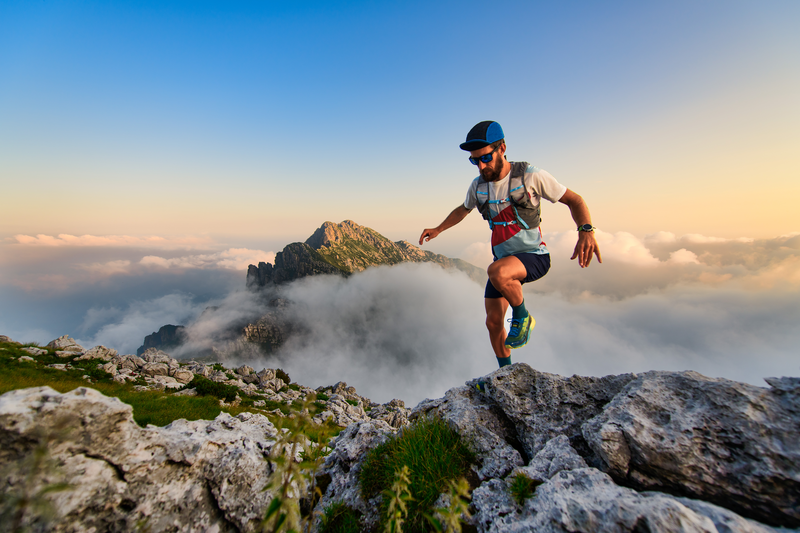
95% of researchers rate our articles as excellent or good
Learn more about the work of our research integrity team to safeguard the quality of each article we publish.
Find out more
MINI REVIEW article
Front. Drug Deliv. , 25 July 2022
Sec. CNS Drug Delivery
Volume 2 - 2022 | https://doi.org/10.3389/fddev.2022.931221
This article is part of the Research Topic Impact of Various Diseases on Brain Barrier Function and Central Nervous System Drug Delivery View all 4 articles
Astronauts have flown to space for decades, but the effects of spaceflight on human health have not been fully clarified yet. Several pathologies have only been detected after it has become customary for astronauts to spend months rather than days in space and with the advance of inflight monitoring. Examples include the neuro-ocular spaceflight associated syndrome, changes to the brain’s white matter, and, more recently, altered cerebral blood flow and related hypercoagulability. This review outlines spaceflight-induced brain disorders in astronauts and putative contributing factors. It next presents ongoing and upcoming studies of the BBB onboard space platforms. Finally, it describes how the space environment can be harnessed for improving drug-delivery across the BBB for humans both in space and on Earth.
Space is considered to be the last frontier of human health and a contributor to medical innovation (Alwood et al., 2017; Cinelli and Russomano, 2021). Important environmental factors endured by space travelers include microgravity, exposure to space radiation, hostile or close environments, isolation, confinement, and distance from Earth (Patel et al., 2020). These conditions affect virtually every system in the human body, including the brain and the vasculature. Risk depends on the type and the duration of flight, and is expected to be considerably higher during missions beyond Low Earth Orbit (LEO) (Eyal and Derendorf, 2019; Stepanek et al., 2019). Two key features of the spaceflight-associated physiological changes are particularly relevant for medical research and development: accelerated aging and disease processes, and their reversibility (or lack thereof) upon return to Earth. At the molecular and cellular level, the microgravity of space can alter the physico-chemical properties of protein crystals, membranes and cells. Such alterations may be harnessed for better understanding of the structure of BBB receptors and therapeutic proteins, engineering of 3D constructs of the neurovascular unit, and designing new drug formulations (Amselem, 2019; Ryder et al., 2020).
Blood-brain interfaces (the BBB and the blood-cerebrospinal fluid barrier, BCSFB) maintain brain homeostasis by controlling the influx and efflux of compounds and cells from the brain. Within the neurovascular unit, microvascular endothelial cells form the major barrier between the circulation and the brain. The microvascular endothelial cells of the brain are non-fenestrated and are sealed together with tight-junction proteins. Uptake transporters and membrane receptors enable the cerebral uptake of essential compounds and medications whereas efflux transporters protect the brain against potentially noxious compounds. Enveloping pericytes and astrocytic endfeet aid in maintaining the BBB integrity and function. Neuronal input and demand further interact with those cells and regulate the cerebral capillary network (Eyal et al., 2009; Han et al., 2017; Lochhead et al., 2020).
Due to the unique features of the BBB, drug delivery for the treatment of neurological diseases and brain disorders remains a major challenge. The BBB prevents the brain uptake of most drugs, with the exception of small hydrophilic compounds with a mass lower than 150 Da and highly hydrophobic compounds with a mass lower than 400–600 Da that can cross the BBB by passive diffusion (Santaguida et al., 2006; Cecchelli et al., 2007; Morofuji and Nakagawa, 2020). Hence, drug treatment of neurological diseases such as Parkinson’s and Alzheimer’s diseases, or their delivery to cerebral sanctuaries of tumor cells and viruses, is challenging. Accordingly, finding new approaches for drug delivery across the BBB is crucial, particularly for macromolecules such as monoclonal antibodies (mAb) (Santaguida et al., 2006; Banks, 2016; McInerney et al., 2017; Han, 2021; Omidi et al., 2021; Parakh et al., 2021; Terstappen et al., 2021; Pan and Nicolazzo, 2022). Current approaches are aimed at increasing paracellular and transcellular BBB permeability, e.g., by opening of tight junctions, enhancing transcytosis, and inhibition of active efflux (Han, 2021). In this context, the spaceflight environment can be disruptive, offering yet unexplored opportunities to improve drug delivery to the brain.
Spaceflights, beginning with the short-duration Apollo era missions, were associated with decrements in operational capabilities, including altered driving performance. Changes were recorded across neurological domains, including cognition, sensation, movement, and coordination (Roy-O'Reilly et al., 2021). More recently, the NASA Twins Study demonstrated significantly reduced cognitive performance after 340 days of spaceflight as compared to preflight values (Garrett-Bakelman et al., 2019). The reports on reduced performance after long space missions have been supported by findings from neuroimaging studies, which provided evidence for alterations in the structure and positioning of the brain and in its ventricles (Roy-O'Reilly et al., 2021). Changes were observed in the CSF volume, along with ventricular expansion and upward shifts of the brain (Roberts et al., 2017; Van Ombergen et al., 2019; Jillings et al., 2020). For instance, one magnetic resonance imaging (MRI) study in 11 cosmonauts whose average mission duration was 169 days identified mean increases of 13.3 and 10.4% in the volumes of the lateral and the third ventricles, respectively (Van Ombergen et al., 2019). Cerebral fluid accumulation might be involved in the etiology of cerebral disorders in space. For example, locally elevated CSF sheath pressures in the orbital subarachnoid space have been linked to the ocular changes that occur during prolonged spaceflight, known as spaceflight-associated neuro-ocular syndrome (SANS) (Lee et al., 2017). Other findings include decreases in frontal and temporal gray matter volumes and changes in white matter volume (Koppelmans et al., 2016; Roberts et al., 2017; Van Ombergen et al., 2018; Van Ombergen et al., 2019; Jillings et al., 2020). Periventricular white matter hyperintensities (WMH), observed in astronauts after prolonged spaceflight (Alperin et al., 2017), have been associated with declines in cognitive and motor performance in healthy aging (Vernooij et al., 2009; Seidler et al., 2010). The rate of spaceflight-associated white matter changes was estimated at 1.5–2.5% per year, approximately 2-fold faster than that in a large subject sample with a mean age of 50 years (Lee et al., 2019). However, in astronauts these changes were limited to regions near the ventricles and partially reversed by 1 month after landing (Alperin et al., 2017). The urgent need in better understanding of the physiology of the brain barriers in space is arguably best demonstrated by recent event of blood clot in the internal jugular vein of an astronaut in space (Auñón-Chancellor et al., 2020) along with jugular venous blood flow stasis in additional astronauts (Marshall-Goebel et al., 2019). The next sections will describe the effects of individual factors encountered in spaceflight on the BBB, followed by presenting the current knowledge obtained from real spaceflights.
The vasculature is particularly susceptible to radiation (Chancellor et al., 2014), and irradiation of the brain has long been shown to cause damage to the neurovascular unit. For instance, in patients with supratentorial glioblastoma radiation enhanced the accumulation of a gadolinium (Gd)-based contrast agent in the brain parenchyma, likely by damaging the BBB (Lim et al., 2018). In mice, irradiation with doses of 5–200 Gy decreased the endothelial cell number by up to 15% compared with the pre-treatment values (Ljubimova et al., 1991). In rats, gamma radiation (60 Gy) caused leakage of horse-radish peroxidase, with severe loss of the capillary network (Rubin et al., 1994). A lower dose (4.5 Gy) increased the extravasation of [3H]alpha-aminoisobutyric acid and [14C]sucrose (Diserbo et al., 2002). Treating mice with an anti-tumor necrosis factor (TNF) monoclonal antibody was associated with attenuated radiation-induced BBB dysfunction, astrocyte activation, and leukocyte adhesion suggesting a role for TNF in these processes (Wilson et al., 2009). The exposure in those studies overestimate the radiation dose of most space missions. For instance, Mercury and Gemini crews were exposed over their missions to a total dose in the range of 0.05–2.31 mGy (except for Gemini X where the total dose was 6.18–7.79 mGy), Apollo XIV astronauts received ∼11 mGy, and Mir 01–23 cosmonauts were exposed to up to 93 mGy (Maalouf et al., 2011). The predicted average galactic cosmic rays (GCR) absorbed dose rate during a mission to Mars is 0.45 mGy/day (Simonsen and Zeitlin, 2017). A similar phenomenon on missions outside of LEO might yield dose rates of up to 100 mGy/h and 500 mGy/h inside a space vehicle and during an extravehicular activity, respectively (Chancellor et al., 2014).
Radiation leads to cellular damage by both direct and indirect pathways. Direct pathways involve ionization of DNA, RNA, proteins and lipids. Indirect damage results from generation of reactive oxygen species (Fauquette et al., 2012; Chancellor et al., 2021). Radiation damage can lead to necrosis, apoptosis, autophagy, or senescence (Allen and Limoli, 2022). Specifically in brain capillary endothelial cells, radiation resulted in reduced expression of the tight junctional protein zonula occludens (ZO)-1 (Fauquette et al., 2012), formation of actin stress fibers (Fauquette et al., 2012), and increased lipid peroxidation, in association with glutathione depletion (Mertsch et al., 2001). In addition, a post-mortem analysis of normal tissue from patients treated with radiation therapy for glioblastoma demonstrated depletion of pericytes in necrotic tissue even in the normal brain, despite the absence of morphological changes to the vasculature (Lee et al., 2018). In mice, heavy-ion irradiation of the brain activated microglia and induced inflammation in the hippocampus (Encinas et al., 2008) and the dentate subgranular zone (Rola et al., 2005), although the involvement of the BBB in the inflammatory processes has not been reported.
The mean levels of carbon dioxide on the International Space Station (ISS) can be approximately ten-fold than those in Earth’s atmosphere (3.4 mmHg vs 0.3 mm Hg at standard pressure). Hypercapnia elevates cerebral blood flow and intracranial pressure, with increased risk of headache for every 1 mmHg increase in carbon dioxide levels (Law et al., 2014). More than 60 years ago, exposure of rabbits to 10% carbon dioxide in oxygen has been shown to cause vascular damage and increased the cerebral permeability of trypan blue (Clemedson et al., 1956). Later studies demonstrated hypercapnia-induced increases in the permeability of the BBB to other molecules, including lactate (Knudsen et al., 1991) and albumin (Cutler and Barlow, 1966; Hochwald et al., 1973). More recently, studies in rodents demonstrated that hypercapnia exacerbates hypoxemia-induced increases in BBB permeability (Liu et al., 2020) and reduces the expression of the tight junctional proteins zonula occludens (ZO)-1, occludin, and claudin-5 (Ding et al., 2020).
While there is a vast literature on spaceflight-induced alterations in endothelial cells of non-cerebral origin, much less in know about endothelial cells that consist the BBB. Many in vitro studies applied simulated microgravity to model the physiological changes that occur in space, although there is a gap between the levels of gravity obtained during simulation and the microgravity of space (10−2–10−3 g for ground simulators and parabolic flights, versus 10−5–10−6 onboard the ISS). Accordingly, these studies usually mimic many, but not all the physiological changes that occur in space (Amselem, 2019). Briefly, true and simulated weightlessness conditions caused dysregulation of motility and adhesion to substrates of endothelial cells which were isolated from peripheral blood vessels, e.g., the aorta and umbilical veins (Infanger et al., 2006; Grimm et al., 2009). Other changes involved the cytoskeleton, extracellular matrix, mitochondrial distribution, angiogenic response, apoptosis, cell growth, and cell cycle regulation (da Silveira et al., 2020; Dittrich et al., 2018; Maier et al., 2015; Morbidelli et al., 2005; Barravecchia et al., 2021; Crawford-Young, 2006; Grenon et al., 2013; Janmaleki et al., 2016; Kapitonova et al., 2013; Kapitonova et al., 2012; Wehland et al., 2013). In human umbilical vein endothelial cells (HUVEC), microgravity increased the permeability to fluorescein isothiocyanate (FITC)-tagged dextran (Shi et al., 2022) and promoted activation of inflammatory reactions with a shift towards senescence (Versari et al., 2013).
A modeling analysis which was published in 2007 predicted that higher steady-state intracranial pressure, together with reduced blood colloid osmotic pressure, would reduce BBB integrity (Lakin et al., 2007). Studies which tested this hypothesis were published only a decade later. Bellone et al. evaluated cerebral effects of simulated gravity (by tail suspension), chronic exposure to low-dose gamma radiation (a total dose of 0.04 Gy), or a combination of both (Bellone et al., 2016). After 3 weeks of simulated microgravity, but not gamma radiation, mice displayed increased exploratory and risk-taking behavior as compared to controls without differences in the outcomes of cognitive tests. The combination of simulated microgravity and radiation, but not each factor alone, was associated with a significant change in aquaporin-4 (AQP4), a water channel protein concentrated at perivascular astrocyte membranes. AQP4 levels, which are elevated when the BBB is compromised, are critically involved in the formation and dissolution of cerebral edema (Amiry-Moghaddam et al., 2003; Frydenlund et al., 2006), suggesting a role for BBB dysfunction in the observed behavioral changes. In another rat study, 21 days of tail suspension resulted in inflammatory cellular infiltration and nuclear pyknosis in the cortex (Yan et al., 2021). Transmission electron microscopy demonstrated a widened intercellular space of endothelial cells, swollen pericytes, and unclear mitochondria cristae. Expression of the tight junction proteins claudin-5, VE-cadherin, and β-catenin decreased by half or more, without a change in occludin and ZO-1 expression. A proteomic analysis discovered 554 differentially expressed proteins, which were mainly enriched in those regulating the cell-cell junction and cell-extracellular matrix biological pathways. Simulated microgravity additionally induced apoptosis and oxidative stress injury, as well as changes in actin cytoskeleton, which is important for cell adhesion. Compared to the control group, the content of Evans blue and Texas red-dextran in the brain was significantly increased, by 31% and 37.2%, respectively, indicating higher BBB permeability. The study also demonstrated that the Ras-related C3 botulinum toxin substrate 1 (Rac1)/Wiskott-Aldrich syndrome protein family verprolin-homologous protein 2 (Wave2)/actin-related protein 3 (Arp3) signaling pathway could be an important contributor to the observed BBB disruption under simulated microgravity.
Taken together, these studies support disorders of cerebral small vessels as one mechanism that could explain the above-mentioned WMH observed in astronauts. On Earth, dynamic contrast-enhanced (DCE) MRI study in 201 patients found that BBB leakage and interstitial fluid volume were higher in WMH than normal-appearing white matter, and that BBB leakage in WMH predicted declining cognition at 1 year (Wardlaw et al., 2017). In another DCE–MRI study, the leakage volume of the WMH was significantly larger in patients with cerebral small vessel disease compared with controls. The authors suggested that in those patients, subtle BBB leakage and extravasation of blood components may cause brain tissue damage and exacerbate local vascular changes (Zhang et al., 2017). More recently, BBB leakage at baseline in patients with cerebral small vessel disease was associated with a change in parenchymal diffusivity (a quantitative marker of microstructural tissue condition) in proximity of the WMH. That is, BBB impairment might play an early role in subsequent white matter degeneration (Kerkhofs et al., 2021).
In contrast to microgravity, the hypergravity experienced by space travelers is transient (during launch and, to a greater extent, during return to Earth). In mice, centrifugation at 2 g for 1 or 50 days, but not short exposure to hypergravity (5 g, as during landing) resulted in immunoglobulin G extravasation into the hippocampal parenchyma. These findings imply that the duration of hypergravity might be more important than its intensity. The authors suggested that centrifugation may serve as means for opening the BBB, although this is less likely to occur in the clinical setting (Dubayle et al., 2020).
A ground-based spaceflight model is parabolic flight, which yields alternating brief periods of microgravity as described above and hypergravity (1.8 g). In volunteers who participated in such a flight, blood flow in the vertebral artery increased during gravitational transitions from micro-to hypergravity, along with reduction in nitric oxide (indicating elevated free radicals) and increased glio-vascular glial fibrillary acidic protein (GFAP) and S100B. The latter is a 10 kDa protein expressed in glia and Schwann cells whose plasma levels may be elevated after trauma without brain injury (Hier et al., 2021). No change was observed in biomarkers of neuronal-axonal damage (neuron-specific enolase, neurofilament light-chain -NFL, ubiquitin carboxy-terminal hydrolase L1 and tau). These findings provided a first indication for the presence of cerebral markers in systemic circulation as an outcome of gravitational transitions, indicating minor BBB damage. The suggested etiology was hyperperfusion, together with oxidative stress (Bailey et al., 2020).
Silvani et al. (2021) developed an innovative hybrid in vitro vascularized glioblastoma-on-a-chip model. Under simulated microgravity conditions, the system demonstrated a significant cell morphological and mechanotransduction response, representing a tool for investigating cancer mechanobiology and the blood-tumor barrier.
Only one published study evaluated spaceflight-associated BBB changes in a preclinical model in real microgravity (Mao et al., 2020). In that study, 35 days of spaceflight increased the expression of hippocampal AQP4 in the mouse brain, supporting the above-mentioned combined effect of microgravity and radiation in mice (Bellone et al., 2016). Other changes included increased expression of platelet endothelial cell adhesion molecule-1 (PECAM-1) and decreased ZO-1 expression, indicating a disturbance of BBB integrity. The proposed contributors to these changes were chronic mild inflammation and oxidative damage.
A pilot study tracked the concentrations in plasma of brain-specific proteins before and after spaceflight. The study was conducted in 5 male cosmonauts (mean age, 49.2 years) who spent almost a year in space. Blood samples were collected 20 days before launch and post-flight (1 day, 1 week, and 21–25 days after landing). The samples were analyzed by a single-molecule array immunoassay for five biomarkers of brain damage: NFL, GFAP, total tau, and two amyloid-beta proteins. Plasma levels of three of the biomarkers—NFL, GFAP and the amyloid beta protein Aß40—were significantly higher after the cosmonauts returned from the ISS, partially overlapping with the results obtained following a parabolic flight (Bailey et al., 2020). The timing of peak values varied across individuals, but the trends of elevated biomarkers were consistent across the five participants. High levels of these biomarkers have been associated with potential axonal disintegration process (NFL) and astrocytic activation (GFAP), suggesting that different components of the brain parenchyma are affected by microgravity (zu Eulenburg et al., 2021). However, the changes in brain function could not be distinguished from BBB disruption during spaceflight or landing. It is hoped the future investigations will clarify the cause for the elevated brain markers in plasma.
The last mission to the ISS investigating the behavior of human BBB in space so far was launched in April 2022 (AXIOM-1), as a part of Rakia mission (Ramon Foundation and Israel Space Agency). Among the experiments performed by Israel’s second space traveler, Eytan Stibbe, was a study led by Dr. Itzik Cooper which analyzes the effects of microgravity on vascular function in the brain (Table 1).
TABLE 1. Blood-brain barrier-associated experiments conducted onboard the International Space Station.
Krishnamurthy and colleagues suggested that altered activity of transporters at the BCSFB with subsequent increase in osmotic load of the cerebrospinal fluid may contribute to the spaceflight-associated hydrocephalus (Krishnamurthy et al., 2021). However, given the absence of information on transporter activity at blood-brain barriers in space, this has yet to be investigated. Data on these transporters are urgently required, because transport across brain barriers not only modifies osmotic load, but also controls the distribution of essential compounds, drugs and other xenobiotics between blood, brain, and the CSF. For instance, downregulation of BBB uptake carriers for glucose and amino acids may deprive the brain of these compounds. On the other hand, reduced functionality of the efflux transporters P-glycoprotein (P-gp) and the breast cancer resistance protein (BCRP) at the BBB can enhance the cerebral distribution of substrate drugs, thus increasing their therapeutic effects, CNS toxicity, or both (Lee et al., 2001; Eyal et al., 2009; Nicolazzo and Katneni, 2009; Han et al., 2017). Examples of P-gp substrate medications which are available onboard the ISS include non-sedating antihistamines, which might become sedative in the absence of effective P-gp-mediated efflux, and calcium channel blockers (Eyal and Derendorf, 2019; Eyal, 2020).
Because spaceflight accelerates aging and tissue degeneration, treatments for preventing microgravity-induced pathophysiological changes have been adapted to diminish age-dependent diseases. One example is the development of the drug denosumab, aimed to prevent loss of bone mass, whose development gained from findings in rodents flown onboard the ISS (Amselem, 2019). Similarly, the space conditions may potentially unveil novel BBB pathways which may be targeted by drug delivery platforms. A study by the pharmaceutical company Emulate conducted on the ISS using an Organ-on-Chip approach (PI: Dr. Chris Hinojosa) analyzed the effect of space-related stressors on the BBB. The brain-chip consisted of neurons and vascular endothelial cells in a micro-engineered environment (Keeter, 2018) (Table 1). The results are hoped to provide insight into the relationship between inflammation and brain function, as well as the effectiveness of anti-inflammatory drugs in maintaining BBB integrity, for a better understanding of neurodegenerative diseases. Emulate also participated in a more recent SpaceX CRS-24 mission to examine the effects of microgravity on the BBB (Table 1).
Three-dimensional cell cultures in microgravity yield improved 3D structures and allow for a more precise appreciation of the role the biophysical constraints play in shaping cell phenotypes and functions (Amselem, 2019). Therefore, understanding microgravity effects can help improve tissue-engineering techniques on Earth. For instance, under simulated weightlessness conditions (but not at 1 g), endothelial EA.hy926 cells formed tube-like structures that resemble vascular intimas, even without scaffolds (Infanger et al., 2006; Grimm et al., 2009). Similarly, the lack of convection and sedimentation in space improved the quality of protein crystals (Giulianotti and Low, 2019), allowing better understanding of the 3D structure of BBB-traversing mAbs (or other proteins) and mAb-receptor interactions (e.g., mAb interactions with the transferrin receptor) (Amselem, 2019; Giulianotti and Low, 2019; Mullard, 2021). This could be utilized to improve drug delivery across the BBB. The use of spaceflight for developing nanofornulations has been recently reviewed and is beyond the scope of this manuscript (Grover et al., 2020).
A limitation inherent to most spaceflight studies is the small number of patients or samples. On the other hand, ground-based simulations do not capture all aspects of the stressor encountered in space. One example is the above-mentioned tail-suspension test which maintains the pressure of visceral organs on vasculature along with several other dissimilarities to the space environment. A major limitation of many radiation studies is the use of mono-energetic radiation sources, particularly gamma-exposure, which are dissimilar to radiation exposures for astronaut crews. In addition, all the above mentioned spaceflight studies were conducted in LEO. Thus, it is difficult to estimate how and to what extent space radiation would affect human tissues in deep space, beyond the protective shield of the Van Allen radiation belts (Chancellor et al., 2018; Chancellor et al., 2021). For instance, if intense space radiation increases the penetration of blood-borne proteins into the brain, the results may be seizures. Current and future research platforms are being further developed to improve researcher accessibility to space thus overcoming the limitations associated with ground simulations. These include privately-owned space stations, satellites carrying remote-controlled laboratories (e.g., SpacePharma’s DIDO satellites) and reusable uncrewed mini shuttles, such as the European Space Agency’s Space RIDER and Sierra Nevada Corporation’s Dream Chaser.
Spaceflight-induced BBB disruption may be associated with the penetration of harmful compounds into the brain and, consequently, potential brain damage. Although the same situation might enhance cerebral drug distribution in space travelers, enhancing both therapeutic and adverse cerebral drug effects, this assumption has yet to be tested. Clearly, any attempt to improve (or avoid) drug delivery across the BBB during spaceflight should consider other physiological changes that may affect systemic pharmacokinetics and pharmacodynamics (Kast et al., 2017; Eyal and Derendorf, 2019; Eyal, 2020; Dello Russo et al., 2022). At the same time, spaceflight offers new opportunities for drug discovery and development. A major benefit of the space environment is finding new biological pathways and drug targets, given that endothelial cells are particularly sensitive to the lack of gravity and radiation.
By definition, treating diseases in astronauts in space implies repurposing, because it cannot be assumed that disease processes are identical on Earth and in space. The need to repurpose drugs for space applications may stimulate research that would yield benefits for patients with brain diseases on Earth. Another advantage is production of 3D tissue constructs of higher quality than under 1 g conditions. Such constructs of endothelial cells could be used for both research and repairment of impaired BBB in patients with cerebrovascular diseases. Moreover, protein crystallization in microgravity can help improve the design of novel antibody-based therapies which target the brain. Hence, the benefits of pharmaceutical research under spaceflight conditions extend well beyond treating astronauts in space; insights gained from such studies can help improve the pharmaceutical care of humans on Earth.
SE and SA conceived, wrote, and edited the manuscript.
The publication of this manuscript has been supported by SpacePharma.
SA is employed by SpacePharma R&D Israel LTD. SE is an external consultant to the company.
All claims expressed in this article are solely those of the authors and do not necessarily represent those of their affiliated organizations, or those of the publisher, the editors and the reviewers. Any product that may be evaluated in this article, or claim that may be made by its manufacturer, is not guaranteed or endorsed by the publisher.
Allen, B. D., and Limoli, C. L. (2022). Breaking Barriers: Neurodegenerative Repercussions of Radiotherapy Induced Damage on the Blood-Brain and Blood-Tumor Barrier. Free Radic. Biol. Med. 178, 189–201. doi:10.1016/j.freeradbiomed.2021.12.002
Alperin, N., Bagci, A. M., and Lee, S. H. (2017). Spaceflight-induced Changes in White Matter Hyperintensity Burden in Astronauts. Neurology 89, 2187–2191. doi:10.1212/wnl.0000000000004475
Alwood, J. S., Ronca, A. E., Mains, R. C., Shelhamer, M. J., Smith, J. D., and Goodwin, T. J. (2017). From the Bench to Exploration Medicine: NASA Life Sciences Translational Research for Human Exploration and Habitation Missions. NPJ Microgravity 3 (1), 5. doi:10.1038/s41526-016-0002-8
Amiry-Moghaddam, M., Otsuka, T., Hurn, P. D., Traystman, R. J., Haug, F.-M., Froehner, S. C., et al. (2003). An α-syntrophin-dependent Pool of AQP4 in Astroglial End-Feet Confers Bidirectional Water Flow between Blood and Brain. Proc. Natl. Acad. Sci. U.S.A. 100 (4), 2106–2111. doi:10.1073/pnas.0437946100
Amselem, S. (2019). Remote Controlled Autonomous Microgravity Lab Platforms for Drug Research in Space. Pharm. Res. 36, 183. doi:10.1007/s11095-019-2703-7
Auñón-Chancellor, S. M., Pattarini, J. M., Moll, S., and Sargsyan, A. (2020). Venous Thrombosis during Spaceflight. N. Engl. J. Med. 382 (1), 89–90. doi:10.1056/NEJMc1905875
Bailey, D. M., Lanéelle, D., Trihan, J.-E., Marchi, N., Stacey, B. S., Tamiya, K., et al. (2020). Gravitational Transitions Increase Posterior Cerebral Perfusion and Systemic Oxidative-Nitrosative Stress: Implications for Neurovascular Unit Integrity. Neuroscience 441, 142–160. doi:10.1016/j.neuroscience.2020.05.048
Banks, W. A. (2016). From Blood-Brain Barrier to Blood-Brain Interface: New Opportunities for CNS Drug Delivery. Nat. Rev. Drug Discov. 15 (4), 275–292. doi:10.1038/nrd.2015.21
Barravecchia, I., De Cesari, C., Forcato, M., Scebba, F., Pyankova, O. V., Bridger, J. M., et al. (2021). Microgravity and Space Radiation Inhibit Autophagy in Human Capillary Endothelial Cells, through Either Opposite or Synergistic Effects on Specific Molecular Pathways. Cell. Mol. Life Sci. 79 (1), 28. doi:10.1007/s00018-021-04025-z
Bellone, J. A., Gifford, P. S., Nishiyama, N. C., Hartman, R. E., and Mao, X. W. (2016). Long-term Effects of Simulated Microgravity And/or Chronic Exposure to Low-Dose Gamma Radiation on Behavior and Blood-Brain Barrier Integrity. NPJ Microgravity 2 (1), 16019. doi:10.1038/npjmgrav.2016.19
Cecchelli, R., Berezowski, V., Lundquist, S., Culot, M., Renftel, M., Dehouck, M.-P., et al. (2007). Modelling of the Blood-Brain Barrier in Drug Discovery and Development. Nat. Rev. Drug Discov. 6, 650–661. doi:10.1038/nrd2368
Chancellor, J. C., Blue, R. S., Cengel, K. A., Auñón-Chancellor, S. M., Rubins, K. H., Katzgraber, H. G., et al. (2018). Limitations in Predicting the Space Radiation Health Risk for Exploration Astronauts. NPJ Microgravity 4, 8. doi:10.1038/s41526-018-0043-2
Chancellor, J., Nowadly, C., Williams, J., Aunon-Chancellor, S., Chesal, M., Looper, J., et al. (2021). Everything You Wanted to Know about Space Radiation but Were Afraid to Ask. J. Environ. Sci. Health, Part C. 39 (2), 113–128. doi:10.1080/26896583.2021.1897273
Chancellor, J., Scott, G., and Sutton, J. (2014). Space Radiation: The Number One Risk to Astronaut Health beyond Low Earth Orbit. Life 4 (3), 491–510. doi:10.3390/life4030491
Cinelli, I., and Russomano, T. (2021). Advances in Space Medicine Applied to Pandemics on Earth. Space Sci. Technol. 2021, 9821480. doi:10.34133/2021/9821480
Clemedson, C.-J., Hartelius, H., and Holmberg, G. (1956). Effect of Carbon Dioxide Inhalation on the Blood-Brain Barrier. Nature 178 (4539), 915. doi:10.1038/178915a0
Crawford-Young, S. J. (2006). Effects of Microgravity on Cell Cytoskeleton and Embryogenesis. Int. J. Dev. Biol. 50 (2-3), 183–191. doi:10.1387/ijdb.052077sc
Cutler, R. W. P., and Barlow, C. F. (1966). The Effect of Hypercapnia on Brain Permeability to Protein. Archives Neurology 14 (1), 54–63. doi:10.1001/archneur.1966.00470070058007
da Silveira, W. A., Fazelinia, H., Rosenthal, S. B., Laiakis, E. C., Kim, M. S., Meydan, C., et al. (2020). Comprehensive Multi-Omics Analysis Reveals Mitochondrial Stress as a Central Biological Hub for Spaceflight Impact. Cell 183 (5), 1185–1201. doi:10.1016/j.cell.2020.11.002
Dello Russo, C., Bandiera, T., Monici, M., Surdo, L., Yip, V. L. M., Wotring, V., et al. (2022). Physiological Adaptations Affecting Drug Pharmacokinetics in Space: what Do We Really Know? A Critical Review of the Literature. Br. J. Pharmacol. 179(11):2538–2557. doi:10.1111/bph.15822
Ding, H., Liu, X., Li, X., Wen, M., Li, Y., Han, Y., et al. (2020). Hypercapnia Exacerbates the Disruption of the Blood-brain B-arrier by I-nducing I-nterleukin-1β O-verproduction in the B-lood of H-ypoxemic A-dult R-ats. Int. J. Mol. Med. 46 (2), 762–772. doi:10.3892/ijmm.2020.4604
Diserbo, M., Agin, A., Lamproglou, I., Mauris, J., Staali, F., Multon, E., et al. (2002). Blood-brain Barrier Permeability after Gamma Whole-Body Irradiation: an In Vivo Microdialysis Study. Can. J. Physiol. Pharmacol. 80 (7), 670–678. doi:10.1139/y02-070
Dittrich, A., Grimm, D., Sahana, J., Bauer, J., Krüger, M., Infanger, M., et al. (2018). Key Proteins Involved in Spheroid Formation and Angiogenesis in Endothelial Cells after Long-Term Exposure to Simulated Microgravity. Cell Physiol. Biochem. 45 (2), 429–445. doi:10.1159/000486920
Dubayle, D., Vanden-Bossche, A., Beraneck, M., Vico, L., and Morel, J.-L. (2020). Effects of Centrifugation and Whole-Body Vibrations on Blood-Brain Barrier Permeability in Mice. NPJ Microgravity 6, 1. doi:10.1038/s41526-019-0094-z
Encinas, J. M., Vazquez, M. E., Switzer, R. C., Chamberland, D. W., Nick, H., Levine, H. G., et al. (2008). Quiescent Adult Neural Stem Cells Are Exceptionally Sensitive to Cosmic Radiation. Exp. Neurol. 210 (1), 274–279. doi:10.1016/j.expneurol.2007.10.021
Eng.rakiamission (2021). The Human BBB in Space - the Effect of Space Flight and Exposure to Microgravity on the Blood-Brain Barrier (BBB) as a Potential Platform for Treating Alzheimer’s Disease. Available at: https://www.eng.rakiamission.co.il/experiements/the-human-bbb-in-space (Accessed April 26 2022 2021).
Eyal, S., and Derendorf, H. (2019). Medications in Space: In Search of a Pharmacologist's Guide to the Galaxy. Pharm. Res. 36, 148. doi:10.1007/s11095-019-2679-3
Eyal, S. (2020). How Do the Pharmacokinetics of Drugs Change in Astronauts in Space? Expert Opin. Drug Metabolism Toxicol. 16 (5), 353–356. doi:10.1080/17425255.2020.1746763
Eyal, S., Hsiao, P., and Unadkat, J. D. (2009). Drug Interactions at the Blood-Brain Barrier: Fact or Fantasy?☆. Pharmacol. Ther. 123, 80–104. doi:10.1016/j.pharmthera.2009.03.017
Fauquette, W., Amourette, C., Dehouck, M.-P., and Diserbo, M. (2012). Radiation-induced Blood-Brain Barrier Damages: An In Vitro Study. Brain Res. 1433, 114–126. doi:10.1016/j.brainres.2011.11.022
Frydenlund, D. S., Bhardwaj, A., Otsuka, T., Mylonakou, M. N., Yasumura, T., Davidson, K. G. V., et al. (2006). Temporary Loss of Perivascular Aquaporin-4 in Neocortex after Transient Middle Cerebral Artery Occlusion in Mice. Proc. Natl. Acad. Sci. U.S.A. 103 (36), 13532–13536. doi:10.1073/pnas.0605796103
Garrett-Bakelman, F. E., Darshi, M., Green, S. J., Gur, R. C., Lin, L., Macias, B. R., et al. (2019). The NASA Twins Study: A Multidimensional Analysis of a Year-Long Human Spaceflight. Science 364 (6436), eaau8650. doi:10.1126/science.aau8650
Giulianotti, M. A., and Low, L. A. (2019). Pharmaceutical Research Enabled through Microgravity: Perspectives on the Use of the International Space Station U.S. National Laboratory. Pharm. Res. 37 (1), 1. doi:10.1007/s11095-019-2719-z
Grenon, S. M., Jeanne, M., Aguado-Zuniga, J., Conte, M. S., and Hughes-Fulford, M. (2013). Effects of Gravitational Mechanical Unloading in Endothelial Cells: Association between Caveolins, Inflammation and Adhesion Molecules. Sci. Rep. 3, 1494. doi:10.1038/srep01494
Grimm, D., Infanger, M., Westphal, K., Ulbrich, C., Pietsch, J., Kossmehl, P., et al. (2009). A Delayed Type of Three-Dimensional Growth of Human Endothelial Cells under Simulated Weightlessness. Tissue Eng. Part A 15 (8), 2267–2275. doi:10.1089/ten.tea.2008.0576
Grover, A., and Pathak, Y. V. (2020). “Implications of Microgravity on Microemulsions and Nanoemulsions,” in Handbook of Space Pharmaceuticals. Editors Y. Pathak, M. Araújo dos Santos, and L. Zea (Cham: Springer International Publishing), 1–11. doi:10.1007/978-3-319-50909-9_31-1
Han, H., Mann, A., Ekstein, D., and Eyal, S. (2017). Breaking Bad: The Structure and Function of the Blood-Brain Barrier in Epilepsy. AAPS J. 19, 973–988. doi:10.1208/s12248-017-0096-2
Han, L. (2021). Modulation of the Blood-Brain Barrier for Drug Delivery to Brain. Pharmaceutics 13 (12), 2024. doi:10.3390/pharmaceutics13122024
Hier, D. B., Obafemi-Ajayi, T., Thimgan, M. S., Olbricht, G. R., Azizi, S., Allen, B., et al. (2021). Blood Biomarkers for Mild Traumatic Brain Injury: a Selective Review of Unresolved Issues. Biomark. Res. 9 (1), 70. doi:10.1186/s40364-021-00325-5
Hochwald, G. M., Malhan, C., and Brown, J. (1973). Effect of Hypercapnia on CSF Turnover and Blood-CSF Barrier to Protein. Archives Neurology 28 (3), 150–155. doi:10.1001/archneur.1973.00490210030002
Infanger, M., Kossmehl, P., Shakibaei, M., Baatout, S., Witzing, A., Grosse, J., et al. (2006). Induction of Three-Dimensional Assembly and Increase in Apoptosis of Human Endothelial Cells by Simulated Microgravity: Impact of Vascular Endothelial Growth Factor. Apoptosis 11 (5), 749–764. doi:10.1007/s10495-006-5697-7
ISS National Laboratory (2021). Spacex Crs-24 Mission Overview. Available at: https://www.issnationallab.org/iss360/spacex-crs-24-mission-overview/ (Accessed April 26, 2022. 2021).
Janmaleki, M., Pachenari, M., Seyedpour, S. M., Shahghadami, R., and Sanati-Nezhad, A. (2016). Impact of Simulated Microgravity on Cytoskeleton and Viscoelastic Properties of Endothelial Cell. Sci. Rep. 6, 32418. doi:10.1038/srep32418
Jillings, S., Van Ombergen, A., Tomilovskaya, E., Rumshiskaya, A., Litvinova, L., Nosikova, I., et al. (2020). Macro- and Microstructural Changes in Cosmonauts' Brains after Long-Duration Spaceflight. Sci. Adv. 6 (36), eaaz9488. doi:10.1126/sciadv.aaz9488
Kapitonova, M. Y., Muid, S., Froemming, G. R., Yusoff, W. N., Othman, S., Ali, A. M., et al. (2012). Real Space Flight Travel Is Associated with Ultrastructural Changes, Cytoskeletal Disruption and Premature Senescence of HUVEC. Malays J. Pathol. 34 (2), 103–113.
Kapitonova, M. Y., Kuznetsov, S. L., Froemming, G. R. A., Muid, S., Nor-Ashikin, M. N. K., Otman, S., et al. (2013). Effects of Space Mission Factors on the Morphology and Function of Endothelial Cells. Bull. Exp. Biol. Med. 154 (6), 796–801. doi:10.1007/s10517-013-2059-7
Kast, J., Yu, Y., Seubert, C. N., Wotring, V. E., and Derendorf, H. (2017). Drugs in Space: Pharmacokinetics and Pharmacodynamics in Astronauts. Eur. J. Pharm. Sci. 109, S2–s8. doi:10.1016/j.ejps.2017.05.025
Keeter, B. (2018). “ISS Daily Summary,”. Report – 12/18. Available from: https://blogs.nasa.gov/stationreport/2018/12/18/iss-daily-summary-report-12182018/ (Accessed April 26, 2022).
Kerkhofs, D., Wong, S. M., Zhang, E., Staals, J., Jansen, J. F. A., van Oostenbrugge, R. J., et al. (2021). Baseline Blood-Brain Barrier Leakage and Longitudinal Microstructural Tissue Damage in the Periphery of White Matter Hyperintensities. Neurology 96 (17), e2192–e2200. doi:10.1212/wnl.0000000000011783
Knudsen, G. M., Paulson, O. B., and Hertz, M. M. (1991). Kinetic Analysis of the Human Blood-Brain Barrier Transport of Lactate and its Influence by Hypercapnia. J. Cereb. Blood Flow. Metab. 11 (4), 581–586. doi:10.1038/jcbfm.1991.107
Koppelmans, V., Bloomberg, J. J., Mulavara, A. P., and Seidler, R. D. (2016). Brain Structural Plasticity with Spaceflight. NPJ Microgravity 2, 2. doi:10.1038/s41526-016-0001-9
Krishnamurthy, S., Zyck, S., Li, J., and Lehmann, D. (2021). Dynamic Disequilibrium of Macromolecular Transport as Possible Mechanism for Hydrocephalus Associated with Long-Term Spaceflight. Brain Res. 1753, 147229. doi:10.1016/j.brainres.2020.147229
Lakin, W. D., Stevens, S. A., and Penar, P. L. (2007). Modeling Intracranial Pressures in Microgravity: the Influence of the Blood-Brain Barrier. asem 78 (10), 932–936. doi:10.3357/asem.2060.2007
Law, J., Van Baalen, M., Foy, M., Mason, S. S., Mendez, C., Wear, M. L., et al. (2014). Relationship between Carbon Dioxide Levels and Reported Headaches on the International Space Station. J. Occup. Environ. Med. 56 (5), 477–483. doi:10.1097/jom.0000000000000158
Lee, A. G., Mader, T. H., Gibson, C. R., and Tarver, W. (2017). Space Flight-Associated Neuro-Ocular Syndrome. JAMA Ophthalmol. 135 (9), 992–994. doi:10.1001/jamaophthalmol.2017.2396
Lee, G., Dallas, S., Hong, M., and Bendayan, R. (2001). Drug Transporters in the Central Nervous System: Brain Barriers and Brain Parenchyma Considerations. Pharmacol. Rev. 53 (4), 569–596.
Lee, J. K., Koppelmans, V., Riascos, R. F., Hasan, K. M., Pasternak, O., Mulavara, A. P., et al. (2019). Spaceflight-Associated Brain White Matter Microstructural Changes and Intracranial Fluid Redistribution. JAMA Neurol. 76 (4), 412–419. doi:10.1001/jamaneurol.2018.4882
Lee, S.-T., Seo, Y., Bae, J.-Y., Chu, K., Kim, J. W., Choi, S. H., et al. (2018). Loss of Pericytes in Radiation Necrosis after Glioblastoma Treatments. Mol. Neurobiol. 55 (6), 4918–4926. doi:10.1007/s12035-017-0695-z
Lim, W. H., Choi, S. H., Yoo, R.-E., Kang, K. M., Yun, T. J., Kim, J.-H., et al. (2018). Does Radiation Therapy Increase Gadolinium Accumulation in the Brain?: Quantitative Analysis of T1 Shortening Using R1 Relaxometry in Glioblastoma Multiforme Patients. PLOS ONE 13 (2), e0192838. doi:10.1371/journal.pone.0192838
Liu, X., Ding, H., Li, X., Deng, Y., Liu, X., Wang, K., et al. (2020). Hypercapnia Exacerbates the Blood-Brain Barrier Disruption via Promoting HIF-1a Nuclear Translocation in the Astrocytes of the Hippocampus: Implication in Further Cognitive Impairment in Hypoxemic Adult Rats. Neurochem. Res. 45 (7), 1674–1689. doi:10.1007/s11064-020-03038-7
Ljubimova, N. V., Levitman, M. K., Plotnikova, E. D., and Eidus, L. K. (1991). Endothelial Cell Population Dynamics in Rat Brain after Local Irradiation. Bjr 64 (766), 934–940. doi:10.1259/0007-1285-64-766-934
Lochhead, J. J., Yang, J., Ronaldson, P. T., and Davis, T. P. (2020). Structure, Function, and Regulation of the Blood-Brain Barrier Tight Junction in Central Nervous System Disorders. Front. Physiol. 11, 914. doi:10.3389/fphys.2020.00914
Low, L. A., and Giulianotti, M. A. (2019). Tissue Chips in Space: Modeling Human Diseases in Microgravity. Pharm. Res. 37, 8. doi:10.1007/s11095-019-2742-0
Maalouf, M., Durante, M., and Foray, N. (2011). Biological Effects of Space Radiation on Human Cells: History, Advances and Outcomes. Jrr 52 (2), 126–146. doi:10.1269/jrr.10128
Maier, J. A., Cialdai, F., Monici, M., and Morbidelli, L. (2015). The Impact of Microgravity and Hypergravity on Endothelial Cells. Biomed. Res. Int. 2015, 434803. doi:10.1155/2015/434803
Mao, X. W., Nishiyama, N. C., Byrum, S. D., Stanbouly, S., Jones, T., Holley, J., et al. (2020). Spaceflight Induces Oxidative Damage to Blood‐brain Barrier Integrity in a Mouse Model. FASEB J. 34 (11), 15516–15530. doi:10.1096/fj.202001754r
Marshall-Goebel, K., Laurie, S. S., Alferova, I. V., Arbeille, P., Auñón-Chancellor, S. M., Ebert, D. J., et al. (2019). Assessment of Jugular Venous Blood Flow Stasis and Thrombosis during Spaceflight. JAMA Netw. Open 2, e1915011. doi:10.1001/jamanetworkopen.2019.15011
McInerney, M. P., Short, J. L., and Nicolazzo, J. A. (2017). Neurovascular Alterations in Alzheimer's Disease: Transporter Expression Profiles and CNS Drug Access. Aaps J. 19 (4), 940–956. doi:10.1208/s12248-017-0077-5
Mertsch, K., Blasig, I., and Grune, T. (2001). 4-Hydroxynonenal Impairs the Permeability of an In Vitro Rat Blood-Brain Barrier. Neurosci. Lett. 314 (3), 135–138. doi:10.1016/s0304-3940(01)02299-6
Morbidelli, L., Monici, M., Marziliano, N., Cogoli, A., Fusi, F., Waltenberger, J., et al. (2005). Simulated Hypogravity Impairs the Angiogenic Response of Endothelium by Up-Regulating Apoptotic Signals. Biochem. Biophysical Res. Commun. 334 (2), 491–499. doi:10.1016/j.bbrc.2005.06.124
Morofuji, Y., and Nakagawa, S. (2020). Drug Development for Central Nervous System Diseases Using In Vitro Blood-Brain Barrier Models and Drug Repositioning. Cpd 26 (13), 1466–1485. doi:10.2174/1381612826666200224112534
Mullard, A. (2021). Blood-brain Barrier-Traversing Biologic Secures Regulatory Approval, in Japan. Nat. Rev. Drug Discov. 20 (5), 332. doi:10.1038/d41573-021-00066-y
NASA (2022). Organs-On-Chips as a Platform for Studying Effects of Microgravity on Human Physiology. Available at: https://www.nasa.gov/mission_pages/station/research/experiments/explorer/Investigation.html?#id=7912 (Accessed April 26 2022).
Nicolazzo, J., and Katneni, K. (2009). Drug Transport across the Blood-Brain Barrier and the Impact of Breast Cancer Resistance Protein (ABCG2). Ctmc 9, 130–147. doi:10.2174/156802609787521580
Omidi, Y., Kianinejad, N., Kwon, Y., and Omidian, H. (2021). Drug Delivery and Targeting to Brain Tumors: Considerations for Crossing the Blood-Brain Barrier. Expert Rev. Clin. Pharmacol. 14 (3), 357–381. doi:10.1080/17512433.2021.1887729
Pan, Y., and Nicolazzo, J. A. (2022). Altered Blood-Brain Barrier and Blood-Spinal Cord Barrier Dynamics in Amyotrophic Lateral Sclerosis: Impact on Medication Efficacy and Safety. Br. J. Pharmacol. 179 (11), 2577–2588. doi:10.1111/bph.15802
Parakh, S., Nicolazzo, J., Scott, A. M., and Gan, H. K. (2021). Antibody Drug Conjugates in Glioblastoma - Is There a Future for Them? Front. Oncol. 11, 718590. doi:10.3389/fonc.2021.718590
Patel, Z. S., Brunstetter, T. J., Tarver, W. J., Whitmire, A. M., Zwart, S. R., Smith, S. M., et al. (2020). Red Risks for a Journey to the Red Planet: The Highest Priority Human Health Risks for a Mission to Mars. NPJ Microgravity 6 (1), 33. doi:10.1038/s41526-020-00124-6
Roberts, D. R., Albrecht, M. H., Collins, H. R., Asemani, D., Chatterjee, A. R., Spampinato, M. V., et al. (2017). Effects of Spaceflight on Astronaut Brain Structure as Indicated on MRI. N. Engl. J. Med. 377 (18), 1746–1753. doi:10.1056/nejmoa1705129
Rola, R., Sarkissian, V., Obenaus, A., Nelson, G. A., Otsuka, S., Limoli, C. L., et al. (2005). High-LET Radiation Induces Inflammation and Persistent Changes in Markers of Hippocampal Neurogenesis. Radiat. Res. 164 (4 Pt 2), 556–560. doi:10.1667/rr3412.1
Roy-O'Reilly, M., Mulavara, A., and Williams, T. (2021). A Review of Alterations to the Brain during Spaceflight and the Potential Relevance to Crew in Long-Duration Space Exploration. NPJ Microgravity 7 (1), 5. doi:10.1038/s41526-021-00133-z
Rubin, P., Gash, D. M., Hansen, J. T., Nelson, D. F., and Williams, J. P. (1994). Disruption of the Blood-Brain Barrier as the Primary Effect of CNS Irradiation. Radiotherapy Oncol. 31 (1), 51–60. doi:10.1016/0167-8140(94)90413-8
Ryder, P., and Braddock, M. (2020). “Harnessing the Space Environment for the Discovery and Development of New Medicines,” in Handbook of Space Pharmaceuticals. Editors Y. Patnak, M. Araújo dos Santos, and L. Zea (Swizerland: Springer Nature). doi:10.1007/978-3-319-50909-9_32-1
Santaguida, S., Janigro, D., Hossain, M., Oby, E., Rapp, E., and Cucullo, L. (2006). Side by Side Comparison between Dynamic versus Static Models of Blood-Brain Barrier In Vitro: a Permeability Study. Brain Res. 1109 (1), 1–13. doi:10.1016/j.brainres.2006.06.027
Seidler, R. D., Bernard, J. A., Burutolu, T. B., Fling, B. W., Gordon, M. T., Gwin, J. T., et al. (2010). Motor Control and Aging: Links to Age-Related Brain Structural, Functional, and Biochemical Effects. Neurosci. Biobehav. Rev. 34 (5), 721–733. doi:10.1016/j.neubiorev.2009.10.005
Shi, S., Li, J., Li, E., Guo, W., He, Y., Wang, J., et al. (2022). Simulated Microgravity Increases the Permeability of HUVEC Monolayer through Up-Regulation of Rap1GAP and Decreased Rap2 Activation. Int. J. Mol. Sci. 23 (2), 630. doi:10.3390/ijms23020630
Silvani, G., Basirun, C., Wu, H., Mehner, C., Poole, K., Bradbury, P., et al. (2021). A 3D‐Bioprinted Vascularized Glioblastoma‐on‐a‐Chip for Studying the Impact of Simulated Microgravity as a Novel Pre‐Clinical Approach in Brain Tumor Therapy. Adv. Ther. 4 (11), 2100106. doi:10.1002/adtp.202100106
Simonsen, L. C., and Zeitlin, C. (2017). Briefing to NAC HEO/SMD Joint Committee Meeting “Mars Radiation Environment – what Have We Learned?”. Available at: https://www.nasa.gov/sites/default/files/atoms/files/mars_radiation_environment_nac_july_2017_final.pdf (Accessed, 2017).
Stepanek, J., Blue, R. S., and Parazynski, S. (2019). Space Medicine in the Era of Civilian Spaceflight. N. Engl. J. Med. 380, 1053–1060. doi:10.1056/nejmra1609012
Terstappen, G. C., Meyer, A. H., Bell, R. D., and Zhang, W. (2021). Strategies for Delivering Therapeutics across the Blood-Brain Barrier. Nat. Rev. Drug Discov. 20 (5), 362–383. doi:10.1038/s41573-021-00139-y
Van Ombergen, A., Jillings, S., Jeurissen, B., Tomilovskaya, E., Rühl, R. M., Rumshiskaya, A., et al. (2018). Brain Tissue-Volume Changes in Cosmonauts. N. Engl. J. Med. 379, 1678–1680. doi:10.1056/nejmc1809011
Van Ombergen, A., Jillings, S., Jeurissen, B., Tomilovskaya, E., Rumshiskaya, A., Litvinova, L., et al. (2019). Brain Ventricular Volume Changes Induced by Long-Duration Spaceflight. Proc. Natl. Acad. Sci. U.S.A. 116 (21), 10531–10536. doi:10.1073/pnas.1820354116
Vernooij, M. W., Ikram, M. A., Vrooman, H. A., Wielopolski, P. A., Krestin, G. P., Hofman, A., et al. (2009). White Matter Microstructural Integrity and Cognitive Function in a General Elderly Population. Arch. Gen. Psychiatry 66 (5), 545–553. doi:10.1001/archgenpsychiatry.2009.5
Versari, S., Longinotti, G., Barenghi, L., Maier, J. A. M., and Bradamante, S. (2013). The Challenging Environment on Board the International Space Station Affects Endothelial Cell Function by Triggering Oxidative Stress through Thioredoxin Interacting Protein Overexpression: the ESA‐SPHINX Experiment. FASEB J. 27 (11), 4466–4475. doi:10.1096/fj.13-229195
Wardlaw, J. M., Makin, S. J., Valdés Hernández, M. C., Armitage, P. A., Heye, A. K., Chappell, F. M., et al. (2017). Blood‐brain Barrier Failure as a Core Mechanism in Cerebral Small Vessel Disease and Dementia: Evidence from a Cohort Study. Alzheimer's & Dement. 13 (6), 634–643. doi:10.1016/j.jalz.2016.09.006
Wehland, M., Ma, X., Braun, M., Hauslage, J., Hemmersbach, R., Bauer, J., et al. (2013). The Impact of Altered Gravity and Vibration on Endothelial Cells during a Parabolic Flight. Cell Physiol. Biochem. 31 (2-3), 432–451. doi:10.1159/000343380
Wilson, C. M., Gaber, M. W., Sabek, O. M., Zawaski, J. A., and Merchant, T. E. (2009). Radiation-Induced Astrogliosis and Blood-Brain Barrier Damage Can Be Abrogated Using Anti-TNF Treatment. Int. J. Radiat. Oncology*Biology*Physics 74 (3), 934–941. doi:10.1016/j.ijrobp.2009.02.035
Yan, R., Liu, H., Lv, F., Deng, Y., and Li, Y. (2021). Rac1/Wave2/Arp3 Pathway Mediates Rat Blood-Brain Barrier Dysfunction under Simulated Microgravity Based on Proteomics Strategy. Int. J. Mol. Sci. 22 (10), 5165. doi:10.3390/ijms22105165
Zhang, C. E., Wong, S. M., van de Haar, H. J., Staals, J., Jansen, J. F. A., Jeukens, C. R. L. P. N., et al. (2017). Blood-brain Barrier Leakage Is More Widespread in Patients with Cerebral Small Vessel Disease. Neurology 88 (5), 426–432. doi:10.1212/wnl.0000000000003556
Keywords: blood-brain barrier, spaceflight, space exploration, astronaut, weightlessness, microgravity, aviation, drug delivery
Citation: Amselem S and Eyal S (2022) The Blood-Brain Barrier in Space: Implications for Space Travelers and for Human Health on Earth. Front. Drug. Deliv. 2:931221. doi: 10.3389/fddev.2022.931221
Received: 28 April 2022; Accepted: 17 June 2022;
Published: 25 July 2022.
Edited by:
Reina Bendayan, University of Toronto, CanadaReviewed by:
Allison Andrews, Temple University, United StatesCopyright © 2022 Amselem and Eyal. This is an open-access article distributed under the terms of the Creative Commons Attribution License (CC BY). The use, distribution or reproduction in other forums is permitted, provided the original author(s) and the copyright owner(s) are credited and that the original publication in this journal is cited, in accordance with accepted academic practice. No use, distribution or reproduction is permitted which does not comply with these terms.
*Correspondence: Sara Eyal, c2FyYS5leWFsQG1haWwuaHVqaS5hYy5pbA==
Disclaimer: All claims expressed in this article are solely those of the authors and do not necessarily represent those of their affiliated organizations, or those of the publisher, the editors and the reviewers. Any product that may be evaluated in this article or claim that may be made by its manufacturer is not guaranteed or endorsed by the publisher.
Research integrity at Frontiers
Learn more about the work of our research integrity team to safeguard the quality of each article we publish.