- 1University of Campania “Luigi Vanvitelli”, Naples, Italy
- 2IRCCS San Raffaele Pisana, Rome, Italy
- 3Department of Gastroenterology, Hepatology and Infectious Diseases, Otto-von-Guericke University Hospital, Magdeburg, Germany
- 4Department of Internal Medicine II, LMU University Hospital, Munich, Germany
- 5Nefrocenter Research Network & Nyx Research Start-Up, Naples, Italy
- 6Health Sciences Department, Anna Meyer Children’s University Hospital, University of Florence, Florence, Italy
- 7Internal Medicine and Hepatology Division, Department of Medicine, Surgery and Odontostomatology “Scuola Medica Salernitana”, University of Salerno, Baronissi, Italy
Introduction: Metabolic syndrome (MetS) is increasingly common in adults as well as in children and adolescents. However, preventing and treating MetS is one of the most pressing challenges for public health services worldwide. At present, the only approved treatments for MetS are dietary changes and physical activity, which are associated with a high rate of non-compliance. On the contrary, no drugs are licensed to treat metabolic syndrome, although a number of drugs are used to treat individual metabolic abnormalities, which increases the risk of adverse events, particularly in children. Policaptil Gel Retard® (PGR), an oral macromolecule complex based on polysaccharides, has been demonstrated to significantly reduce body weight, peak blood glucose (BG) levels, insulin levels, and lipid levels, providing an interesting non-pharmacological therapeutic option for MetS-associated metabolic abnormalities, especially in younger patients.
Aims: To review available studies on the use of PGR in children, adolescents, or adults with obesity or metabolic syndrome.
Methods: A systematic search of electronic databases for PGR and MetS. A total of six studies were identified and included.
Results: Across four randomized clinical studies and one retrospective clinical study including a total of 359 obese children and adolescents with or without MetS and 157 overweight/obese adults with or without MetS and/or T2DM, a single dose of PGR resulted in a reduction in appetite and postprandial triglyceride levels in younger patients and peak postprandial BG levels in adults. Decreased lipid levels were observed in adults following a normocaloric diet who received PGR for 30 days. As a long-term treatment, in combination with a low-glycemic index diet with or without metformin, PGR resulted in reduced body mass index and waist circumference, improved insulin sensitivity with reduction of glucose-metabolism abnormalities, increased insulin reserve and, finally, an improved circulating lipid profile, regardless of age. No safety issues were reported.
Conclusion: Policaptil Gel Retard® is an effective and safe non-pharmacological approach to improve the treatment of MetS-associated cardiovascular risk factors in children, adolescents, and adults.
Introduction
Metabolic syndrome (MetS) is a clinical condition with a cluster of risk factors for cardiovascular diseases, including abdominal obesity, hypertension, dyslipidemia, and hyperglycemia or diabetes (Expert Panel on Detection E 2001; National Cholesterol Education Program Expert Panel on Detection E 2002). These factors are regarded as a syndrome rather than individual diseases because they share the same pathophysiological mechanism(s) (Mancia et al., 2010; Nsiah et al., 2015; James et al., 2020). The current diagnostic criteria for MetS in children, adolescents, and adults were defined in 2007 and 2009 by the International Diabetes Federation. Obesity (particularly abdominal obesity) is the primary criterion (waist circumference ≥95 cm in adult men or ≥80 cm in adult women), which should be associated with at least two of the additional criteria: triglycerides (TGs) ≥ 150 mg/dl; high-density lipoprotein (HDL) cholesterol ≤ 40 mg/dl in men or ≤ 50 mg/dl in women (or currently on lipid-lowering treatment); systolic blood pressure ≥ 130 mmHg or diastolic blood pressure ≥ 85 mmHg (or ongoing anti-hypertensive treatment); or fasting blood glucose (BG) ≥ 100 mg/dl (Alberti et al., 2009). In children and adolescents, due to substantial changes in the body mass index (BMI) during periods of growth, abdominal obesity is defined by a waist circumference exceeding the 89th percentile of patient’s age (Zimmet et al., 2007). The Western lifestyle and diet are the root causes of MetS, obesity, and, subsequently, type 2 diabetes mellitus (T2DM), which all increase health care burdens and costs (Nolan et al., 2011; Bhupathiraju and Hu 2016). Obesity and MetS are becoming “epidemic” among younger age groups, not only in high-income settings but also in developing countries (Kelishadi 2007; Collaborators et al., 2017; DeBoer 2019), making the development of effective treatments to prevent cardiovascular disease and liver-related morbidity and mortality of vital importance (Knowler et al., 2002). Dietary and general lifestyle changes often need to be combined with specific drugs to control single factors such as hypercholesterolemia, hyperglycemia, and hypertension associated with MetS (Koskinen et al., 2017; Rask Larsen et al., 2018; Wang et al., 2018; DeBoer 2019). One of the most used drugs is the insulin-sensitizing agent metformin (MTF). The use of MTF is not advised before overt T2DM has been diagnosed and should be avoided in younger patients (Axon et al., 2016; Weihe and Weihrauch-Blüher 2019; Davidson 2020).
Policaptil Gel Retard® (PGR, European patent no. 1679009) is a natural macromolecule complex of functional constituents of medicinal plants that are selected on the basis of emerging behavior (i.e., the behavior acquired by the final complex when its components are pooled together) in order to generate a final system that displays enhanced water binding and swelling capacities while promoting positive health outcomes (lower cholesterol, improved glycemic control, and normal stools) for which reproducible evidence of clinical efficacy has been published and linked to fiber intake, especially fiber with water-holding capacity (McRorie and McKeown 2017; Fornari et al., 2020). The PGR complex is obtained by combining different types of dietary fiber (from polysaccharide-enriched plant extracts) and processed raw materials, including glucomannan (from Amorphophallus konjac) (Martino et al., 2005; Fornari et al., 2020), cellulose (from Opuntia ficus-indica), chicory root (Cichorium intybus) (Pushparaj et al., 2007; Shim et al., 2016), and mucilage (from Althaea officinalis, Linum usitatissimum, and Tilia platyphyllos Scop) (Mani et al., 2011). Under EU Regulation 2017/745 and EU Directive 93/42/EC (as amended), PGR is classified as a medical device and is controlled for efficacy, safety, and production quality by certified third parties. Quality control includes the monitoring of chemical and physical parameters such as average weight, hardness, and weight loss on drying. Metabolomic fingerprinting is performed by near-infrared spectroscopy, and biological parameters are evaluated through water binding capacity and fiber tests. In the gastrointestinal tract, the PGR complex acts as an active, non-pharmacological system, which, in vitro and in experimental animal models, has been shown to reduce the availability of dietary components to both the microbiota and the epithelial cells involved in absorption (Greco et al., 2020).
Aim
This systematic review aims to analyze studies on the activity, efficacy, and safety of PGR in children, adolescents, and adults with obesity or MetS, including data obtained from an animal model study supporting its mechanisms of action.
Methods
We searched for literature studies in the most relevant electronic databases (MEDLINE, SCOPUS, EMBASE, PubMed, Web of Science, and CrossRef) by entering Policaptil Gel Retard® (PGR) as the keyword. A total of five clinical studies, of which four studies were in extenso, were retrieved and selected for inclusion in this systematic review. Statistical analysis: we reviewed the studies, extracted the results, and summarized them in tables and figures. We also performed a forest plot analysis to highlight the most critical changes in the explored metabolic parameters by using the program RevMan 5.4 for MacOs (Version 5.4, The Cochrane Collaboration, 2020).
Results
Among the clinical studies, one demonstrated the short-term effect (within 4 h) of PGR on blood glucose regulation in obese children, two dealt with the efficacy and safety of PGR in children/adolescents with obesity or MetS, and two (one abstract and one study in in extenso) involved overweight or obese adults with MetS or T2DM, as summarized in Table 1. All important parameters evaluated in the long-term studies (Stagi et al., 2015; Stagi et al., 2017; Guarino et al., 2021) are summarized in Figures 1–3, in which differences in BMI, HOMA-IR, total cholesterol, and TGs before and after PGR administration are reported, and in Supplementary Figure S1, in which the differences in LDL cholesterol per single study are reported. Figure 4 is a forest plot analysis showing a significant estimated mean difference in favor of PGR vs. control treatments when the individual studies are combined and averaged together for the following outcome measures: BMI SDS, HOMA-IR, total cholesterol, HDL, LDL, and TGs.
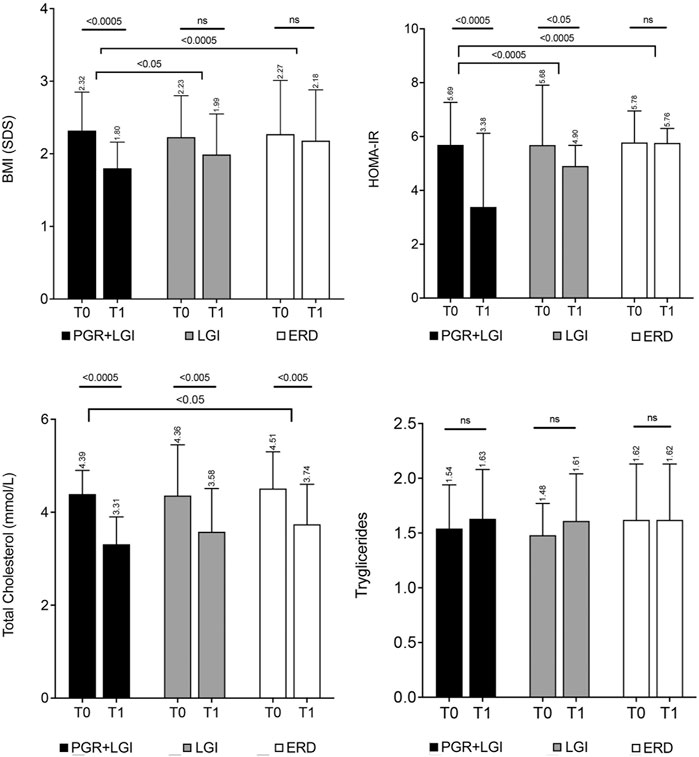
Figure 1. Principal metabolic parameters of the patients involved in the randomized study in children by Stagi et al. (2015). The four panels show the changes in BMI normalized for chronological age by conversion to standard deviation scores (BMI SDS), HOMA-IR, total cholesterol, and triglycerides in the three groups of the study (PGR + LGI: Policaptil Gel Retard plus low-glycemic index diet; LGI: low-glycemic index diet only; ERD: energy restriction diet) from T0 (baseline -first column) to T1 (after 1 year). The PGR-LGI group showed a significant improvement from T0 to T1 in BMI SDS, HOMA-IR, and total cholesterol (p < 0.0005) as well as a significantly greater decrease in BMI (p < 0.05) and HOMA-IR (p < 0.0005) than the LGI group. No significant improvement was obtained in the ERD group, other than that of total cholesterol (p < 0.05). The bars represent mean values and standard deviation.
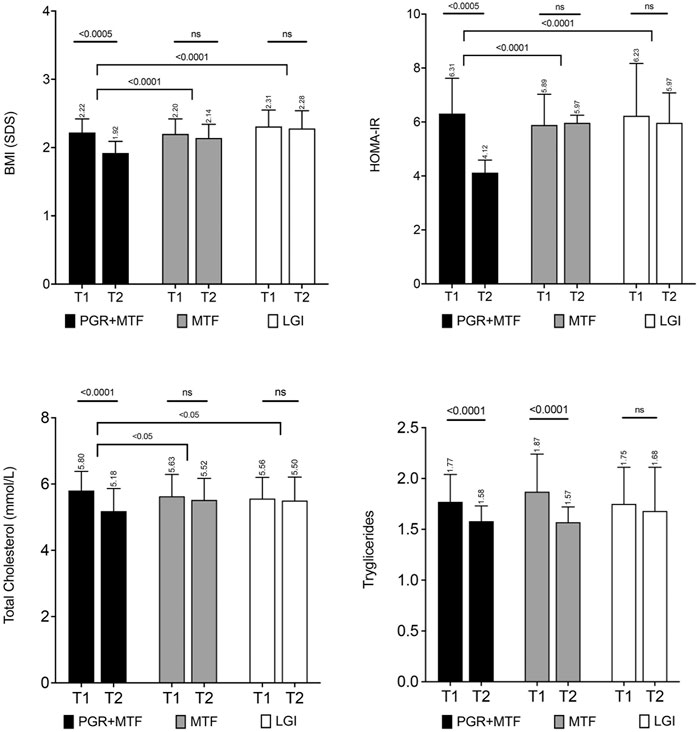
Figure 2. Principal metabolic parameters of the patients involved in the retrospective study in children conducted by Stagi et al. (2017). The four panels show the changes in BMI (SDS), HOMA-IR, total cholesterol, and triglycerides in the three groups whose data were collected (PGR + MTF: Policaptil Gel Retard plus metformin; MTF: metformin alone; LGI: low-glycemic index diet) from T1 (after 12 months of therapy) to T2 (after a further 12 months in which PGR was taken in addition to MTF by patients of the first group). Only the PGR + MTF group showed a significant improvement in BMI (SDS), HOMA-IR (p < 0.0005 for each), total cholesterol, and LDL cholesterol (see Supplementary Figure S1) (p < 0.0001 for each) together with that of triglycerides (p < 0.0001) in common with the MTF group. The bars represent mean values and standard deviation.
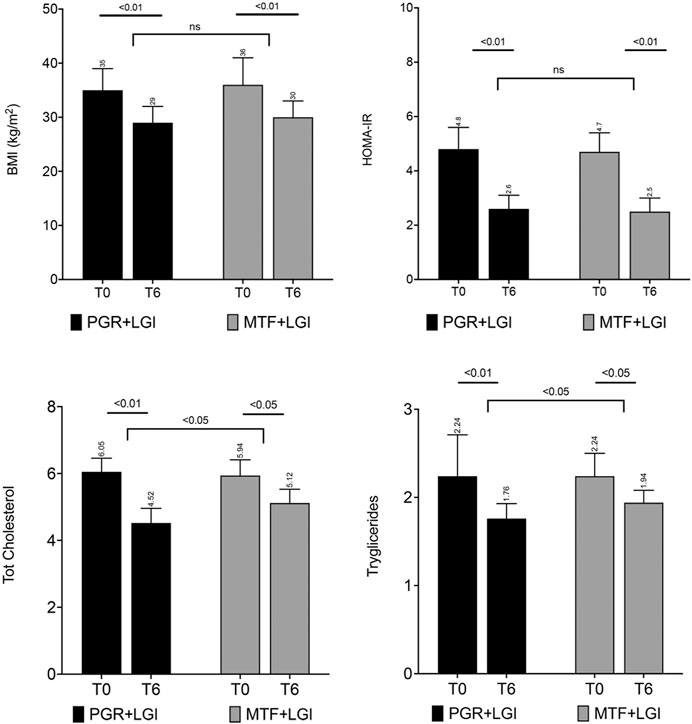
Figure 3. Principal metabolic parameters of the patients involved in the randomized study in adults by Guarino et al. (2021). The four panels show the changes in BMI (SDS), HOMA-IR, total cholesterol, and triglycerides in the two study groups (PGR + LGI: Policaptil Gel Retard plus low-glycemic index diet; MTF + LGI: metformin plus low-glycemic index diet) after 6 months of treatment (T6). Both groups showed significant improvements after treatment in BMI, HOMA-IR (p < 0.01 for each), total cholesterol, LDL cholesterol (see Supplementary Figure S1), and triglycerides, but a significant between-group difference (p < 0.05) in favor of PGR for all serum lipid parameters was also found. The bars represent mean values and standard deviation.
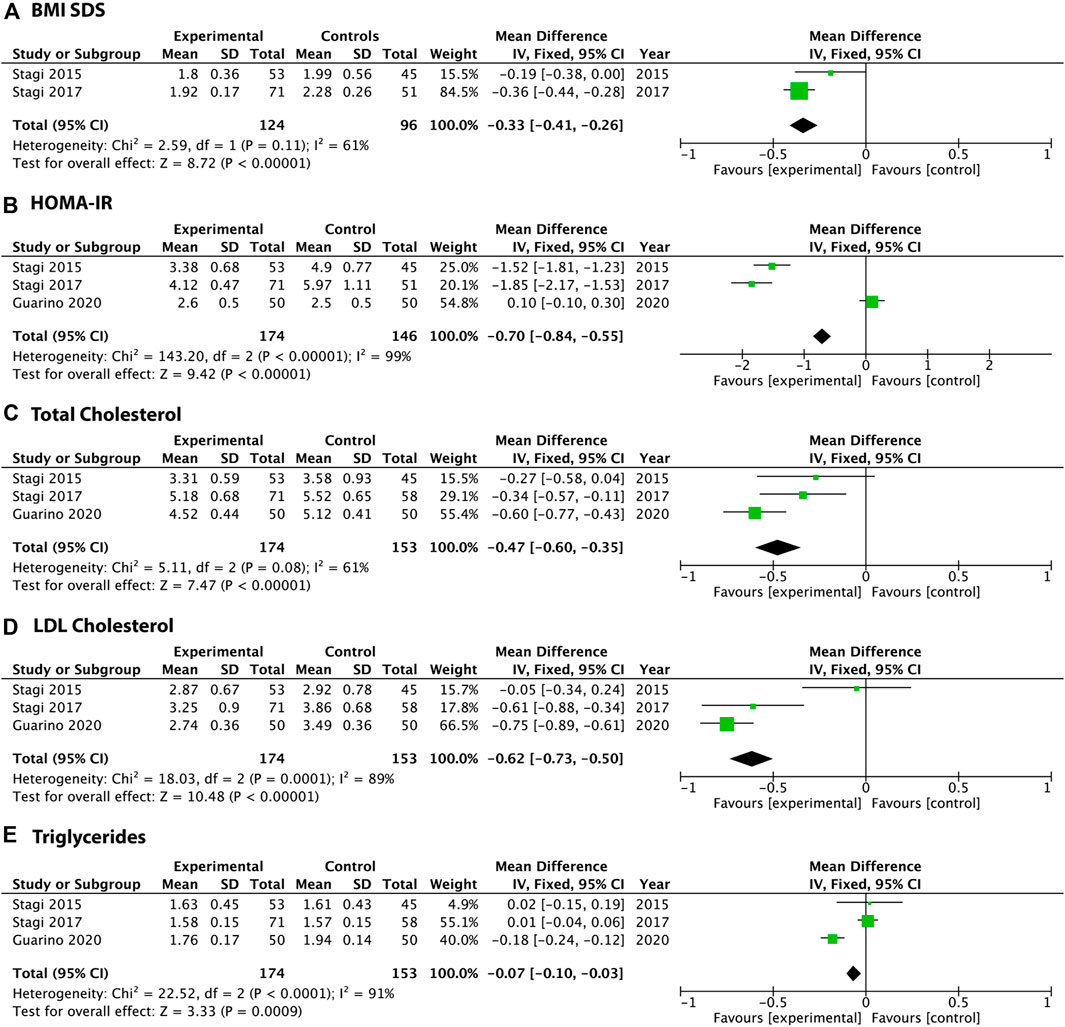
Figure 4. Forest plots of the principal metabolic parameters at the end of the treatment period in three clinical studies, published in extenso, that evaluated the administration of PGR to patients with metabolic dysfunction. Even if there were different study designs, different populations (children or adults either fulfilling or not fulfilling the current MetS diagnosis criteria), and different durations, the patients in the experimental groups treated with PGR demonstrated greater improvements in BMI, insulin sensitivity (reduction of HOMA-IR), total cholesterol, LDL cholesterol, and, less markedly, in triglycerides. The meta-analysis was performed with Review Manager software for Macintosh ((RevMan). To evaluate the homogeneity of the studies, we performed a homogeneity test based on the χ2 calculation. To overcome the low power of the test, a minimum cutoff p-value of 0.1 was established as a threshold for heterogeneity. We obtained the pooled estimates, with a relative confidence interval of 95%, through the use of random-effects models. The fourth human study (Belligoli and Vettor 2018) was excluded because of a lack of sufficient data to perform the calculations.
We focus on the clinical studies, grouping them according to the age of the participants; however, we also provide a comprehensive description of the preclinical study.
Studies in Children and Adolescents
In a longitudinal randomized clinical study, Stagi et al. (2015) evaluated the effects of PGR in 133 obese children and adolescents (with a BMI above the 95th percentile) with a family history of obesity and T2DM. The subjects were randomized into three different groups, each assigned a specific dietary regime: a low-glycemic index (LGI) diet with PGR, an LGI diet only, and a 30% energy-restricted diet (ERD) compared to individual daily energy requirements. Patients were followed up for 1 year. Baseline, 1-year laboratory measurements, and anthropometric data were compared. A significant decrease in BMI (converted to standard deviation scores, SDS, to normalize for chronological age) was achieved both in the LGI + PGR and LGI-only groups, as compared to baseline (BMI SDS LGI + PGR: T0 2.32 ± 0.53 vs. T1 1.80 ± 0.36, p < 0.0005; LGI-only: T0 2.23 ± 0.57 vs. T1 1.99 ± 0.56, p < 0.05; Figure 1). In the LGI + PGR group, glycosylated hemoglobin [HbA1c (percentage): T0 5.63 ± 0.54 vs. T1 5.37 ± 0.35%, p < 0.005], HOMA-IR [mean (range): T0 5.69 (3.60–9.92) vs. T1 3.38 (2.64–5.38), p < 0.0005; Figure 1], insulinogenic index [mean (range): T0 3.66 (1.90–9.25) vs. T1 2.79 (1.84–8.27), p < 0.05], and disposition index [mean (range): T0 4.42 (2.25–11.97) vs. T1 10.28 (5.36–21.65), p < 0.0005] significantly improved, compared to baseline, after 1 year, while only HOMA-IR [mean (range): T0 5.68 (2.00–10.90) vs. T1 4.90 (3.15–6.26), p < 0.05; Figure 1] and disposition index [mean (range): T0 4.01 (0.83–11.72) vs. T1 4.93 (1.53–7.27), p < 0.05] significantly improved, compared to baseline, in the LGI-only group. Change in mean absolute values of selected parameters of lipid metabolism (total cholesterol, triglycerides, and LDL cholesterol) together with those of the BMI and HOMA-index, error bars, and within- and between-group differences are reported in Figure 1 and Supplementary Figure S1. Total cholesterol levels improved in all groups with a more significant reduction in the LGI + PGR group (T0 4.39 ± 0.51 vs. T1 3.31 ± 0.59 mmol/L, p < 0.0005) than those in the ERD group (T0 4.51 ± 0.79 vs. T1 3.74 ± 0.86 mmol/L, p < 0.05), whereas TGs and LDL cholesterol were not affected by any of the treatments (p: ns in all groups, see also Supplementary Figure S1).
The addition of PGR to the LGI diet significantly reduced the occurrence of acanthosis nigricans, a hyperpigmentation of the skin that is often localized in body folds and on the forehead, which has been linked to insulin resistance and hyperinsulinemia (Sinha and Schwartz 2007). In fact, acanthosis nigricans, which was present at baseline in 13.2% (7/53) of subjects in the PGR + LGI diet group, was to be present in 5.6% (3/53) (p < 0.005) after treatment. This did not occur in the LGI and ERD diet groups where acanthosis nigricans was present in 13.3 and 11.4% of the subjects, respectively, before and after the dietary intervention.
The long-term effects of PGR were also investigated in a retrospective single center study analyzing three cohorts of patients to evaluate PGR added to MTF, both as an add-on to lifestyle intervention (Stagi et al., 2017). Data from 129 obese children and adolescents with MetS treated for a minimum of 2 years with MTF, who were followed at the pediatric hospital, were collected. As per clinical practice, in all patients, MTF dosage was progressively increased according to a pre-specified algorithm until reaching the maximum daily dose of 1,500 mg after 4 weeks. After 12 months of MTF, 71 patients voluntarily started PGR as an add-on treatment, whereas 58 patients continued on MTF alone. Both groups followed an LGI diet. The two groups were compared to an historical control cohort consisting of 51 age-, sex-, and BMI-matched subjects with obesity and MetS on an LGI diet only. The same selection criteria were applied for patient inclusion in all groups, which did not differ significantly in auxological and metabolic terms at baseline. Compared to the controls, over the first 12 months, MTF-treated patients displayed a significant reduction in BMI SDS (2.18 ± 0.21 vs. 2.31 ± 0.24, MTF and controls, respectively; p < 0.005) and waist SDS (2.78 ± 0.52 vs. 2.99 ± 0.61, MTF and controls, respectively; p < 0.05), as well as a significant increase on the Matsuda index (ISOGTT SDS: 1.51 ± 0.22 vs. 1.42 ± 0.24; MTF and controls; p < 0.05), i.e., the index of insulin sensitivity calculated from the oral glucose tolerance test (OGTT) (Matsuda and DeFronzo 1999). After the following 12 months, the combined MTF + PGR treatment significantly improved (MTF + PGR group vs. MTF-only group, respectively) BMI SDS (1.92 ± 0.17 vs. 2.14 ± 0.20, p < 0.001), waist SDS (2.42 ± 0.43 vs. 2.75 ± 0.43, p < 0.001), HOMA-IR (4.12 ± 0.47 vs. 5.97 ± 1.11, p < 0.001), HbA1c (5.71 ± 0.28 vs. 5.94 ± 0.26%, p < 0.001), total cholesterol (5.18 ± 0.68 vs. 5.52 ± 0.65 mmol/L, p < 0.005), HDL cholesterol (1.06 ± 0.11 vs. 0.92 ± 0.15 mmol/L, p < 0.001), LDL cholesterol (3.25 ± 0.90 vs. 3.86 ± 0.68 mmol/L, p < 0.001), the Matsuda index (ISOGTT SDS: 2.27 ± 0.52 vs. 1.65 ± 0.21, SDS, p < 0.001), insulinogenic index (2.24 ± 1.12 vs. 2.97 ± 1.98, p < 0.05), and disposition index (6.78 ± 2.99 vs. 4.92 ± 2.56, p < 0.005). Absolute values and both within- and between-group differences in BMI, HOMA-IR, total cholesterol, and TGs from the 12th (T1) to the 24th (T2) month are also shown in Figure 2. Since no significant differences between MTF and control groups were observed from the 12th to the 24th month across numerous analytical metabolic parameters, i.e., the HOMA-IR (Figure 2), Matsuda, insulinogenic, and disposition indices, as well as total (Figure 2) and LDL cholesterol (Supplementary Figure S2) (p: ns for each). The addition of PGR significantly extended and potentiated the positive effects of MTF treatment, allowing for significant further improvement of the adiposity parameters associated with a significant reduction in glucose, insulin, and lipid alterations. Of note, the aspartate aminotransferase (AST) and alanine aminotransferase (ALT) mean values, that were mildly elevated in all groups both at baseline (Patients T0: ALT: 57.23 ± 20.89 U/L; AST: 59.66 ± 27.53 U/L; Controls T0: ALT: 53.67 ± 18.91 U/L; AST: 55.21 ± 24.90 U/L) and after 12 months (Patients T1: ALT: 52.32 ± 20.24 U/L; AST: 53.76 ± 23.19 U/L; Controls T1: ALT: 51.45 ± 17.76 U/L; AST: 53.45 ± 22.84 U/L), showed a significant decrease, as compared to baseline, only in the MTF + PGR group (PGR T2: ALT: 40.02 ± 13.27 U/L; AST: 42.00 ± 17.10 U/L, p < 0.0001 vs. T1; MTF T2 ALT: 48.26 ± 14.98 U/L; AST 48.55 ± 16.53 U/L, p:ns vs. T1; Controls T2: ALT 55.67 ± 24.39 U/L; AST: 55.67 ± 24.39 U/L, p:ns vs. T1), with AST significantly decreasing, after PGR treatment, compared to controls (p < 0.005) and ALT compared to both the MTF and control groups (p < 0.05 vs. MFT and p < 0.0001 vs. controls). In these latter groups, mean values remained substantially unchanged.
This study also reports safety and adherence data. During the first 12 months of MTF only, adverse events (AE) were reported in 20.1% of cases (hypoglycemia 2.3%, diarrhea 6.2%, constipation 2.3%, flatulence 4.6%, and abdominal pain 7.0%). These symptoms were reduced or eliminated after a reduction of the dose of MTF with no need to interrupt MTF treatment. Following the addition of PGR between 12 and 24 months, AEs occurred in 16.9% of patients (hypoglycemia 2.8%, diarrhea 5.6%, flatulence 2.8%, and abdominal pain 5.6%), which was comparable to the rate reported before in the MTF-only group. Comparable rates of AEs were also observed in the MTF-only group between 12 and 24 months, except for abdominal pain, which had a frequency of 8.6%. No serious AEs were reported. The adherence to PGR therapy was 91%.
A short-term randomized, double-blind, placebo-controlled trial investigated postprandial changes in the metabolic state and appetite of 46 obese children (Fornari et al., 2020). At baseline and at selected intervals over the course of 4 hours following a mixed meal (15 kcal/kg of lean body mass) consumed 20 min after PGR or placebo, the following parameters were measured: lipid levels (TGs and non-esterified fatty acids (NEFA)), BG, insulin levels, ghrelin, glucagon-like peptide 1 (GLP-1), and appetite level, measured through visual analog scales (VAS), simplified for children (Flint et al., 2000). The scale was made up of color boxes to improve the children’s comprehension of subjective hunger. The incremental area under the curve (iAUCs) for each metabolite and hormone were compared. The increase after 240 min in TGs was significantly lower in the PGR group (+3,021 ± 2,879 mg/dl) as compared to placebo (+5,038 ± 3,738 mg/dl) (p:0.046). NEFA decreased similarly in both groups (p:ns). The iAUC of ghrelin was significantly lower after taking PGR (−8,179 ± 8,073 pg/ml) than after placebo (−2,800 ± 7,579 pg/ml) (p:0.026). Glucose, GLP-1, and insulin increased similarly for both PGR and placebo (p:ns for each), while the appetite score was significantly lower in the PGR group (−234 ± 274 vs. 36 ± 239; p:0.004).
Therefore, the data in children and adolescents demonstrate a positive short-term (i.e., immediately following the administration) effect of PGR on lipid metabolism and appetite in obese children compared to placebo.
Data from the aforementioned studies (Stagi et al., 2015; Stagi et al., 2017) also seem to show that, in the long term, PGR in combination with an LGI diet in obese children and adolescents at high risk of developing MetS (i.e., with a family history of obesity and T2DM) induces significantly greater weight loss and a significant increase in insulin sensitivity than diet alone. In obese children and adolescents with established MetS, PGR enhanced the positive effects of MTF on the adiposity parameters and significantly improved glucose and insulin parameters and lipid profiles compared to patients who were either on an MFT plus LGI diet, or an LGI diet alone.
Studies in Adults
The first study in adult subjects was retrieved as an abstract (Belligoli and Vettor 2018) reporting preliminary data from a randomized, double-blind, placebo-controlled clinical trial involving 63 normoglycemic overweight or mildly obese subjects following a balanced normocaloric diet and a regular program of physical activity, who were treated with PGR or placebo for 30 days. In addition to changes in the anthropometric and postprandial lipid profile data after 30 days, the variation in postprandial BG was studied after a single dose of PGR or placebo. In the single-dose assessment, PGR-treated patients exhibited a statistically significant improvement in their postprandial BG profiles without any differences in the fasting parameters (PGR BG at t30-BG at t60 0.45 ± 0.65 vs. Placebo 0.67 ± 0.76 mmol/L, p < 0.05). Due to its publication format as an abstract, several details on the evaluated parameters are lacking. However, the authors stated that after 30 days of intake, PGR was able to significantly reduce LDL cholesterol 300 after a standard meal in comparison with placebo (p < 0.05). Moreover, in PGR-treated patients, fasting and postprandial LDL cholesterol and total cholesterol levels were reduced compared to baseline (data not reported by authors; reported statistical significance as < 0.001).
Finally, the authors also report that both groups showed a significant reduction in body weight as well as waist and hip circumference compared to baseline at this early time point without providing further information (data and p values were not available).
In a recent single-blind, randomized trial, 100 adult patients with MetS and T2DM were randomized to either the PGR or MTF group for 6 months as an add-on therapy to background lifestyle intervention (i.e., an LGI hypocaloric diet) (Guarino et al., 2021). MTF was administered at a daily dose of 1,500–2000 mg. Serum lipids, anthropometric measures, glucose–insulin metabolism changes, and safety/tolerability were evaluated at baseline and at 6 months. A similar significant reduction was observed in both groups in BMI (PGR: T0 35 ± 4 vs. T6 29 ± 3 kg/m2; MTF: T0 36 ± 5 vs. T6 30 ± 3 kg/m2; p < 0.01 for each), waist circumference (PGR: T0 114 ± 10 vs. T6 86 ± 5 cm; MTF T0 115 ± 9 vs. T6 88 ± 5 cm; p < 0.01 for each), visceral fat percentage (PGR: T0 23 ± 6 vs. T6 15 ± 4%; MTF T0 24 ± 6 vs. T6 14 ± 4%; p < 0.01 for each), HbA1c (PGR: T0 60 ± 15 vs. T6 50 ± 14 mmol/mol; MTF: T0 58 ± 14 vs. T6 50 ± 16 mmol/mol; p < 0.05 for each), C-peptide (PGR: T0 1.6 ± 0.5 vs. T6 1.0 ± 1.5 μg/L; MTF T0 1.6 ± 0.5 vs. T6 1.0 ± 1.5 μg/L; p < 0.05 for each), fasting plasma glucose (PGR: T0 197 ± 9.0 vs. T6 117.5 ± 10.3 mg/dl; MTF: T0 2.18 ± 0.21 vs. T6 2.31 ± 0.24 mg/dl; p < 0.01 for each), and HOMA-IR (PGR: T0 4.8 ± 0.8 vs. T6 2.6 ± 0.5; MTF: T0 4.7 ± 0.7 vs. T6 2.5 ± 0.5; p < 0.01 for each), while a significantly more marked decrease in all serum lipid parameters was observed in the PGR group as compared to baseline and to MTF, after treatment (total cholesterol, LDL cholesterol, TGs, T6 vs. T0; p < 0.01 for each in PGR arm vs. p < 0.05 in MTF arm; difference between PGR and MTF arms p < 0.05 in favor of PGR). Results for lipid levels are reported in Figure 3 and Supplementary Figure S3, together with mean absolute values and errors bars. No serious AEs were reported in either group. Mild, non-specific AEs (such as drowsiness, reflux, headache, and dizziness) were reported in both groups in a few cases. However, significantly more gastrointestinal AEs were reported in the MTF group: tympanites (PGR n = 3 vs. MTF n = 19), flatulence (n = 5 vs. n = 21), diarrhea (n = 0 vs. n = 5), and slow digestion (n = 1 vs. n = 7).
According to these studies, in overweight or mildly obese adults, PGR treatment positively affects postprandial BG and reduces cholesterol levels as early as 1 month after treatment. Over a 6-month-treatment period, in obese adults with T2DM and MetS, the positive effects exhibited by PGR on glucose and insulin metabolism were comparable to those offered by MTF and the effects on lipid levels were better.
Preclinical Study
The only animal model study (Greco et al., 2020) evaluated the efficacy of PGR in counteracting weight gain and insulin resistance in a high-fat diet (HFD) mouse model and assessed the mechanisms underlying the favorable metabolic outcomes occurring in vivo after PGR. Two experimental protocols were used. In the first protocol, mice were fed either a regular diet (RD) or an HFD plus PGR or vehicle (placebo) for 2 weeks. In contrast to those on placebo, the PGR-treated HFD-fed mice displayed complete protection against weight gain, meaning that they did not show any weight gain as compared to RD-fed mice, whereas the mean body weight of HFD-control mice increased by 20% between week 4 and week 6 (10 animals per experimental group; p < 0.001). Moreover, since the chosen mouse strain (i.e., C57BL/6) characteristically develops marked glucose intolerance and compromised insulin response as early as within 1 week of an HFD diet, an oral glucose tolerance test (OGTT) was carried out 15 and 30 min after the glucose load, and the PGR-treated HFD mice displayed high BG peaks as experienced by the vehicle-treated HFD mice, but they reverted to baseline at 60 min, thus showing an area under the curve comparable to that of the RD-fed mice (p:ns). The second protocol investigated the ability of PGR to stop or reverse weight gain in overweight mice by feeding the animals an HFD or an RD for 4 weeks and randomizing both groups to receive either PGR or vehicle starting from week 3. During the last 2 weeks of the study, HFD-control mice continued to gain weight (a 6% increase), whereas those receiving PGR lost around 2% of their body weight (10 animals per experimental group; p < 0.01). Also, the OGTT and insulin tolerance test (ITT) improved only in the latter group in which BG values remained below 300 mg/dl at 30 and 60 min after glucose load (five animals per experimental group; p < 0.05 for each time point) and were significantly lower than those of HFD-control mice, at 15 and 60 min after insulin injection (five animals per experimental group; p < 0.05 for each time point). In the same study, to explore the hypothesis that PGR could induce a specific hepatic gene expression signature, the authors investigated the circadian expression of liver genes through RNA-sequencing analysis in mice fed an HFD for 6 weeks and receiving PGR or vehicle in the last 2 weeks. Gene expression and the subsequent functional enrichment analysis showed that PGR is capable of reversing disrupted expression of several lipid metabolism–related transcription factors (TF), such as peroxisome proliferator–activated receptor (PPAR)-gamma and sterol regulatory element–binding protein (SREBP). In particular, PGR treatment downregulated the genes involved in lipid storage and induced the genes involved in insulin sensitivity as reflected by normalization (comparable to the RD-fed mice) of the glycogen and triglyceride content in the livers of the PGR-treated mice (five animals per experimental group; p < 0.05 for each). Furthermore, the HFD-fed mice demonstrated an impaired expression of insulin-like growth factor binding protein-2 (IGFBP-2), a protein essential to insulin action and sensitivity, which was “rescued” after the administration of PGR (p < 0.05).
By analyzing the composition of the experimental animals’ gut microbiota (GM) through 16S rRNA sequencing, the authors also found a shift toward the enrichment of Firmicutes (p < 0.05) and the depletion of Bacteroidetes (p < 0.05) in the HFD mice, a known effect of increased HFD-driven lipid accumulation (Kojima et al., 1999; Matsuda and DeFronzo 1999; Flint et al., 2000). On the other hand, the PGR-treated mice displayed changes in GM composition that could lead to decreased energy harvest from diet.
Finally, to verify whether the effects of PGR depend on the availability of dietary components in the gut, the authors evaluated fecal nutrient excretion.
The feces from each animal were collected the day before the experiment began (Day 0), as well as on the first (Day 1) and seventh (Day 7) day of treatment and analyzed for carbohydrate and lipid levels. The PGR-treated animals displayed a significant increase in fecal excretion of lipids and carbohydrates (five animals per experimental group; p < 0.05 and p < 0.01, respectively) on Day 7 compared to the control HFD mice.
In conclusion, PGR achieved remarkable, beneficial effects on metabolic dysfunctions caused by consumption of an HFD. The mechanisms by which such effects are obtained, despite being due to sequestration, and, therefore, being non-pharmacological events elicited in the intestinal lumen, were revealed to be profound, involving a key insulin-responsive organ, and thus of systemic nature (Greco et al., 2020).
Discussion
The available evidence indicates that PGR is an effective treatment in the clinical management of patients with obesity with or without established MetS, across different age groups. PGR showed beneficial effects and good tolerability also when diabetes was present, in those patients the same degree of control of glucose–insulin metabolism alterations of the standard of care (MTF) was achieved together with significantly higher lipid-lowering effects.
The available clinical data indicate that PGR, despite not having any pharmacological action, still improves MetS abnormalities. This seems to be due to a “systemic” metabolic health-promoting effect that leads to a reduced availability of carbohydrates and lipids both for the intestinal epithelia and the microbiota which positively modulates the impaired “axis” of the intestine and the organs involved in insulin response and energy metabolism and thus counteracts weight gain and insulin resistance as well as improving lipid metabolism. The biological plausibility of this “systemic” effect is supported by the data from the animal model study (Greco et al., 2020). In this study the observed favorable outcomes, including the “rewiring” of hepatic energy metabolism, were attributable to the sequestration of macronutrients by PGR in the intestine and to consequent partial restoration of the HFD-induced increase in the abundance of species belonging to the Firmicutes phylum, which have been shown to promote the absorption of dietary fats in the gut (Turnbaugh et al., 2006; Greco et al., 2020). This is consistent with studies on the relationship between gut microbiota and obesity, which have revealed important changes in the composition and metabolic function of gut microbiota in obese patients, which appear to enable the “obese microbiota” to extract more energy from the diet (Bäckhed et al., 2004; Ley et al., 2005; Turnbaugh et al., 2007; Turnbaugh and Gordon 2009). These studies have demonstrated additional interaction mechanisms with the host, including direct interaction with host epithelial cells, allowing the gut microbiota to control energy expenditure and storage (Turnbaugh et al., 2006; Petraroli et al., 2021). Thus, it is likely that the observed clinical benefit of PGR is attributable to its combined capacity to subtract energetic dietary components from intestinal absorption and, due to a change in substrate availability, to modulate the composition and function of the gut microbiota in a way that influences energy harvest from diet (Figure 5).
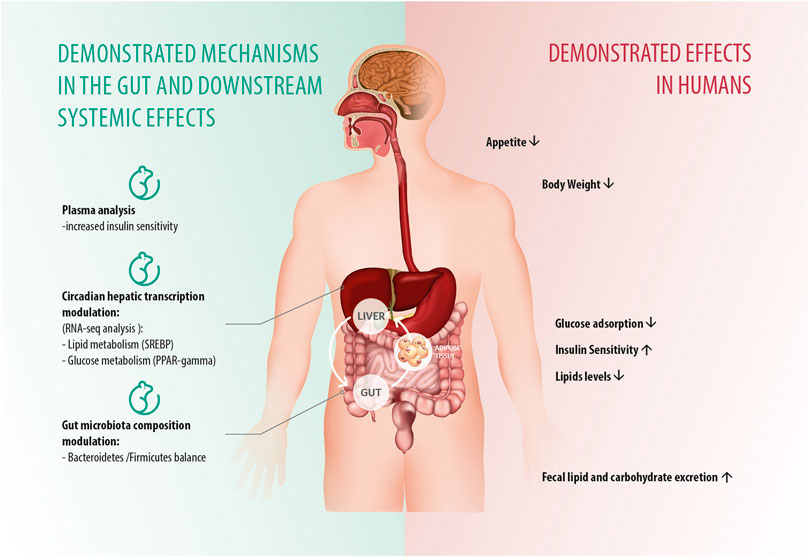
Figure 5. Graphical conceptualization of PGR’s translational mechanisms of action in animal experimental models and their corresponding effects in humans. Across the analyzed clinical studies (four randomized studies and one retrospective study), the addition of PGR to background lifestyle intervention achieved multiple favorable metabolic outcomes both in obese children and adolescents and overweight/obese adults. PGR decreased lipid levels in adults as early as after 30 days of treatment, while the addition of PGR significantly extended and potentiated the positive effects of MTF treatment, allowing for significant further improvement of the adiposity parameters associated with a significant reduction in glucose, insulin, and lipid alterations in obese children and adolescents with established MetS. The biological plausibility of a “systemic” effect of PGR in treated patients is supported by the data from the animal model study in which similar favorable outcomes, including the “rewiring” of hepatic energy metabolism, could be attributable to the sequestration of macronutrients by PGR in the intestine and the consequent changes in gut microbiota composition and function.
This combined capacity is in line with the reported short- and long-term positive treatment outcomes. The “immediate” effect of PGR on glucose bioavailability demonstrated in the animal study was in part confirmed in the human studies on the short-term effects of PGR administration (Belligoli and Vettor 2018) (Fornari et al., 2020) both in adults and children. In the randomized, controlled trial evaluating the single-dose effects of PGR in obese children (Fornari et al., 2020), even if the bioavailability of TGs was significantly reduced in the PGR group as compared to placebo, glucose and insulin increased similarly in both groups. According to the authors, several factors could account for the lack of effect on postprandial glucose and insulin levels, including the mixed meal administered in the study which provided a lower amount of glucose and had a lower glycemic index compared to the glucose load provided in the OGTT performed in the prior studies by Stagi et al. (Stagi et al., 2015; Stagi et al., 2017); the improvement of glucose–insulin metabolism reported by those studies may have been due to the effect of PGR on the anthropometric parameters over the long treatment period. The effects on the metabolic phenotype of PGR treatment observed in animals with established excess weight and glucose–insulin and lipid metabolism abnormalities were confirmed in the long-term studies in obese children and adults with and without T2DM or MetS (Stagi et al., 2015; Stagi et al., 2017). What is particularly interesting is the ability of PGR, unlike MTF, to achieve multiple favorable metabolic outcomes in adult patients with MetS and T2DM (Guarino et al., 2021): a result resembling that obtained with multiple drug treatments. In these patients, PGR displayed a significantly superior lipid-lowering capacity and similar insulin-sensitizing efficacy compared to MTF. It could be speculated that the aforementioned “systemic action” of PGR, is the driver of this beneficial effect.
Currently, MetS affects approximately 20–25% of the world’s adult population in developed and developing countries (Cameron et al., 2004). In recent years, with growing rates of obesity in children and adolescents, MetS is increasingly appearing in the pediatric population. Furthermore, as suggested by the retrospective study by Stagi et al.(Stagi et al., 2017), non-alcoholic fatty liver disease (NAFLD) is probably more common than available studies report, even in patients not fulfilling the current criteria for MetS. For instance, a cross-sectional study evaluating the prevalence of comorbidities in school-age children with obesity found elevated liver transaminase levels in 54% of the patients (Karnebeek et al., 2019).
Even though lifestyle changes may produce significant weight reduction, the long-term efficacy of lifestyle intervention programs on BMI and the related metabolic dysfunctions is questionable, especially in children and adolescents, given the high drop-out rates from diet programs and the frequent representation of obesity in these patients (Marques et al., 2017). For this reason drugs, such as MTF, which can be used in addition to lifestyle interventions have been widely studied in clinical trials, especially in children and adolescents, and are often used in clinical practice (Wiegand et al., 2010; Brufani et al., 2013; Marques et al., 2017).
The high and increasing prevalence of obesity and MetS makes it vital to develop effective and safe therapies. Safety data for PGR are available from two studies. They demonstrate that PGR is well tolerated in children, adolescents and adults, with obesity/MetS or T2DM, also for long periods, with subsequent good adherence (Stagi et al., 2017; Guarino et al., 2021).
At present, clinical experience with PGR is limited due to the low number of studies (5 in total); therefore, more clinical studies are needed to confirm the positive results reported thus far. Other limitations must be considered when interpreting the here reviewed results, such as the quite small sample size. It is possible that other studies exist but were not retrieved because smaller studies tend to be more difficult to find. Selection criteria, especially regarding BMI, were not the same for all studies and the duration of treatment was also markedly varied. There are no direct data regarding the efficacy of PGR compared to drugs specifically indicated for single MetS components (e.g., lipid-lowering drugs or drugs approved for weight loss).
However, the positive metabolic effects of PGR are consistent among the studies, despite their considerable differences in design and patient types. Moreover, the fact that the clinical data included in this review, with one exception, are from interventional studies merits careful consideration, as most patients were investigated within an “ideal setting”, where dietary counseling (and the resulting patient adherence to the prescribed diet) was rigorously pursued. Therefore, it is conceivable that, in a real-life setting, the results attainable with a “diet-only” approach could be more modest, particularly in the long term. Finally, as already highlighted, we must consider that in pediatric and adolescent populations pharmacological approaches should be carefully evaluated because of the possibility of continuous administration for a very long period. In this context, PGR represents a valid and safe option for a non-pharmacological treatment.
The environmental effects of MTF should also be mentioned. Because of its widespread use, MTF enters the environment in large quantities, where it is partly transformed into the active contaminant, guanylurea. Both MTF and guanylurea can be detected in wastewater, plants, influents, effluents and surface waters. They accumulate in edible plants, mussels and fish, where they act as an endocrine disruptor (resulting in higher rates of intersex organisms and a reduction in the reproductive rate) and can contaminate human food (Briones et al., 2016; Elizalde-Velázquez and Gómez-Oliván 2020). This raises further concerns about increasing the use of MTF for common conditions such as obesity or MetS.
In conclusion, PGR appears to be effective in the treatment of obesity and the associated metabolic abnormalities in children or adults, even after the onset of diabetes, thus providing a non-pharmacological treatment option with a favorable risk–benefit profile for the clinical management of major metabolic derangements.
Author Contributions
GG: conceptualization, methodology, validation, and writing–review and editing. MM: formal analysis, investigation, data curation, and writing–original draft preparation. FS, PM, TC, SS, and SG: writing–review and editing, visualization, supervision, and project administration.
Funding
This study received funding from Aboca S.p.A to cover the article processing charge (APC). The sponsor was not involved in the study design, collection, analysis, interpretation of data, writing of this article, or the decision to submit it for publication.
Conflict of Interest
The authors declare that the research was conducted in the absence of any commercial or financial relationships that could be construed as a potential conflict of interest.
Publisher’s Note
All claims expressed in this article are solely those of the authors and do not necessarily represent those of their affiliated organizations, or those of the publisher, the editors, and the reviewers. Any product that may be evaluated in this article, or claim that may be made by its manufacturer, is not guaranteed or endorsed by the publisher.
Supplementary Material
The Supplementary Material for this article can be found online at: https://www.frontiersin.org/articles/10.3389/fdsfr.2022.844256/full#supplementary-material
Reference
Alberti, K. G. M. M., Eckel, R. H., Grundy, S. M., Zimmet, P. Z., Cleeman, J. I., Donato, K. A., et al. (2009). Harmonizing the Metabolic Syndrome. Circulation 120 (16), 1640–1645. doi:10.1161/CIRCULATIONAHA.109.192644
Axon, E., Atkinson, G., Richter, B., Metzendorf, M.-I., Baur, L., Finer, N., et al. (2016). Drug Interventions for the Treatment of Obesity in Children and Adolescents. Cochrane Database Syst. Rev. 11. doi:10.1002/14651858.CD012436
Bäckhed, F., Ding, H., Wang, T., Hooper, L. V., Koh, G. Y., Nagy, A., et al. (2004). The Gut Microbiota as an Environmental Factor that Regulates Fat Storage. Proc. Natl. Acad. Sci. U.S.A. 101 (44), 15718–15723. doi:10.1073/pnas.0407076101
Belligoli, A. S. M., and Vettor, R. (2018). Italian Society of Obesity IX National Congress. Eat. Weight Disord. 23 (5), 705–729. doi:10.1007/s40519-018-0576-0
Bhupathiraju, S. N., and Hu, F. B. (2016). Epidemiology of Obesity and Diabetes and Their Cardiovascular Complications. Circ. Res. 118 (11), 1723–1735. doi:10.1161/CIRCRESAHA.115.306825
Briones, R. M., Sarmah, A. K., and Padhye, L. P. (2016). A Global Perspective on the Use, Occurrence, Fate and Effects of Anti-diabetic Drug Metformin in Natural and Engineered Ecosystems. Environ. Pollut. 219, 1007–1020. doi:10.1016/j.envpol.2016.07.040
Brufani, C., Crinò, A., Fintini, D., Patera, P. I., Cappa, M., and Manco, M. (2013). Systematic Review of Metformin Use in Obese Nondiabetic Children and Adolescents. Horm. Res. Paediatr. 80 (2), 78–85. doi:10.1159/000353760
Cameron, A. J., Shaw, J. E., and Zimmet, P. Z. (2004). The Metabolic Syndrome: Prevalence in Worldwide Populations. Endocrinol. Metabolism Clin. N. Am. 33 (2), 351–375. doi:10.1016/j.ecl.2004.03.005,
Collaborators, G. B. D. O., Afshin, A., Forouzanfar, M. H., Reitsma, M. B., Sur, P., Estep, K., et al. (2017). Health Effects of Overweight and Obesity in 195 Countries over 25 Years. N. Engl. J. Med. 377 (1), 13–27. doi:10.1056/NEJMoa1614362
Davidson, M. B. (2020). Metformin Should Not Be Used to Treat Prediabetes. Diabetes Care 43 (9), 1983–1987. doi:10.2337/dc19-2221
DeBoer, M. D. (2019). Assessing and Managing the Metabolic Syndrome in Children and Adolescents. Nutrients 11, 1788. doi:10.3390/nu11081788
Elizalde-Velázquez, G. A., and Gómez-Oliván, L. M. (2020). Occurrence, Toxic Effects and Removal of Metformin in the Aquatic Environments in the World: Recent Trends and Perspectives. Sci. Total Environ. 702, 134924. doi:10.1016/j.scitotenv.2019.134924
Expert Panel on Detection E (2001). Executive Summary of the Third Report of the National Cholesterol Education Program (NCEP) Expert Panel on Detection, Evaluation, and Treatment of High Blood Cholesterol in Adults (Adult Treatment Panel III). JAMA 285 (19), 2486–2497. doi:10.1001/jama.285.19.2486
Flint, A., Raben, A., Blundell, J., and Astrup, A. (2000). Reproducibility, Power and Validity of Visual Analogue Scales in Assessment of Appetite Sensations in Single Test Meal Studies. Int. J. Obes. 24 (1), 38–48. doi:10.1038/sj.ijo.0801083
Fornari, E., Morandi, A., Piona, C., Tommasi, M., Corradi, M., and Maffeis, C. (2020). Policaptil Gel Retard Intake Reduces Postprandial Triglycerides, Ghrelin and Appetite in Obese Children: A Clinical Trial. Nutrients 12, 214. doi:10.3390/nu12010214
Greco, C. M., Garetto, S., Montellier, E., Liu, Y., Chen, S., Baldi, P., et al. (2020). A Non-pharmacological Therapeutic Approach in the Gut Triggers Distal Metabolic Rewiring Capable of Ameliorating Diet-Induced Dysfunctions Encompassed by Metabolic Syndrome. Sci. Rep. 10 (1), 12915. doi:10.1038/s41598-020-69469-y
Guarino, G., Della Corte, T., Strollo, F., and Gentile, S. (2021). Policaptil Gel Retard in Adult Subjects with the Metabolic Syndrome: Efficacy, Safety, and Tolerability Compared to Metformin. Diabetes. Metab. Syndr. 15 (3), 901–907. doi:10.1016/j.dsx.2021.03.032
James, M., Varghese, T. P., and Sharma, R.ChandAssociation Between Metabolic Syndrome, S., and Mellitus, Diabetes. (2020). Association between Metabolic Syndrome and Diabetes Mellitus According to International Diabetic Federation and National Cholesterol Education Program Adult Treatment Panel III Criteria: a Cross-Sectional Study. J. Diabetes Metab. Disord. 19 (1), 437–443. doi:10.1007/s40200-020-00523-2
Karnebeek, K., Thapar, S., Willeboordse, M., van Schayck, O. C. P., and Vreugdenhil, A. C. E. (2019). Comorbidities in Primary vs Secondary School Children with Obesity and Responsiveness to Lifestyle Intervention. J. Clin. Endocrinol. Metab. 104, 3803–3811. doi:10.1210/jc.2018-02318
Kelishadi, R. (2007). Childhood Overweight, Obesity, and the Metabolic Syndrome in Developing Countries. Epidemiol. Rev. 29, 62–76. doi:10.1093/epirev/mxm003
Knowler, W. C., Barrett-Connor, E., Fowler, S. E., Hamman, R. F., Lachin, J. M., Walker, E. A., et al. (2002). Reduction in the Incidence of Type 2 Diabetes with Lifestyle Intervention or Metformin. N. Engl. J. Med. 346 (6), 393–403. doi:10.1056/NEJMoa012512
Kojima, M., Hosoda, H., Date, Y., Nakazato, M., Matsuo, H., and Kangawa, K. (1999). Ghrelin Is a Growth-Hormone-Releasing Acylated Peptide from Stomach. Nature 402 (6762), 656–660. doi:10.1038/45230
Koskinen, J., Magnussen, C. G., Sinaiko, A., Woo, J., Urbina, E., Jacobs, D. R., et al. (2017). Childhood Age and Associations between Childhood Metabolic Syndrome and Adult Risk for Metabolic Syndrome, Type 2 Diabetes Mellitus and Carotid Intima Media Thickness: The International Childhood Cardiovascular Cohort Consortium. Jaha 6, 6. doi:10.1161/JAHA.117.005632
Ley, R. E., Bäckhed, F., Turnbaugh, P., Lozupone, C. A., Knight, R. D., and Gordon, J. I. (2005). Obesity Alters Gut Microbial Ecology. Proc. Natl. Acad. Sci. U.S.A. 102 (31), 11070–11075. doi:10.1073/pnas.0504978102
Mancia, G., Bombelli, M., Facchetti, R., Casati, A., Ronchi, I., Quarti-Trevano, F., et al. (2010). Impact of Different Definitions of the Metabolic Syndrome on the Prevalence of Organ Damage, Cardiometabolic Risk and Cardiovascular Events. J. Hypertens. 28 (5), 999–1006. doi:10.1097/HJH.0b013e328337a9e3
Mani, U. V., Mani, I., Biswas, M., and Kumar, S. N. (2011). An Open-Label Study on the Effect of Flax Seed Powder (Linum usitatissimum) Supplementation in the Management of Diabetes Mellitus. J. Diet. Suppl. 8 (3), 257–265. doi:10.3109/19390211.2011.593615
Marques, P., Limbert, C., Oliveira, L., Santos, M. I., and Lopes, L. (2017). Metformin Effectiveness and Safety in the Management of Overweight/obese Nondiabetic Children and Adolescents: Metabolic Benefits of the Continuous Exposure to Metformin at 12 and 24 Months. Int. J. Adolesc. Med. Health 29, 29. doi:10.1515/ijamh-2015-0110
Martino, F., Martino, E., Morrone, F., Carnevali, E., Forcone, R., and Niglio, T. (2005). Effect of Dietary Supplementation with Glucomannan on Plasma Total Cholesterol and Low Density Lipoprotein Cholesterol in Hypercholesterolemic Children. Nutr. Metabolism Cardiovasc. Dis. 15 (3), 174–180. doi:10.1016/j.numecd.2004.04.004
Matsuda, M., and DeFronzo, R. A. (1999). Insulin Sensitivity Indices Obtained from Oral Glucose Tolerance Testing: Comparison with the Euglycemic Insulin Clamp. Diabetes Care 22 (9), 1462–1470. doi:10.2337/diacare.22.9.1462
McRorie, J. W., and McKeown, N. M. (2017). Understanding the Physics of Functional Fibers in the Gastrointestinal Tract: An Evidence-Based Approach to Resolving Enduring Misconceptions about Insoluble and Soluble Fiber. J. Acad. Nutr. Dietetics 117 (2), 251–264. doi:10.1016/j.jand.2016.09.021
National Cholesterol Education Program Expert Panel on Detection E (2002). Third Report of the National Cholesterol Education Program (NCEP) Expert Panel on Detection, Evaluation, and Treatment of High Blood Cholesterol in Adults (Adult Treatment Panel III) Final Report. Circulation 106 (25), 3143. doi:10.1161/circ.106.25.3143
Nolan, C. J., Damm, P., and Prentki, M. (2011). Type 2 Diabetes across Generations: from Pathophysiology to Prevention and Management. Lancet 378 (9786), 169–181. doi:10.1016/S0140-6736(11)60614-4
Nsiah, K., Shang, V., Boateng, K., and Mensah, F. (2015). Prevalence of Metabolic Syndrome in Type 2 Diabetes Mellitus Patients. Int. J. App Basic Med. Res. 5 (2), 133–138. doi:10.4103/2229-516X.157170
Petraroli, M., Castellone, E., Patianna, V., and Esposito, S. (2021). Gut Microbiota and Obesity in Adults and Children: The State of the Art. Front. Pediatr. 9, 657020. doi:10.3389/fped.2021.657020
Pushparaj, P. N., Low, H. K., Manikandan, J., Tan, B. K. H., and Tan, C. H. (2007). Anti-diabetic Effects of Cichorium Intybus in Streptozotocin-Induced Diabetic Rats. J. Ethnopharmacol. 111 (2), 430–434. doi:10.1016/j.jep.2006.11.028
Rask Larsen, J., Dima, L., Correll, C. U., and Manu, P. (2018). The Pharmacological Management of Metabolic Syndrome. Expert Rev. Clin. Pharmacol. 11 (4), 397–410. doi:10.1080/17512433.2018.1429910
Shim, D.-W., Han, J.-W., Ji, Y.-E., Shin, W.-Y., Koppula, S., Kim, M.-K., et al. (2016). Cichorium Intybus Linn. Extract Prevents Type 2 Diabetes through Inhibition of NLRP3 Inflammasome Activation. J. Med. Food 19 (3), 310–317. doi:10.1089/jmf.2015.3556
Sinha, S., and Schwartz, R. A. (2007). Juvenile Acanthosis Nigricans. J. Am. Acad. Dermatology 57 (3), 502–508. doi:10.1016/j.jaad.2006.08.016
Stagi, S., Lapi, E., Seminara, S., Pelosi, P., Del Greco, P., Capirchio, L., et al. (2015). Policaptil Gel Retard Significantly Reduces Body Mass Index and Hyperinsulinism and May Decrease the Risk of Type 2 Diabetes Mellitus (T2DM) in Obese Children and Adolescents with Family History of Obesity and T2DM. Ital. J. Pediatr. 41, 10. doi:10.1186/s13052-015-0109-7
Stagi, S., Ricci, F., Bianconi, M., Sammarco, M., Municchi, G., Toni, S., et al. (2017). Retrospective Evaluation of Metformin And/or Metformin Plus a New Polysaccharide Complex in Treating Severe Hyperinsulinism and Insulin Resistance in Obese Children and Adolescents with Metabolic Syndrome. Nutrients 9, 524. doi:10.3390/nu9050524
Turnbaugh, P. J., and Gordon, J. I. (2009). The Core Gut Microbiome, Energy Balance and Obesity. J. Physiol. 587 (Pt 17), 4153–4158. doi:10.1113/jphysiol.2009.174136
Turnbaugh, P. J., Ley, R. E., Hamady, M., Fraser-Liggett, C. M., Knight, R., and Gordon, J. I. (2007). The Human Microbiome Project. Nature 449 (7164), 804–810. doi:10.1038/nature06244
Turnbaugh, P. J., Ley, R. E., Mahowald, M. A., Magrini, V., Mardis, E. R., and Gordon, J. I. (2006). An Obesity-Associated Gut Microbiome with Increased Capacity for Energy Harvest. Nature 444 (7122), 1027–1031. doi:10.1038/nature05414
Wang, L. X., Gurka, M. J., and Deboer, M. D. (2018). Metabolic Syndrome Severity and Lifestyle Factors Among Adolescents. Minerva Pediatr. 70 (5), 467–475. doi:10.23736/S0026-4946.18.05290-8
Weihe, P., and Weihrauch-Blüher, S. (2019). Metabolic Syndrome in Children and Adolescents: Diagnostic Criteria, Therapeutic Options and Perspectives. Curr. Obes. Rep. 8 (4), 472–479. doi:10.1007/s13679-019-00357-x
Wiegand, S., l'Allemand, D., Hübel, H., Krude, H., Bürmann, M., Martus, P., et al. (2010). Metformin and Placebo Therapy Both Improve Weight Management and Fasting Insulin in Obese Insulin-Resistant Adolescents: a Prospective, Placebo-Controlled, Randomized Study. Eur. J. Endocrinol. 163 (4), 585–592. doi:10.1530/EJE-10-0570
Keywords: Policaptil Gel Retard, metabolic syndrome components, obesity, type 2 diabetes mellitus, metabolic syndrome therapy
Citation: Guarino G, Strollo F, Malfertheiner P, Della Corte T, Stagi S, Masarone M and Gentile S (2022) Efficacy and Safety of a Polysaccharide-Based Natural Substance Complex in the Treatment of Obesity and Other Metabolic Syndrome Components: A Systematic Review. Front. Drug Saf. Regul. 2:844256. doi: 10.3389/fdsfr.2022.844256
Received: 04 January 2022; Accepted: 19 19 April 20222022;
Published: 24 May 2022.
Edited by:
Juan L. Tamargo, Complutense University of Madrid, SpainReviewed by:
Bianca Rocca, Catholic University of the Sacred Heart, ItalyRicardo Caballero, Complutense University of Madrid, Spain
Copyright © 2022 Guarino, Strollo, Malfertheiner, Della Corte, Stagi, Masarone and Gentile. This is an open-access article distributed under the terms of the Creative Commons Attribution License (CC BY). The use, distribution or reproduction in other forums is permitted, provided the original author(s) and the copyright owner(s) are credited and that the original publication in this journal is cited, in accordance with accepted academic practice. No use, distribution or reproduction is permitted which does not comply with these terms.
*Correspondence: Mario Masarone, mmasarone@unisa.it