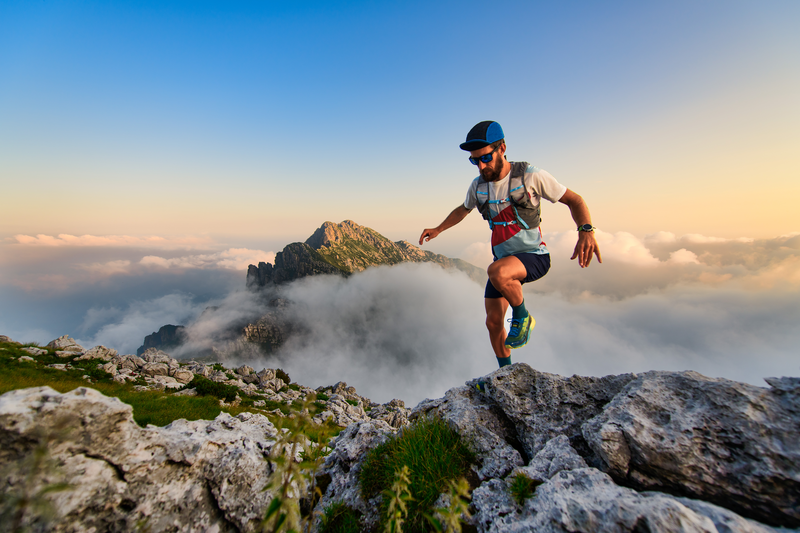
94% of researchers rate our articles as excellent or good
Learn more about the work of our research integrity team to safeguard the quality of each article we publish.
Find out more
MINI REVIEW article
Front. Drug Deliv. , 14 December 2022
Sec. CNS Drug Delivery
Volume 2 - 2022 | https://doi.org/10.3389/fddev.2022.1073815
This article is part of the Research Topic Impact of Various Diseases on Brain Barrier Function and Central Nervous System Drug Delivery View all 4 articles
The global pandemic of coronavirus disease 2019 (COVID-19) is caused by severe acute respiratory syndrome coronavirus 2 (SARS-CoV-2) persists despite the progress of vaccination and increased natural immunity. SARS-CoV-2 is associated not only with pneumonia and acute respiratory distress, but also with many symptoms related to the central nervous system (CNS), including loss of the sense of taste and smell, headache, convulsions, visual disturbances, and impaired consciousness. In addition, the virus has been implicated in CNS diseases such as cerebral hemorrhage, cerebral infarction, and encephalitis. SARS-CoV-2 binds to the receptor angiotensin-converting enzyme 2 (ACE2), which is used by the virus as a cell entry receptor. Although the mechanism by which SARS-CoV-2 enters the brain is still unclear, the possibility of direct entry through the olfactory nerve tract and entry into the brain through the blood-brain barrier (BBB) and blood-cerebrospinal fluid barrier (BCSFB) via blood circulation is indicated. The BBB likely serves as a site of entry for SARS-CoV-2 into the brain, and possibly contributes to the CNS symptoms of COVID-19 due to its dysfunction as a result of SARS-CoV-2 infection. The present review will focus on the effects of COVID-19 on the CNS, particularly on the BBB related cells involved.
Coronavirus disease 2019 (COVID-19) is a widespread pandemic caused by the highly contagious severe acute respiratory syndrome coronavirus 2 (SARS-CoV-2) that has not been eradicated despite much time and vaccination progress. Many variants have been reported, and a variety of symptoms observed, often focusing on respiratory dysfunction, but it has also been shown to cause disorder in many other organs. Most patients with COVID-19 present mild to moderate symptoms, with the most common symptoms occurring as fever, upper respiratory tract symptoms such as dry cough and sore throat and headache, although some who are infected can remain asymptomatic. However, only a small percentage of cases progress to severe stages, leading to severe pneumonia, multiorgan dysfunction, and in the worst cases, death (Huang et al., 2020; Pascarella et al., 2020; Shi et al., 2020). An early review of data from the pandemic showed that approximately 5% of COVID-19 patients had severe symptoms, such as acute respiratory distress syndrome (ARDS) and multiorgan-dysfunction (Wiersinga et al., 2020; Rahman et al., 2021), and the mortality was estimated to be around 0.15%–1.63%, with a median of 0.27% ((Ioannidis 2020; AuthorAnonymous, 2021).
Neurological symptoms in COVID-19 patients have been noted since the early days of the disease. Common neurological symptoms include headache, vomiting and dizziness, in addition to a characteristic loss of the senses of taste and smell (Menni et al., 2020; Wang et al., 2020). Interestingly, even in the absence of typical symptoms of COVID-19, SARS-CoV-2 can cause neurological disorders such as encephalitis, Guillain-Barré syndrome and seizures (Karadaş, Öztürk, and Sonkaya 2020; Nalleballe et al., 2020; Johansson et al., 2021). In severe cases, acute cerebrovascular disorders and impaired consciousness have been reported (Mao et al., 2020). The severity of COVID-19 has been implicated in the increased risk of subsequent neurological and psychiatric outcomes, involving mechanisms such as direct brain damage associated with viral entry into the CNS and induction of BBB dysfunction, as well as the neural effects of the immune response, inflammation, and hypercoagulability (Shehata et al., 2021; Taquet et al., 2021). This review summarizes the understanding of CNS dysfunction from the viewpoint of the blood-brain barrier (BBB) with SARS-CoV-2 infection and COVID-19.
Many human coronaviruses cause respiratory diseases; some are endemic and among the causes of the common cold, whereas others, such as SARS-CoV and MERS-CoV, have caused serious epidemics with high mortality rates. SARS-CoV-2, like SARS-CoV and MERS-CoV, was likely first transmitted to humans via exposure to an infected animal, although all three can pass from human-to-human. The main structure of SARS-CoV-2 is composed of four proteins, Spike (S), Envelop (E), Nucleocapsid (N), and Membrane (M). The S protein plays the major role in cell binding and invasion (Kumar and Al Khodor 2020; Wu et al., 2020). The S protein is a transmembrane protein with spike-like projections and is characterized by two extracellular subunits S1 and S2; the S1 subunit facilitates receptor-binding, and the S2 subunit mediates membrane fusion and internalization of the virion (Du et al., 2009). The S protein promotes viral attachment to angiotensin-converting enzyme 2 (ACE2), thus fusing with the membrane and allowing the virus to enter the cell. SARS-CoV-2 closely resembles SARS-CoV virus that also causes severe acute respiratory syndrome (SARS), but several mutations in the receptor-binding region (RBD) of the S1 protein have greatly enhanced the binding affinity of the SARS-CoV-2 virus to ACE2. Such differences can be the basis for the high infectivity of COVID-19 (Angeletti et al., 2020; Tai et al., 2020). The S protein entry into target cells requires protein priming with cellular proteases, which has been shown to be mediated by the cellular transmembrane serine protease 2 (TMPRSS2) (Glowacka et al., 2011; Hoffmann et al., 2020). SARS-CoV-2 mainly infects cells in the respiratory tract, but infection of the CNS has also been observed and the mechanism has become clarified (3). Quantification of the virus in patients who died from COVID-19 indicated the neurotropic potential of the virus (Matschke et al., 2020; Puelles et al., 2020; Solomon et al., 2020; Serrano et al., 2021), and other evidence has been presented from cells, organoid models, and animals. Primary human brain endothelial cells were shown to be productively infected with SARS-CoV-2 by expressing ACE2 via lentivirus transduction (Nascimento Conde et al., 2020). Experiments on 3D human brain organoids similarly show that SARS-CoV-2 can enter the cells and have the potential neurotoxic effect (Ramani et al., 2020; Song et al., 2021). Several experiments using animal models, mainly mice, also indicate evidence for viral entry into the brain. Infection models using transgenic mice such as hACE and Hfh4-ACE2 mice have shown evidence for the presence of viral RNA in the brain (Menachery et al., 2016; Bao et al., 2020; Dinnon et al., 2020; Dong et al., 2022). Studies of non-human primate infection models in Rhesus macaques and African green monkeys similarly detected viral RNA in the brain, while pathological findings of neuroinflammation, microhemorrhage, and neuronal damage were obtained, suggesting that SARS-CoV-2 infection of the CNS may cause some of its neurological symptoms (Rutkai et al., 2022).
Clinical symptoms, such as olfactory disorders, and experimental data suggest that SARS-CoV-2 may invade the CNS via the olfactory sensory neurons, although it is also likely that the virus crosses the BBB and enters the CNS. As for SARS-CoV-2 entry into the CNS via olfactory neurons, it has been posited that the virus can travel along their axons into the olfactory bulbs and beyond to other parts of the brain (Bougakov, Podell, and Goldberg 2021; Burks et al., 2021). The pathway of axonal transport through the olfactory nerve begins with infection of the olfactory epithelial cells via ACE2 receptors and spreads to the CNS by traversing synapses shared between olfactory neurons and neurons in the olfactory bulb (OB). Data from RNA mapping of the olfactory nerve tract to the CNS region of SARS-CoV-2 infection from human autopsy material confirms viral amplification along the olfactory nerve tract (Meinhardt et al., 2021). Neuropilin-1 (NRP-1) is a multifunctional transmembrane receptor that affects development of axons and is suggested to contribute to the entry of SARS-CoV-2 into the brain via the olfactory epithelium (Perez-Miller et al., 2021). NRP-1 increases the infectivity of viruses in olfactory epithelial cells, and the high expression of NRP-1 in the olfactory epithelium of COVID-19 patients suggests that NRP-1 plays an important role in the entry of viruses through the olfactory pathway (Cantuti-Castelvetri et al., 2020). However, several studies have been presented that provide limited support for this pathway of viral entry. A kinetics study of S1 uptake by brain found that the olfactory rate was much lower than the rate of transport across the BBB(Rhea et al., 2021). Also, when SARS-CoV-2 is detected in olfactory neurons, it is mostly present in immature neurons, which lack the axonal projections necessary to transport the virus to the brain. Therefore, it is controversial whether the olfactory route is the main pathway by which SARS-CoV-2 enters the brain (Butowt et al., 2021; Zhang, Lee, et al., 2021).
Another promising route of SARS-CoV-2 entry into the brain is via the hematogenous route, crossing the BBB and/or blood-cerebrospinal fluid barrier (BCSFB). The interactions between SARS-CoV-2 and the BBB are multifactorial in that the virus may cross the BBB, infect brain endothelial cells, or alter functions of the BBB, with data suggesting that several receptors and signaling cascades are involved (Torices et al., 2021; Haidar et al., 2022; Krasemann et al., 2022). The BBB is primarily composed of brain endothelial cells, which are closely associated with other cells such as the pericytes and astrocytes that support the BBB endothelial phenotype. Brain endothelial cells express tight junction proteins that inhibit the diffusion of substances between cells, and a low number of pinocytic vesicles that prevents transcellular diffusion. Thus, viruses that can enter the CNS via the BBB must do so either by being transported via processes such as adsorptive transcytosis, or by altering BBB properties which then permits their direct entry (Erickson et al., 2021). The BCSFB, formed from epithelial cells of the choroid plexus, is also a barrier separating the central and peripheral systems and is considered a potential entry route of SARS-CoV-2. Experiments evaluating the neurotropism of the virus using an organoid model of choroid plexus epithelial cells showed that SARS-CoV-2 selectively infects choroid plexus epithelial cells and also disrupts BCSFB (Pellegrini et al., 2020). However, SARS-CoV-2 is variably detected by PCR in CSF samples from COVID-19 patients, including those with neurological symptoms (Jarius et al., 2022), indicating that most of the virus may be sequestered in the brain tissue. Since CNS entry via the BCSFB would first require SARS-CoV-2 to enter the CSF, it is controversial to consider the BCSFB as the main entry route.
Several studies have focused on the S protein, the main component of the virus, and its interactions with the BBB. In early experiments using 2D static and 3D microfluidic in vitro BBB models to verify the relation between the BBB and the proteins that compose SARS-CoV-2, including S protein, showed that those proteins disrupt the integrity of the barrier function of the BBB (Buzhdygan et al., 2020). However, the dosage of S protein used in the study are variable, with some higher than S protein concentrations typically detected in blood (Ogata et al., 2020), they range from 0 to 25 ng/ml and in most cases less than 1 ng/ml, so careful consideration is needed. Experiments that injected intravenously radioiodinated S1 protein showed that S1 is taken up into the brain much better in comparison to the intranasal route. This shows that S1 readily crosses the BBB, and additional studies suggest the mechanism involved is adsorptive transcytosis (Rhea et al., 2021). The same study also found that inflammation induced by bacterial lipopolysaccharide (LPS) caused some minor leakage of S1 across the BBB, but did not alter the transport mechanism which accounted for most of the S1 entry into brain. In mouse and hamster models that are permissive to SARS-CoV-2 infection, the virus caused no obvious ultrastructural or expression change in BBB tight junction proteins but altered the basement membrane and caused Evans blue dye leakage into the brain, indicating that there was BBB disruption. The virus infected brain endothelial cells, upregulated inflammatory cytokine expression, and crossed the BBB in vitro, although it caused only a moderate amount of paracellular disruption in hamster endothelial cells, suggesting that the virus crossed the BBB via transcellular mechanism s and without causing paracellular leakage (Zhang, Zhou, et al., 2021). Therefore, SARS-CoV-2 likely exerts effects on the BBB via its direct interactions with brain endothelial cells and indirectly through the immune system. However, it should be carefully considered that SARS-CoV-2 have low replication capacity in brain endothelial cells without inflammatory conditions or overexpression of ACE2 (Constant et al., 2021; Schimmel et al., 2021). Evidence supports that both the virus and its spike proteins can cross the BBB and enter the brain. Effects of S1 and SARS-CoV-2 on BBB as mediated through other types of brain cells are ongoing.
Brain endothelial cells are thought to play an important role in SARS-CoV-2 invasion because they face circulatory dynamics and express receptors associated with viral invasion, such as ACE2 and TMPRSS2 (Baig et al., 2020). SARS-CoV-2 infects endothelial cells via ACE2 receptors without apparent the BBB disruption and enters the brain, simultaneously, it is suggested that SARS-CoV-2 infection causes endotheliitis in several organs, which leads to the BBB dysfunction and enables the virus to enter the brain directly (Varga et al., 2020). That human brain endothelial cells express ACE2 is supported by experiments using primary human brain endothelial cells and the hCMEC/D3 cell line, which also showed that SARS-CoV-2 can infect and proliferate in brain endothelial cells (Buzhdygan et al., 2020; Zhang, Zhou, et al., 2021). Interestingly, the results of these studies showed that SARS-CoV-2 infection of brain endothelial cells did not cause any gross phenotypic alterations and did not affect tight junction protein integrity. The host protein nuclear factor (NF)-κB essential modulator (NEMO), which is involved in signalling cascades that regulate the transcription of numerous genes, regulates cell viability, and SARS-CoV-2 caused NEMO cleavage and cell apoptosis in brain endothelial cells, leading to possible vulnerability of the BBB (Wenzel et al., 2021).
Several studies suggest that the interactions of brain endothelial cells and SARS-CoV-2 could be increased with certain comorbid conditions. Cerebral ischemia, smoking, and diabetes have been shown to enhance ACE2 receptor expression in brain endothelial cells, supporting that these factors could potentially increase the ability of SARS-CoV-2 to infect or cross brain endothelial cells and thus increase the risk of COVID-19-associated neurological sequalae (Choi et al., 2020). Hypoxia was also shown to modulate expression of ACE2 and TMPRSS2 in human brain endothelial cells, suggesting involvement of endothelial damage caused by SARS-CoV-2 (Imperio et al., 2021). The results suggest that COVID-19 could be implicated in the induction of inflammation and apoptosis of brain endothelial cells involved in enhanced thrombus formation in patients with severe COVID-19 (Pons et al., 2020), which may cause cerebrovascular disease. Beta-secretase 1 (BACE1), an aspartic acid protease, is a transmembrane protein that can contribute to the loss of integrity of the BBB (Cheng et al., 2014). SARS-CoV-2 increased BACE1 in brain endothelial cells, resulting in decreased expression of tight junction proteins and accumulation of senescence-associatedβ-gal and p21, which are involved in cellular senescence. These results support the possibility that SARS-CoV-2 causes BBB vulnerability and senescence of brain endothelial vascular cells resulting in cerebrovascular disease (Choi et al., 2022).
Astrocytes have an important role in the induction and maintenance of the BBB phenotype and are anatomically close to brain endothelial cells. Several studies indicate that astrocytes can be infected by SARS-CoV-2. Of the samples that showed pathological signs of brain damage in autopsy patients with COVID-19, particularly in astrocytes, lesions from viral replication were observed (Crunfli et al., 2022). The same study also found that the susceptibility to infection via NRP-1 was shown in human astrocytes derived from neural stem cells, emphasizing the possibility that astrocytes may contribute to CNS infection Plasma biomarker testing of patients with COVID-19 showed increased glial fibrillary acidic protein (GFAP), a marker of astrocyte activity and damage (Kanberg et al., 2020). This finding is significant in severe COVID-19 patients and could be involved in the development of brain disorders in these patients. In the data investigating gene expression in astrocytes after SARS-CoV-2 infection, subpopulations of astrocytes were identified in which the expression of genes involved in inflammation and neurotoxicity, such as IFITM3, GFAP, and CHI3L1, were upregulated, and dysregulation of genes supporting neurotransmission was also observed (Yang et al., 2021). Additionally, infection and viral replication induced in human astrocytes from SARS-CoV-2 exposure was confirmed, indicating extensive inflammation and cytokine secretion. Interestingly, the ACE2 receptor was not identified in these astrocytes, suggesting that other invasion factors, DPP4 and CD147, are closely involved (Andrews et al., 2022). Brain pericytes surround blood vessels and play an important role in maintaining the BBB, including vessel maturation and stabilization, neuroprotection and repair in the event of damage. ACE2 has been shown to be abundantly expressed in platelet-derived growth factor receptor (PDGFR) β perivascular cells, which consist mainly of pericytes, and has been implicated in possible SARS-CoV-2 infection. Infection of pericytes in COVID-19 patients was associated with perivascular inflammation and fibrinogen leakage, suggesting that the integrity of the BBB is compromised (Bocci et al., 2021). S protein exposure increased ACE2 expression and triggered an inflammatory response in pericytes Additional evidence indicates that hypoxia enhances S protein related effects on brain pericytes, and that SARS-CoV-2 can cause vascular-mediated brain damage (Khaddaj-Mallat et al., 2021). Microglia are immune cells that respond to infection and have a wide range of functions, including activation of astrocytes and regulation of neurons. Microglia clustered prominently around blood vessels in COVID-19 patients, suggesting that they induce neuroinflammation (Schwabenland et al., 2021). Microglia, as well as astrocytes, showed subpopulations associated with COVID-19, showing increased levels of several genes involved in neuroinflammation (Yang et al., 2021).
Since the early stages of the COVID-19 pandemic, neurological symptoms such as loss of sense of smell and taste have been noted, and many studies have focused on that aspect. Neurological complications are particularly common in severely ill COVID-19 patients, and residual effects are observed even after symptoms have abated (Helms et al., 2020). Serious brain disorders such as cerebral infarction, encephalitis, acute toxic encephalopathy, and epileptic seizures have also been reported in many cases (Roy et al., 2021). Recent studies have reported Long-COVID syndrome and identified several neurological signs, notably brain fog, suggesting neuroinflammation is involved (Theoharides et al., 2021). This could be related to damage to brain endothelial cells caused by S protein and to the induction of inflammation and autoimmune reactions (Theoharides 2022).
These many accumulated reports suggest that the CNS is affected by COVID-19, and there have been various discussions regarding the mechanism, but no clear evidence has been presented. This review focused on the neurotropism of SARS-CoV-2, particularly with respect to its ability to cross the BBB and infect brain endothelial cells, astrocytes, and pericytes. Numerous papers indicate that the BBB is involved in COVID-19 infection of the CNS and that SARS-CoV-2 alters the homeostatic functions of the BBB. Therefore, the BBB is an ideal target for drug development aimed at preventing or facilitating recovery from neurological damage and disease caused by SARS-CoV-2.
TF performed the literature review, interpreted the data, and prepared the manuscript. ME and WB wrote or contributed to the writing of the manuscript.
This work was supported by JSPS KAKENHI Grant Number 21K16635.
The authors declare that the research was conducted in the absence of any commercial or financial relationships that could be construed as a potential conflict of interest.
All claims expressed in this article are solely those of the authors and do not necessarily represent those of their affiliated organizations, or those of the publisher, the editors and the reviewers. Any product that may be evaluated in this article, or claim that may be made by its manufacturer, is not guaranteed or endorsed by the publisher.
Andrews, M. G., Mukhtar, T., Eze, U. C., Simoneau, C. R., Ross, J., Parikshak, N., et al. (2022). 'Tropism of SARS-CoV-2 for human cortical astrocytes. Proc. Natl. Acad. Sci. U. S. A. 119, e2122236119. doi:10.1073/pnas.2122236119
Angeletti, S., Benvenuto, D., Bianchi, M., Giovanetti, M., Pascarella, S., and Ciccozzi, M. (2020). COVID-2019: The role of the nsp2 and nsp3 in its pathogenesis. J. Med. Virol. 92, 584–588. doi:10.1002/jmv.25719
Baig, A. M., Khaleeq, A., Ali, U., and Syeda, H. (2020). 'Evidence of the COVID-19 virus targeting the CNS: Tissue distribution, host-virus interaction, and proposed neurotropic mechanisms. ACS Chem. Neurosci. 11, 995–998. doi:10.1021/acschemneuro.0c00122
Bao, L., Deng, W., Huang, B., Gao, H., Liu, J., Ren, L., et al. (2020). The pathogenicity of SARS-CoV-2 in hACE2 transgenic mice. Nature 583, 830–833. doi:10.1038/s41586-020-2312-y
Bocci, M., Oudenaarden, C., Sàenz-Sardà, X., Simrén, J., Edén, A., Sjölund, J., et al. (2021). 'Infection of brain pericytes underlying neuropathology of COVID-19 patients. Int. J. Mol. Sci. 22, 11622. doi:10.3390/ijms222111622
Bougakov, D., Podell, K., and Goldberg, E. (2021). 'Multiple neuroinvasive pathways in COVID-19. Mol. Neurobiol. 58, 564–575. doi:10.1007/s12035-020-02152-5
Burks, S. M., Rosas-Hernandez, H., Alejandro Ramirez-Lee, M., Cuevas, E., and Talpos, J. C. (2021). Can SARS-CoV-2 infect the central nervous system via the olfactory bulb or the blood-brain barrier? Brain Behav. Immun. 95, 7–14. doi:10.1016/j.bbi.2020.12.031
Butowt, R., Meunier, N., Bryche, B., and von Bartheld, C. S. (2021). 'The olfactory nerve is not a likely route to brain infection in COVID-19: A critical review of data from humans and animal models. Acta Neuropathol. 141, 809–822. doi:10.1007/s00401-021-02314-2
Buzhdygan, T. P., DeOre, B. J., Baldwin-Leclair, A., Bullock, T. A., McGary, H. M., Khan, J. A., et al. (2020). 'The SARS-CoV-2 spike protein alters barrier function in 2D static and 3D microfluidic in-vitro models of the human blood-brain barrier. Neurobiol. Dis. 146, 105131. doi:10.1016/j.nbd.2020.105131
Cantuti-Castelvetri, L., Ojha, R., Pedro, L. D., Djannatian, M., Franz, J., Kuivanen, S., et al. (2020). 'Neuropilin-1 facilitates SARS-CoV-2 cell entry and infectivity. Science 370, 856–860. doi:10.1126/science.abd2985
Cheng, X., He, P., Yao, H., Dong, Q., Li, R., and Shen, Y. (2014). 'Occludin deficiency with BACE1 elevation in cerebral amyloid angiopathy. Neurology 82, 1707–1715. doi:10.1212/WNL.0000000000000403
Choi, J. Y., Lee, H. K., Park, J. H., Cho, S. J., Kwon, M., Jo, C., et al. (2020). 'Altered COVID-19 receptor ACE2 expression in a higher risk group for cerebrovascular disease and ischemic stroke. Biochem. Biophys. Res. Commun. 528, 413–419. doi:10.1016/j.bbrc.2020.05.203
Choi, J. Y., Park, J. H., Jo, C., Kim, K. C., and Koh, Y. H. (2022). SARS-CoV-2 spike S1 subunit protein-mediated increase of beta-secretase 1 (BACE1) impairs human brain vessel cells. Biochem. Biophys. Res. Commun. 626, 66–71. doi:10.1016/j.bbrc.2022.07.113
Constant, O., Barthelemy, J., Bolloré, K., Tuaillon, E., Gosselet, F., Chable-Bessia, C., et al. (2021). 'SARS-CoV-2 poorly replicates in cells of the human blood-brain barrier without associated deleterious effects. Front. Immunol. 12, 697329. doi:10.3389/fimmu.2021.697329
Crunfli, F., Carregari, V. C., Veras, F. P., Silva, L. S., Nogueira, M. H., Antunes, Aslm, et al. (2022). 'Morphological, cellular, and molecular basis of brain infection in COVID-19 patients. Proc. Natl. Acad. Sci. U. S. A. 119, e2200960119. doi:10.1073/pnas.2200960119
Dinnon, K. H., Leist, S. R., Schäfer, A., Edwards, C. E., Martinez, D. R., Montgomery, S. A., et al. (2020). 'A mouse-adapted model of SARS-CoV-2 to test COVID-19 countermeasures. Nature 586, 560–566. doi:10.1038/s41586-020-2708-8
Dong, W., Mead, H., Tian, L., Park, J. G., Garcia, J. I., Jaramillo, S., et al. (2022). 'The K18-human ACE2 transgenic mouse model recapitulates non-severe and severe COVID-19 in response to an infectious dose of the SARS-CoV-2 virus. J. Virol. 96, e0096421. doi:10.1128/JVI.00964-21
Du, L., He, Y., Zhou, Y., Liu, S., Zheng, B. J., and Jiang, S. (2009). 'The spike protein of SARS-CoV-a target for vaccine and therapeutic development. Nat. Rev. Microbiol. 7, 226–236. doi:10.1038/nrmicro2090
Erickson, M. A., Rhea, E. M., Knopp, R. C., and Banks, W. A. (2021). 'Interactions of SARS-CoV-2 with the blood-brain barrier. Int. J. Mol. Sci. 22, 2681. doi:10.3390/ijms22052681
Glowacka, I., Bertram, S., Müller, M. A., Allen, P., Soilleux, E., Pfefferle, S., et al. (2011). 'Evidence that TMPRSS2 activates the severe acute respiratory syndrome coronavirus spike protein for membrane fusion and reduces viral control by the humoral immune response. J. Virol. 85, 4122–4134. doi:10.1128/JVI.02232-10
Haidar, M. A., Jourdi, H., Haj Hassan, Z., Ashekyan, O., Fardoun, M., Wehbe, Z., et al. (2022). 'Neurological and neuropsychological changes associated with SARS-CoV-2 infection: New observations, new mechanisms. Neuroscientist. 28, 552–571. doi:10.1177/1073858420984106
Helms, J., Kremer, S., Merdji, H., Clere-Jehl, R., Schenck, M., Kummerlen, C., et al. (2020). 'Neurologic features in severe SARS-CoV-2 infection. N. Engl. J. Med. 382, 2268–2270. doi:10.1056/NEJMc2008597
Hoffmann, M., Kleine-Weber, H., Schroeder, S., Krüger, N., Herrler, T., Erichsen, S., et al. (2020). 'SARS-CoV-2 cell entry depends on ACE2 and TMPRSS2 and is blocked by a clinically proven protease inhibitor. Cell 181, 271–280. doi:10.1016/j.cell.2020.02.052
Huang, C., Wang, Y., Li, X., Ren, L., Zhao, J., Hu, Y., et al. (2020). Clinical features of patients infected with 2019 novel coronavirus in Wuhan, China. Lancet 395, 497–506. doi:10.1016/S0140-6736(20)30183-5
Imperio, G. E., Lye, P., Mughis, H., Hamada, H., Bloise, E., Lye, S. J., et al. (2021). Hypoxia alters the expression of ACE2 and TMPRSS2 SARS-CoV-2 cell entry mediators in hCMEC/D3 brain endothelial cells. Microvasc. Res. 138, 104232. doi:10.1016/j.mvr.2021.104232
Ioannidis, J. P. A. (2020). 'Global perspective of COVID-19 epidemiology for a full-cycle pandemic. Eur. J. Clin. Invest. 50, e13423. doi:10.1111/eci.13423
Jarius, S., Pache, F., Körtvelyessy, P., Jelčić, I., Stettner, M., Franciotta, D., et al. (2022). 'Cerebrospinal fluid findings in COVID-19: A multicenter study of 150 lumbar punctures in 127 patients. J. Neuroinflammation 19, 19. doi:10.1186/s12974-021-02339-0
Johansson, A., Mohamed, M. S., Moulin, T. C., and Schiöth, H. B. (2021). 'Neurological manifestations of COVID-19: A comprehensive literature review and discussion of mechanisms. J. Neuroimmunol. 358, 577658. doi:10.1016/j.jneuroim.2021.577658
Kanberg, N., Ashton, N. J., Andersson, L. M., Yilmaz, A., Lindh, M., Nilsson, S., et al. (2020). 'Neurochemical evidence of astrocytic and neuronal injury commonly found in COVID-19. Neurology 95, e1754–e1759. doi:10.1212/WNL.0000000000010111
Karadaş, Ö., Öztürk, B., and Sonkaya, A. R. (2020). 'A prospective clinical study of detailed neurological manifestations in patients with COVID-19. Neurol. Sci. 41, 1991–1995. doi:10.1007/s10072-020-04547-7
Khaddaj-Mallat, R., Aldib, N., Bernard, M., Paquette, A. S., Ferreira, A., Lecordier, S., et al. (2021). 'SARS-CoV-2 deregulates the vascular and immune functions of brain pericytes via Spike protein. Neurobiol. Dis. 161, 105561. doi:10.1016/j.nbd.2021.105561
Krasemann, S., Haferkamp, U., Pfefferle, S., Woo, M. S., Heinrich, F., Schweizer, M., et al. (2022). 'The blood-brain barrier is dysregulated in COVID-19 and serves as a CNS entry route for SARS-CoV-2. Stem Cell Rep. 17, 307–320. doi:10.1016/j.stemcr.2021.12.011
Kumar, M., and Al Khodor, S. (2020). Pathophysiology and treatment strategies for COVID-19. J. Transl. Med. 18, 353. doi:10.1186/s12967-020-02520-8
Mao, L., Jin, H., Wang, M., Hu, Y., Chen, S., He, Q., et al. (2020). Neurologic manifestations of hospitalized patients with coronavirus disease 2019 in wuhan, China. JAMA Neurol. 77, 683–690. doi:10.1001/jamaneurol.2020.1127
Matschke, J., Lütgehetmann, M., Hagel, C., Sperhake, J. P., Schröder, A. S., Edler, C., et al. (2020). 'Neuropathology of patients with COVID-19 in Germany: A post-mortem case series. Lancet. Neurol. 19, 919–929. doi:10.1016/S1474-4422(20)30308-2
Meinhardt, J., Radke, J., Dittmayer, C., Franz, J., Thomas, C., Mothes, R., et al. (2021). Olfactory transmucosal SARS-CoV-2 invasion as a port of central nervous system entry in individuals with COVID-19. Nat. Neurosci. 24, 168–175. doi:10.1038/s41593-020-00758-5
Menachery, V. D., Yount, B. L., Sims, A. C., Debbink, K., Agnihothram, S. S., Gralinski, L. E., et al. (2016). 'SARS-like WIV1-CoV poised for human emergence. Proc. Natl. Acad. Sci. U. S. A. 113, 3048–3053. doi:10.1073/pnas.1517719113
Menni, C., Valdes, A. M., Freidin, M. B., Sudre, C. H., Nguyen, L. H., Drew, D. A., et al. (2020). 'Real-time tracking of self-reported symptoms to predict potential COVID-19. Nat. Med. 26, 1037–1040. doi:10.1038/s41591-020-0916-2
Nalleballe, K., Reddy Onteddu, S., Sharma, R., Dandu, V., Brown, A., Jasti, M., et al. (2020). 'Spectrum of neuropsychiatric manifestations in COVID-19. Brain Behav. Immun. 88, 71–74. doi:10.1016/j.bbi.2020.06.020
Nascimento Conde, J., Schutt, W. R., Gorbunova, E. E., and Mackow, E. R. (2020). Recombinant ACE2 expression is required for SARS-CoV-2 to infect primary human endothelial cells and induce inflammatory and procoagulative responses. mBio 11 (6), 031855–e3220. doi:10.1128/mBio.03185-20
Oannidis, J. P. A. (2021). 'Infection fatality rate of COVID-19 inferred from seroprevalence data. Bull. World Health Organ. 99, 19–33f. doi:10.2471/BLT.20.265892
Ogata, A. F., Maley, A. M., Wu, C., Gilboa, T., Norman, M., Lazarovits, R., et al. (2020). Ultra-sensitive serial profiling of SARS-CoV-2 antigens and antibodies in Plasma to understand disease progression in COVID-19 patients with severe disease. Clin. Chem. 66, 1562–1572. doi:10.1093/clinchem/hvaa213
Pascarella, G., Strumia, A., Piliego, C., Bruno, F., Del Buono, R., Costa, F., et al. (2020). 'COVID-19 diagnosis and management: A comprehensive review. J. Intern. Med. 288, 192–206. doi:10.1111/joim.13091
Pellegrini, L., Albecka, A., Mallery, D. L., Kellner, M. J., Paul, D., Carter, A. P., et al. (2020). 'SARS-CoV-2 infects the brain choroid plexus and disrupts the blood-CSF barrier in human brain organoids. Cell Stem Cell 27, 951–961. doi:10.1016/j.stem.2020.10.001
Perez-Miller, S., Patek, M., Moutal, A., Duran, P., Cabel, C. R., Thorne, C. A., et al. (2021). 'Novel compounds targeting neuropilin receptor 1 with potential to interfere with SARS-CoV-2 virus entry. ACS Chem. Neurosci. 12, 1299–1312. doi:10.1021/acschemneuro.0c00619
Pons, S., Fodil, S., Azoulay, E., and Zafrani, L. (2020). 'The vascular endothelium: The cornerstone of organ dysfunction in severe SARS-CoV-2 infection. Crit. Care 24, 353. doi:10.1186/s13054-020-03062-7
Puelles, V. G., Lütgehetmann, M., Lindenmeyer, M. T., Sperhake, J. P., Wong, M. N., Allweiss, L., et al. (2020). Multiorgan and renal tropism of SARS-CoV-2. N. Engl. J. Med. 383, 590–592. doi:10.1056/NEJMc2011400
Rahman, S., Montero, M. T. V., Rowe, K., Kirton, R., and Kunik, F. (2021). 'Epidemiology, pathogenesis, clinical presentations, diagnosis and treatment of COVID-19: A review of current evidence. Expert Rev. Clin. Pharmacol. 14, 601–621. doi:10.1080/17512433.2021.1902303
Ramani, A., Müller, L., Ostermann, P. N., Gabriel, E., Abida-Islam, P., Müller-Schiffmann, A., et al. (2020). 'SARS-CoV-2 targets neurons of 3D human brain organoids. Embo J. 39, e106230. doi:10.15252/embj.2020106230
Rhea, E. M., Logsdon, A. F., Hansen, K. M., Williams, L. M., Reed, M. J., Baumann, K. K., et al. (2021). 'The S1 protein of SARS-CoV-2 crosses the blood-brain barrier in mice. Nat. Neurosci. 24, 368–378. doi:10.1038/s41593-020-00771-8
Roy, D., Ghosh, R., Dubey, S., Dubey, M. J., Benito-León, J., and Kanti Ray, B. (2021). Neurological and neuropsychiatric impacts of COVID-19 pandemic. Can. J. Neurol. Sci. 48, 9–24. doi:10.1017/cjn.2020.173
Rutkai, I., Mayer, M. G., Hellmers, L. M., Ning, B., Huang, Z., Monjure, C. J., et al. (2022). 'Neuropathology and virus in brain of SARS-CoV-2 infected non-human primates. Nat. Commun. 13, 1745. doi:10.1038/s41467-022-29440-z
Schimmel, L., Chew, K. Y., Stocks, C. J., Yordanov, T. E., Essebier, P., Kulasinghe, A., et al. (2021). Endothelial cells are not productively infected by SARS-CoV-2. Clin. Transl. Immunol. 10, e1350. doi:10.1002/cti2.1350
Schwabenland, M., Salié, H., Tanevski, J., Killmer, S., Lago, M. S., Schlaak, A. E., et al. (2021). Deep spatial profiling of human COVID-19 brains reveals neuroinflammation with distinct microanatomical microglia-T-cell interactions. Immunity 54, 1594–1610. doi:10.1016/j.immuni.2021.06.002
Serrano, G. E., Walker, J. E., Arce, R., Glass, M. J., Vargas, D., Sue, L. I., et al. (2021). Mapping of SARS-CoV-2 brain invasion and histopathology in COVID-19 disease. medRxiv. doi:10.1101/2021.02.15.21251511
Shehata, G. A., Lord, K. C., Grudzinski, M. C., Elsayed, M., Abdelnaby, R., and Elshabrawy, H. A. (2021). 'Neurological complications of COVID-19: Underlying mechanisms and management. Int. J. Mol. Sci. 22, 4081. doi:10.3390/ijms22084081
Shi, Y., Wang, G., Cai, X. P., Deng, J. W., Zheng, L., Zhu, H. H., et al. (2020). An overview of COVID-19. J. Zhejiang Univ. Sci. B 21, 343–360. doi:10.1631/jzus.B2000083
Solomon, I. H., Normandin, E., Bhattacharyya, S., Mukerji, S. S., Keller, K., Ali, A. S., et al. (2020). Neuropathological features of covid-19. N. Engl. J. Med. 383, 989–992. doi:10.1056/NEJMc2019373
Song, E., Zhang, C., Israelow, B., Lu-Culligan, A., Prado, A. V., Skriabine, S., et al. (2021). 'Neuroinvasion of SARS-CoV-2 in human and mouse brain. J. Exp. Med. 218, e20202135. doi:10.1084/jem.20202135
Tai, W., He, L., Zhang, X., Pu, J., Voronin, D., Jiang, S., et al. (2020). 'Characterization of the receptor-binding domain (RBD) of 2019 novel coronavirus: Implication for development of RBD protein as a viral attachment inhibitor and vaccine. Cell. Mol. Immunol. 17, 613–620. doi:10.1038/s41423-020-0400-4
Taquet, M., Geddes, J. R., Husain, M., Luciano, S., and Harrison, P. J. (2021). '6-month neurological and psychiatric outcomes in 236 379 survivors of COVID-19: A retrospective cohort study using electronic health records. Lancet. Psychiatry 8, 416–427. doi:10.1016/S2215-0366(21)00084-5
Theoharides, T. C., Cholevas, C., Polyzoidis, K., and Politis, A. (2021). 'Long-COVID syndrome-associated brain fog and chemofog: Luteolin to the rescue. Biofactors 47, 232–241. doi:10.1002/biof.1726
Theoharides, T. C. (2022). Could SARS-CoV-2 spike protein Be responsible for long-COVID syndrome? Mol. Neurobiol. 59, 1850–1861. doi:10.1007/s12035-021-02696-0
Torices, S., Cabrera, R., Stangis, M., Naranjo, O., Fattakhov, N., Teglas, T., et al. (2021). 'Expression of SARS-CoV-2-related receptors in cells of the neurovascular unit: Implications for HIV-1 infection. J. Neuroinflammation 18, 167. doi:10.1186/s12974-021-02210-2
Varga, Z., Flammer, A. J., Steiger, P., Haberecker, M., Andermatt, R., Zinkernagel, A. S., et al. (2020). Endothelial cell infection and endotheliitis in COVID-19. Lancet 395, 1417–1418. doi:10.1016/S0140-6736(20)30937-5
Wang, D., Hu, B., Hu, C., Zhu, F., Liu, X., Zhang, J., et al. (2020). Clinical characteristics of 138 hospitalized patients with 2019 novel coronavirus-infected pneumonia in wuhan, China. Jama 323, 1061–1069. doi:10.1001/jama.2020.1585
Wenzel, J., Lampe, J., Müller-Fielitz, H., Schuster, R., Zille, M., Müller, K., et al. (2021). 'The SARS-CoV-2 main protease M(pro) causes microvascular brain pathology by cleaving NEMO in brain endothelial cells. Nat. Neurosci. 24, 1522–1533. doi:10.1038/s41593-021-00926-1
Wiersinga, W. J., Rhodes, A., Cheng, A. C., Peacock, S. J., and Prescott, H. C. (2020). 'Pathophysiology, transmission, diagnosis, and treatment of coronavirus disease 2019 (COVID-19): A review. Jama 324, 782–793. doi:10.1001/jama.2020.12839
Wu, C., Liu, Y., Yang, Y., Zhang, P., Zhong, W., Wang, Y., et al. (2020). 'Analysis of therapeutic targets for SARS-CoV-2 and discovery of potential drugs by computational methods. Acta Pharm. Sin. B 10, 766–788. doi:10.1016/j.apsb.2020.02.008
Yang, A. C., Kern, F., Losada, P. M., Agam, M. R., Maat, C. A., Schmartz, G. P., et al. (2021). 'Dysregulation of brain and choroid plexus cell types in severe COVID-19. Nature 595, 565–571. doi:10.1038/s41586-021-03710-0
Zhang, A. J., Lee, A. C., Chu, H., Chan, J. F., Fan, Z., Li, C., et al. (2021). 'Severe acute respiratory syndrome coronavirus 2 infects and damages the mature and immature olfactory sensory neurons of hamsters. Clin. Infect. Dis. 73, e503–e512. doi:10.1093/cid/ciaa995
Keywords: COVID-19, SARS-CoV-2, blood-brain barrier, olfactory nerve, CNS
Citation: Fujimoto T, Erickson MA and Banks WA (2022) Neurotropism and blood-brain barrier involvement in COVID-19. Front. Drug. Deliv. 2:1073815. doi: 10.3389/fddev.2022.1073815
Received: 18 October 2022; Accepted: 05 December 2022;
Published: 14 December 2022.
Edited by:
Reina Bendayan, University of Toronto, CanadaReviewed by:
Allison Andrews, Temple University, United StatesCopyright © 2022 Fujimoto, Erickson and Banks. This is an open-access article distributed under the terms of the Creative Commons Attribution License (CC BY). The use, distribution or reproduction in other forums is permitted, provided the original author(s) and the copyright owner(s) are credited and that the original publication in this journal is cited, in accordance with accepted academic practice. No use, distribution or reproduction is permitted which does not comply with these terms.
*Correspondence: Takashi Fujimoto, dGZ1amltb0B1dy5lZHU=
Disclaimer: All claims expressed in this article are solely those of the authors and do not necessarily represent those of their affiliated organizations, or those of the publisher, the editors and the reviewers. Any product that may be evaluated in this article or claim that may be made by its manufacturer is not guaranteed or endorsed by the publisher.
Research integrity at Frontiers
Learn more about the work of our research integrity team to safeguard the quality of each article we publish.