- 1Physiotherapy Department, Faculty of Allied Health Sciences, Thammasat University, Pathumthani, Thailand
- 2Thammasat University Research Unit for Physical Therapy in Respiratory and Cardiovascular Systems, Thammasat University, Pathumthani, Thailand
- 3Biomedical Electronics and Systems Research Team Assistive Technology and Medical Devices Research Group, National Electronics and Computer Technology Center (NECTEC), Pathumthani, Thailand
Introduction: Virtual reality (VR) exercises are reportedly beneficial as a physical activity tool for health promotion and rehabilitation, and can also help individuals exercise under professional supervision. We developed and investigated the potential feasibility of a VR-based aerobic exercise program using the XBOX ONE console and Kinect sensor with real-time pulse rate monitoring. The VR setting consisted of two-dimensional (2D) environments via computer, laptop, or television screens. In addition, the study investigated the potential feasibility of the VR-based exercise program on hemodynamic response and arterial stiffness in healthy participants of various ages.
Methods: Healthy participants (n = 30) aged > 18 years were enrolled in the VR exercise-based program. All participants were required to wear a polar heart rate (HR) monitor set for moderate-intensity exercise, targeting 40%–59% of their HR reserve. Hemodynamic and arterial stiffness (pulse wave velocity) were noninvasively measured. The Borg scale rate of perceived exertion (RPE) was also assessed.
Results: Following a VR-guided exercise routine, all participants performed moderate-intensity exercise with no adverse health outcomes during or after the exercise. The effects of VR-based aerobic exercise extended beyond enhanced central hemodynamic and arterial stiffness. However, neither hemodynamic nor arterial stiffness showed significant differences before and after the VR exercise, except for a higher RPE response following the exercise program.
Conclusion: VR-based aerobic exercise with pulse rate monitoring is a promising physical activity tool to induce physiological changes and impact dyspnea scales and is also feasible for administration to healthy populations.
Introduction
The World Health Organization (WHO) has recommended that both the general population and individuals with specific health conditions should increase their physical activity through exercise. WHO's reports indicate that a quarter of adults fail to meet the recommended levels of physical activity; it was the third in women and the fourth in men who could not accomplish the recommendation (1). Additionally, people individuals who do not meet the recommended physical activity level reportedly have a 20%–30% increased risk of mortality compared to those who achieve physical activity (1).
To address these challenges and enhance exercise adherence, this study explores the potential of virtual reality (VR) technology. VR enables individuals to immerse themselves in virtual environments and engage with various scenarios. Furthermore, VR has demonstrated the potential to improve the overall exercise experience (2, 3). VR exercise applications offer several advantages, such as real-time feedback and diverse exercise options. These features can help combat motivational challenges and reduce the likelihood of boredom, ultimately enhancing exercise adherence (4, 5). VR programs have applications in healthcare for enhancing physical activity and rehabilitation (6, 7). While VR holds considerable promise in the healthcare sector, its widespread adoption faces challenges due to the high cost of the devices, making them less accessible, especially for individuals requiring supervision from healthcare providers due to health issues.
Exercise plays a role in the prevention of cardiovascular disease by improving endothelial function. Increased nitric oxide and the sheering force of blood flow are mediated through evoked endothelium-dependent vasodilation in blood flow, thereby reducing sympathetic nerve activity (8–10). Collectively, arterial stiffness and the resulting central hemodynamic effects have been linked to adverse cardiovascular events, including coronary artery disease, stroke, and hypertension (11, 12). Pulse wave velocity (PWV) is a non-invasive method used to assess the speed of arterial pressure waves traveling along the aorta and large arteries by measuring arterial stiffness (13, 14) PWV has been widely used as a clinical indicator of cardiovascular risk (15–17), and both carotid-femoral PWV and brachial-ankle PWV (baPWV) are commonly used as predictive techniques. In East Asia, baPWV is widely used in the general population and high-risk populations (18). Consequently, the acute effect of VR-based aerobic exercise on central hemodynamic and peripheral PWV values seems necessary to evaluate the feasibility and possible beneficial or adverse acute effects of exercise. Although the acute effects of VR-based aerobic exercise have received attention, factors contributing to hemodynamic changes and arterial stiffness in different age groups may differ (19, 20). Therefore, the objective of this study was to create and assess the viability of VR-based exercise programs, incorporating real-time heart rate (HR) monitoring within a healthy participant group. We hypothesize that, after performing VR-based aerobic exercise, increased hemodynamic responses and arterial stiffness will be observed in participants.
Materials and methods
Participants
This study aimed to develop VR-based aerobic exercise and explore the hemodynamic changes and arterial stiffness in different ages. This pilot study enrolled 30 participants, with 10 participants in each age group: (1) young adults (18–34 years), (2) middle-aged adults (35–59 years), and (3) older adults (60–75 years) (21). Participants with a history of respiratory exacerbation before 6 months, resting HR greater than 120 beats per min (bpm) (22, 23), crisis hypertension (i.e., >180/120 mmHg) (24), those using assistive devices such as a cane or walker, those having uncontrollable blood glucose (i.e., fasting blood sugar greater than 130 mg/dl) (25), a history of cardiopulmonary stroke, or those with psychiatric conditions diagnosed by a physician were excluded. This study protocol was approved by the Human Research Ethics Committee of Thammasat University (Science), COA No. 076/2565. The Thai Clinical Trials Registry is TCTR20230601005. Informed consent was obtained from all participants involved in the study. Written informed consent has been obtained from the participants to publish this paper.
Development of VR-based aerobic exercise and software development tools
The composition of VR hardware is as follows:
• Mini-PC Android board: Employed for software installation.
• Webcam: A USB camera to capture the participant's images during the exercise.
• Air Mouse G10S (wireless 2.4G) Remote Control.
• Television with HDMI compatibility: Displayed the VR coach and used for on-screen heart rate (HR) monitoring via an HDMI connection.
• Polar Verity Sense (Optical Heart Rate Sensor): A Bluetooth-connected wristband that monitors HR during exercise, with an appropriate range tailored to the user-specific exercise program.
The VR-based aerobic exercise system consisted of an armband HR sensor, web camera, display screen, and Android mini-PC with the required software application. After wearing the HR sensor, the software application guided the user to turn on the device and pair it with the software on the mini-PC via Bluetooth. The software was developed using the Dart programming language (Dart.js) to run on the Android OS. A webcam captured video footage of the user engaged in physical exercise. The video was displayed live on the right side of the display as feedback to allow the user to compare their movement with the guiding video clip playing on the left side of the screen.
The HR monitor was attached to the left upper arm. A real-time HR was displayed on the screen during exercise and the marching intensity was adjusted accordingly using HR monitor reading. The HR data was shown as a transparent time series overlay on the running video. This real-time feedback notified the participant if their HR was too high or too low during the exercise (Figure 1).
The Polar Verity Sense (Polar Electro, Kempele, Finland), functioning as an optical HR monitor armband for VR exercises, has been previously established as reliable. It has shown a high intraclass correlation coefficient and has been deemed valid based on the Concordance Correlation Coefficient (26).
The exercise intensity was set at 40%–59% of the HR reserve, representing a definition of moderate aerobic exercise (27). The aerobic exercise program was tailored and refined based on findings from previous research studies (28, 29). Furthermore, the protocol received endorsement and approval from experts in cardiac rehabilitation, including professionals in physical therapy and physical medicine and rehabilitation. The VR-based aerobic exercise program started with a breathing exercise (i.e., slow breathing exercise) for 3-min, followed by a series of warm-up exercises conducted with the upper limbs for 9-min. A 30-min aerobic exercise with moderate intensity exercise is then performed, followed by a cool-down exercise involving marching for 5-min and upper limbs exercise for 9-min. Finally, breathing exercises are performed to conclude the session. Figure 2 illustrates the VR-based aerobic exercise program.
Procedures
The primary objective of this study was to ascertain the feasibility and safety of VR-based aerobic exercises in healthy participants in different age groups. As part of the assessment, all participants underwent hemodynamic evaluations before and after engaging in VR-based aerobic exercises using PhysioFlow® Enduro TM and VP-1000 Plus. The hemodynamic parameters were assessed by a portable Physioflow® Enduro TM (Manatec Biomedical, PhysioFlow Enduro, Paris, France) which is a non-invasive method employed to detect changes in cardiac output (CO), stroke volume (SV), and HR. It relies on transthoracic bioimpedance as the primary measure of cardiovascular responses. To conduct these measurements, participants had sensors attached to their chest wall and were instructed to sit quietly in a chair for 10-min. Subsequently, CO, SV, and HR were recorded.
Arterial wall stiffness was assessed using the VP-1000 Plus (Omron Healthcare, Japan), and brachial-ankle pulse wave velocity (baPWV) was measured as part of vascular screening. It is worth noting that baPWV is closely linked to carotid-femoral pulse wave velocity (cf PWV) (30) and can serve as a risk indicator for cardiovascular disease (31). To measure baPWV, pressure cuffs on both arms and ankles measure pulse waves of the brachial and posterior tibial arteries while participants were instructed to lie on their backs for a 5-min period. The present study included the right baPWV measurement for analysis,
After switching on the VR hardware, all participants were required to connect the HR monitor above the left elbow. Resting HR was shown on the screen and then the instructor set 40%–59% of heart rate reserve (HRR) for moderate-intensity exercise. This study used an equation (220—age) to determine the age-predicted maximal heart rate (32). The VR exercise protocol proposed aerobic activities started with breathing exercises, then participants followed the VR exercise protocol for 60-min.
Following the 60-min session of VR-based aerobic exercise, participants were allowed a 10-min rest period, after which they were invited to undergo evaluations of hemodynamic changes and vascular stiffness using PhysioFlow® Enduro TM and VP-1000 Plus, respectively. Additionally, the Borg rate of perceived exertion (RPE) was employed to assess dyspnea levels both before and after exercise.
Statistical analysis
The normal distribution of data was assessed using the Shapiro-Wilk test, and a two-way repeated mixed ANOVA was conducted to compare within and between groups (2 × 3). The statistical analysis was performed using IBM SPSS Statistics for Windows version 24.0 software, with p < 0.05 considered as statistically significant.
Results
The three participant groups are detailed in Table 1. The study included 30 healthy participants, with 10 participants in each group. Out of the 30 participants, 20 were female and the average age was 44 years (range 20–75 years). All participants achieved the VR-based aerobic exercise protocol by maintaining a 40%–59% HRR without any adverse outcomes. All participants achieved their expected exercise intensity (40% and 59% of their HRR for moderate intensity exercise; 129–150 bpm for young adults, 113–133 bpm for middle adults, and 104–120 bpm for older adults. During VR-based aerobic exercise, the minimal and maximal HR achieved was 115–143 bpm for young adults, 99–126 bpm for middle adults, and 98–113 for older adults. There were no significant differences in sex and body mass index among three groups of age (i.e., young adults, middle adults, and older adults) in the present study (p = .058 and p = .521, respectively).
The PhysioFlow® device failed to measure CO in seven participants, likely due to poor signal quality or electrode contact failure (three in the middle-aged group and four in the older age group). Accordingly, the SV and CO were analyzed in the remaining 23 participants. The results revealed significant increases in SBP and DBP after performing VR-based exercise program in young adulthood (Δ6 mmHg and Δ3 mmHg, respectively), and high HR values were noted in middle-aged adults (Δ9 bpm). In addition, significant variations were observed in dyspnea scores post-VR exercise, with RPE scores of 6–12 (p < .001), as shown in Table 2.
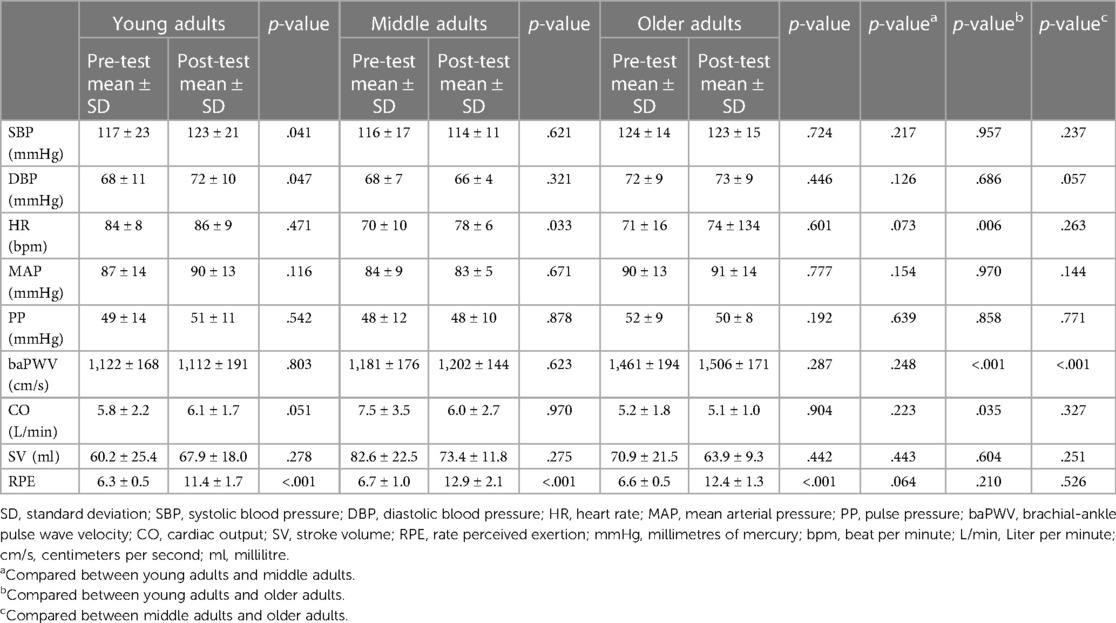
Table 2. Comparison of hemodynamic responses and pulse wave velocity between subgroups of cases based on different aged groups.
Focusing on the age groups, significant differences in baPWV values were exhibited in older adults compared to middle-aged adults and young adults (Δ394 and Δ304 cm/s, respectively). Additionally, it was found that the young adults had high CO values compared to older adults (Δ2.0 L/min).
Discussion
This study aimed to develop and assess the feasibility of VR-based aerobic exercise programs, incorporating real-time HR monitoring within a healthy participant group. We hypothesized that all participants could achieve their expected moderate intensity during VR-based aerobic exercise and that, there would be increased hemodynamic responses and arterial stiffness observed in healthy populations after performing the VR-based aerobic exercise.
Immersive VR is kinesthetic engagement in performing an exercise that can be immersion in the virtual world (33). The prototype of a VR-based exercise device in the present study allows a user to interact with virtual environments and it offers a low-cost VR. In addition, the development of VR-based aerobic exercises has been successfully studied in healthy participants, and no adverse events were observed in this study. Initially, all participants presented increased HR, SBP, DBP, MAP, PP, PWV, and RPE while practicing the VR-based aerobic exercise program. Additionally, all the participants achieved the target HR through moderate-intensity training provided through the VR program. Regarding the safety of a device that affects hemodynamic changes during exercise, none of the measured parameters, such as SBP, DBP, HR, CO, SV, baPWV values, or RPE, exceeded the exercise termination criteria established by the American College of Sports Medicine (ACSM) (27). The present study found that these parameters showed a modest increase 10-min after exercise. A systematic review and meta-analysis reported that exercise acutely elevates PWV, which peaks immediately after exercise and subsequently diminishes 30-min after exercise (34). In the present study, the mean values pre-exercise ranged from 1,122–1,461 cm/s, and the mean values post-exercise ranged from 1,112–1,506 cm/s. The normal values of baPWV ranged from 1,200–1,630 cm/s in individuals aged between 15 and 88 years old (35). Therefore, the increased baPWV after performing VR based aerobic exercise program is not higher than the normal range of baPWV range. Additionally, we observed a slightly elevated SBP, DBP, and HR after performing a VR-based exercise program for 10-min. Notably, these averages in SBP and DBP are considered normal by the American Heart Association (36). Regarding the subjective assessment, dyspnea scores were assessed before the exercise program and ranged from no exertion at all to extremely light (RPE scale = 6–7). These scores were reevaluated after exercise and ranged from light to somewhat hard (RPE scale = 11–13) (37). Alternatively, the ratings of perceived exertion were significantly greater during VR-based aerobic exercise in healthy participants. Therefore, the perceived exertion or breathlessness experienced during VR exercise, which is a subjective measure, could significantly influence individual responses to the VR exercise, and this may be a sufficient tool for healthy people to enhance cardiovascular responses to exercise.
For the effects of VR exercise on health outcomes, a recent systematic review and meta-analysis of 15 studies indicated a positive effect of VR on physiological (e.g., increases in balance, performance) and psychological (e.g., decreased depression, fatigue) health, and no study reported the negative effects on health in VR exercise (38). Furthermore, VR exercise improves cardiovascular performances such as HR, and blood pressure in patients with heart disease, and patients with respiratory problems (39, 40). Therefore, these findings suggest that VR-based aerobic exercise can help improve cardiovascular response and safety. Consequently, VR-based aerobic exercise might be optimal for enhancing hemodynamic responses to exercise in healthy participants, irrespective of their age group.
Despite the significant insights uncovered, this study also had some limitations. This study primarily focused on the immediate hemodynamic and perceived exertion responses to VR-based aerobic exercise. Long-term effects on health outcomes or safety were not assessed. It is important to conduct follow-up studies to determine if the positive effects observed in this study are sustained over time and if any potential adverse effects emerge with prolonged use. In addition, different sports recommend different heart rate monitors. The Polar Verity Sense Optical Heart Rate Sensor is recommended for swimming; therefore, the Polar H10 Heart Rate Sensor might be beneficial for overall activities (41). Additionally, we did not calculate the sample size, and only healthy participants were recruited because this study aimed to develop and explore the feasibility of a VR device. Therefore, further studies are needed with a sufficient sample size and the clinical application of VR-based aerobic exercise in various populations, including those with heart disease or those who have undergone cardiac surgery.
Conclusions
VR-based aerobic exercise in healthy participants was associated with high acceptance and no adverse events. Future research endeavors must encompass a broader scope, encompassing diverse patient demographics, protracted observation periods, and comparative analyses, to comprehensively elucidate the therapeutic potential and safety parameters associated with this innovative exercise modality.
Data availability statement
The original contributions presented in the study are included in the article/Supplementary Material, further inquiries can be directed to the corresponding author.
Ethics statement
The studies involving humans were approved by Human Research Ethics Committee of Thammasat University (Science), COA No. 076/2565. The studies were conducted in accordance with the local legislation and institutional requirements. Written informed consent for participation in this study was provided by the participants’ legal guardians/next of kin.
Author contributions
KY: Conceptualization, Formal Analysis, Funding acquisition, Methodology, Project administration, Resources, Supervision, Writing – original draft, Writing – review & editing. NS: Data curation, Investigation, Writing – original draft. PS: Formal Analysis, Writing – original draft. NC: Formal Analysis, Writing – original draft. SB: Formal Analysis, Writing – original draft. CB: Investigation, Software, Writing – original draft. CT: Software, Validation, Writing – original draft, Writing – review & editing.
Funding
The author(s) declare financial support was received for the research, authorship, and/or publication of this article.
This research project was supported by the Thailand Science Research and Innovation Fundamental Fund fiscal year 2024, Thammasat University.
Acknowledgments
The authors would like to thank all patients for participating in the study and the Ufam Clinique Company Limited for supporting our research. The authors also thank the Thammasat University Research Unit for Physical Therapy in Respiratory and Cardiovascular Systems, Thammasat University, Pathumthani, Thailand.
Conflict of interest
The authors declare that the research was conducted in the absence of any commercial or financial relationships that could be construed as a potential conflict of interest.
Publisher's note
All claims expressed in this article are solely those of the authors and do not necessarily represent those of their affiliated organizations, or those of the publisher, the editors and the reviewers. Any product that may be evaluated in this article, or claim that may be made by its manufacturer, is not guaranteed or endorsed by the publisher.
References
1. Fletcher GF, Landolfo C, Niebauer J, Ozemek C, Arena R, Lavie CJ. Promoting physical activity and exercise: JACC health promotion series. J Am Coll Cardiol. (2018) 72:1622–39. doi: 10.1016/j.jacc.2018.08.2141
2. Gao Z, Lee JE, McDonough DJ, Albers C. Virtual reality exercise as a coping strategy for health and wellness promotion in older adults during the COVID-19 pandemic. J Clin Med. (2020) 9:1986. doi: 10.3390/jcm9061986
3. Giakoni-Ramirez F, Godoy-Cumillaf A, Espoz-Lazo S, Duclos-Bastias D. Martin PDL physical activity in immersive virtual reality: a scoping review. Healthcare. (2023) 11(11):1553. doi: 10.3390/healthcare11111553
4. Mouatt B, Smith AE, Mellow ML, Parfitt G, Smith RT, Stanton TR. The use of virtual reality to influence motivation, affect, enjoyment, and engagement during exercise: a scoping review. Front Virtual Real. (2020) 1:564664. doi: 10.3389/frvir.2020.564664
5. Jo H, Park DH. Affordance, usefulness, enjoyment, and aesthetics in sustaining virtual reality engagement. Sci Rep. (2023) 13(1):15097. doi: 10.1038/s41598-023-42113-1
6. Asadzadeh A, Samad-Soltani T, Salahzadeh Z, Rezaei-Hachesu P. Effectiveness of virtual reality-based exercise therapy in rehabilitation: a scoping review. Inform Med Unlocked. (2021) 24:100562. doi: 10.1016/j.imu.2021.100562
7. Karamians R, Proffitt R, Kline D, Gauthier LV. Effectiveness of virtual reality- and gaming-based interventions for upper extremity rehabilitation poststroke: a meta-analysis. Arch Phys Med Rehabil. (2020) 101(5):885–96. doi: 10.1016/j.apmr.2019.10.195
8. Green DJ, Smith KJ. Effects of exercise on vascular function, structure, and health in humans. Cold Spring Harb Perspect Med. (2018) 8(4):a029819. doi: 10.1101/cshperspect.a029819
9. Higashi Y, Yoshizumi M. Exercise and endothelial function: role of endothelium-derived nitric oxide and oxidative stress in healthy subjects and hypertensive patients. Pharmacol Ther. (2004) 102(1):87–96. doi: 10.1016/j.pharmthera.2004.02.003
10. Sakellariou XM, Papafaklis MI, Domouzoglou EM, Katsouras CS, Michalis LK, Naka KK. Exercise-mediated adaptations in vascular function and structure: beneficial effects in coronary artery disease. World J Cardiol. (2021) 13(9):399–415. doi: 10.4330/wjc.v13.i9.399
11. Palatini P, Casiglia E, Gąsowski J, Głuszek J, Jankowski P, Narkiewicz K. Arterial stiffness, central hemodynamics, and cardiovascular risk in hypertension. Vasc Health Risk Manag. (2011) 7:725–39. doi: 10.2147/VHRM.S25270
12. Shirwany NA, Zou MH. Arterial stiffness: a brief review. Acta Pharmacol Sin. (2010) 31(10):1267–76. doi: 10.1038/aps.2010.123
13. Kim HL, Kim SH. Pulse wave velocity in atherosclerosis. Front Cardiovasc Med. (2019) 6:41. doi: 10.3389/fcvm.2019.00041
14. Davies JI, Struthers AD. Pulse wave analysis and pulse wave velocity: a critical review of their strengths and weaknesses. J Hypertens. (2003) 21(3):463–72. doi: 10.1097/00004872-200303000-00004
15. Yu WC, Chuang SY, Lin YP, Chen CH. Brachial-ankle vs carotid-femoral pulse wave velocity as a determinant of cardiovascular structure and function. J Hum Hypertens. (2008) 22:24–31. doi: 10.1038/sj.jhh.1002259
16. Mancia G, Fagard R, Narkiewicz K, Redon J, Zanchetti A, Bohm M, et al. 2013 ESH/ESC guidelines for the management of arterial hypertension: the task force for the management of arterial hypertension of the European society of hypertension (ESH) and of the European Society of Cardiology (ESC). J Hypertens. (2013) 31:1281–357. doi: 10.1097/01.hjh.0000431740.32696.cc
17. Townsend RR, Wilkinson IB, Schiffrin EL, Avolio AP, Chirinos JA, Cockcroft JR, et al. American Heart association council on hypertension. Recommendations for improving and standardizing vascular research on arterial stiffness: a scientific statement from the American heart association. Hypertension. (2015) 66:698–722. doi: 10.1161/HYP.0000000000000033
18. He D, Gao L, Yang Y, Jia J, Jiang Y, Sun P, et al. Brachial-ankle pulse wave velocity as a measurement for increased carotid intima-media thickness: a comparison with carotid-femoral pulse wave velocity in a Chinese community-based cohort. J Clin Hypertens (Greenwich). (2022) 24(4):409–17. doi: 10.1111/jch.14448
19. Wolsk E, Bakkestrøm R, Thomsen JH, Balling L, Andersen MJ, Dahl JS, et al. The influence of age on hemodynamic parameters during rest and exercise in healthy individuals. JACC Heart Fail. (2017) 5(5):337–46. doi: 10.1016/j.jchf.2016.10.012
20. Koshiba H, Maeshima E. Effects of exercise intervention on arterial stiffness in middle-aged and older females: evaluation by measuring brachial-ankle pulse wave velocity and cardio-ankle vascular index. J Phys Ther Sci. (2019) 31(1):88–92. doi: 10.1589/jpts.31.88
21. Petry NM. A comparison of young, middle-aged, and older adult treatment-seeking pathological gamblers. Gerontologist. (2002) 42(1):92–9. doi: 10.1093/geront/42.1.92
22. Zhabyeyev P, Oudit GY. Mechanisms of cardiac arrhythmias: molecular and cellular perspective. In: Ramachandran S, Douglas V, Sawyer B, editors. Encyclopedia of Cardiovascular Research and Medicine. Amsterdam: Elsevier (2018). p. 316–27. ISBN 97801280515
23. Anderson KP, DeCamilla J, Moss AJ. Clinical significance of ventricular tachycardia (3 beats or longer) detected during ambulatory monitoring after myocardial infarction. Circulation. (1978) 57(5):890–7. doi: 10.1161/01.cir.57.5.890
24. Whelton PK, Carey RM, Aronow WS, Casey DE Jr, Collins KJ, Dennison Himmelfarb C, et al. 2017 ACC/AHA/AAPA/ABC/ACPM/AGS/APhA/ASH/ASPC/NMA/PCNA guideline for the prevention, detection, evaluation, and management of high blood pressure in adults: executive summary: a report of the American College of Cardiology/American Heart Association Task Force on clinical practice guidelines. Hypertension. (2018) 71(6):1269–324. doi: 10.1161/HYP.0000000000000066
25. American Diabetes Association. Standards of medical care in diabetes–2006. Diabetes Care. (2006) 29(Supplement 1):S4–42. doi: 10.2337/diacare.29.s1.06.s4
26. Navalta JW, Davis DW, Malek EM, Carrier B, Bodell NG, Manning JW, et al. Heart rate processing algorithms and exercise duration on reliability and validity decisions in biceps-worn polar verity sense and OH1 wearables. Sci Rep. (2023) 13:11736. doi: 10.1038/s41598-023-38329-w
27. American College of Sports Medicine. ACSM’s Guideline for Exercise Testing and Prescription, 10th ed. Philadelphia, PA: Lippincott Williams & Wilkins (2018).
28. Lima HJ, Souza RDSP, Santos ASMSE, Borges DL, Guimaraes ARF, Ferreira GVDBA, et al. Virtual reality on pulmonary function and functional independence after coronary artery bypass grafting: clinical trial. Am J Cardiovasc Dis. (2020) 10:499–505. PMID: 33224601; PMCID: PMC767515733224601
29. García-Bravo S, Cano-de-la-Cuerda R, Domínguez-Paniagua J, Campuzano-Ruiz R, Barreñada-Copete E, López-Navas MJ, et al. Effects of virtual reality on cardiac rehabilitation programs for ischemic heart disease: a randomized pilot clinical trial. Int J Environ Res Public Health. (2020) 17(22):8472. doi: 10.3390/ijerph17228472
30. Munakata M, Ito N, Nunokawa T, Yoshinaga K. Utility of automated brachial ankle pulse wave velocity measurements in hypertensive patients. Am J Hypertens. (2003) 16:653–7. doi: 10.1016/S0895-7061(03)00918-X
31. Koji Y, Tomiyama H, Ichihashi H, Nagae T, Tanaka N, Takazawa K. Comparison of ankle-brachial pressure index and pulse wave velocity as markers of the presence of coronary artery disease in subjects with a high risk of atherosclerotic cardiovascular disease. Am J Cardiol. (2004) 94(7):868–72. doi: 10.1016/j.amjcard.2004.06.020
32. Fox SM, Naughton JP, Haskell WL. Physical activity and the prevention of coronary heart disease. Ann Clin Res. (1971) 3(6):404–32. PMID: 5243890; PMCID: PMC17502984945367
33. Kiper P, Przysiężna E, Cieślik B, Broniec-Siekaniec K, Kucińska A, Szczygieł J, et al. Effects of immersive virtual therapy as a method supporting recovery of depressive symptoms in post-stroke rehabilitation: randomized controlled trial. Clin Interv Aging. (2022) 17:1673–85. doi: 10.2147/CIA.S375754
34. Saz-Lara A, Cavero-Redondo I, Alvarez-Bueno C, Notario-Pacheco B, Ruiz-Grao MC, Martinez-Vizcaino VJ. The acute effect of exercise on arterial stiffness in healthy subjects: a meta-analysis. Clin Med. (2021) 10:291. doi: 10.3390/jcm10020291
35. Yiming G, Zhou X, Lv W, Peng Y, Zhang W, Cheng X, et al. Reference values of brachial-ankle pulse wave velocity according to age and blood pressure in a Central Asia population. PLoS One. (2017) 12(4):e0171737. doi: 10.1371/journal.pone.0171737
36. Lee H, Yano Y, Cho SMJ, Park JH, Park S, Lloyd-Jones DM, et al. Cardiovascular risk of isolated systolic or diastolic hypertension in young adults. Circulation. (2020) 141:1778–86. doi: 10.1161/CIRCULATIONAHA.119.044838
38. Qian J, McDonough DJ, Gao Z. The effectiveness of virtual reality exercise on individual’s physiological, psychological and rehabilitative outcomes: a systematic review. Int J Environ Res Public Health. (2020) 17:4133. doi: 10.3390/ijerph17114133
39. Mediano MF, Mendes Fde S, Pinto VL, Silva GM, Silva PS, Carneiro FM, et al. Cardiac rehabilitation program in patients with chagas heart failure: a single-arm pilot study. Rev Soc Bras Med Trop. (2016) 49(3):319–28. doi: 10.1590/0037-8682-0083-2016
40. Ribeiro CM, Gomes R, Monteiro C, Dias RM, Simcsik AO, Araújo L, et al. Heart rate variability during virtual reality activity in individuals after hospitalization for COVID-19: a cross-sectional control study. Electronics (Basel). (2023) 12(8):1925. doi: 10.3390/electronics12081925
Keywords: virtual reality, exercise therapy, health promotion, rehabilitation, hemodynamics, pulse wave velocity, dyspnea
Citation: Yuenyongchaiwat K, Sermsinsathong N, Songsorn P, Charususin N, Buranapuntalug S, Buekban C and Thanawattano C (2024) Development and feasibility assessment of a virtual reality-based aerobic exercise program with real-time pulse rate monitoring on hemodynamic and arterial stiffness in healthy people: a pilot study. Front. Digit. Health 6:1356837. doi: 10.3389/fdgth.2024.1356837
Received: 16 December 2023; Accepted: 25 March 2024;
Published: 8 April 2024.
Edited by:
Yasumasa Okada, Murayama Medical Center (NHO), JapanReviewed by:
Masahiro Horiuchi, National Institute of Fitness and Sports in Kanoya, JapanRobert Gajda, Grupa Gajda-Med. Medical Center, Poland
© 2024 Yuenyongchaiwat, Sermsinsathong, Songsorn, Charususin, Buranapuntalug, Buekban and Thanawattano. This is an open-access article distributed under the terms of the Creative Commons Attribution License (CC BY). The use, distribution or reproduction in other forums is permitted, provided the original author(s) and the copyright owner(s) are credited and that the original publication in this journal is cited, in accordance with accepted academic practice. No use, distribution or reproduction is permitted which does not comply with these terms.
*Correspondence: Kornanong Yuenyongchaiwat ykornano@tu.ac.th