- 1ICMR-National Institute of Malaria Research, New Delhi, India
- 2Academic of Scientific and Innovative Research (AcSIR), Ghaziabad, India
- 3CSIR-National Institute of Oceanography, Dona Paula, Goa, India
Malaria poses several challenges to the global research community on both diagnostic and therapeutic fronts. Most prominent of them are deletion of target genes (pfhrp2/3) used in rapid diagnostic tests (RDTs) and the emergence of resistance against frontline antimalarials by the evolving parasite. Exploration of novel therapeutics for malaria in view of limited vaccine options is a promising resort for malaria control and elimination. The scope of marine-derived chemotherapeutics is exciting, with a significant number of FDA-approved drugs or therapeutic leads under clinical trials for other diseases. This review article discusses the significant antimalarial potential of marine-derived natural products extracted from diverse biota including sponges, bacteria, sea hare and algae etc. Bioassay-guided fractionation of raw extracts from marine organisms for lead identification and further structural characterization of purified compounds compose a sustainable marine-derived drug discovery pipeline; which can be particularly diverted towards the exploration of antimalarials. It is to be noted that the Indian peninsula is largely unexplored, particularly for antimalarials screening; which has a huge marine biodiversity owing to the three distinct water bodies- Bay of Bengal, Indian Ocean and Arabian sea. This review also envisions a collaborative initiative to explore the potential of marine natural products in an economically feasible manner.
Introduction
As the world is recovering from the pandemic- COVID-19, the concern over malaria has regained its focus; globally, the estimated malaria cases have gone up from 225 million in 2019 to 241 million in 2020 (WHO, 2021), with most of the burden coming form the African region. Amongst the biological threats to global malaria elimination programs-deletion of the pfhrp2/3 genes; parasite resistance to antimalarials and vector resistance to insecticides are the major threats identified by WHO; thus, the onus now is on the research community in mitigating these threats. Except for the first WHO-approved malaria vaccine (RTS, S/AS01) in 2021 with its limited efficacy, antimalarial agents are the only resort for therapeutic management of malaria. The majority of the malaria burden is caused by two Plamosdium species- P. falciparum (∼193 million cases) and P. vivax (∼14 million cases), globally (Battle and Baird, 2021). Numerous events of antimalarial failures have been documented in the past; recent one is the development of resistance against Artemisinin combination therapies (ACTs)- the first line of defence against P. falciparum malaria (Nass and Efferth, 2019). Since the discovery of artemisinin and its combinatorial partner drugs, very few antimalarial drugs have reached advanced clinical trials. It is worth noting that a collection of potent antimalarial compounds called Malaria box [Medicines for Malaria Venture (MMV), Switzerland], is the only commercially available resource for exploration of novel therapeutic targets against the malaria parasite (Subramanian et al., 2018). Therefore, in light of the evidences of emerging resistance against the currently available antimalarials and limited therapeutic leads, it becomes imperative to explore novel antimalarial compounds from the vast diversity offered by nature - creating a sustainable malaria drug discovery pipeline.
Amongst the notable nature-derived antimalarials- Artemisinin is an immensly valuable nature-derived antimalarial and its discovery by Dr. Tu Youyou is worth mentioning. Amongst 617 documented recipes from Chinese traditional medicine, Dr. Youyou identified the cold extracts of Artemis annua to be effective against malaria, which has become the first line of defense against P. falciparum malaria till date. The chemical space offered by the nature-derived extracts sourced from plants, marine or fungi is vastly diverse, yielding greater hits/leads compared to synthetic compound libraries (Harvey et al., 2015). Inherently, natural extracts are complex bioactive molecules, often containing a mixture of different functional groups acting synergistically. Moreover, compared to synthetic compounds, the bioactive fractions from natural extracts are also better targeted due to metabolite-likeness (Harvey et al., 2015). These bioactive fractions are usually extracted by preparative scale high-pressure liquid chromatography (HPLC) and characterized through techniques like nuclear magnetic resonance (NMR) and liquid chromatography-mass spectrometry (LC-MS) (Koehn, 2008). Attributing to the world’s largest microbial collection and natural products library- MEDINA is a wonderful resource, that has generated three crucial anti-malarial leads (Pérez-Moreno et al., 2016).
Observant of the immense potential of nature-derived antimalarials, this review article discusses the potential of marine-derived antimalarials acting on multiple stages of the parasite. With the discovery of novel marine-derived antimalarials and their structural characterization offering new scaffolds, that may lead to development of new generation synthetic antimalarials. It is also worth discussing that the Indian peninsula surrounded by three distinct water bodies offers a great range of marine biodiversity to explore.
The potential of therapeutics from marine biodiversity
According to the World Register of Marine Species (WoRMS), ∼228,450 species have been identified in the oceans (World Register of Marine Species), and the exploration of bioactive compounds from these marine organisms is negligible (Ahyong et al., 2022). The marine environment harbors a wide range of structurally and physiologically different marine organisms/microorganisms contributing to the rich biodiversity-such as marine phytoplankton, bacteria, fungi and algae (brown/red/green algae), marine invertebrates (sponges, cnidarians, tunicates) and their associated microorganisms. The chemical-mediated interactions among the organisms or microbes via primary/secondary metabolites constitute the chemical ecology of a marine ecosystem. The primary metabolites serve functions such as growth, development and reproduction, while secondary metabolites act as info chemicals (messenger molecules) or allelochemicals (toxic compounds) for communication and defense purposes (Thakur and Müller, 2004; Pallela et al., 2016; Singh and Thakur, 2016). Unlike eukaryotes, microbes respond quickly to environmental stimuli (temperature, salinity and pressure) via structurally and functionally unique metabolites aiding in their survival and often found to have significant therapeutic potential. Specifically, marine organisms produce a range of structurally novel bioactive compounds offering therapeutic potential in different diseases including cancer, inflammatory diseases, acquired immune deficiency syndrome (AIDS) etc. (Xie et al., 2018; Ameen et al., 2021). Microorganisms are crucial part of the marine biodiversity usually inhabiting the surface of marine sponges, corals, tunicates and other invertebrates, often constituting up to 60% of their body mass (Mehbub et al., 2014). Notably, during 2007–2017, a total of 267 natural compounds were isolated from rare marine actinomycetes strains (Subramani and Sipkema, 2019) and 774 novel compounds were obtained from sponge-derived microorganisms during 1998–2017 (Cheng et al., 2020). In terms of the origin of compounds, the majority of the compounds were derived from fungi (73%), followed by actinomycetes (16%) and other bacteria (11%) (Cheng et al., 2020). Databases like Seaweed Metabolite Database (SWMD) (Davis and Vasanthi, 2011; Kildgaard et al., 2017; Barbosa and Roque, 2019) and MarinLit (Royal Society of Chemistry, 2022) are a good resource to refer to marine natural products and avoiding the dereplication of already isolated/characterized compounds. Table 1 highlights some of the FDA-approved and commercialy available drugs sourced from marine organisms, emphasizing their immense potential in drug discovery. Table 2 enlists a few marine-sourced antimalarials, which are undergoing pre-clinical trials.
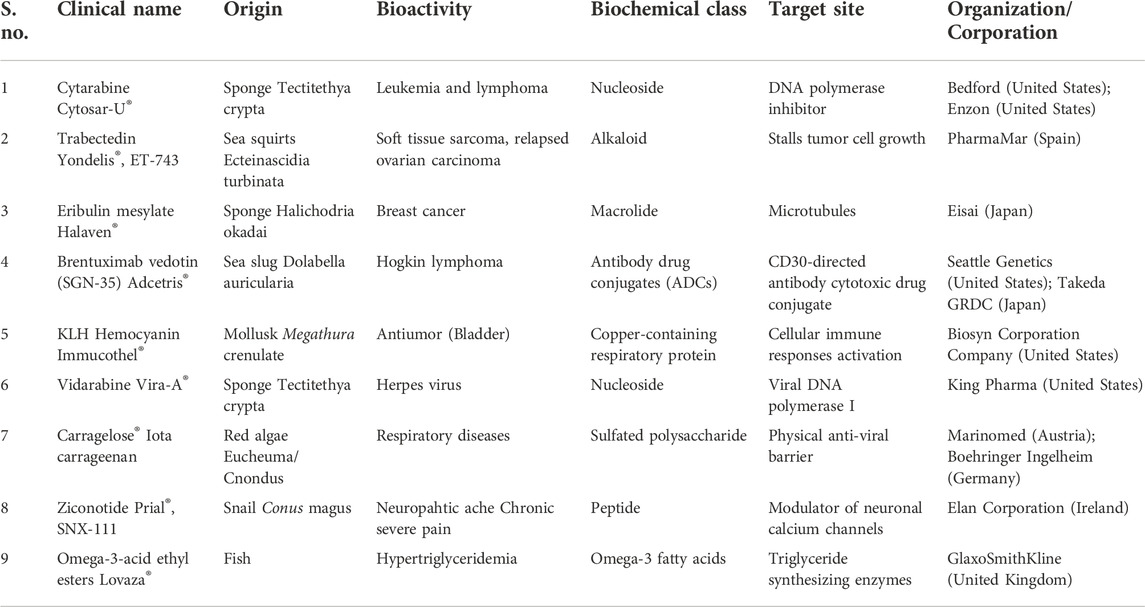
TABLE 1. List of clinically approved drugs sourced from marine bodies [adapted from (Ghareeb et al., 2020)].

TABLE 2. List of marine natural products in pre-clinical trials for malaria [adapted from (Malve, 2016)].
Majority of the marine natural products can be classified as-sterols, terpenoids, alkaloids, peptides, polysaccharides, acetogenins, polyketoids, polyacetylenes, shikimates and toxins (Blunt et al., 2016). Terpenoids consist of repeated units of isoprene (C5H8), imparting significant bioactivities (Bakkali et al., 2008; Ebada and Proksch, 2012; Varijakzhan et al., 2021). Many alkaloids isolated from marine sponges have been reported to possess antimalarial activity (Ang et al., 2000). Peptides from marine sources can be found in both cyclic or linear conformation consisting of rare amino acids and are often considered inexpensive and safe (Nakao et al., 1995; Yeung et al., 1996) (Wang et al., 2017). Sterols are usually isolated from marine invertebrates and are diversely bioactive (Ioannou et al., 2009). However, a comprehensive description of these classes of marine natural products is beyond the scope of this review. Interestingly, compounds from the marine invertebrates (ascidians) offer unique bioactivities including antimalarial activity (6%), otherwise dominated by anti-cancerous (64%) and antimicrobial activities (14%) (Palanisamy et al., 2017).
A sustainable marine-derived drug discovery pipeline for malaria shall include collection and identification of marine organisms/associated microorganisms from different oceans; screening of crude extracts/partial fractions through a series of biochemical assays in vitro followed by in vivo testing; structural characterization; scaling up of selected molecules/hits or chemical synthesis to accomplish pharmacokinetics and pharmacodynamics (PKPD) studies (Hughes et al., 2011). The molecules with safer PKPD profiles can undergo clinical trials and further be validated as drugs available for commercial use. A schematic for the development of marine-derived antimalarial drugs is shown in Figure 1.
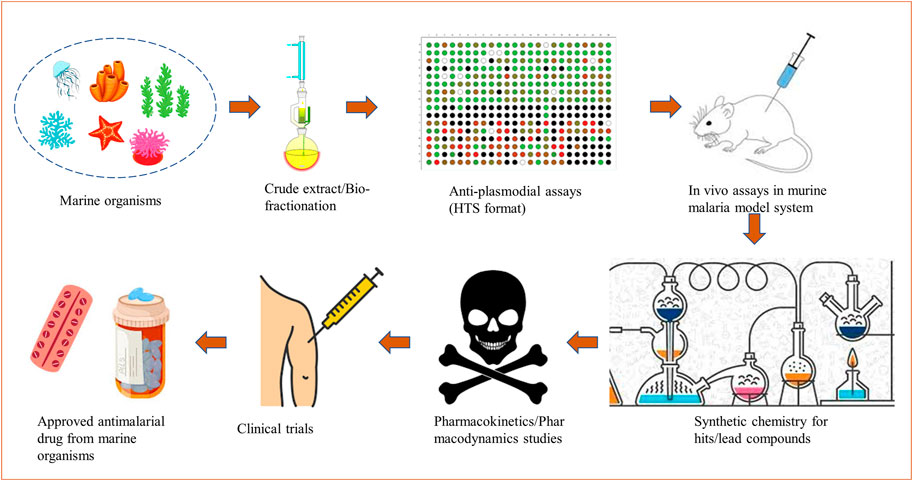
FIGURE 1. A schematic depicting the development of marine-derived antimalarial drugs from extraction to a market available drug.
High throughput screening assays for antimalarial screening
HTS makes use of optimized/validated assays to conduct mass screening with minimal resources and generate a multitude of experimental data, edgeing over the traditional drug screening assays that usually were laborious and time-intensive, with a limited set of experimental data. HTS assays are categorized as target-specific assays (biochemical) and phenotypic assays (whole-cell based). Specifically, phenotypic assays are employed for unknown targets and are based on reporters/viability/receptor/ion channel assays. For known targets, binding/enzyme/receptor assays are usually performed to accomplish target-specific screening. A case in point, the current pandemic SARS-CoV-2, where HTS assays have been thoroughly employed in screening of therapeutic hits against the viral proteases (papain-like proteases) (Shen et al., 2019; Smith et al., 2020; Zhao et al., 2021). The lack of nucleus in the human RBC makes visualization of the Plasmodium parasite feasible in HTS assays. Some of the commonly used anti-malarial HTS assays are- 1) Detection of fluorescence generated by the DNA binding dye (Joanny et al., 2012) (SYBR Green-I); 2) Lactate dehydrogenase (LDH) (Makler et al., 1993; Druilhe et al., 2001; Nambati et al., 2018) assay, where the parasite specifically utilize 3-acetylpyridine adenine dinucleotide (APAD) and its conversion into reduced APADH is followed; 3) Histidine-rich Protein-II (HRP2) based drug susceptibility assays which are sensitive and suitable for screening purposes (Noedl et al., 2002) and 4) Transgenic parasite lines expressing luciferase reporter cassettes (Cui et al., 2008; Hasenkamp et al., 2013; Swann et al., 2016; Caridha et al., 2019). Specific assays that measure the parasitemia in a high-throughput manner are PicoGreen (Corbett et al., 2004), YOYO-1 (Li et al., 2007), (3H)-Hypoxanthine based assays (Desjardins et al., 1979), etc. Owing to the sensitivity and ease of performance, SYBR Green-I based assays are routinely used to detect low levels of parasitemia (Rason et al., 2008; Cheruiyot et al., 2016) including mixed-strain infections (Dennull et al., 2009). HTS assays have also been employed to screen compounds against the sexual stages of the parasite (Buchholz et al., 2011). Of note are the inexpensive HTS assays designed to screen 10,000 compounds against stage IV and V gametocytes (Lucantoni et al., 2016). 64 hits for gametocyte stages I-III (IC50 < 2.5 µM) were identified from 400 MMV compounds, using a transgenic P. falciparum line expressing the GFP-luciferase (gametocyte stage I-III) (Lucantoni et al., 2013). Moreover, 681 hits were received from a library of 500,000 compounds against the liver stages of the P. falciparum with IC50 < 1 µM; further, phenotypic and functional assays reported 58 compounds to be mitochondrial inhibitors (Antonova-Koch et al., 2018). RNA-binding dyes for P. falciparum RNA had also been demonstrated their use in the high-content live cell imaging to observe cell growth and proliferation in HTS format (Cervantes et al., 2009). State-of-the-art techniques for conducting HTS assay includes semi-automation and high-content live cell imaging to investigate the cellular physiology in a real-time manner. These prominent studies emphasize the use of the HTS format in screening antimalarials from marine-derived compounds, saving expensive reagents, time and labour of the research personnel.
Global advancements in marine-derived antimalarials
This section discusses the notable marine-derived natural products with significant antimalarial potential. Table 3 collates a list of marine-derived antimalarial compounds, their bioactivities, active functional groups and respective chemical structures.
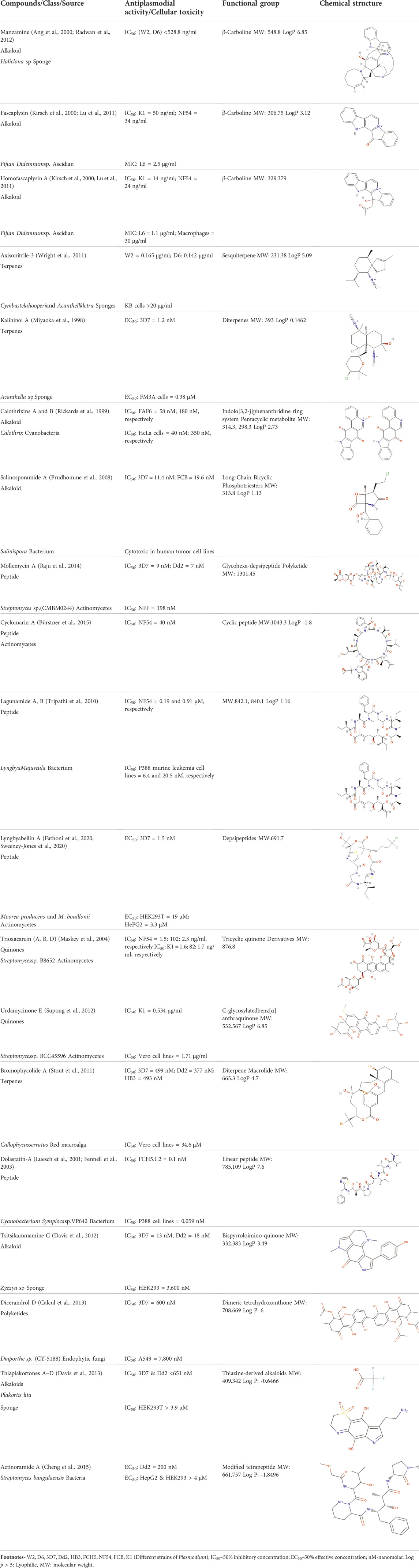
TABLE 3. A list of potent compounds isolated from marine organisms/microbes with bioactivity against malaria parasites.
Marine-derived antimalarials from sponges
1. Manzamine A is a marine-derived β-carboline alkaloid from sponges found in Indian and Pacific oceans (Haliclona sp., Pellina, Pachypellina, Xestospongia, Ircina and Amphimedon). With significant antimalarial activity in addition to the anti-inflammatory and antimicrobial activities (Ang et al., 2000; Radwan et al., 2012). The antimalarial activity of Manzamine A and its derivative (−)-8-hydroxymanzamine A were screened in the culture of P. berghei, the rodent parasite. Morphological changes and subsequent degeneration were noticed by transmission electron microscopy in the P. berghei asexual stage parasites, in a similar fashion to the chloroquine treatment. In vivo studies of mice malaria with chloroquine and mefloquine-resistant P. berghei strains found the IC50 concentrations of manzamine A to be <529 ng/ml (El Sayed, 1996). It was interesting that manzamine F carrying a ketonic group at C-31, hydroxyl group at C-8 and double bond between C-32 and C-33 and hydrogen at N-27 did not show any antimalarial activity compared to manzamine A.
2. Fascaplysin and homofascaplysin A derived from sponge Hyrtios cf. erecta from Fiji have been known to possess antimalarial activities along with other biological activities in Trypanosoma brucei, Trypanosoma cruzi, hepatitis A virus and against molecular targets- HIV-1-RT and p56lck tyrosine kinase (Kirsch et al., 2000). 3-bromohomofascaplysin A, an analogue derived from Fijian Didemnum sp. ascidan has also been reported (Lu et al., 2011). In vitro stage-specific inhibition assays using chloroquine and mefloquine-resistant P. falciparum by homofascaplysin A demonstrated significant IC50 values - 0.55 nM and 105 nM against ring-stage parasites and all live parasites, respectively. The minimum inhibitory concentrations (MIC) of fascaplysin and homofascaplysin A in L6 and macrophages were observed to be 1.1 μg/ml and 2.5 μg/ml, indicating their selective action against the malaria parasites.
3. Marine sponge, Cymbastela hooperi from Great barrier reef, Australia have been observed to be a rich source of biologically active molecules-a series of diterpenes and isonitrile-containing diterpenes. These compounds demonstrated potent antimalarial activities against P. falciparum strains D6 and W2. Isonitriles (1–5) derived from C. hooperi and axisonitrile from Acanthella kletra are notable with their potent antimalarial activities, while tolerable cytoxicity was observed in KB cells. (Wright et al., 2011). Using molecular modeling, diisocyanoadociane and axisonitrile-3 interact with the heme of the hemoglobin via the formation of a coordination complex. Interestingly, the isonitrile moiety forms a coordinate bond with the heme iron, thus inhibits the hemozoin formation-a significant mode of action for developing antimalarials and have been used in synthetic approaches to design novel scaffolds.
4. Kalihinane diterpenoids derived from marine sponges have been shown to possess antimicrobial, antifungal, cytotoxic, antihelminthic, antifouling activities. Specifically, okinawan marine sponge, Acanthella sp. extracts containing isonitrile/isothionitrile kalihinane diterpenoids-kalihinol A, kalihiene, 6-hydroxykalihinene demonstrated significant antimalarial activities in nanomolar range, comparable/better than mefloquine (Miyaoka et al., 1998).
Marine-derived antimalarials from bacteria
1. Cyanobacteria have been well known to produce bioactive metabolites; two Calothrix extracts were identified for their bioactivity against P. falciparum in a dose-dependent manner (Rickards et al., 1999). Further, bioassay-guided fractionation of these extracts led to identification of two pure compounds calothrixins A and B and their structural characterization was accomplished. The atypical indolo[3,2-j]phenanthridine ring system in these pentacyclic metabolites was observed as the active chemical moiety exerting the antiplasmodial activity. IC50 values of calothrixins A and B (58 and 180 nM, respectively) were found to be comparable to chloroquine.
2. Marine actinomycetes have been recognized as a rich source of secondary metabolites with anticancer potential. Extracts from ocean sediment-derived microbes were screened and a pure compound-salinosporamide A, was isolated from Salinispora tropica (marine actinomycete), which demonstrated antimalarial activity against P. falciparum (Prudhomme et al., 2008). In vitro antiplasmodial activity with IC50 11.4 nM of salinosporamide A were reported, which was comparable to the established antimalarial drugs-chloroquine, mefloquine and artemisinin. Similar to its mode of action against human multiple myeloma the inhibition of ubiquitination mediated proteasome degradation (20 S proteasome) was shown to be the plausible inhibitory property of salinosporamide A against the asexual stages of the human malaria parasite.
3. A first-in-class glycol-hexadepsipeptide-polyketide (Mollemycin A) was extracted from Streptomyces sp. (CMB-M0244) from a marine sediment from south Molle Island, Australia. The anti-parasitic activity of the mollemycin A was exceptionally potent with IC50 7 nM and 9 nM for drug-sensitive (3D7) and drug-resistant (Dd2) Plasmodium falciparum (Raju et al., 2014), in addition to its potent antibacterial activity.
4. An unusual cyclic peptide cyclomarin A derived from Streptomyces sp. has been found to inhibit P. falciparum growth with IC50 value of 40 nM, in addition to its potent antibacterial activity, albeit with a different mode of action in Mycobacterium (Bürstner et al., 2015). PF14_0349 (PfAp3Ase) was found to be the target enzyme in P. falciparum, which was inhibited by cyclomarin A derivative with IC50 4 nM, with no inhibition for the human orthologue hFHIT (human fragile histidine triad). Co-crystalization of PfAp3Ase dimer and cyclomarin A also validated their binding and potent inhibition of cellular growth due to enhanced levels of P(1)-P(3)-bis(5′-adenosyl) triphosphate (Ap3A). These kinds of potent marine-derived natural products and elucidation of their mode of action propel the exploration efforts of other marine organisms.
5. Marine cyanobacterium Lyngbya Majuscula from Pulau Hantu Besar region found to possess biologically important activities (antimalarial and cytotoxic) cyclodepsipeptides- Lagunamides A and B, structurally related to potent marine cyanobacterial aurilides (Tripathi et al., 2010). IC50 values of 190 and 910 nM of lagunamides A and B, respectively were found against P. falciparum NF54 strain, the former was comparable to the dolostatin 15- another potent marine cyanobacterial compound.
6. Other marine cyanobacteria- Moorea producens, Moorea bouillonii and Okeania sp., as rich source of depsipeptides (lyngbyabellins) have been found to possess significant antimalarial activities. Lyngbyabellin A was the most potent cyclic peptide extracted from Fijian Moorea producens with EC50 1.5 nM against the asexual P. falciparum stages (Sweeney-Jones et al., 2020). Other compounds from antimalarial extracts of Moorea producens were observed to be moderately active against P. berghei liver stage parasites, further computationally predicted to bind to Plasmodium actin-like proteins and sortilin, possibly inhibiting cellular invasion of the parasite. So far 18 lyngbyabellins have been identified, of which LYN1 and LYN2 exhibited potent anti-plasmodial activities (EC50 73 and 100 nM, respectively). Lyngbyabellin A isolated from M. bouillonii and Lyngbyabellin G from Okeania sp. were also found to be anti-plasmodial with IC50 300 and 1,100 nM, respectively (Fathoni et al., 2020). Interestingly, the cytotoxicity of Lyngbyabellin A in HEK293T and HepG2 cell lines was negligible as compared to the anti-plasmodial activity making it a potential candidate for further in vivo experiments and pre clinical studies.
7. Trioxacarcins A-F (antibiotics) from marine Streptomycetes sp. (isolate B8652) were purified and tested for anti-bacterial, anti-tumor and anti-plasmodial activities (Maskey et al., 2004). Trioxacarcins A & D were observed to be highly potent (IC50 1.6 and 2.3 ng/ml, respectively) against P. falciparum NF54 and K1 strain, comparable to artemisinin.
8. C-glycosylated benz[α]anthraquinone derivatives-urdamycinone E, urdamycinone G, dehydroxyaquayamycin; were isolated from marine Streptomycetes sp. (isolate BCC45596) and potent antiplasmodial activities (IC50 0.053, 0.14 and 2.9 nM, respectively) against P. falciparum K1 strain were observed, along with anti-tubercular activities (Supong et al., 2012).
9. Salinipostins A-K derived from marine bacterium Salinispora sp., are novel bioactive compounds containing bicyclic phosphotriester core structure, never observed in natural antimalarials, promising a new lead scaffold (Schulze et al., 2015). Salinipostin A for P. falciparum EC50 50 nM was the most potent derivative, potency majorly derived from the length of aliphatic chains and no. of C atoms in them. Failure of resistance selection experiments using Salinipostin A and further tagged-probes led to the identification of multiple essential serine hydrolases as targets for Salinipostin A, promising a potent antimalarial lead (Yoo et al., 2020).
Marine-derived antimalarials from diverse biota
1. Fijian red macroalga Callophycus serratus has been shown to contain novel brominated macrocyclic meroditerpenes which were antimalarial in vitro. Notably, the most abundant and potent compound was bromophycolide A, arresting the parasite growth strongly (Stout et al., 2011). While the mode of action (MOA) of most of the marine natural products is not clear; to delineate the MOA of bromophyclide A, immunoaffinity (IAF) tags were attached via acetyl linkage. Using IAF tags and confocal microscopy, bromophycolides were found to fluoresce with hemozoin crystals and colocalized with neutral lipids, suggesting inhibition of heme crystallization as plausible MOA of bromophycolides. Structurally, bromophycolides are unique therapeutic motifs, as they do not resemble other natural inhibitors of heme crystallization (xanthones and terpene isonitriles from marine sponges) or other natural products (quinine and artemisinin); thus offering potent lead structures to block heme crystallizaiton-a major physiological target in P. falciparum asexual life cycle.
2. Dolostatin 10 and dolostatin 15 (potential anticancer agents) are two marine peptides derived from sea hare Dolabella auricularia and their synthetic derivatives-auristatins, have been investigated for their antimalarial activities (Fennell et al., 2003). Amongst these, dolostatin 10 was the most effective with IC50 0.1 nM against P. falciparum. The mode of action of dolostatin 10 included nuclear division arrest apparently by disassembly of microtubular mitotic structures, similar to the mode of action of vinblastine-a potent anticancer agent blocking microtubule assembly.
Marine antimalarials screened in high-throughput manner
1. In a unique intiative, Harbor Branch Marine Microorganisms Culture Collection (HBMMCC) has a collection of >19,000 isolates; from which a subset of 2,365 marine isolates was explored for antimalarial screening using SYBR green-I fluorescence assay with P. falciparum Dd2 chloroquine-resistant strain (McCarthy et al., 2019). 17 potent leads were identified with EC50 values < 1 μg/ml; derived from broad taxa including actinomycetes, fungi and gram-negative bacteria; supporting the idea of antimalarials in the form of marine-derived natural products.
2. Using highthroughput screening (HTS) of over 18,000 marine and terrestrial biota extracts to identify some lead antimalarial compounds, fractions from a marine sponge Zyzzya sp. were found to inhibit the in vitro parasite growth and were not observed to be toxic to human cell lines (HEK293). Further, bioassay-guided fractionation and HPLC purification led to the identification of bispyrroloimminoquinone alkaloid -tsitsikammamine C (Davis et al., 2012). The IC50 values of tsitsikammamine C against the P. falciparum (3D7 & Dd2-chloroquine resistant strains) were 13 and 18 nM, indicating the high potency of the marine sponge derived natural product. While, the cytotoxicity in HEK293 cell lines was found to be ∼200 fold higher than the anti-plasmodial activity. Moreover, makaluvamine G-a previously reported compound was also isolated from Zyzzya sponge and also reported therapeutic potential in P. berghei infected mice.
3. Rational screening of endophytic fungal extracts from mangrove plants derived from the coast of Hong-Kong and Taiwan yielded one lead compound-dimeric tetrahydroxanthone, dicerandrol D with antimalarial activity in nanomolar range, while the cytotoxicity was reported ∼8 fold higher in A549 human pulmonary adenocarcinoma cells. (Calcul et al., 2013).
4. High-throughput screening of a prefractionated library including marine invertebrates identified an extract of marine sponge- Plakortis lita with the potential to inhibit P. falciparum 3D7 (Davis et al., 2013). Bioassay guided fractionation led to the identification of four compounds (thiazine-derived alkaloids)- thioplakortones A-D; with IC50 51 and 6.6 nM for the P. falciparum 3D7 and Dd2 strain.
5. Quantitative high-throughput screening of a natural product extracts (NPE) library derived from diverse marine microbes 18 highly potent natural product extracts were identified (Cheng et al., 2015). HPLC fractionation was carried out to identify the active compounds from marine Streptomyces bangulaensis-as Actinormides A and B (non-proteinogenic tetrapeptides), with EC50 200 nM for Actinormaide A against five P. falciparum strains were observed, while the EC50 for HEK293T and HepG2 cell lines was observed to be >4 µM.
6. A targeted screen of 62 aqueous extracts from Cuban marine organisms identified potent protease inhibitors of P. falciparum hemoglobinases (Plasmepsin-II and Falcipain-2; aspartic and cysteine proteases, respectively) and resulted in the functional characterization of a protease inhibitor sourced from Plexaura homomalla (PhPI) (Salas-Sarduy et al., 2017).
7. Pseudilins (halogenated marine natural products) as inhibitors of the IspD enzyme (third enzyme of the non-mevalonate pathway) in Arabidopsis thaliana and P. vivax had been identified from a screen of ∼100,000 compounds (Kunfermann et al., 2014).
Discussion
The objective of this review article is to draw the focus of the readers on the potential of marine-derived antimalarials, especially in the current situation where the emergence of resistance against the frontline antimalarial drugs has become a global concern. The review has collated and summarized the marine-derived antimalarial compounds (FDA-approved drugs and lead compounds) so far, globally. Numerous lead compounds have been identified for their antimalarial potential using HTS platform and hence emphasizing the use of HTS in exploring marine-derived antimalarials. In Section 4, specific emphasis has been given to the global advancements in the identification of marine therapeutics from diverse biota including sponges, bacteria, sea hare and algae etc. Particularly, marine-derived bacteria has come up as the richest source of lead antimalarials with IC50 values in nM range, such as calothrixins, salinosporamide, mollemycin, cyclomarin, lagunamides, lyngbyabellin, trioxacarcins, urdamycinone, and salinipostins. The antimalarial activities of these compounds were observed to be comparable to the present day antimalarials (chloroquine and artemisinin). For ease of handling and high throughput feasibility in the antimalarial assays, majority of the compunds were screened only for the asexual stages of the Plasmodium parasite except for the cyclic peptides from marine cyanobacteria- Moorea producens, which were found to be moderately active against liver stage of P. berghei. There are limited studies worth highlighting which deduced the mode of action of the lead compounds-salinisporamide inhibiting ubiquitination-mediated proteasomal degradation (20S proteasome); cyclomarin A targeting the PF14_0349 (PfAp3Ase) enzyme of P. falciparum and lynbyabellin A computationally predicted to bind Plasmodium actin-like proteins and sortilin, inhibiting parasite invasion. After marine-derived bacteria, sponges also are a significant source of lead antimalarials. Some of the notable sponge-derived potent antimalarials are manzamines, fascaplysins, isonitriles and kalihinols. The mode of action of manzamine A was similar in action as chloroquine via induction of morphological changes and degradation of the parasite observed by transmission electron microscopy. Fascaplysin is peculiar as it was found to have antimalarial activity in against all asexual stages of P. falciparum, but for ring-stage parasites it was ∼190 times more potent than other stages, indicating a stage-specific mode of action. Isonitriles were computationally deduced to inhibit the hemoglobin degradation in Plasmodium, which has been a crucial target for many natural and synthetic antimalarials. Bromophycolides from macroalga- Callophycus serratus, imparted their antimalarial activity by inhibition of heme crystallization to hemozoin. Marine-derived peptides such as dolostatin 10 is a highly potent antimalarial which causes alteration in microtubule assembly during mitosis.
It is notable that marine-derived compounds also provide novel chemical scaffolds or active chemical moieties, on which new or modified synthetic derivatives can be developed. Calothrixins are pentacyclic metabolites extracted from marine cyanobacteria, with potent antimalarial activity, where the bioactive scaffold contained atypical indolo[3,2-j]phenanthridine ring system. Another important lead antimalarial-salinipostins contained unique bicyclic phosphotriester core structure, where the antimalarial activity was dependent on the length of aliphatic chains. Isonitriles are important class of antimalarials derived from marine sponge- Cymbastela hooperi, which inhibits hemoglobin degradation via coordinate bond with the heme molecule. Bromophycolides are brominated macrocyclic meroditerpenes possessing novel therapeutic motifs, that has not been observed in other heme crystallization inhibitors such as xanthones, terpene isonitriles, quinine and artemisinin. Synthetic derviatives of dolostatins-auristatins have been found to be potent antimalarial compounds.
The discovery of these lead antimalarials derived from marine orgnaisms clearly makes the case for vast unexplored potential of the marine biodiversity, especially focusing on discovery of novel drugs for malaria. However, with their significant antimalarial activity and chemical structures available, detailed investigations elucidating the mechanisms of action against the human malaria parasite- P. falciparum are lacking; only few notable studies are available. These studies elicit enough confidence that marine therapeutics could be worth exploring potent antimalarials.
In the context of the antimalarial landscape of India, particularly marine-derived, there is not much information/exploration available. Moreover, the Indian coastline is approximately 8,000 km, spanning the peninsular region as well as the islands (Andaman and Nicobar Islands and Lakshadweep Islands), which harbors unique marine habitats with a great marine biodiversity. The Indian peninsula is surrounded by three distinct ocean bodies- Bay of Bengal, Indian Ocean and Arabian Sea with their own marine ecological systems defining the marine biodiversity in estuaries, lagoons, mangroves, backwaters, salt marshes, rocky coasts, sandy stretches and coral reefs. The eastern and western coast of India comprise of huge marine floral and faunal diversity (no. of species)- marine algae (844), sponges (451), corals (200), crustacean (2,900), marine mollusks (3,370), echinoderm (765), tunicates (47) etc. and theire associated microorganisms (Saxena, 2012). There are a number of marine research institutes across the Indian peninsula, with their core research expertise in the isolation and characterizing of the marine extracts; but rarely an antimalarial-focused study was undertaken. While writing this review, we have embarked on a collaborative project to undertake an exploration of marine-derived natural extract from different research institutes across the Indian peninsula; few leads have been identified from ∼100 marine-derived extracts. ICMR-National Institute of Malaria Research with its field units spanning across India (Figure 2) can play a central role in the exploration of new antimalarials from marine-derived natural extracts in a cost-effective and resource limited manner.
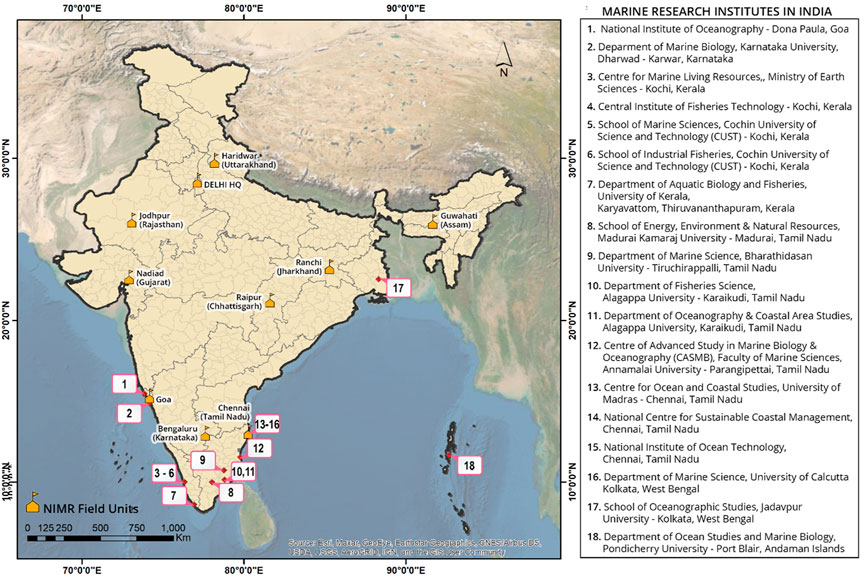
FIGURE 2. A list of marine research institutes in India, particularly located at the peninsular region with the potential to explore marine biodiversity.
Conclusion and future outlook
This review article has now clearly established that marine-derived extracts/compounds have significant antimalarial potential, which has not been explored to its capacity. The marine biodiversity offers a vast chemical space for discovery of novel chemical scaffolds for development of novel compounds, which can be used as therapeutics in various diseases including malaria. Particularly, in Indian perspective with its vast coastline and huge marine biodiversity, there is clear lack of efforts in screening the marine-derived extracts for discovery of novel antimalarials, which of course have multiple factors-lack of state-of-art technologies/infrastructure, collaborations and sharing of expertise etc. Therefore, it is apt fro the malaria research community to come forward and collaborate with the marine research community to screen the marine-derived extracts/compounds as potent antimalarials. The lead compounds can be further investigated for their mode of action, target identification and preclinical studies. In light of the challenges of malaria elimination, globally, drug discovery is a prominent tool against malaria parasite and should be explored thoroughly from natural sources.
Author contributions
Conceived the study: KP, KV, CP, and NT. Wrote the manuscript: CP, KV, and SH. Reviewed the manuscript: KP, NT, and SC. All authors read and approved the final manuscript.
Funding
Junior Research Fellowship of CP is funded by Council of Scientific and Industrial Research (CSIR) (09/905(0020)/2019-EMR-I), Delhi. The research work pertaining to this review article is funded by intramural funding support from ICMR-NIMR, Delhi. The publication bears the NIMR publication committee no. RIC-18/2022. We thank the funding support from SC (Ramalingaswami Fellowship, DBT, India) and KV (Indo-Korean Global Research Program on Importable Infectious Diseases, National Research Foundation, South Korea).
Acknowledgments
We thank our collaborators from various marine research institutes across the country for providing marine and natural products extracts for antimalarials screening. We would like to thank Rahul for his thoughtful insights in drafting the review manuscript.
Conflict of interest
The authors declare that the research was conducted in the absence of any commercial or financial relationships that could be construed as a potential conflict of interest.
Publisher’s note
All claims expressed in this article are solely those of the authors and do not necessarily represent those of their affiliated organizations, or those of the publisher, the editors and the reviewers. Any product that may be evaluated in this article, or claim that may be made by its manufacturer, is not guaranteed or endorsed by the publisher.
References
Ahyong, S., Boyko, C. B., Bailly, N., Bernot, J., Bieler, R., Brandão, , et al. (2022). World Register of Marine Species. Available at: https://www.marinespecies.org at VLIZ (Accessed November 26, 2022). doi:10.14284/170
Ameen, F., AlNadhari, S., and Al-Homaidan, A. A. (2021). Marine microorganisms as an untapped source of bioactive compounds. Saudi J. Biol. Sci. 28, 224–231. doi:10.1016/j.sjbs.2020.09.052
Ang, K. K., Holmes, M. J., Higa, T., Hamann, M. T., and Kara, U. A. (2000). In vivo antimalarial activity of the beta-carboline alkaloid manzamine A. Antimicrob. Agents Chemother. 44, 1645–1649. doi:10.1128/aac.44.6.1645-1649.2000
Antonova-Koch, Y., Meister, S., Abraham, M., Luth, M. R., Ottilie, S., Lukens, A. K., et al. (2018). Open-source discovery of chemical leads for next-generation chemoprotective antimalarials. Science 362, eaat9446. doi:10.1126/science.aat9446
Bakkali, F., Averbeck, S., Averbeck, D., and Idaomar, M. (2008). Biological effects of essential oils–a review. Food Chem. Toxicol. 46, 446–475. doi:10.1016/j.fct.2007.09.106
Barbosa, A. J., and Roque, A. C. (2019). Free marine natural products databases for biotechnology and bioengineering. Biotechnol. J. 14, 1800607. doi:10.1002/biot.201800607
Battle, K. E., and Baird, J. K. (2021). The global burden of Plasmodium vivax malaria is obscure and insidious. PLoS Med. 18, e1003799. doi:10.1371/journal.pmed.1003799
Blunt, J. W., Copp, B. R., Keyzers, R. A., Munro, M. H., and Prinsep, M. R. (2016). Marine natural products. Nat. Prod. Rep. 33, 382–431. doi:10.1039/c5np00156k
Buchholz, K., Burke, T. A., Williamson, K. C., Wiegand, R. C., Wirth, D. F., and Marti, M. (2011). A high-throughput screen targeting malaria transmission stages opens new avenues for drug development. J. Infect. Dis. 203, 1445–1453. doi:10.1093/infdis/jir037
Bürstner, N., Roggo, S., Ostermann, N., Blank, J., Delmas, C., Freuler, F., et al. (2015). Gift from nature: Cyclomarin A kills mycobacteria and malaria parasites by distinct modes of action. ChemBioChem 16, 2433–2436. doi:10.1002/cbic.201500472
Calcul, L., Waterman, C., Ma, W. S., Lebar, M. D., Harter, C., Mutka, T., et al. (2013). Screening mangrove endophytic fungi for antimalarial natural products. Mar. Drugs 11, 5036–5050. doi:10.3390/md11125036
Caridha, D., Hickman, M., Xie, L., Ngundam, F., Milner, E., Schenk, A., et al. (2019). Updating the modified Thompson test by using whole-body bioluminescence imaging to replace traditional efficacy testing in experimental models of murine malaria. Malar. J. 18, 38–15. doi:10.1186/s12936-019-2661-x
Cervantes, S., Prudhomme, J., Carter, D., Gopi, K. G., Li, Q., Chang, Y.-T., et al. (2009). High-content live cell imaging with RNA probes: Advancements in high-throughput antimalarial drug discovery. BMC Cell Biol. 10, 45–10. doi:10.1186/1471-2121-10-45
Cheng, K. C.-C., Cao, S., Raveh, A., MacArthur, R., Dranchak, P., Chlipala, G., et al. (2015). Actinoramide A identified as a potent antimalarial from titration-based screening of marine natural product extracts. J. Nat. Prod. 78, 2411–2422. doi:10.1021/acs.jnatprod.5b00489
Cheng, M.-M., Tang, X.-L., Sun, Y.-T., Song, D.-Y., Cheng, Y.-J., Liu, H., et al. (2020). Biological and chemical diversity of marine sponge-derived microorganisms over the last two decades from 1998 to 2017. Molecules 25, 853. doi:10.3390/molecules25040853
Cheruiyot, A. C., Auschwitz, J. M., Lee, P. J., Yeda, R. A., Okello, C. O., Leed, S. E., et al. (2016). Assessment of the Worldwide Antimalarial Resistance Network standardized procedure for in vitro malaria drug sensitivity testing using SYBR green assay for field samples with various initial parasitemia levels. Antimicrob. Agents Chemother. 60, 2417–2424. doi:10.1128/AAC.00527-15
Corbett, Y., Herrera, L., Gonzalez, J., Cubilla, L., Capson, T. L., Coley, P. D., et al. (2004). A novel DNA-based microfluorimetric method to evaluate antimalarial drug activity. Am. J. Trop. Med. Hyg. 70, 119–124. doi:10.4269/ajtmh.2004.70.119
Cui, L., Miao, J., Wang, J., Li, Q., and Cui, L. (2008). Plasmodium falciparum: Development of a transgenic line for screening antimalarials using firefly luciferase as the reporter. Exp. Parasitol. 120, 80–87. doi:10.1016/j.exppara.2008.05.003
Davis, G. D. J., and Vasanthi, A. H. R. (2011). Seaweed metabolite database (SWMD): A database of natural compounds from marine algae. Bioinformation 5, 361–364. doi:10.6026/97320630005361
Davis, R. A., Buchanan, M. S., Duffy, S., Avery, V. M., Charman, S. A., Charman, W. N., et al. (2012). Antimalarial activity of pyrroloiminoquinones from the Australian marine sponge Zyzzya sp. J. Med. Chem. 55, 5851–5858. doi:10.1021/jm3002795
Davis, R. A., Duffy, S., Fletcher, S., Avery, V. M., Quinn, R. J., and Thiaplakortones, A–D. (2013). Thiaplakortones A-D: Antimalarial thiazine alkaloids from the Australian marine sponge Plakortis lita. J. Org. Chem. 78, 9608–9613. doi:10.1021/jo400988y
Dennull, R. A., Reinbold, D. D., Waters, N. C., and Johnson, J. D. (2009). Assessment of malaria in vitro drug combination screening and mixed-strain infections using the malaria Sybr green I-based fluorescence assay. Antimicrob. Agents Chemother. 53, 2557–2563. doi:10.1128/AAC.01370-08
Desjardins, R. E., Canfield, C., Haynes, J., and Chulay, J. (1979). Quantitative assessment of antimalarial activity in vitro by a semiautomated microdilution technique. Antimicrob. Agents Chemother. 16, 710–718. doi:10.1128/aac.16.6.710
Druilhe, P., Moreno, A., Blanc, C., Brasseur, P. H., and Jacquier, P. (2001). A colorimetric in vitro drug sensitivity assay for Plasmodium falciparum based on a highly sensitive double-site lactate dehydrogenase antigen-capture enzyme-linked immunosorbent assay. Am. J. Trop. Med. Hyg. 64, 233–241. doi:10.4269/ajtmh.2001.64.233
Ebada, S. S., and Proksch, P., The chemistry of marine sponges. Handb. Mar. Nat. Prod. (2012) 298, 191, doi:10.1007/978-90-481-3834-0_4
El Sayed, K. A. (1996). The marine environment: A resource for prototype antimalarial agents. J. Nat. Toxins 5, 261–285.
Fathoni, I., Petitbois, J. G., Alarif, W. M., Abdel-Lateff, A., Al-Lihaibi, S. S., Yoshimura, E., et al. (2020). Bioactivities of lyngbyabellins from cyanobacteria of Moorea and Okeania genera. Molecules 25, 3986. doi:10.3390/molecules25173986
Fennell, B., Carolan, S., Pettit, G., and Bell, A. (2003). Effects of the antimitotic natural product dolastatin 10, and related peptides, on the human malarial parasite Plasmodium falciparum. J. Antimicrob. Chemother. 51, 833–841. doi:10.1093/jac/dkg151
Ghareeb, M. A., Tammam, M. A., El-Demerdash, A., and Atanasov, A. G. (2020). Insights about clinically approved and Preclinically investigated marine natural products. Curr. Res. Biotechnol. 2, 88–102. doi:10.1016/j.crbiot.2020.09.001
Harvey, A. L., Edrada-Ebel, R., and Quinn, R. J. (2015). The re-emergence of natural products for drug discovery in the genomics era. Nat. Rev. Drug Discov. 14, 111–129. doi:10.1038/nrd4510
Hasenkamp, S., Sidaway, A., Devine, O., Roye, R., and Horrocks, P. (2013). Evaluation of bioluminescence-based assays of anti-malarial drug activity. Malar. J. 12, 58–10. doi:10.1186/1475-2875-12-58
Hughes, J. P., Rees, S., Kalindjian, S. B., and Philpott, K. L. (2011). Principles of early drug discovery. Br. J. Pharmacol. 162, 1239–1249. doi:10.1111/j.1476-5381.2010.01127.x
Ioannou, E., Abdel-Razik, A. F., Zervou, M., Christofidis, D., Alexi, X., Vagias, C., et al. (2009). 5alpha, 8alpha-epidioxysterols from the gorgonian eunicella cavolini and the ascidian trididemnum inarmatum: Isolation and evaluation of their antiproliferative activity. Steroids 74, 73–80. doi:10.1016/j.steroids.2008.09.007
Joanny, F., Held, J., and Mordmüller, B. (2012). In vitro activity of fluorescent dyes against asexual blood stages of Plasmodium falciparum. Antimicrob. Agents Chemother. 56, 5982–5985. doi:10.1128/AAC.00709-12
Kildgaard, S., Subko, K., Phillips, E., Goidts, V., De la Cruz, M., Díaz, C., et al. (2017). A dereplication and bioguided discovery approach to reveal new compounds from a marine-derived fungus Stilbella fimetaria. Mar. Drugs 15, 253. doi:10.3390/md15080253
Kirsch, G., König, G. M., Wright, A. D., and Kaminsky, R. (2000). A new bioactive sesterterpene and antiplasmodial alkaloids from the marine sponge Hyrtios cf. erecta. J. Nat. Prod. 63, 825–829. doi:10.1021/np990555b
Koehn, F. E. (2008). High impact technologies for natural products screening. Nat. Compd. as Drugs Vol. I, 175–210.
Kunfermann, A., Witschel, M., Illarionov, B., Martin, R., Rottmann, M., Höffken, H. W., et al. (2014). Pseudilins: Halogenated, allosteric inhibitors of the non-mevalonate pathway enzyme IspD. Angew. Chem. Int. Ed. Engl. 53, 2235–2239. doi:10.1002/anie.201309557
Li, Q., Gerena, L., Xie, L., Zhang, J., Kyle, D., and Milhous, W. (2007). Development and validation of flow cytometric measurement for parasitemia in cultures of P. falciparum vitally stained with YOYO-1. Cytom. A 71, 297–307. doi:10.1002/cyto.a.20380
Lu, Z., Ding, Y., Li, X.-C., Djigbenou, D. R., Grimberg, B. T., Ferreira, D., et al. (2011). 3-Bromohomofascaplysin A, a fascaplysin analogue from a Fijian Didemnum sp. ascidian. Bioorg. Med. Chem. 19, 6604–6607. doi:10.1016/j.bmc.2011.05.046
Lucantoni, L., Duffy, S., Adjalley, S. H., Fidock, D. A., and Avery, V. M. (2013). Identification of MMV malaria box inhibitors of Plasmodium falciparum early-stage gametocytes using a luciferase-based high-throughput assay. Antimicrob. Agents Chemother. 57, 6050–6062. doi:10.1128/AAC.00870-13
Lucantoni, L., Fidock, D. A., and Avery, V. M. (2016). Luciferase-based, high-throughput assay for screening and profiling transmission-blocking compounds against Plasmodium falciparum gametocytes. Antimicrob. Agents Chemother. 60, 2097–2107. doi:10.1128/AAC.01949-15
Luesch, H., Moore, R. E., Paul, V. J., Mooberry, S. L., and Corbett, T. H. (2001). Isolation of dolastatin 10 from the marine cyanobacterium Symploca species VP642 and total stereochemistry and biological evaluation of its analogue symplostatin 1. J. Nat. Prod. 64, 907–910. doi:10.1021/np010049y
Makler, M., Ries, J., Williams, J., Bancroft, J., Piper, R., Gibbins, B., et al. (1993). Parasite lactate dehydrogenase as an assay for Plasmodium falciparum drug sensitivity. Am. J. Trop. Med. Hyg. 48, 739–741. doi:10.4269/ajtmh.1993.48.739
Malve, H. (2016). Exploring the ocean for new drug developments: Marine pharmacology. J. Pharm. Bioallied Sci. 8, 83–91. doi:10.4103/0975-7406.171700
Maskey, R. P., Helmke, E., Kayser, O., Fiebig, H. H., Maier, A., Busche, A., et al. (2004). Anti-cancer and antibacterial trioxacarcins with high anti-malaria activity from a marine Streptomycete and their absolute stereochemistry. J. Antibiot. 57, 771–779. doi:10.7164/antibiotics.57.771
McCarthy, P. J., Roberts, B. F., Carbonell, A., Roberts, J., Wright, A. E., and Chakrabarti, D. (2019). Marine microbiome as a source of antimalarials. Trop. Med. Infect. Dis. 4, 103. doi:10.3390/tropicalmed4030103
Mehbub, M. F., Lei, J., Franco, C., and Zhang, W. (2014). Marine sponge derived natural products between 2001 and 2010: Trends and opportunities for discovery of bioactives. Mar. Drugs 12, 4539–4577. doi:10.3390/md12084539
Miyaoka, H., Shimomura, M., Kimura, H., Yamada, Y., Kim, H.-S., and Yusuke, W. (1998). Antimalarial activity of kalihinol A and new relative diterpenoids from the Okinawan sponge, Acanthella sp. Tetrahedron 54, 13467–13474. doi:10.1016/s0040-4020(98)00818-7
Nakao, Y., Yeung, B. K., Yoshida, W. Y., Scheuer, P. J., Kelly-Borges, M., and Kapakahine, B. (1995). Kapakahine B, a cyclic hexapeptide with an .alpha.-carboline ring system from the marine sponge Cribrochalina olemda. J. Am. Chem. Soc. 117, 8271–8272. doi:10.1021/ja00136a026
Nambati, E. A., Kiarie, W. C., Kimani, F., Kimotho, J. H., Otinga, M. S., Too, E., et al. (2018). Unclear association between levels of Plasmodium falciparum lactate dehydrogenase (pf LDH) in saliva of malaria patients and blood parasitaemia: Diagnostic implications? Malar. J. 17, 9–5. doi:10.1186/s12936-017-2151-y
Nass, J., and Efferth, T. (2019). Development of artemisinin resistance in malaria therapy. Pharmacol. Res. 146, 104275. doi:10.1016/j.phrs.2019.104275
Noedl, H., Wernsdorfer, W. H., Miller, R. S., and Wongsrichanalai, C. (2002). Histidine-rich protein II: A novel approach to malaria drug sensitivity testing. Antimicrob. Agents Chemother. 46, 1658–1664. doi:10.1128/aac.46.6.1658-1664.2002
Palanisamy, S. K., Rajendran, N., and Marino, A. (2017). Natural products diversity of marine ascidians (Tunicates; Ascidiacea) and successful drugs in clinical development. Nat. Prod. Bioprospect. 7, 1–111. doi:10.1007/s13659-016-0115-5
Pallela, R., Ehrlich, H., and Bhatnagar, I. (2016). Marine sponges: Chemicobiological and biomedical applications. Springer.
Pérez-Moreno, G., Cantizani, J., Sánchez-Carrasco, P., Ruiz-Pérez, L. M., Martín, J., El Aouad, N., et al. (2016). Discovery of new compounds active against Plasmodium falciparum by high throughput screening of microbial natural products. PLoS One 11, e0145812. doi:10.1371/journal.pone.0145812
Prudhomme, J., McDaniel, E., Ponts, N., Bertani, S., Fenical, W., Jensen, P., et al. (2008). Marine actinomycetes: A new source of compounds against the human malaria parasite. PloS one 3, e2335. doi:10.1371/journal.pone.0002335
Radwan, M., Hanora, A., Khalifa, S., and Abou-El-Ela, S. H. (2012). Manzamines: A potential for novel cures. Cell Cycle 11, 1765–1772. doi:10.4161/cc.20135
Raju, R., Khalil, Z. G., Piggott, A. M., Blumenthal, A., Gardiner, D. L., Skinner-Adams, T. S., et al. (2014). Mollemycin A: An antimalarial and antibacterial glyco-hexadepsipeptide-polyketide from an Australian marine-derived Streptomyces sp. (CMB-M0244). Org. Lett. 16, 1716–1719. doi:10.1021/ol5003913
Rason, M. A., Randriantsoa, T., Andrianantenaina, H., Ratsimbasoa, A., and Menard, D. (2008). Performance and reliability of the SYBR Green I based assay for the routine monitoring of susceptibility of Plasmodium falciparum clinical isolates. Trans. R. Soc. Trop. Med. Hyg. 102, 346–351. doi:10.1016/j.trstmh.2008.01.021
Rickards, R. W., Rothschild, J. M., Willis, A. C., de Chazal, N. M., Kirk, J., Kirk, K., et al. (1999). Calothrixins A and B, novel pentacyclic metabolites from Calothrix cyanobacteria with potent activity against malaria parasites and human cancer cells. Tetrahedron 55, 13513–13520. doi:10.1016/s0040-4020(99)00833-9
Salas-Sarduy, E., Guerra, Y., Covaleda Cortés, G., Avilés, F. X., and Chávez Planes, M. A. (2017). Identification of tight-binding plasmepsin II and falcipain 2 inhibitors in aqueous extracts of marine invertebrates by the combination of enzymatic and interaction-based assays. Mar. Drugs 15, 123. doi:10.3390/md15040123
Saxena, A. (2012). “Marine biodiversity in India: Satus and issues,” in D. Indira gandhi national forest academy (Uttarakhand, IndiaUttarakhand, 127–134.
Schulze, C. J., Navarro, G., Ebert, D., DeRisi, J., Linington, R. G., and Salinipostins, A–K. (2015). Salinipostins A-K, long-chain bicyclic phosphotriesters as a potent and selective antimalarial chemotype. J. Org. Chem. 80, 1312–1320. doi:10.1021/jo5024409
Shen, L., Niu, J., Wang, C., Huang, B., Wang, W., Zhu, N., et al. (2019). High-throughput screening and identification of potent broad-spectrum inhibitors of coronaviruses. J. Virol. 93, 19. doi:10.1128/JVI.00023-19
Singh, A., and Thakur, N. L. (2016). Significance of investigating allelopathic interactions of marine organisms in the discovery and development of cytotoxic compounds. Chem. Biol. Interact. 243, 135. doi:10.1016/j.cbi.2015.09.009
Smith, E., Davis-Gardner, M. E., Garcia-Ordonez, R. D., Nguyen, T.-T., Hull, M., Chen, E., et al. (2020). High-throughput screening for drugs that inhibit papain-like protease in SARS-CoV-2. SLAS Discov. 25, 1152–1161. doi:10.1177/2472555220963667
Stout, E. P., Cervantes, S., Prudhomme, J., France, S., La Clair, J. J., Le Roch, K., et al. (2011). Bromophycolide A targets heme crystallization in the human malaria parasite Plasmodium falciparum. ChemMedChem 6, 1572–1577. doi:10.1002/cmdc.201100252
Subramani, R., and Sipkema, D. (2019). Marine rare actinomycetes: A promising source of structurally diverse and unique novel natural products. Mar. Drugs 17, 249. doi:10.3390/md17050249
Subramanian, G., Belekar, M. A., Shukla, A., Tong, J. X., Sinha, A., Chu, T. T., et al. (2018). Targeted phenotypic screening in Plasmodium falciparum and Toxoplasma gondii reveals novel modes of action of medicines for malaria venture malaria box molecules. Msphere 3, 005344-17–e617. doi:10.1128/mSphere.00534-17
Supong, K., Thawai, C., Suwanborirux, K., Choowong, W., Supothina, S., and Pittayakhajonwut, P. (2012). Antimalarial and antitubercular C-glycosylated benz [α] anthraquinones from the marine-derived Streptomyces sp. BCC45596. Phytochem. Lett. 5, 651–656. doi:10.1016/j.phytol.2012.06.015
Swann, J., Corey, V., Scherer, C. A., Kato, N., Comer, E., Maetani, M., et al. (2016). High-throughput luciferase-based assay for the discovery of therapeutics that prevent malaria. ACS Infect. Dis. 2, 281–293. doi:10.1021/acsinfecdis.5b00143
Sweeney-Jones, A. M., Gagaring, K., Antonova-Koch, J., Zhou, H., Mojib, N., Soapi, K., et al. (2020). Antimalarial peptide and polyketide natural products from the Fijian marine cyanobacterium Moorea producens. Mar. Drugs 18, 167. doi:10.3390/md18030167
Thakur, N. L., and Müller, W. E. (2004). Biotechnological potential of marine sponges. Curr. Sci., 1506–1512.
Tripathi, A., Puddick, J., Prinsep, M. R., Rottmann, M., Tan, L. T., and Lagunamides, A., (2010). Lagunamides A and B: Cytotoxic and antimalarial cyclodepsipeptides from the marine cyanobacterium Lyngbya majuscula. J. Nat. Prod. 73, 1810–1814. doi:10.1021/np100442x
Varijakzhan, D., Yang, S.-K., Chong, C. M., Akseer, R., Alhosani, M. S., Thomas, W., et al. (2021). Essential oils as potential antimicrobial agents. Sustain. Agric. Rev. 49, 93–122.
Wang, X., Yu, H., Xing, R., and Li, P. (2017). Characterization, preparation, and purification of marine bioactive peptides. Biomed. Res. Int. 2017, 9746720. doi:10.1155/2017/9746720
Wright, A. D., McCluskey, A., Robertson, M. J., MacGregor, K. A., Gordon, C. P., and Guenther, J. (2011). Anti-malarial, anti-algal, anti-tubercular, anti-bacterial, anti-photosynthetic, and anti-fouling activity of diterpene and diterpene isonitriles from the tropical marine sponge Cymbastela hooperi. Org. Biomol. Chem. 9, 400–407. doi:10.1039/c0ob00326c
Xie, C.-L., Xia, J.-M., Wang, J.-S., Lin, D.-H., and Yang, X.-W. (2018). Metabolomic investigations on Nesterenkonia flava revealed significant differences between marine and terrestrial actinomycetes. Mar. Drugs 16, 356. doi:10.3390/md16100356
Yeung, B. K., Nakao, Y., Kinnel, R. B., Carney, J. R., Yoshida, W. Y., Scheuer, P. J., et al. (1996). The kapakahines, cyclic peptides from the marine sponge Cribrochalina olemda. J. Org. Chem. 61, 7168–7173. doi:10.1021/jo960725e
Yoo, E., Schulze, C. J., Stokes, B. H., Onguka, O., Yeo, T., Mok, S., et al. (2020). The antimalarial natural product salinipostin A identifies essential α/β serine hydrolases involved in lipid metabolism in P. falciparum parasites. Cell Chem. Biol. 27, 143–157. e5. doi:10.1016/j.chembiol.2020.01.001
Keywords: malaria, plasmodium, natural product, antimalarial, marine natural bioactive compounds, marine natural product, artemisinin resistance
Citation: Prashar C, Thakur N, Chakraborti S, Areeb Hussain SS, Vashisht K and Pandey KC (2022) The landscape of nature-derived antimalarials-potential of marine natural products in countering the evolving Plasmodium. Front. Drug. Discov. 2:1065231. doi: 10.3389/fddsv.2022.1065231
Received: 09 October 2022; Accepted: 18 November 2022;
Published: 07 December 2022.
Edited by:
Brijesh Rathi, University of Delhi, IndiaReviewed by:
Vinoth Rajendran, Pondicherry University, IndiaOlusola Ojurongbe, Ladoke Akintola University of Technology, Nigeria
Copyright © 2022 Prashar, Thakur, Chakraborti, Areeb Hussain, Vashisht and Pandey. This is an open-access article distributed under the terms of the Creative Commons Attribution License (CC BY). The use, distribution or reproduction in other forums is permitted, provided the original author(s) and the copyright owner(s) are credited and that the original publication in this journal is cited, in accordance with accepted academic practice. No use, distribution or reproduction is permitted which does not comply with these terms.
*Correspondence: Kailash C. Pandey, cGFuZGV5LmthaWxhc2g3MEBnbWFpbC5jb20=; Kapil Vashisht, dmFzaGlzaHQua2FwaWwwN0BnbWFpbC5jb20=