses to phosphorus of contrasting successional tree seedling species from the tropical deciduous forest of Mexico. Funct. Ecol. 9, 760–766. doi: 10.2307/2390249
Jenny, H. (1941). Factors of Soil Formation: A System of Quantitative Pedology. New York, NY: McGraw-Hill.
Johnson, N. C., Rowland, D. L., Corkidi, L., Egerton-Warburton, L. M., and Allen, E. B. (2003). Nitrogen enrichment alters mycorrhizal allocation at five mesic to semiarid grasslands. Ecology 84, 1895–1908. doi: 10.1890/0012-9658(2003)084[1895:NEAMAA]2.0.CO;2
Johnson, N. C., Wilson, G. W. T., Wilson, J. A., Miller, R. M., and Bowker, M. A. (2015). Mycorrhizal phenotypes and the Law of the Minimum. New Phytol. 205, 1473–1484. doi: 10.1111/nph.13172
Kaspari, M., Yanoviak, S. P., Dudley, R., Yuan, M., and Clay, N. A. (2009). Sodium shortage as a constraint on the carbon cycle in an inland tropical rainforest. Proc. Nat. Acad. Sci. U.S.A. 106, 19405–19409. doi: 10.1073/pnas.0906448106
Kauffman, J. B., Sanford R. L. Jr., Cummings, D. L., Salcedo, I. H., and Sampaio, E. V. S. B. (1993). Biomass and nutrient dynamics associated with slash fires in neotropical dry forests. Ecology 74, 140–151.
Khurana, E., and Singh, J. S. (2004). Impact of elevated nitrogen inputs on seedling growth of five dry tropical tree species as affected by life-history traits. Can. J. Bot. 82, 158–167. doi: 10.1139/b03-132
Krashevska, V., Dandmann, D., Maraun, M., and Scheu, S. (2014). Moderate changes in nutrient input alter tropical microbial and protest communities and belowground linkages. ISME 8, 1126–1134. doi: 10.1038/ismej.2013.209
Kwabiah, A. B., Voroney, R. P., Palm, C. A., and Stoskopf, N. C. (1999). Inorganic fertilizer enrichment of soil: effect on decomposition of plant litter under subhumid tropical conditions. Biol. Fertil. Soils 30, 224–231. doi: 10.1007/s003740050612
Lambert, J. D. H., Arnason, J. T., and Gale, J. L. (1980). Leaf-litter and changing nutrient levels in seasonally dry tropical hardwood forest, Belize, CA. Plant Soil 55, 429–443. doi: 10.1007/bf02182703
Lawrence, D. (2003). The response of tropical tree seedlings to nutrient supply: meta-analysis for understanding a changing tropical landscape. J. Trop. Ecol. 19, 239–250. doi: 10.1017/S0266467403003274
Lehto, T., and Zwiazek, J. (2011). Ectomycorrhizas and water relations of trees: a review. Mycorrhiza 21, 71–90. doi: 10.1007/s00572-010-0348-9
Li, J., Li, Z. A., Wang, F. M., Zou, B., Chen, Y., Zhao, J., et al. (2015a). Effects of nitrogen and phosphorus addition on soil microbial community in a secondary tropical forest of China. Biol. Fertil. Soils 51, 207–215. doi: 10.1007/s00374-014-0964-1
Li, W., Jin, C., Guan, D., Wang, Q., Wang, A., Yuan, F., et al. (2015b). The effects of simulated nitrogen deposition on plant root traits: a meta-analysis. Soil Biol. Biochem. 82, 112–118. doi: 10.1016/j.soilbio.2015.01.001
Liu, L. L., and Greaver, T. L. (2010). A global perspective on belowground carbon dynamics under nitrogen enrichment. Ecol. Lett. 13, 819–828. doi: 10.1111/j.1461-0248.2010.01482.x.
Lu, M., Zhou, X. H., Luo, Y. Q., Yang, Y. H., Fang, C. M., Chen, J. K., et al. (2011). Minor stimulation of soil carbon storage by nitrogen addition: a meta-analysis. Agric. Ecosyst. Environ. 140, 234–244. doi: 10.1016/j.agee.2010.12.010
Lv, Y. N., Wang, C. Y., Wang, F. Y., Zhao, G. Y., Pu, G. Z., Ma, X., et al. (2013). Effects of nitrogen addition on litter decomposition, soil microbial biomass, and enzyme activities between leguminous and non-leguminous forests. Ecol. Res. 28, 793–800. doi: 10.1007/s11284-013-1060-y
Lv, Y., Wang, C. Y., Jia, Y. Y., Wang, W. W., Ma, X., Du, J. J., et al. (2014). Effects of sulfuric, nitric, and mixed acid rain on litter decomposition, soil microbial biomass, and enzyme activities in subtropical forests of China. Appl. Soil Ecol. 79, 1–9. doi: 10.1016/j.apsoil.2013.12.002
Manzoni, S., Schaeffer, S. M., Katul, G., Porporato, A., and Schimel, J. P. (2014). A theoretical analysis of microbial eco-physiological and diffusion limitations to carbon cycling in drying soils. Soil Biol. Biochem. 73, 69–83. doi: 10.1016/j.soilbio.2014.02.008
Meentemeyer, V. (1978). Macroclimate and lignin control of litter decomposition rates. Ecology 59, 465–472.
Mendieta-Araica, B., Sporndly, E., Reyes-Sanchez, N., Salmeron-Miranda, F., and Halling, M. (2013). Biomass production and chemical composition of Moringa oleifera under different planting densities and levels of nitrogen fertilization. Agroforestry Syst. 87, 81–92. doi: 10.1007/s10457-012-9525-5
Montano, N. M., Garcia-Oliva, F., and Jaramillo, V. J. (2007). Dissolved organic carbon affects soil microbial activity and nitrogen dynamics in a Mexican tropical deciduous forest. Plant Soil 295, 265–277. doi: 10.1007/s11104-007-9281-x
Mooshammer, M., Wanek, W., Schnecker, J., Wild, B., Leitner, S., Hofhansl, F., et al. (2012). Stoichiometric controls of nitrogen and phosphorus cycling in decomposing beech leaf litter. Ecology 93, 770–782. doi: 10.1890/11-0721.1
Murphy, P. G., and Lugo, A. E. (1986). Ecology of tropical dry forest. Ann. Rev. Ecol. Syst. 17, 67–88.
Nottingham, A. T., Turner, B. L., Stott, A. W., and Tanner, E. V. J. (2015). Nitrogen and phosphorus constrain labile and stable carbon turnover in lowland tropical forest soils. Soil Biol. Biochem. 80, 26–33. doi: 10.1016/j.soilbio.2014.09.012
Phillips, R. P., Finzi, A. C., and Bernhardt, E. S. (2011). Enhanced root exudation induces microbial feedbacks to N cycling in a pine forest under long-term CO2 fumigation. Ecol Lett. 14, 187–194. doi: 10.1111/j.1461-0248.2010.01570.x
Powers, J. S., and Salute, S. (2011). Macro- and micronutrient effects on decomposition of leaf litter from two tropical tree species: inferences from a short-term laboratory incubation. Plant Soil 346, 245–257. doi: 10.1007/s11104-011-0815-x
Rauch, J. N., and Pacyna, J. M. (2009). Earth's global Ag, Al, Cr, Cu, Fe, Ni, Pb, and Zn cycles. Glob. Biogeochem. Cycles 23, 1–16. doi: 10.1029/2008GB003376
Read, L., and Lawrence, D. (2006). “Interactions between water availability and nutrient cycling in tropical dry forests,” in Dryland Ecohydrology, eds P. D'Odorico and A. Porporato (Netherlands: Springer), 217–232.
Reich, P. B., and Oleksyn, J. (2004). Global patterns of plant leaf N and P in relation to temperature and latitude. Proc. Natl. Acad. Sci. U.S.A. 101, 11001–11006. doi: 10.1073/pnas.0403588101
Rousk, J., and Baath, E. (2007). Fungal and bacterial growth in soil with plant materials of different C/N ratios. FEMS Microbiol. Ecol. 62, 258–267. doi: 10.1111/j.1574-6941.2007.00398.x
Salinas-Peba, L., Parra-Tabla, V., Campo, J., and Munguia-Rosas, M. A. (2014). Survival and growth of dominant tree seedlings in seasonally tropical dry forests of Yucatan: site and fertilization effects. J. Plant Ecol. 7, 470–479. doi: 10.1093/jpe/rtt055
Saynes, V., Hidalgo, C., Etchevers, J. D., and Campo, J. E. (2005). Soil C and N dynamics in primary and secondary seasonally dry tropical forests in Mexico. Appl. Soil Ecol. 29, 282–289. doi: 10.1016/j.apsoil.2004.11.007
Schimel, J. P., and Weintraub, M. N. (2003). The implications of exoenzyme activity on microbial carbon and nitrogen limitation in soil: a theoretical model. Soil Biol. Biochem. 35, 549–563. doi: 10.1016/s0038-0717(03)00015-4
Siddique, I., Vieira, I. C. G., Schmidt, S., Lamb, D., Carvalho, C. J. R., Figueiredo, R. D., et al. (2010). Nitrogen and phosphorus additions negatively affect tree species diversity in tropical forest regrowth trajectories. Ecology 91, 2121–2131. doi: 10.1890/09-0636.1
Sinsabaugh, R. L., Manzoni, S., Moorhead, D. L., and Richter, A. (2013). Carbon use efficiency of microbial communities: stoichiometry, methodology and modelling. Ecol. Lett. 16, 930–939. doi: 10.1111/ele.12113
Smil, V. (2000). Phosphorus in the environment: natural flows and human interfaces. Ann. Rev. Energy Environ. 25, 53–88. doi: 10.1146/annurev.energy.25.1.53
Solís, E., and Campo, J. (2004). Soil N and P dynamics in two secondary tropical dry forests after fertilization. For. Ecol. Manage. 195, 409–418. doi: 10.1016/j.foreco.2004.03.006
Sollins, P., Homann, P., and Caldwell, B. A. (1996). Stabilization and destabilization of soil organic matter: mechanisms and controls. Geoderma 74, 65–105.
Sullivan, B. W., Alvarez-Clare, S., Castle, S. C., Porder, S., Reed, S. C., Schreeg, L., et al. (2014b). Assessing nutrient limitation in complex forested ecosystems: alternatives to large-scale fertilization experiments. Ecology 95, 668–681. doi: 10.1890/13-0825.1
Sullivan, B. W., Smith, W. K., Townsend, A. R., Nasto, M. K., Reed, S. C., Chazdon, R. L., et al. (2014a). Spatially robust estimates of biological nitrogen (N) fixation imply substantial human alteration of the tropical N cycle. Proc. Natl. Acad. Sci. U.S.A. 111, 8101–8106. doi: 10.1073/pnas.1320646111
Torres, A., and Franco, W. (1994). Growth of plantations of Pinus caribaea var hondurensis in response to fertilization with rock phosphate and boraz in eastern Venezuela. Interciencia 19, 374–379.
Townsend, A. R., Asner, G. P., and Cleveland, C. C. (2008). The biogeochemical heterogeneity of tropical forests. Tree 23, 424–431. doi: 10.1016/j.tree.2008.04.009
Treseder, K. K. (2004). A meta-analysis of mycorrhizal responses to nitrogen, phosphorus, and atmospheric CO2 in field studies. New Phytol. 164, 347–355. doi: 10.1111/j.1469-8137.2004.01159.x
Treseder, K. K. (2008). Nitrogen additions and microbial biomass: a meta-analysis of ecosystem studies. Ecol. Lett. 11, 1111–1120. doi: 10.1111/j.1461-0248.2008.01230.x
Tripathi, S. N., and Raghubanshi, A. S. (2014). Seedling growth of five tropical dry forest tree species in relation to light and nitrogen gradients. J. Plant Ecol. 7, 250–263. doi: 10.1093/jpe/rtt026
Turner, B. L., and Wright, S. J. (2014). The response of microbial biomass and hydrolytic enzymes to a decade of nitrogen, phosphorus, and potassium addition in a lowland tropical rain forest. Biogeochemistry 117, 115–130. doi: 10.1007/s10533-013-9848-y
Verma, P., Verma, P., and Sagar, R. (2013). Variations in N mineralization and herbaceous species diversity due to sites, seasons, and N treatments in a seasonally dry tropical environment of India. For. Ecol. Manage. 297, 15–26. doi: 10.1016/j.foreco.2013.02.006
Vitousek, P. M. (1999). Nutrient limitation to nitrogen fixation in young volcanic sites. Ecosystems 2, 505–510.
Walker, T. W., and Syers, J. K. (1976). The fate of phosphorus during pedogenesis. Geoderma 15, 1–19.
Wang, F. M., Li, J., Wang, X. L., Zhang, W., Zou, B., Neher, D. A., et al. (2014). Nitrogen and phosphorus addition impact soil N2O emission in a secondary tropical forest of South China. Sci. Rep. 4, 1–8. doi: 10.1038/srep05615
Waring, B. G., Averill, C., and Hawkes, C. V. (2013). Differences in fungal and bacterial physiology alter soil carbon and nitrogen cycling: insights from meta-analysis and theoretical models. Ecol. Lett. 16, 887–894. doi: 10.1111/ele.12125
Warren, J. M., Hanson, P. J., Iversen, C. M., Kumar, J., Walker, A. P., and Wullschleger, S. D. (2015). Root structural and functional dynamics in terrestrial biosphere models - evaluation and recommendations. New Phytol. 205, 59–78. doi: 10.1111/nph.13034
Wright, S. J., Yavitt, J. B., Wurzburger, N., Turner, B. L., Tanner, E. V. J., Sayer, E. J., et al. (2011). Potassium, phosphorus, or nitrogen limit root allocation, tree growth, or litter production in a lowland tropical forest. Ecology 92, 1616–1625. doi: 10.1890/10-1558.1
Keywords: tropical dry forest, nutrient limitation, ecosystem processes, carbon cycling, decomposition, nitrogen deposition, nitrogen cycling
Citation: Powers JS, Becklund KK, Gei MG, Iyengar SB, Meyer R, O'Connell CS, Schilling EM, Smith CM, Waring BG and Werden LK (2015) Nutrient addition effects on tropical dry forests: a mini-review from microbial to ecosystem scales. Front. Earth Sci. 3:34. doi: 10.3389/feart.2015.00034
Received: 04 May 2015; Accepted: 16 June 2015;
Published: 30 June 2015.
Edited by:
Juergen Homeier, University of Goettingen, GermanyReviewed by:
Carlos A. Sierra, Max Planck Institute for Biogeochemistry, GermanyJulio Campo, Universidad Nacional Autónoma de México, Mexico
Copyright © 2015 Powers, Becklund, Gei, Iyengar, Meyer, O'Connell, Schilling, Smith, Waring and Werden. This is an open-access article distributed under the terms of the Creative Commons Attribution License (CC BY). The use, distribution or reproduction in other forums is permitted, provided the original author(s) or licensor are credited and that the original publication in this journal is cited, in accordance with accepted academic practice. No use, distribution or reproduction is permitted which does not comply with these terms.
*Correspondence: Jennifer S. Powers, Department of Ecology, Evolution, and Behavior, 1987 Upper Buford Circle, University of Minnesota, Saint Paul, MN 55108, USA,cG93ZXJzQHVtbi5lZHU=