- 1Department of Pharmaceutical Sciences, Temple University School of Pharmacy, Philadelphia, PA, United States
- 2Oral Microbiome Research Laboratory, Kornberg School of Dentistry, Temple University, Philadelphia, PA, United Stated
Invasive candidiasis remains a significant health concern, as it is associated with a high mortality risk. In addition, the risk of infection is significantly elevated in immunocompromised patients such as those with HIV, cancer, or those taking imcmunosuppressive drugs as a result of organ transplantation. The majority of these cases are caused by C. albicans, and C. glabrata is the second most common cause. These infections are typically treated using approved antifungal agents, but the rise of drug-resistant fungi is a serious concern. As part of our on-going effort to identify novel antifungal agents, we have studied the in vitro antifungal properties of a series of sulfonamide analogs of (2S, 4R)-Ketoconazole. Herein we report on the in vitro activity against the key fungal pathogens C. albicans, and C. glabrata.
Introduction
According to the U.S. Center for Disease Control (CDC), invasive candidiasis remains a significant health issue. The average incidence rate of candidemia in 2013–2017 was 9 per 100,000, and it is estimated that ∼25,000 new cases occur annually nationwide (Tsay et al., 2018). The annual global incidence of invasive candidiasis is ∼750,000 (Bongomin et al., 2017). Candida is one of the most common causes of bloodstream infections in the U.S (Magill et al., 2018). In addition, candidemia is associated with a high mortality risk. The U.S. CDC reported that between 2012 and 2016, ∼25% of candidemia patients died while hospitalized (US CDC website 2022b). Though less common, invasive candidiasis can also occur in various organs in the absence of bloodstream infection. The danger of fungal infection is significantly elevated when the immune system is compromised by viral infection (e.g. Human Immunodeficiency Virus (HIV)/Acquired Immunodeficiency Syndrome (AIDS)), immunosuppressive agents designed to support organ transplants, anticancer agents, and antibody therapies used to treat autoimmune disorders. Risks are also elevated when natural barriers are disrupted (e.g., surgery, burns) (Monk et al., 2020).
Importantly, nearly 95% of all forms of invasive candida infection in the U.S. are caused by 5 candida species (C. albicans, C. glabrata, C. parapsilosis, C. tropicalis, and C. krusei.). C. albicans is the leading cause of candida infection in the U.S., but non-C albicans infections account for nearly 2/3 of candidemia case in the U.S. (US CDC website (2022b). C. glabrata is the second most common invasive fungal species, accounting for 15-25% of clinical isolates in the U.S. (Pfaller and Diekema, 2007; McCarty and Pappas 2016). This species is associated with high morbidity and mortality (40-60%) (Timmermans et al., 2018). In addition, there has also been a steady increase in fungal drug resistance (Roemer and Krysan, 2014). Much like antibacterial agents, prolonged and/or repeated clinical use of antifungal agents induces resistance pathways and the emergence of drug-resistant fungi (Sanglard et al., 1999; Vermitsky and Edlind, 2004; Chau et al., 2006). The broad use of antifungal agents in the agrochemical sector has also been linked to the development of cross-resistance to antifungal agents used in medicine (Snelders et al., 2008; Snelders et al. 2011; Snelders et al. 2015). Drug resistance is increasingly problematic with C. albicans as well as non-albicans Candida species such as C. glabrata (Vallabhaneni et al., 2015; Kolaczkowska and Kolaczkowski. 2016).
Fungal infections are typically treated with medications from one of four class of antifungal agents; polyenes, echinocandins, polyenes, and azoles (Griffith et al. (2020)). In 1958. Chlormidazole (1) (Figure 1) became the first clinically available azole antifungal agent, and in 1981, Janssen Pharmaceuticals received U.S. Food and Drug Administration (FDA) approval for the first orally available antifungal agent, (±)-Ketoconazole (2) (Figure 1). For nearly a decade, this medication was the only orally available antifungal agent (Maertens, 2004), but it has been conclusively linked to potentially severe safety issues (hepatoxicity, adrenal insufficiency, and Cytochrome P450 3A4 (Cyp3A4) mediated drug/drug interaction). This led the FDA to severely restricted oral use of (±)-Ketoconazole (2) in 2013 (FDA Website, 2022).
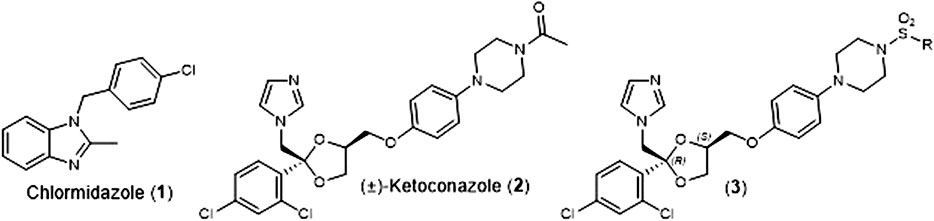
FIGURE 1. Structures of Chlormidazole (1), (±)-Ketoconazole (2), and (2S, 4R)-Ketoconazole sulfonamide analogs (3).
As part of our effort to address the need for new antifungal agents, we have been studying the chemical space surround (±)-Ketoconazole (2). We were surprised to discover that since its initial discovery (Backx et al., 1979), there have been very few reports of direct analogs of (±)-Ketoconazole (2) or its individual enantiomers. In 1992, D. M. Rotstein et al. reported on the ability of the 4 stereoisomers for (±)-Ketoconazole (2) to inhibit lanosterol 14α-demthylase (Cytochrome P450 51a, Cyp51a), the biochemical target that gives rise to the azole’s antifungal properties. They found that (2S, 4R)-Ketoconazole was the most potent inhibitor of the four (Rotstein et al., 1992). Separately, we have reported on the synthesis and evaluation of a series of (2S, 4R)-Ketoconazole sulfonamide analogs (3) (Figure 1) as potential treatments for metabolic syndrome (Blass et al., 2016). Herein, we report the in vitro antifungal properties of these compounds against C. albicans and C. glabrata.
Material and methods
Chemical compounds: The (2S, 4R)-Ketoconazole sulfonamide analogs were prepared according to our previously reported methods (Blass et al., 2016). Briefly, (2S, 4R)-Ketoconazole (4) was hydrolyzed with NaOH in refluxing methanol/water and the resulting product was converted to a sulfonamide (3a-3l) using the corresponding sulfonyl chloride (5) in the presence of NEt3 and CH2Cl2 (Figure 2). Amphotercin B was purchased from ACTGene Inc. (Catalog number R1397-1 g). (±)-ketoconazole we purchased from Combi-Blocks Inc. (Catalog number QA-7778-1 g).
Broth microdilution susceptibility test: The minimum inhibitory concentrations (MIC75) were determined by the broth microdilution method according to the Clinical and Laboratory Standards Institute (CLSI) M27-A4 standard (CLSI, 2017)). Briefly, a suspension of C. albicans or C. glabrata culture (∼0.5–2.5 × 103 cells/ml) was resuspended in RPMI 1640 media (Fisher Catalog number 11875093). The resuspended culture was further incubated with 100 μl of two-fold serially diluted test compounds (100–0.8 µM and 1.0 µM to 7 nM) at 30 °C for 24-48 h. Positive and negative control (wells without antifungal agents and wells without yeast) were conducted. Increased optical density (OD) corresponds to the Candida growth and was quantified by comparison with untreated Candida control samples. The MIC75 of test compounds was determined visually and spectrophotometrically (OD600) after 24-48 h. Experiments were run in triplicate, and data was reported as a mean.
Cyp3A4 inhibition assay: Human Cyp3A4 Inhibition assay: An IC50 for inhibition human Cyp3A4 metabolism of midazolam to 1-OH-midazolam was determined with 10 concentrations of test compounds (half-log serial dilutions; duplicate points). Assays were conducted in 1 ml 96 well polypropylene plates containing 100 µL of 100 mM potassium phosphate (pH 7.4), 3 mM MgCl2, 2 µM midazolam, 1 mM NADPH (tetra Na salt), Corning Gentest supersomes (product 456202: 3 pmol/ml human Cyp3A4, 47 nmol/min human P450 reductase activity, 14 pmol human cytochrome b5; and product 456201: insect control microsome protein; total final microsome protein concentration 0.15 mg/ml) and test compound. All components except NADPH were added to a prewarmed plate and reactions were initiated by adding NADPH. After 30 min at 37°C, reactions were terminated with 200 µL acetonitrile containing 30 µM prednisone. After centrifugation for 10 min at 2200 x g, 165 µL of supernatants were transferred to analysis plate. Samples were analyzed for 1-OH-midazalam concentration, using prednisone as the internal standard, using a Waters Aquity UPLC in tandem with a Xevo TQ MS mass spectrometer (BEH C18 column; 5-95% acetonitrile gradient with 0.1% formic acid; ESP + mode). IC50’s were determined using GraphPad’s Prism v 5.04 nonlinear curve fitting program.
Results
The in vitro antifungal activity of our of (2S, 4R)-Ketoconazole sulfonamide analogs (3a) –(3l) against C. albicans and C. glabrata is described in Table 1. Replacing the acetamide of (2S, 4R)-Ketoconazole (4) with small, linear suflonamides (3a-3c) produced compounds whose in vitro potency against the test fungi was maintained (C. albicans MIC75 = 125 nM, C. glabrata MIC75 = 500 nM). Insertion of a branch point as seen in the isopropyl analog (3d), however, led to a complete loss of in vitro antifungal activity. Constraining the branch point through the formation of the corresponding cyclopropane ring (3e) restore in vitro antifungal activity against both species (C. albicans MIC75 = 800 nM, C. glabrata MIC75 = 800 nM), but not to the levels observed in (3a) –(3c). Insertion of an additional methylene unit between the cyclopropane ring and the sulfonamide moiety (3f) produced a significant loss in vitro activity against C. glabrata (MIC75 = 25,000 nM), but activity against C. albicans (MIC75 = 800 nM) was unchanged in comparison to (3d).
The addition of polar groups to the sulfonamide moiety produced mixed results. Appending a cyano group on the sulfonamide moiety (3g) provided a compound with in vitro antifungal activity comparable to that of (±)-Ketoconazole (2) (C. albicans MIC75 = 250 nM, C. glabrata MIC75 = 500 nM), but adding a methyl sulphone unit (3h) caused in vitro antifungal activity to drop substantially (C. albicans MIC75 = 800 nM, C. glabrata MIC75 = 6250 nM).
Fluorination of the small, linear sulfonamides produced some surprising results. The CF3 analog (3i) was less potent (C. albicans MIC75 = 800 nM, C. glabrata MIC75 = 1600 nM) than the parent of series (3a). Similar results were observed when the ethyl sulfonamide and n-propyl sulfonamide where capped with a CF3 moiety (3j C. albicans MIC75 = 1600 nM, C. glabrata MIC75 = 800 nM, 3k C. albicans MIC75 = 1600 nM, C. glabrata no effect). Interestingly, capping the ethyl sulfonamide with a difluoro unit (3l), however, produced a significant increase in vitro antifungal activity against both species examined (C. albicans MIC75 = 62 nM, C. glabrata MIC75 = 250 nM). Importantly, the in vitro antifungal activity of (3l) exceeded that of both Amphotericin B and (±)-Ketoconazole against C. albicans (MIC75 = 250 nM) and matched their activity against C. glabrata (MIC75 = 250 nM).
We also determined the capacity of our compounds to inhibit human Cyp3A4. As noted above, the use of (±)-Ketoconazole (2) was heavily restricted in 2013 based in part on its ability to cause drug/drug interaction via inhibition of this key metabolic enzyme (FDA Website, 2022). In our hands, (±)-Ketoconazole (2) and (2S, 4R)-Ketoconazole (4) displayed potent Cyp3A4 inhibition (IC50 = 133 and 146 nM respectively). Our (2S, 4R)-Ketoconazole sulfonamide analogs (3a-3l) displayed moderate to potent Cyp3A4 inhibition (IC50 = 75.6–450 nM). The n-propyl analog (3c) was the most potent (IC50 = 75.6 nM), while the trifluoroethyl analog (3j) was the least potent of the set (IC50 = 450 nM).
Discussion
As noted above, C. albicans and C. glabrata are responsible for a large portion of Candida infection in the US and represent a serious health threat (US CDC website, 2022a). Blood stream infection of either of these organisms are associated with high mortality (Timmermans et al., 2018). Immunocompromised patients are at an even greater risk should they be unfortunate enough to experience infections with C. albicans, C. glabrata, or other candida species (Monk et al., 2020). In addition, the rise of drug resistant fungi (Sanglard et al., 1999; Vermitsky and Edlind, 2004; Chau et al., 2006) suggests that the current staple of antifungal agents may eventually be insufficient to meet patient needs. Similar to antibiotics and antimicrobial agents, the appearance of clinically relevant drug resistant fungi has been driven by prolonged use of antifungal agent in clinical setting as well as in the agricultural sector (Snelders et al., 2008; Snelders et al. 2011; Snelders et al. 2015).
Our studies focused on the further exploration of the chemical space surrounding (±)-Ketoconazole (2) began with the observation that the initial disclosure of this antifungal agent (Beckx 1979) described only a handful of analogs. This initial disclosure predates the advent of both high through put chemistry and high throughput screening, which may explain the limited nature of the original disclosure. Surprisingly, we found that the literature contained very few additional disclosures of (±)-Ketoconazole analogs, and we viewed this as an opportunity to explore the chemical space surrounding this antifungal agent. Specifically, we have demonstrated that the acetamide of (2S, 4R)-Ketoconazole (4) can be replaced with sulfonamides (3a-3l) to produce compounds that have in vitro antifungal properties with respect to C. albicans and C. glabrata. We have also found that similar to (±)-Ketoconazole (2), these compounds inhibit human Cyp3A4 (IC50 = 75.6–450 nM), a key metabolic enzyme that has been linked to drug/drug interactions (Molenaar-Kuijsten et al., 2021). Interestingly, we noted a divergence between antifungal activity and Cyp3A4 inhibition, Specifically, we observed that our most potent in vitro antifungal agent (3l) demonstrated a wider window between Cyp3A4 inhibition and its activity against C. albicans than (±)-Ketoconazole (2) (2.6-fold versus 0.5-fold). This 5-fold improvement in selectivity suggests that it may be possible to identify analogs with a wide enough separation to avoid the risk of Cyp3A4 mediated drug/drug interaction. Additional studies will be required to determine the true potential of this opportunity.
Data availability statement
The original contributions presented in the study are included in the article/Supplementary material, further inquiries can be directed to the corresponding authors.
Author contributions
BB was responsible for designing the compounds, developing the necessary synthetic methods and preparing some of the (2S, 4R)-ketoconazole sulfonamide analogs. He also co-mentored BD as he prepared additional compounds. SP. oversaw the effort of RS as he executed the in vitro antifungal assay described herein. RS executed the in vitro antifungal assays described herein. BD was responsible for preparing some of the (2S, 4R)-ketoconazole sulfonamide analogs.
Funding
Funding for the studies described herein was provided by R01DE030130 (SP), funded by the National Institute of Dental and Craniofacial Research, National Institutes of Health, Temple University School of Pharmacy and Temple University’s Kornberg School of Dentistry.
Conflict of interest
The authors declare that the research was conducted in the absence of any commercial or financial relationships that could be construed as a potential conflict of interest.
Publisher’s note
All claims expressed in this article are solely those of the authors and do not necessarily represent those of their affiliated organizations, or those of the publisher, the editors and the reviewers. Any product that may be evaluated in this article, or claim that may be made by its manufacturer, is not guaranteed or endorsed by the publisher.
References
Backx, L. J. J., Heeres, J., and Mostmans, J. H. (1979).Dioxolan-2-ylmethyl)-1H-1, 2, 4-triazoles, US patent application US76426377AU.S. Pat. Trademark Office, 1. 3.
Blass, B. E., Iyer, P., Abou-Gharbia, M., Childers, W. E., Gordon, J. C., Ramanjulu, M., et al. (2016). Design, synthesis, and evaluation of (2S, 4R)-Ketoconazole sulfonamide analogs as potential treatments for Metabolic Syndrome. Bioorg. Med. Chem. Lett. 26 (23), 5825–5829. doi:10.1016/j.bmcl.2016.10.016
Bongomin, F., Gago, S., Oladele, R. O., and Denning, D. W. (2017). Global and multi-national prevalence of fungal diseases-estimate precision. J. Fungi 3, 57. doi:10.3390/jof3040057
Chau, A. S., Chen, G., McNicholas, P. M., and Mann, P. A. (2006). Molecular basis for enhanced activity of posaconazole against Absidia corymbifera and Rhizopus oryzae. Antimicrob. Agents Chemother. 50, 3917–3919. doi:10.1128/AAC.00747-06
Clinical and Laboratory Standards Institute (CLSI) (2017). “Reference method for broth dilution antifungal susceptibility testing of yeasts,” in Approved standard. fourth edition (Wayne, PA: CLSI document M27- A4).
FDA websie, (2022) FDA websie. Available at: https://www.fda.gov/drugs/drug-safety-and-availability/fda-drug-safety-communication-fda-limits-usage-nizoral-ketoconazole-oral-tablets-due-potentially, [Accessed June 27 2022].
Griffith, R. K. (2020).Drug used to treat fungal infection” in Foye’s Principles of Medicinal Chemistry. Editors V. F. Roche, S. W. Zito, T. L. Lemke, and D. A. Williams. 8th edition (Netherlands: Walter Kluwer), 1260–1275.
Kolaczkowska, A., and Kolaczkowski, M. (2016). Drug resistance mechanisms and their regulation in non-albicans Candida species. J. Antimicrob. Chemother. 71 (6), 1438–1450. doi:10.1093/jac/dkv445
Maertens, J. A. (2004). History of the development of azole derivatives. Clin. Microbiol. Infect. 10 (1), 1–10. doi:10.1111/j.1470-9465.2004.00841.x
Magill, S. S., O’Leary, E., Janelle, S. J., Thompson, D. L., Dumyati, G., Nadle, J., et al. (2018). Changes in prevalence of health care-associated infections in U.S. hospitals. N. Engl. J. Med. 379, 1732–1744. doi:10.1056/NEJMoa1801550
McCarty, T. P., and Pappas, P. G. (2016). Invasive candidiasis. Infect. Dis. Clin. North Am. 30, 103–124. doi:10.1016/j.idc.2015.10.013
Molenaar-Kuijsten, L., Van Balen, D. E. M., Beijnen, J. H., Steeghs, N., and Huitema, A. D. R. (2021). A review of CYP3A drug-drug interaction studies: Practical guidelines for patients using targeted oral anticancer drugs. Front. Pharmacol. 12, 670862. doi:10.3389/fphar.2021.670862
Monk, B. C., Sagatova, A. A., Hosseini, P., Ruma, Y. N., Wilson, R. K., and Keniya, M. V. (2020). Fungal lanosterol 14α-demethylase: A target for next-generation antifungal design. Biochim. Biophys. Acta. Proteins Proteom. 3, 140206. doi:10.1016/j.bbapap.2019.02.008
Pfaller, M. A., and Diekema, D. (2007). Epidemiology of invasive candidiasis: A persistent public health problem. Clin. Microbiol. Rev. 20, 133–163. doi:10.1128/CMR.00029-06
Roemer, T., and Krysan, D. J. (2014). Antifungal drug development: Challenges, unmet clinical needs, and new approaches. Cold Spring Harb. Perspect. Med. 4 (5), a019703. doi:10.1101/cshperspect.a019703
Rotstein, D. M., Kertesz, D. J., Walker, K. A., and Swinney, D. C. (1992). Stereoisomers of ketoconazole: Preparation and biological activity. J. Med. Chem. 35 (15), 2818–2825. doi:10.1021/jm00093a015
Sanglard, D., Ischer, F., Calabrese, D., Majcherczyk, P. A., and Bille, J. (1999) The ATP binding cassette transporter gene CgCDR1 from Candida glabrata is involved in the resistance of clinical isolates to azole antifungal agents, Antimicrob. Agents Chemother. 43, 2753, doi:10.1128/AAC.43.11.2753
Snelders, E., Camps, S. M., Karawajczyk, A., Rijs, A. J., Zoll, J., Verweij, P. E., et al. (2015). Genotype-phenotype complexity of the TR46/Y121F/T289A cyp51A azole resistance mechanism in Aspergillus fumigatus. Fungal Genet. Biol. 82, 129–135. doi:10.1016/j.fgb.2015.06.001
Snelders, E., Karawajczyk, A., Verhoeven, R. J., Venselaar, H., Schaftenaar, G., Verweij, P. E., et al. (2011). The structure-function relationship of the Aspergillus fumigatus cyp51A L98H conversion by site-directed mutagenesis: The mechanism of L98H azole resistance. Fungal Genet. Biol. 48, 1062–1070. doi:10.1016/j.fgb.2011.08.002
Snelders, E., van der Lee, H. A., Kuijpers, J., Rijs, A. J., Varga, J., Samson, R. A., et al. (2008). Emergence of azole resistance in Aspergillus fumigatus and spread of a single resistance mechanism. PLoS Med. 5, e219. doi:10.1371/journal.pmed.0050219
Timmermans, B., De Las Peñas, A., Castaño, I., and Van Dijck, P. (2018). Adhesins in Candida glabrata. J. Fungi 4, 60. doi:10.3390/jof4020060
Tsay, S., Williams, S., Mu, Y., Epson, E., Johnston, H., Farley, M. M., et al. (2018). 363. National burden of candidemia, United States, 2017. Open Forum Infect. Dis. 5, S142–S143. doi:10.1093/ofid/ofy210.374
US CDC website. US CDC website (2022a) Available at: https://www.cdc.gov/hai/eip/candida.html [Accessed June 27 2022].
US CDC website US CDC website (2022b) Available at: https://www.cdc.gov/fungal/diseases/candidiasis/invasive/statistics.html [Accessed June 27 2022].
Vallabhaneni, S., Cleveland, A. A., Farley, M. M., Harrison, L. H., Schaffner, W., Beldavs, Z. G., et al. (2015). Epidemiology and risk factors for echinocandin nonsusceptible Candida glabrata bloodstream infections: Data from a large multisite population-based candidemia surveillance program, 2008-2014. Open Forum Infect. Dis. 2 (4), ofv163. doi:10.1093/ofid/ofv163
Keywords: antifungal agent, antimicrobial agents, ketoconazole, Candida albicans, Candida glabrata
Citation: Blass BE, Puri S, Sharma R and Day BM (2022) Antifungal properties of (2S, 4R)-Ketoconazole sulfonamide analogs. Front. Drug. Discov. 2:1000827. doi: 10.3389/fddsv.2022.1000827
Received: 22 July 2022; Accepted: 08 August 2022;
Published: 29 August 2022.
Edited by:
Afzal Basha Shaik, Jawaharlal Nehru Technological University, IndiaReviewed by:
Suraj Narayan Mali, Institute of Chemical Technology, IndiaAdil Shareef Mohammed, Post-Doctoral Research Scientist, United States
Copyright © 2022 Blass, Puri, Sharma and Day. This is an open-access article distributed under the terms of the Creative Commons Attribution License (CC BY). The use, distribution or reproduction in other forums is permitted, provided the original author(s) and the copyright owner(s) are credited and that the original publication in this journal is cited, in accordance with accepted academic practice. No use, distribution or reproduction is permitted which does not comply with these terms.
*Correspondence: Benjamin E. Blass, QmVuamFtaW4uQmxhc3NAVGVtcGxlLmVkdQ==; Sumant Puri, c3VtYW50cHVyaUB0ZW1wbGUuZWR1