- 1Carle Illinois College of Medicine, University of Illinois Urbana–Champaign, Champaign, IL, United States
- 2Chicago Electrical Trauma Rehabilitation Institute, Chicago, IL, United States
- 3The Department of Surgery, University of Chicago, Chicago, IL, United States
This article examines the precision of medical terminology commonly used to diagnose and understand the pathogenesis of electrical shock injuries. As everyday technology increasingly depends on advanced electrical mechanisms that utilize more efficient modes of electrical energy transmission, waveforms, and frequencies, emergency and trauma physicians will continue to encounter a broader array of electrical injury manifestations. This phenomenon prompts a closer examination of the diagnostic terminology associated with electrical shocks. The pathogenesis of electrical injury depends on the tissue electric field strength, frequency, current duration, and tissues involved. Some traditional diagnostic terms, for example, “entry” and “exit” wounds, arc-flash burns, and “high-voltage” and “low-voltage” electrical injuries, obscure the complexity of this pathogenesis, likely impeding medical management and advances in electrical safety science. This article presents the scientific rationale for suggested changes to medical terminology and aims to encourage future refinement.
1 Introduction
Electrical shock occurrence continues to be a significant and likely underreported public health concern that is primarily linked to workplace accidents (1). The severity of injuries resulting from electrical shock ranges from medically inconsequential to life-threatening (2). Despite considerable progress and positive impact of electrical worker safety guidelines, these injuries still account for substantial disability-adjusted life years (1). With new and expanding use of electrical power in every aspect of modern industrialized societies, the risk of adverse human contact and resulting use of emergency medical services can be expected to increase.
The need for consistent terminology is growing as the nature of electrical injuries has evolved due to the increased sophistication and broader range of high-energy electrical devices produced for human use today. Fundamentally, the pathogenesis of electrical shock injuries is multi-physical in nature, involving both thermal and non-thermal mechanisms (3). Non-thermal damage results from direct electric forces acting on tissue structures, whereas thermal damage results from the passing electrical current heating the tissue. The combined tissue structural alterations, reversible or not, are governed by the electrical power dissipation density at any point along the current path. Of course, power dissipation at any point along the current path depends on the tissue properties, the electrical current density, the current frequency, and the duration of exposure (3). Understanding these deterministic parameters provides useful clinical insights into optimal triage and management of electrical power injuries.
2 Fundamental concept 1: significance of electrical current frequency
Although the effect of electrical current frequency is rarely discussed in the trauma medicine literature, it is a fundamental factor governing the damaging effects of current on tissues. The electrical current frequency influences the types of cells and molecules acted on by the electrical forces in the tissue during electrical shock. In addition, the frequency affects the anatomical distribution and path of the current when passing through the body during electrical shock and the current's efficiency in heating tissue water. The range of frequency-dependent biophysical and atomic effects is summarized in Table 1.
For electric shock, the most common presentation in emergency departments is survivors of contact with a standard 50–60-Hz commercial electrical power source. In this frequency range (i.e., <10,000 Hz), the size and shape of cells affect their risk of injury such that peripheral nerve axons and long skeletal muscles are particularly vulnerable to membrane puncture (i.e., electroporation) by the direct action of electrical forces (4). Electroporation is a non-thermal injury mechanism that does not require general heating of tissue water. Electropores can be transient or stable (5). Stable defects are often called “irreversible electroporation” (6). Thus, even brief contact with a 50–60-Hz electrical power source is not long enough to cause thermal damage but can result in nerve and striated muscle injury along the current path (Table 2) (4, 7).
At higher electrical current frequencies, such as radiofrequency or microwave current (i.e., 1–10,000 MHz), resulting tissue injury is primarily electrical burn injury due to tissue water heating (2, 8) wherein cell size and shape are not factors affecting vulnerability to hyperthermia (9). Until recently, human injury resulting from electrical currents in the megahertz range was very unusual and only seen in instances of electrical arc-fault or lightning strike–related injuries. However, in the future, electrical power will likely include more high-frequency electrical power sources and transmission devices, for example, devices widely used for electrical vehicle charging and some military applications (10). Thus, emergency department physicians will likely encounter high-frequency electrical burn injuries more frequently in the future.
An important clinical consideration is that the anatomical spread of the high-frequency current in the body is frequency-dependent due to electromagnetic skin-depth effects (11). Thus, for an electrical shock with a frequency of 10 MHz (i.e., megahertz) or higher, the current passing through the body will be confined to the skin and perhaps subcutaneous tissue. The superficial fern-pattern “Lichtenberg” burns associated with a lightning burn wound is an example of this skin-depth effect (2, 12).
3 Fundamental concept 2: significance of electrical contact voltage
Most clinical reports today describe electrical burn injuries as “high voltage” or “low voltage” as the contact voltage is one of the few ascertainable electrical parameters for individuals presenting to an emergency department after an electrical shock injury (13–15). As a result, these terms have become commonly used to stratify adverse outcome risks for electrical shock injury patients (15). Because this concept has statistical validity, there appears to be a common misunderstanding about the role of voltage per se in determining the extent of injury. Correlating electrical cable caliber to electrical injury severity with the same reliability is also possible because high-voltage electrical cables have a larger caliber than low-voltage electrical wires. However, electrical wire caliber obviously does not determine the extent of tissue injury during electrical shock. In other words, a correlation is not sufficient evidence to attribute causation.
3.1 Can contact voltage alone determine injury risk?
All manufactured electrical power sources have a limit to their capability to generate electrical current. For example, electrical stun guns (i.e., TASERS®) used by law enforcement typically operate at voltages above 25,000 volts (16). However, they do not cause extensive tissue injury because they are only capable of delivering a very small amount of current into the body in short pulses. The electrical power dissipation in the tissue between the stun gun contact points is too small to cause more than microscopic damage adjacent to the contact points. Yet a stun gun shock is a “high-voltage” but low-energy electrical shock.
Voltage (V) is specifically the electrical potential difference that causes electrical charges to move (i.e., current [I]). The power (P) delivered to tissue during electrical shock is what creates tissue damage and is calculated as the product of the voltage times the current (i.e., P = V × I). The work of the tissue injury process during electrical shock is primarily linked to the electrical power dissipation at any point in the current path. Figure 1 provides a mechanical analogy to illustrate this concept. Using terms like high-power electrical injury or low-power electrical injury when characterizing electrical shock injuries would have greater clinical relevance.
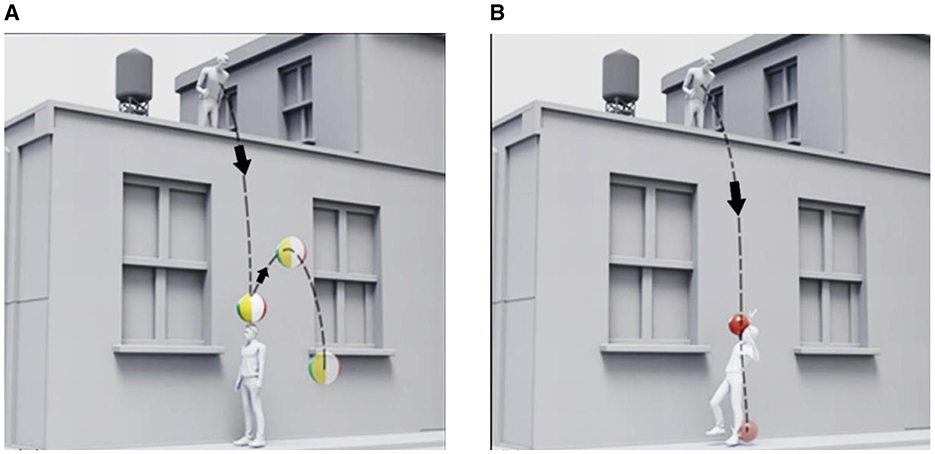
Figure 1. Mechanical trauma analogy: power dissipation governs the magnitude and distribution of tissue injury in physical trauma. In comparison to a beachball (A) dropped from a roof, a bowling ball (B) dropped from the same point will transfer greater energy to the person below and inflict more extensive injury. In addition to the height from which the ball was dropped, the mass of the ball must be known to model the injury magnitude.
4 Fundamental concept 3: existence of “entry” and “exit” wounds?
“Entry” and “exit” wounds are terms given to ballistic wounds resulting from projectiles (i.e., bullets) that have a vector path through the body. The nature, size, and characteristics of bullet wounds provide insight into the mechanics of injury and the bullet's trajectory vector through the body. The wounds caused by a projectile are smaller at the skin entry point because subcutaneous tissue absorbs energy and provides structural support. The wounds are often larger where the projectile leaves the body due to the lack of external mechanical support of the skin at the exit point.
Although the terminology “entry” and “exit” wounds, similar to those shown in Figure 2, is commonly extended to the discussion of electrical shock injuries, it is inconsistent with the actual electrical forces acting on the tissue. Tissues are electrically neutral (17), meaning that, on a scale much greater than the size of a protein, the sum of electrical charges on proteins and other biomolecules plus the mobile ions in solution equals zero. This is referred to as the “bulk electroneutrality condition” of electrochemical systems like biological tissues (17).
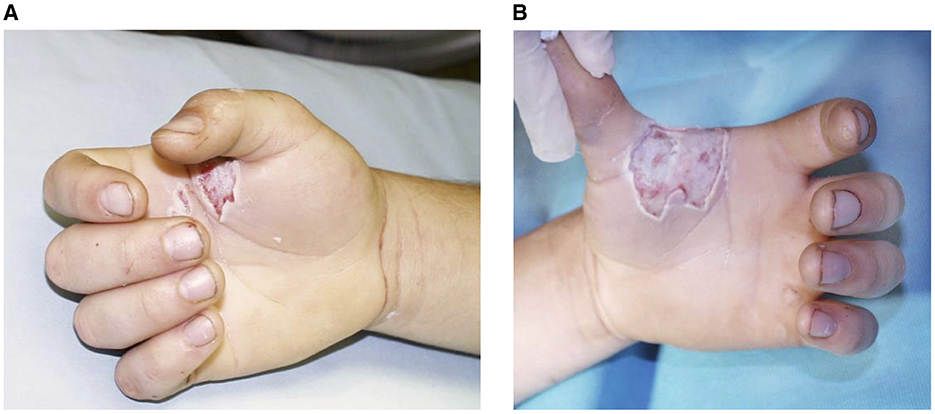
Figure 2. Electrical contact wounds: skin electrical (~408-volt, 60-Hz power frequency) contact wounds resulting from a hand-to-hand electrical shock. Both left-hand (A) and right-hand (B) wounds of shown soon after hospital admission. The exact duration of contact is unknown. The existence of full thickness burns suggests multiple cycles of current passed through the wounds.
This means that an electrical shock current passing through the body is carried by both positive and negative mobile salt ions that must move in opposite directions to conduct the electrical current. Because an equal number of ions are moving in opposite directions, no net vector electrical force can act on the tissue during electrical shock. As such, there can be no ballistic-type entrance or exit effects. There may be differences in the wounds at the contact points due to other contact factors such as the size and geometry of the electrical contacts.
Furthermore, most electrical shocks result from the passage of alternating current (AC; Figure 3). Alternating current oscillates in the direction of the current flow. For 60-Hz electrical power, the current changes direction 120 times per second. Thus, during an AC electrical shock, each skin contact point experiences both current entry and exit. For these reasons, using entry wounds and exit wounds should be abandoned clinical terminology for electrical shock body surface contact wounds.
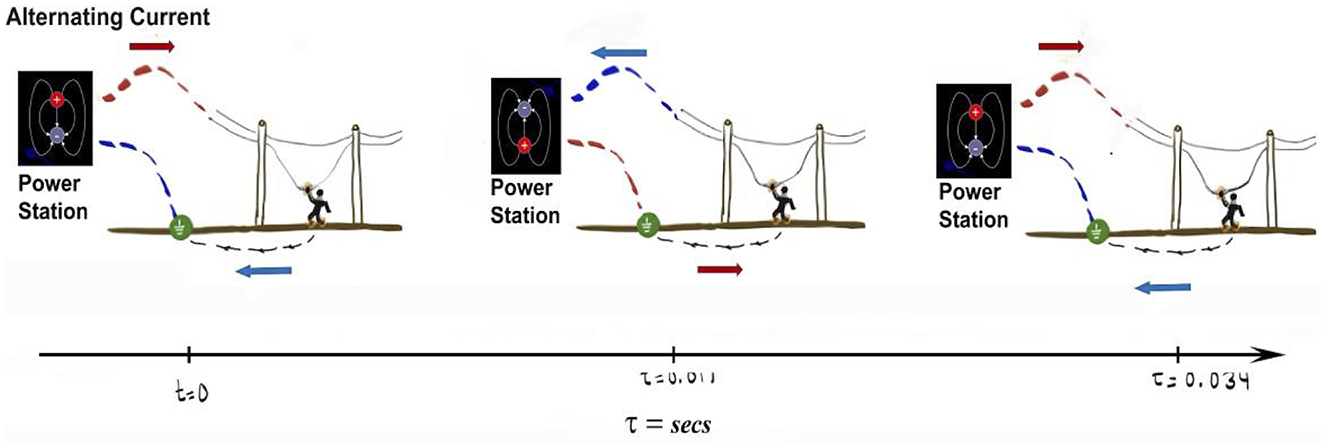
Figure 3. Illustration of alternating current: alternating current is produced by alternating the direction of current passage through the circuit. This illustration shows that the current from the electrical power station is changing directions approximately every 8.4 milliseconds to generate a 60-Hz sine wave. The illustrated person contacting a powerline would have current entering and exiting the same skin contact points multiple times for an electrical shock lasting only 32 milliseconds or more. The arrows indicate the direction electrical current flow into and out of the power station.
5 Fundamental concept 4: electrical arc-flash injuries
Electrical flash injuries or arc-flash burns are terms used to refer to injuries resulting from a person being exposed to electrical arc–mediated currents that typically result from electrical short-circuit faults, electrical switchgear activation, or lightning strikes. Electrical flashes, or arcs, occur when two electrical conductors at different electrical potentials produce a strong enough electric field between them to break down the molecules in the air. This breakdown process generates hot ionized molecules in the air called plasma. The plasma is electrically conductive, meaning that it readily conducts electrical current (18, 19).
Clinically, “electrical flash burns” are often considered thermal burns rather than electrical injuries because the patient did not mechanically contact the electrical power source. Because an electrical arc is a very hot gas, it can rapidly expand; thus, a person close to an arc flash from a high-power industrial electrical device may not only experience pressure-wave “blast” trauma and thermal burns but also receive an electrical shock current.
Lightning strike injuries are a natural form of electrical arc-flash injury. When lightning occurs, a massive electrical current travels through an atmospheric arc from charged clouds to the ground. A person contacted by arc flash will experience an electrical current contact from the electrical current passage along superficial layers of the body (19), a magnetic pulse–induced electrical current throughout the body that can produce neuromuscular effects, as well as shock-wave blast forces associated with the rapidly expanding air. The current's effect may be significant or negligible compared to the heat or blast injury. Any of these forces could result in severe injury or death. Of course, if someone is in contact with an object, such as a fence or tree hit by lightning, the frequency of the current passing through that person changes.
6 Discussion
One controversial and important aspect of emergency medicine is the criteria for post–electrical shock hospital admission for cardiac monitoring when the patient has no clinical or diagnostic signs of cardiac injury when presenting at the emergency department. Several reports have noted the late development of signs or manifestations of cardiac injury despite diagnostic evidence being absent on initial presentation (20, 21). However, extensive, large-scale studies also demonstrate that nearly all electrical injury patients manifesting cardiac abnormalities present in the emergency department with evidence of cardiac injury (22–24). These results suggest that a delayed presentation of cardiac injury is very unusual.
Regarding the risk of electrical shock–mediated cardiac injury presenting at admission to the emergency department, considerable effort has been made to identify risk factors. In particular, contact voltage has been the most investigated risk. Some clinical studies conclude that cardiac injury occurred only in patients who received high-voltage (>1,000-volt) electrical shocks, and therefore, low-voltage shock victims can be safely discharged (22, 23). Other reports conclude that cardiac injury and fatal cardiac arrhythmias (electrocution) are well known to be linked to low-voltage electrical shocks (i.e., electrocution) and may result from low-voltage shocks (24–26).
However, as pointed out by Bailey (27), voltage is only one consideration when it comes to cardiac effects. The deterministic parameters are the induced cardiac electric field by the shock and the duration of exposure. For example, applying a very high-voltage electrical stun gun on the trunk does not generate enough current to cause cardiac injury (28). By comparison, a low-voltage (i.e., <500-volt) hand-to-hand electrical shock can trigger a fatal cardiac arrhythmia by interfering with cardiac electrophysiological dynamics when the skin is moist at the contact point (25, 26).
If the included study population of electrical shock patients in the emergency department is limited to individuals shocked by high-energy industrial or commercial power sources, voltage may be found to be a significant deterministic parameter. But other factors, such as clothing resistance, anatomic position, and anatomical path of the current through the body, are likely to influence the study results.
This article highlights common terminology and principles relevant to electrical shock injury. Electrical injury results from any one or more of several biophysical injury modes, including hyperthermic injury (i.e., “Joule heating”), electroporation injury, and, often, thermoacoustic blast injury associated with electrical arc–mediated pressure waves. For facilitating emergency medicine triage, identifying readily available injury parameters that correlate with injury acuity is an important objective for electrical injury research. Treating clinicians should be aware of basic terminology and principles to facilitate appropriate treatment plans.
Author contributions
SG: Conceptualization, Investigation, Methodology, Writing – original draft, Writing – review & editing. MG: Conceptualization, Investigation, Methodology, Writing – original draft. KM: Project administration, Writing – review & editing. RL: Conceptualization, Funding acquisition, Investigation, Methodology, Project administration, Supervision, Writing – original draft, Writing – review & editing.
Funding
The author(s) declare financial support was received for the research, authorship, and/or publication of this article. The authors acknowledge grant funding from the Exelon Foundation (CETRI0819MM) to the Chicago Electrical Trauma Rehabilitation Institute (RL, principal investigator).
Conflict of interest
The authors declare that the research was conducted in the absence of any commercial or financial relationships that could be construed as a potential conflict of interest.
Publisher's note
All claims expressed in this article are solely those of the authors and do not necessarily represent those of their affiliated organizations, or those of the publisher, the editors and the reviewers. Any product that may be evaluated in this article, or claim that may be made by its manufacturer, is not guaranteed or endorsed by the publisher.
References
1. Campbell RB, Dini DA. Occupational Injuries From Electrical Shock and Arc Flash Events. New York, NY: Springer. (2016).
2. Lee RC. Injury by electrical forces: pathophysiology, manifestations, and therapy. Curr Probl Surg. (1997) 34:677–764. doi: 10.1016/S0011-3840(97)80007-X
3. Lee RC, Zhang D, Hannig J. Biophysical injury mechanisms in electrical shock trauma. Annu Rev Biomed Eng. (2000) 2:477–509. doi: 10.1146/annurev.bioeng.2.1.477
4. Block TA, Aarsvold JN, Matthews KL, Mintzer RA, River LP, Capelli-Schellpfeffer M, et al. The 1995 Lindberg Award. Nonthermally mediated muscle injury and necrosis in electrical trauma. J Burn Care Rehabil. (1995) 16:581–8. doi: 10.1097/00004630-199511000-00004
5. Lee RC, Canaday DJ, Hammer SM. Transient and stable ionic permeabilization of isolated skeletal muscle cells after electrical shock. J Burn Care Rehabil. (1993) 14:528–40. doi: 10.1097/00004630-199309000-00007
6. Edd JF, Davalos RV. Mathematical modeling of irreversible electroporation for treatment planning. Technol Cancer Res Treat. (2007) 6:275–86. doi: 10.1177/153303460700600403
7. Gaylor DC, Prakah-Asante K, Lee RC. Significance of cell size and tissue structure in electrical trauma. J Theor Biol. (1988) 133:223–37. doi: 10.1016/S0022-5193(88)80007-9
8. Cherkasova OP, Serdyukov DS, Nemova EF, Ratushnyak AS, Kucheryavenko AS, Dolganova IN, et al. Cellular effects of terahertz waves. J Biomed Opt. (2021) 26:090902. doi: 10.1117/1.JBO.26.9.090902
9. Bischof JC, He X. Thermal stability of proteins. Ann N Y Acad Sci. (2005) 1066:12–33. doi: 10.1196/annals.1363.003
10. Akhila PM, Devi V. A high frequency resonant EF2 converter for electric vehicle charging. In: 2018 International Conference on Power, Signals, Control and Computation (EPSCICON). Thrissur: IEEE (2018).
11. Lamb H, Glaisher JWL. XIII. On electrical motions in a spherical conductor. Philos Trans Royal Soc London. (1883) 174:519–49. doi: 10.1098/rstl.1883.0013
12. Cherington M, Olson S, Yarnell PR. Lightning and Lichtenberg figures. Injury. (2003) 34:367–71. doi: 10.1016/S0020-1383(02)00313-3
13. Arnoldo BD, Purdue GF, Kowalske K, Helm PA, Burris A, Hunt JL. Electrical injuries: a 20-year review. J Burn Care Rehabil. (2004) 25:479–84. doi: 10.1097/01.BCR.0000144536.22284.5C
14. Shih JG, Shahrokhi S, Jeschke MG. Review of adult electrical burn injury outcomes worldwide: an analysis of low-voltage vs high-voltage electrical injury. J Burn Care Res. (2017) 38:e293–8. doi: 10.1097/BCR.0000000000000373
15. Arnoldo B, Klein M, Gibran NS. Practice guidelines for the management of electrical injuries. J Burn Care Res. (2006) 27:439–47. doi: 10.1097/01.BCR.0000226250.26567.4C
16. Sloane CM, Chan TC, Levine SD, Dunford JV, Neuman T, Vilke GM. Serum troponin I measurement of subjects exposed to the Taser X-26. J Emerg Med. (2008) 35:29–32. doi: 10.1016/j.jemermed.2007.08.073
17. Stell G, Joslin CG. The donnan equilibrium: a theoretical study of the effects of interionic forces. Biophys J. (1986) 50:855–9. doi: 10.1016/S0006-3495(86)83526-3
18. Pauna H, Willms T, Aula M, Echterhof T, Huttula M, Fabritius T, et al. Electric arc length-voltage and conductivity characteristics in a pilot-scale ac electric arc furnace. Metallurg Mater Trans B. (2020) 51:4–51. doi: 10.1007/s11663-020-01859-z
19. Lindford A, Juteau S, Jaks V, Klaas M, Lagus H, Vuola J, et al. Case report: unravelling the mysterious lichtenberg figure skin response in a patient with a high-voltage electrical injury. Front Med. (2021) 8:663807. doi: 10.3389/fmed.2021.663807
20. Ghazal-Asswad A, Holm S, Engström O, Huss F, Lipcsey M, Rudolph Delayed A. Unprovoked, hemodynamic collapse with following asystole in a pediatric patient following a high-voltage injury: a case report and literature review. Pediatr Cardiol. (2022) 43:1163–8. doi: 10.1007/s00246-022-02838-8
21. Arrowsmith J, Usgaocar RP, Dickson WA. Electrical injury and the frequency of cardiac complications. Burns. (1997) 23:576–8. doi: 10.1016/S0305-4179(97)00050-8
22. Pawlik AM, Lampart A, Stephan FP, Bingisser R, Ummenhofer W, Nickel CH. Outcomes of electrical injuries in the emergency department: a 10-year retrospective study. Eur J Emerg Med. (2016) 23:448–54. doi: 10.1097/MEJ.0000000000000283
23. Seyfrydova M, Rokyta R, Rajdl D, Huml Arrhythmias M. and laboratory abnormalities after an electrical accident: a single-center, retrospective study of 333 cases. Clin Res Cardiol. (2023) 112:1835–47. doi: 10.1007/s00392-023-02274-5
24. Jensen PJ, Thomsen PE, Bagger JP, Nørgaard A, Baandrup U. Electrical injury causing ventricular arrhythmias. Br Heart J. (1987) 57:279–83. doi: 10.1136/hrt.57.3.279
25. Ichikawa N. Three hundred forty-nine case studies and their consideration of electrical accidents in Japan. In: 2016 IEEE IAS Electrical Safety Workshop (ESW). Jacksonville, FL: IEEE (2016) p. 1–8.
26. Bernstein T. Electrocution and fires involving 120/240-V appliances. IEEE Trans Indust Appl. (1983) 19:155–159. doi: 10.1109/TIA.1983.4504175
27. Bailey B. Risk factors for cardiac injury following an electrical exposure. Ann Emerg Med. (2007) 50:92. doi: 10.1016/j.annemergmed.2007.01.029
Keywords: electrical injury, voltage, frequency, power, arc-flash, cardiac injury
Citation: Gangidi S, Govande M, McCollum K and Lee RC (2024) Electrical shock injuries: an analysis of voltage, frequency, and contact mode determinants. Front. Disaster Emerg. Med. 2:1477987. doi: 10.3389/femer.2024.1477987
Received: 08 August 2024; Accepted: 22 October 2024;
Published: 19 November 2024.
Edited by:
Benoit Viault, Centre Hospitalier Universitaire de Toulouse, FranceReviewed by:
James Killeen, University of California, San Diego, United StatesJoseph Pompa, University of Florida, United States
Copyright © 2024 Gangidi, Govande, McCollum and Lee. This is an open-access article distributed under the terms of the Creative Commons Attribution License (CC BY). The use, distribution or reproduction in other forums is permitted, provided the original author(s) and the copyright owner(s) are credited and that the original publication in this journal is cited, in accordance with accepted academic practice. No use, distribution or reproduction is permitted which does not comply with these terms.
*Correspondence: Raphael C. Lee, r-lee@uchicago.edu