- 1Department for Life Quality Studies, University of Bologna, Rimini, Italy
- 2Instituto de Investigación, Desarrollo e Innovación en Biotecnología Sanitaria de Elche (IDiBE), Universidad Miguel Hernández (UMH), Elche, Spain
- 3Department of Neuroscience, Psychology, Drug Research and Child Health—NEUROFARBA—Section of Pharmacology and Toxicology, University of Florence, Florence, Italy
- 4Department of Medical and Surgical Sciences, University of Bologna, Bologna, Italy
The Medical Device (MD) Regulation EU 2017/745 (MDR) has provided a completely new and more robust regulatory framework at guarantee of the safety and efficacy of therapeutic options accessing the market. At the same time, the MDR poses several challenges for stakeholders, among which, the most significant lying on MDs made of substances (MDMS) whose mechanism of action should be non-pharmacological, immunological, or metabolic.
Moving from single active substances to very complex mixtures, such as the case of natural products, the demonstration of the non-targeted, non-pharmacological mechanism, is even much more challenging since it is very hard to specifically identify and characterize all the interactions each constituent can have within the body.
New scientific paradigms to investigate these multiple interactions and delineate the principal mechanism of action through which the effect is achieved are necessary for the correct regulatory classification and placement in the market of MDMS.
This article will discuss the difficulties in delineating the boundaries between pharmacological and non-pharmacological mechanisms, practical approaches to the study of complex mixtures and the challenges on the application of current experimental paradigms to the study of the mechanism of action of MDMS.
Introduction
The incoming Medical Devices Regulation (MDR) No 2017/745 (Regulation (EU) 2017/745), fully in force since 26 May 2021, has introduced several innovative aspects throughout the life-cycle of a medical device (MD), especially for high-risk MDs, including: 1) mandatory clinical data (introducing, albeit not defining, the concept of sufficient clinical evidence); 2) proactive post-marketing clinical follow-up (PMCF) plan, comprising a Periodic Safety Update Report (PSUR) to be updated at least annually; 3) a new international Unique Device Identification (UDI) system and a publicly accessible European database (EUDAMED) to increase traceability and transparency of MDs (Antich-Isern et al., 2021). This European legal framework mirrors the regulatory scenario of medicinal products, albeit with notable differences (Van Norman, 2016a; Van Norman, 2016b; Van Norman, 2018; Cipriani et al., 2020; Naci et al., 2020) (Table 1).
While promoting a real proactive pre- and post-marketing risk-benefit assessment, the MDR poses a number of challenges for stakeholders, including manufactures, notified bodies and researchers (pharmacologists and toxicologists), and formally recognizes the role of “medical devices made of substances” (MDMS), especially addressed by Rule 14 and 21. The former covers MDs incorporating, as an integral part, a substance which, if used separately, can be considered to be a medicinal product, albeit with an ancillary action (e.g., drug-eluting stents); the latter identifies substance-based MDs introduced via a body orifice such as the gastrointestinal tract or applied to the skin.
Two key issues should be tackled for MDMS. First, the non-targeted mechanism of action “not pharmacological, immunological or metabolic” of the MD is different from the “therapeutic effect” and should be determined (from the literature or experimentally) (Racchi et al., 2016; Racchi and Govoni, 2020), as exemplified by the case of lubiprostone and glycerine as laxatives (Sardi et al., 2018). Second, pharmacokinetic and toxicological data, including biocompatibility, deserve careful assessment, by demonstrating local and systemic exposure to the (ancillary) medicinal substance, its potential impact on safety, interactions with other substances, and relevant disposition of metabolites, taking into account individual variability.
The most challenging aspect lies on MDs made of complex natural products, encompassing food supplements, botanicals, and herbal medicinal products, which do not fit the so-called key-lock model due to their complex multitarget mechanism (Bilia et al., 2021), with notable impact on environmental sustainability. “System biology” has been regarded as a new scientific paradigm to investigate these multiple interactions between complex substances and the human body.
Marking off boundaries between pharmacological and non-pharmacological mechanism of action
The regulatory definitions of pharmacological, immunological, or metabolic means given in Meddev 2.1/3 rev 3 (European Commission, 2009) have been recently amended in the Guidance on borderline between medical devices and medicinal products (MDCG 2022–5) with the aim at adding more precision to such definitions (Supplementary Table S1). Notably, the term “receptor”, historically associated with the pharmacological mean, is no more the focus and the “pharmacological mean” was acknowledged to encompass several molecular interactions (e.g., covalent bonding, H-bonds, electrostatic, and van der Waals forces) with constituents of the human body. It is likely that this new definition will further fuel the discussion but, an “interaction at the molecular level”, is still understood as a specific interaction that should be demonstrated and identified unequivocally. As proposed by Racchi et al. (2016) and by Bilia et al. (2021), when a specific primary target cannot define the mechanism of action, this should not be regarded as a pharmacologic mean. Moreover, the interaction itself is not sufficient to determine the therapeutic effect but should trigger a subsequent signal transduction pathway resulting in “initiation, enhancement, reduction or blockade of physiological functions or pathological processes” (Leone, 2022). Note 5 also reads that “Although not an exhaustive criterion, the presence of a dose-response correlation is indicative of a pharmacological, metabolic or immunological mode of action”.
Moving from single active substances to very complex mixtures, such as the case of natural products, the correct interpretation of “pharmacological mechanism” is even much more challenging since it is very hard to specifically identify, describe and measure all the interactions each constituent can have each other and within the body.
Typically, the claimed therapeutic effect of MDs is achieved by a physicochemical mechanism of action. However, based on Rule 21, the MDR expressly recognizes the existence of MDMS acting systemically other than locally, de facto acknowledging that the concept of non-pharmacological mechanisms of action may go beyond chemico-physical means. Even in such cases, the effect should not be achieved through specific ligand-primary target interactions eliciting definite cell signaling changes.
Rule 21 represents the main novelty of the MDR paving the way for a strong therapeutic innovation while establishing a robust, regulatory framework as guarantee of the efficacy and safety of these products. Notably, MDMS can no longer be classified as class I products and will face a conformity assessment by the Notified Body; in addition, for systemically absorbed products, the scientific opinion of a medicinal competent authority or of the European Medicines Agency (EMA) will be asked and a case-by-case evaluation on whether these product fall within the MDR will be provided.
However, the practical experimental approaches for classifying MDMS under the definition of “non-pharmacological mean” remains a major challenge.
Research methodologies on natural products: From a reductionist approach to the biological complexity of mixtures of substances
Historically, ethnopharmacological research on complex natural products has been aimed at identifying and isolating the bioactive principle to be further developed as a medicinal product once the target and pharmacological mechanism of action was identified. A typical starting point is the measurement of a given biological activity using in vitro phenotypic assays followed by target deconvolution (Terstappen et al., 2007). This approach is particularly valuable for natural products since bioactive molecules can be identified without preconceived molecular mechanisms (Chang and Kwon, 2016).
In the case of complex natural substances, investigations usually begin with a crude extract tested in vitro assays followed by biological activity-guided fractionation until a single active principle or structurally related compounds, are isolated (Najmi et al., 2022). The mode of action of the main active ingredient is then characterized by pharmacological and molecular tools (receptor binding studies, downstream pathways activation/inhibition or enzymatic activity modulation).
Several important guidelines for performing rigorous pharmacological research into natural products were recently published (Izzo et al., 2020): among those, the selection of appropriate concentrations or doses reflecting real-life exposure patterns and the evaluation of the mechanism of action at such thresholds, are particularly valuable also for MDMS research. Moreover, since natural products are often very complex mixtures of different chemical entities that can be prone to geographical, seasonal, and environmental variations, the determination of the chemical composition and its standardization, is another crucial aspect to be addressed (Mattoli et al., 2022). Lack of phytochemical qualitative and quantitative analysis of a particular natural product, may affect the potency, may result in unreproducible effects, whether they are achieved through a pharmacological or a non-pharmacological mechanism of action, and ultimately, may impact its placement in the market and its clinical applications.
When the biological activity of a multicomponent mixture is investigated, the phenotypic change assayed should be observed in the presence of the combinatorial components acting in concert in multiple, but not individually identifiable targets, otherwise, the active principle(s) should be identified, and the mechanism of action further characterized. Synergistic effects can be demonstrated against the phenotypic changes observed with single ingredients: Long et al. (2015) elucidated the predominant combinatory compounds of an herbal extract contributing to an anti-inflammatory effect and demonstrated additive effects by comparing dose-effect curves for single compounds alone and for multiple combinations. However, this strategy assumes that the underling mechanism of action is pharmacological and its relevance and applicability for MDMS is uncertain.
The concept of additive effects and synergisms
Regardless of the mechanism through which the effect is reached, the biological activity as well as the toxicological profile of either drugs combinations and complex mixtures to be developed as MDMS, can be influenced, either positively or negatively, by combinatory effects.
As it is shown in Supplementary Figure S1, the first step is to demonstrate the real subsistence of an interaction resulting in the change of the biological activity, if not, different components, should be analyzed individually. If an interaction is present and it is positive, it leads to an increased biological activity. This increase can be additive or synergic depending on whether the result is the sum or more than the sum of the individual contributions of each component. On the contrary, if negative, the reduction in biological activity can be identified as antagonism, further classifiable as competitive and non-competitive.
The identification and characterization of interactions are always important since in case of positive interactions, a similar final effect can be obtained using lower doses of the different components, saving costs, reducing any putative secondary effect, and minimizing the risk of drug resistance development or MDMS activity reduction. On the other hand, any negative effect can increase toxicity and/or provoke a loss of biological activity.
Although these concepts are classically applicable to drug research, they can also be used for MDMS as they can be constituted by different interactive molecules whose resulting mode of action is chemical-physical rather than pharmacological.
How to design an interaction study?
Designing is probably the most important bottleneck for interaction studies and the most frequent source of mistakes (Herranz-López et al., 2018). Once an interaction has been detected, different doses (for drugs) or different substance combinations and relative abundance (for MDMS) should be combined to fully characterized the nature of the interaction. The most common and useful is the checkerboard or dose-response matrix design, where different mixtures of the different compounds are mixed in different ratios (Figure 1). For reference, it is important to previously know the performance of the individual compounds to avoid both no and over responses (Vlot et al., 2019). This design can be used even for 3 compounds including the third in different plates as described by Herranz-López et al. (2018). The design for interaction tests using MDMS depends on the nature of these devices and normally cannot be developed using plates or similar stuff, but similar procedures, mixing different conditions in a similar way to checkerboard design is the most recommendable approach.
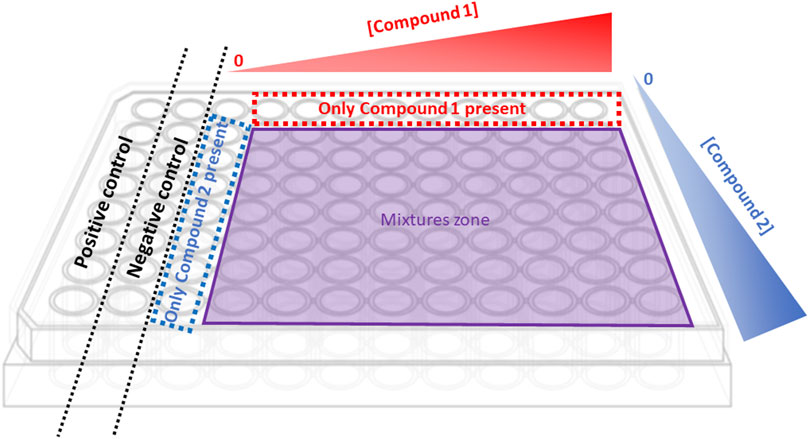
Figure 1. Plate design for interaction testing of two drugs or compounds. Increasing concentrations are mixed in the different wells (purple area) at different ratios and concentrations depending on the well. Data for individual compounds are also obtained from red and blue dotted areas. Positive and negative controls (PC and NC respectively) should be also included. Image adapted from Herranz-López et al. (2018).
Methods for assessing synergy
There are different mathematical approaches to assess synergy, some of them really complex to non-expert users. All these methods have their own limitations and assumptions (Pemovska et al., 2018) and sometimes provide contradictory results, making the initial selection of the method to be arbitrary in most cases (Pemovska et al., 2018; Vlot et al., 2019). Although in a not exhaustive manner, here, some easy-to-use approaches are summarized.
Fractional inhibition combinatory index (FICI) is probably the most used calculus for synergy determination, formerly developed for antimicrobial research, but applicable to any biological activity, even for MDMS. It is based on the minimum inhibitory concentration (MIC) of the individual components and their respective concentrations in the tested mixture (Tomas-Menor et al., 2015) but can be easily adapted by substituting the MIC values with the effective concentration (EC) that is the one leading to a 50% of the effect or whatever other level, if described by the authors. According to this last statement, FICI can be converted to FECI (Fractional Effective Combinatory Index) as it is shown in Eq. 1, where two compounds A and B are tested. In this equation, ECx represents the effective concentration leading to a X% of the biological effect, obtained for both individual compounds and for their mixture.
FECI results can be interpreted in the same way than FICI ones: a synergistic effect will be present when FECI≤ 0.5; an additive effect when 0.5 < FECI≤ 1; an indifferent effect when 1 < FECI<2; and an antagonism when FECI≥ 2 (Tomas-Menor et al., 2015).
Another interesting approach is the isobole method, exceptionally described by Wagner (2011) and updated for more complex analyses by Ezechiáš and Cajthaml (2018). This is a qualitative graphical method providing interesting insights on synergy studies in a more visual way. As FECI, isobole can be obtained for any given specific effect level, i.e., 50% of the maximum effect (Ezechiáš and Cajthaml, 2018). Another tool particularly worthy to mention is the polygonogram (Chou, 2006) that allows to graphically represent the results for interaction studies in a simple but effective way.
Other free, but reliable online tools, fully adaptable to this topic, include Compusyn, developed by Chou (2006), probably the most well-known, Synergy Finder (Ianevsky et al., 2020a), Synergy Finder Plus (Zheng et al., 2022) or Syntoxprofiler, among others (Ianevsky et al., 2020b).
Finally, as in many other disciplines, artificial intelligence is undoubtedly the future for predicting synergistic interactions (Rani et al., 2022) but since appropriate training of the scientific community is required before it could be broadly applied, the evaluation of the exponential number of potential interactions among complex mixtures it is still challenging.
Experimental approaches to clarify the mechanism of action
Once established that the observed phenotypic change is due to the “system of molecules” acting in concert, a further step is to demonstrate that the effect is not achieved by a pharmacological, immunological, or metabolic mechanism of action. Sardi et al. (2018) reported a methodological example of how it could be experimentally proved; the non-pharmacological mechanism of action of glycerine was demonstrated against that of lubiprostone by using two different cellular models, one expressing the specific target of lubiprostone (the ClC-2 receptor-channel) and the other almost lacking the same receptor. Other valuable approaches could be the ones used for target validation in drug discovery such as genetic manipulation of targets by knocking out/in strategies. However, there may be cases such as the use of citicoline in glaucoma, in which the discrimination between pharmacological and non-pharmacological mode of action is hard to demonstrate and may change according to the dose, the route of administration and target selection (Marchesi et al., 2022).
These difficulties exponentially increase in the case of complex mixtures of substances acting via a multi-target, and/or synergistic mode of action. Greco et al. (2020) demonstrated the enhanced water binding and swelling capacity of a natural molecular complex compared to those reachable by the sum of the individual components. The mechanism of action was deemed non-pharmacological since in virtue of its gel forming abilities, the tested MD was capable to physically sequester lipids and carbohydrates from the diet. In vivo, the same MD, improved metabolic parameters by modulating the gut microbiome and, as authors stated, by indirectly modulating, the expression of genes involved in hepatic metabolism.
In virtue of Rule 21, multi-component mixtures acting systemically, pose further challenges. In this regard, like for drugs, omics techniques may be valuable in providing a first overview of the multiple interactions that complex mixtures can have with a biological system in terms of genes, proteins and pathways modulated. However, these indications should be further validated by performing target engagement of the critical pathogenic targets underlying the disease and by verifying the absence of any specific interaction of each constituent at the doses/routes of administration used for the intended purpose. A comparative analysis of omics data with those of a reference drug tested in the same biological system, could be valuable. Guo et al. (2021) proposed that natural products sharing similar pathway fingerprints with approved drugs may have analogous mechanisms of action; by inference, if not, a non-pharmacological mechanism of action could be hypothesized.
The network pharmacology approach
Within system biology, network pharmacology (NP) has emerged as a paradigm shift for tackling drug attrition during drug discovery and pre-clinical development (Hopkins, 2008; Berg, 2014; Gomez-Verjuan et al., 2019). By accounting for and understanding multiple drug-target-disease interactions, multi-component and multi-target agents represent a more effective and less toxic therapeutic approach in complex diseases, as compared to single-target therapy (Hopkins, 2008; Medina-Franco et al., 2013).
In the last decade, NP was especially exploited to elucidate potential targets and toxicity of natural products, including traditional herbal medicines, and detect possible synergism of their constituents (Li et al., 2011; Yuan et al., 2017; Lee et al., 2019). Thus, can NP help deal with the challenge of benefit-risk assessment for SBMD of natural products imposed by rule 21?
With regards to clinical benefit, the non-targeted mechanism of action of MD imposes a careful reflection on the use of NP, which, by definition, usually exploits druggable targets. A network should be designed based on physicochemical and/or physical mechanisms of action that cannot be pinpointed at the single target/receptor level, as exemplified by MDMS used for gastrointestinal disorders (Bilia et al., 2021).
With regards to safety, both medicinal products and MDMS need a full characterization of their potential toxicity. MDMS made by complex mixtures of substances make harder the definition of the toxicological profile, which cannot be considered the sum of the toxicity of single components (Racchi and Govoni, 2020). In this intricate scenario, where the target is considered the function instead of the receptor, the global safety evaluation could be predicted through a network toxicological approach, as applied for traditional Chinese medicine (Zhang et al., 2019). The network approach, also applied to system biology, could take advantage from the so-called “omics-based” technologies (Thomford et al., 2018) to booster innovation and development in the field of MDMS made of natural products.
Conclusions and open questions
Several chronic, multifactorial disorders with unsatisfying medical treatment can benefit from MDMS regulation; this is the case of treatment of some gastrointestinal illness (Corazziari, 2020; Bilia et al., 2021) or metabolic disorders (Guarino et al., 2021), even in the pediatric population (Strisciuglio et al., 2021; Stagi, 2022). In this scenario, MDR provides opportunities for therapeutic innovation with complex substances while guaranteeing patients with standards of efficacy and safety. Another therapeutic opportunity for complex substances, either natural or synthetic, is Rule 21, and its first and second indent referring to MD which need to be absorbed to achieve their intended purpose.
However, the practical approaches and scientific evidence that is required to demonstrate a non-pharmacological mechanism of action are still blurred: current paradigms, including system biology and network pharmacology are applied to the study of the complexity of the biological activities elicited by both drugs and mixtures of natural substances and how they can be applied to the study of the mechanism of action of MD is still uncertain. The difficulties in delineating the boundaries between pharmacological and non-pharmacological mechanism of action and the regulatory classification of even a single substance, such as citicoline in glaucoma (Marchesi et al., 2022), exponentially increase in the case of complex mixtures of substances.
We encourage pharmacologists and toxicologists to take advantage of this journal section as a forum for open discussion of novel proposals on experimental set ups, for reviewing current research and sharing opinions and experiences on both preclinical and clinical research in this area.
Data availability statement
The original contributions presented in the study are included in the article/Supplementary Material, further inquiries can be directed to the corresponding author.
Author contributions
All authors listed have made a substantial, direct, and intellectual contribution to the work and approved it for publication.
Funding
This research was funded by the Spanish Ministry of Economy and Competitiveness (MINECO, RTI 2018-096724-B-C21), the Generalitat Valenciana (PROMETEO/2021/059) and by University of Florence and University of Bologna as part of the institutional working duties.
Conflict of interest
The authors declare that the research was conducted in the absence of any commercial or financial relationships that could be construed as a potential conflict of interest.
Publisher’s note
All claims expressed in this article are solely those of the authors and do not necessarily represent those of their affiliated organizations, or those of the publisher, the editors and the reviewers. Any product that may be evaluated in this article, or claim that may be made by its manufacturer, is not guaranteed or endorsed by the publisher.
Supplementary material
The Supplementary Material for this article can be found online at: https://www.frontiersin.org/articles/10.3389/fdsfr.2022.1001614/full#supplementary-material
References
Antich-Isern, P., Caro-Barri, J., and Aparicio-Blanco, J. (2021). The combination of medical devices and medicinal products revisited from the new European legal framework. Int. J. Pharm. 607, 120992. doi:10.1016/j.ijpharm.2021.120992
Berg, E. L. (2014). Systems biology in drug discovery and development. Drug Discov. Today 19 (2), 113–125. doi:10.1016/j.drudis.2013.10.003
Bilia, A. R., Corazziari, E. S., Govoni, S., Mugelli, A., and Racchi, M. (2021). Medical devices made of substances: Possible innovation and opportunities for complex natural products. Planta Med. 87 (12-13), 1110–1116. doi:10.1055/a-1511-8558
Chang, J., and Kwon, H. J. (2016). Discovery of novel drug targets and their functions using phenotypic screening of natural products. J. Ind. Microbiol. Biotechnol. 43 (2-3), 221–231. doi:10.1007/s10295-015-1681-y
Chou, T. C. (2006). Theoretical basis, experimental design, and computerized simulation of synergism and antagonism in drug combination studies. Pharmacol. Rev. 58 (3), 621–681. doi:10.1124/pr.58.3.10
Cipriani, A., Ioannidis, J. P. A., Rothwell, P. M., Glasziou, P., Li, T., Hernandez, A. F., et al. (2020). Generating comparative evidence on new drugs and devices after approval. Lancet 395 (10228), 998–1010. doi:10.1016/S0140-6736(19)33177-0
Corazziari, E. S. (2020). Medical devices made of substances in the management of patients with gastrointestinal diseases. Pharm. Adv. 01, 27–29. doi:10.36118/pharmadvances.01.2020.08s
European Commission (2009). Medical devices: Guidance document-borderline products, drug-delivery products and medical devices incorporating, ad an integral part, an ancillary medicinal substance or an ancillary human blood derivative. MEDDEV. 2.1/3 rev. 3.
Ezechiáš, M., and Cajthaml, T. (2018). New insight into isobolographic analysis for combinations of a full and partial agonist: Curved isoboles. Toxicology 402-403, 9–16. doi:10.1016/j.tox.2018.04.004
Gomez-Verjan, J. C., Ramírez-Aldana, R., Pérez-Zepeda, M. U., Quiroz-Baez, R., Luna-López, A., Gutierrez Robledo, L. M., et al. (2019). Systems biology and network pharmacology of frailty reveal novel epigenetic targets and mechanisms. Sci. Rep. 9 (1), 10593. doi:10.1038/s41598-019-47087-7
Greco, C. M., Garetto, S., Montellier, E., Liu, Y., Chen, S., Baldi, P., et al. (2020). A non-pharmacological therapeutic approach in the gut triggers distal metabolic rewiring capable of ameliorating diet-induced dysfunctions encompassed by metabolic syndrome. Sci. Rep. 10 (1), 12915. doi:10.1038/s41598-020-69469-y
Guarino, G., Della Corte, T., Strollo, F., and Gentile, S.Nefrocenter Research Study Group (2021). Policaptil Gel Retard in adult subjects with the metabolic syndrome: Efficacy, safety, and tolerability compared to metformin. Diabetes Metab. Syndr. 15 (3), 901–907. doi:10.1016/j.dsx.2021.03.032
Guo, F., Jiang, C., Xi, Y., Wang, D., Zhang, Y., Xie, N., et al. (2021). Investigation of pharmacological mechanism of natural product using pathway fingerprints similarity based on "drug-target-pathway" heterogenous network. J. Cheminform. 13 (1), 68. doi:10.1186/s13321-021-00549-5
Herranz-López, M., Losada-Echeberría, M., and Barrajón-Catalán, E. (2018). The multitarget activity of natural extracts on cancer: Synergy and xenohormesis. Med. (Basel) 6 (1), 6. doi:10.3390/medicines6010006
Hopkins, A. L. (2008). Network pharmacology: The next paradigm in drug discovery. Nat. Chem. Biol. 4 (11), 682–690. doi:10.1038/nchembio.118
Ianevski, A., Giri, A. K., and Aittokallio, T. (2020a). SynergyFinder 2.0: Visual analytics of multi-drug combination synergies. Nucleic Acids Res. 48 (W1), W488–W493. doi:10.1093/nar/gkaa216
Ianevski, A., Timonen, S., Kononov, A., Aittokallio, T., and Giri, A. K. (2020b). SynToxProfiler: An interactive analysis of drug combination synergy, toxicity and efficacy. PLoS Comput. Biol. 16 (2), e1007604. doi:10.1371/journal.pcbi.1007604
Izzo, A. A., Teixeira, M., Alexander, S. P. H., Cirino, G., Docherty, J. R., George, C. H., et al. (2020). A practical guide for transparent reporting of research on natural products in the British Journal of Pharmacology: Reproducibility of natural product research. Br. J. Pharmacol. 177 (10), 2169–2178. doi:10.1111/bph.15054
Lee, W. Y., Lee, C. Y., Kim, Y. S., and Kim, C. E. (2019). The methodological trends of traditional herbal medicine employing network pharmacology. Biomolecules 9 (8), 362. doi:10.3390/biom9080362
Leone, M. G. (2022). Medical devices made of substances: A new challenge. Front. Drug Saf. Regul. 2, 952013. doi:10.3389/fdsfr.2022.952013
Li, S., Zhang, B., and Zhang, N. (2011). Network target for screening synergistic drug combinations with application to traditional Chinese medicine. BMC Syst. Biol. 5 (1), S10. doi:10.1186/1752-0509-5-S1-S10
Long, F., Yang, H., Xu, Y., Hao, H., and Li, P. (2015). A strategy for the identification of combinatorial bioactive compounds contributing to the holistic effect of herbal medicines. Sci. Rep. 5, 12361. doi:10.1038/srep12361
Marchesi, N., Fahmideh, F., Barbieri, A., Racchi, M., Pascale, A., and Govoni, S. (2022). Pharmacological versus non-pharmacological and ancillary mechanisms in eye drops used in the treatment of glaucoma. Front. Drug Saf. Regul. 2, 933471. doi:10.3389/fdsfr.2022.933471
Mattoli, L., Gianni, M., and Burico, M. (2022). Mass spectrometry based metabolomic analysis as a tool for quality control of natural complex products. Mass Spectrom. Rev., e21773. doi:10.1002/mas.21773
Medina-Franco, J. L., Giulianotti, M. A., Welmaker, G. S., and Houghten, R. A. (2013). Shifting from the single to the multitarget paradigm in drug discovery. Drug Discov. Today 18 (9-10), 495–501. doi:10.1016/j.drudis.2013.01.008
Naci, H., Salcher-Konrad, M., Kesselheim, A. S., Wieseler, B., Rochaix, L., Redberg, R. F., et al. (2020). Generating comparative evidence on new drugs and devices before approval. Lancet 395 (10228), 986–997. doi:10.1016/S0140-6736(19)33178-2
Najmi, A., Javed, S. A., Al Bratty, M., and Alhazmi, H. A. (2022). Modern approaches in the discovery and development of plant-based natural products and their analogues as potential therapeutic agents. Molecules 27 (2), 349. doi:10.3390/molecules27020349
Pemovska, T., Bigenzahn, J. W., and Superti-Furga, G. (2018). Recent advances in combinatorial drug screening and synergy scoring. Curr. Opin. Pharmacol. 42, 102–110. doi:10.1016/j.coph.2018.07.008
Racchi, M., Govoni, S., Lucchelli, A., Capone, L., and Giovagnoni, E. (2016). Insights into the definition of terms in European medical device regulation. Expert Rev. Med. Devices 13 (10), 907–917. doi:10.1080/17434440.2016.1224644
Racchi, M., and Govoni, S. (2020). The concept of non-pharmacological mechanism of action in medical devices made of substances in practice: What pharmacology can do to promote the scientific implementation of the European medical device regulation. Pharm. Adv. 01, 4–12. doi:10.36118/pharmadvances.01.2020.02s
Rani, P., Dutta, K., and Kumar, V. (2022). Artificial intelligence techniques for prediction of drug synergy in malignant diseases: Past, present, and future. Comput. Biol. Med. 144, 105334. doi:10.1016/j.compbiomed.2022.105334
REGULATION (EU) (2017). 2017/745 of the EUROPEAN parliament and of the council of 5 april 2017 on medical devices, amending directive 2001/83/EC, regulation (EC) No 178/2002 and regulation (EC) No 1223/2009 and repealing council directives 90/385/EEC and 93/42/EEC. Available at: https://eur-lex.europa.eu/legal-content/EN/TXT/PDF/?uri=CELEX:32017R0745.
Sardi, C., Garetto, S., Capone, L., Galbiati, V., Racchi, M., Govoni, S., et al. (2018). Experimental paradigm for the assessment of the non-pharmacological mechanism of action in medical device classification: The example of glycerine as laxative. Front. Pharmacol. 7 (9), 1410. doi:10.3389/fphar.2018.01410
Stagi, S. (2022). Medical devices made of substances: The need for a change in approach in paediatrics. Front. Drug Saf. Regul. 2, 867143. doi:10.3389/fdsfr.2022.867143
Strisciuglio, C., Coppola, V., Russo, M., Tolone, C., Marseglia, G. L., Verrotti, A., et al. (2021). Promelaxin microenemas are non-inferior to oral polyethylene glycol for the treatment of functional constipation in young children: A randomized clinical trial. Front. Pediatr. 29 (9), 753938. doi:10.3389/fped.2021.753938
Terstappen, G. C., Schlüpen, C., Raggiaschi, R., and Gaviraghi, G. (2007). Target deconvolution strategies in drug discovery. Nat. Rev. Drug Discov. 6 (11), 891–903. doi:10.1038/nrd2410
Thomford, N. E., Senthebane, D. A., Rowe, A., Munro, D., Seele, P., Maroyi, A., et al. (2018). Natural products for drug discovery in the 21st century: Innovations for novel drug discovery. Int. J. Mol. Sci. 19 (6), 1578. doi:10.3390/ijms19061578
Tomás-Menor, L., Barrajón-Catalán, E., Segura-Carretero, A., Martí, N., Saura, D., Menéndez, J. A., et al. (2015). The promiscuous and synergic molecular interaction of polyphenols in bactericidal activity: An opportunity to improve the performance of antibiotics? Phytother. Res. 29 (3), 466–473. doi:10.1002/ptr.5296
Van Norman, G. A. (2016a). Drugs and devices: Comparison of European and U.S. Approval processes. JACC. Basic Transl. Sci. 1 (5), 399–412. doi:10.1016/j.jacbts.2016.06.003
Van Norman, G. A. (2016b). Drugs, devices, and the FDA: Part 2: An overview of approval processes: FDA approval of medical devices. JACC. Basic Transl. Sci. 1 (4), 277–287. doi:10.1016/j.jacbts.2016.03.009
Van Norman, G. A. (2018). Expanded patient Access to investigational new devices: Review of emergency and nonemergency expanded use, custom, and 3D-printed devices. JACC. Basic Transl. Sci. 3 (4), 533–544. doi:10.1016/j.jacbts.2018.06.006
Vlot, A. H. C., Aniceto, N., Menden, M. P., Ulrich-Merzenich, G., and Bender, A. (2019). Applying synergy metrics to combination screening data: Agreements, disagreements and pitfalls. Drug Discov. Today 24 (12), 2286–2298. doi:10.1016/j.drudis.2019.09.002
Wagner, H. (2011). Synergy research: Approaching a new generation of phytopharmaceuticals. Fitoterapia 82 (1), 34–37. doi:10.1016/j.fitote.2010.11.016
Yuan, H., Ma, Q., Cui, H., Liu, G., Zhao, X., Li, W., et al. (2017). How can synergism of traditional medicines benefit from network pharmacology? Molecules 22 (7), 1135. doi:10.3390/molecules22071135
Zhang, R., Zhu, X., Bai, H., and Ning, K. (2019). Network pharmacology databases for traditional Chinese medicine: Review and assessment. Front. Pharmacol. 10, 123. doi:10.3389/fphar.2019.00123
Keywords: medical devices made of substances, substance based medical device, regulation 2017/745/EC, natural products, pharmacological mechanism, network pharmacology, system biology, synergy
Citation: Fimognari C, Barrajón-Catalán E, Luceri C, Turrini E, Raschi E and Bigagli E (2022) New regulation on medical devices made of substances: Opportunities and challenges for pharmacological and toxicological research. Front. Drug Saf. Regul. 2:1001614. doi: 10.3389/fdsfr.2022.1001614
Received: 23 July 2022; Accepted: 31 August 2022;
Published: 16 September 2022.
Edited by:
Juan L Tamargo, Complutense University of Madrid, SpainReviewed by:
Silvia Di Giacomo, Sapienza University of Rome, ItalyCopyright © 2022 Fimognari, Barrajón-Catalán, Luceri, Turrini, Raschi and Bigagli. This is an open-access article distributed under the terms of the Creative Commons Attribution License (CC BY). The use, distribution or reproduction in other forums is permitted, provided the original author(s) and the copyright owner(s) are credited and that the original publication in this journal is cited, in accordance with accepted academic practice. No use, distribution or reproduction is permitted which does not comply with these terms.
*Correspondence: Elisabetta Bigagli, ZWxpc2FiZXR0YS5iaWdhZ2xpQHVuaWZpLml0
†These authors have contributed equally to this work