- 1School of Psychology, University of Roehampton, London, United Kingdom
- 2School of Life and Health Sciences, University of Roehampton, London, United Kingdom
- 3Reckitt/Mead Johnson Nutrition, Slough, United Kingdom
- 4School of Psychology and Clinical Language Sciences, University of Reading, Reading, United Kingdom
Background: It is well known that having maladaptive emotion regulation skills during childhood may predict mental health issues later in life, therefore, establishing links with gut microbiome could help develop gut-derived interventions directed at improving maladaptive emotion regulation skills during this critical developmental period. While differences in gut microbiome diversity and composition have been associated with several mental health problems in adults and in infants, the current study is the first one to examine whether the gut microbiome diversity and composition are related to emotional regulation abilities during early childhood.
Method: In the current cross-sectional study, mothers of seventy-three children aged 2–6 years completed several questionnaires assessing their child's and their own emotion regulation skills and mental health, provided a stool sample from their child (via at-home gut microbiome testing kits), and reported on their child's dietary intake.
Results: Results revealed that compared to children in the low maladaptive emotion regulation group, alpha diversity, relative abundance of butyrate-producing genera (Butyricicoccus and Odoribacter), and vitamin synthesis scores (Vitamins B2, B3, B6, and B9) were significantly lower in the high maladaptive emotion regulation group.
Discussion: These findings suggest that maladaptive emotion regulation skills link to gut microbiome alterations in early childhood, a crucial time of both brain and gut-immune development, hence, may open the way to the development of early novel (dietary or pre/probiotic) interventions.
1 Introduction
The relationship between the gut microbiome and brain health has gained popularity over recent years, spurred by an increased understanding of the key role microbiome may play in mediating the bidirectional communication between the gut and the brain (i.e., the microbiome gut-brain axis [MGBA]) (Cryan and Dinan, 2012; Allen et al., 2017; Foster et al., 2017; Cryan et al., 2019). It has now been well-accepted that several environmental factors such as traumatic/chronic stress can cause disruptions in the gut microbiome (Leigh et al., 2023), on the other hand, altered gut microbiome can induce changes in behavior and cognition (Cryan et al., 2019).
Of specific importance, increasing evidence is showing the impact of the microbiota in regulating mood, behavior, and cognitive function (Cryan and Dinan, 2012; Allen et al., 2017; Foster et al., 2017; Cryan et al., 2019) and on a variety of psychological disorders such as anxiety (Jiang et al., 2018), depression (Jiang et al., 2015), schizophrenia (Li et al., 2021), bipolar disorder (Evans et al., 2017), neurodevelopmental conditions such as autism spectrum disorder (Kelly et al., 2017; Garcia-Gutierrez et al., 2020), and attention deficit hyperactivity disorder (Boonchooduang et al., 2020). These psychopathologies are known to be promoted by a range of factors, including cognitive impairments in attentional control (Eysenck et al., 2007; Derakhshan, 2020) and difficulties in emotion regulation (Cisler et al., 2010; Compare et al., 2014). Notably, emotion dysregulation (i.e., difficulties in self-regulating one's own negative emotions) have been repeatedly shown to be related to the development of a myriad of mental health disorders including major depressive disorder, generalized anxiety disorder, eating, and alcohol related disorders (Aldao et al., 2010).
It is believed that the MGBA plays a role in regulating emotion, possibly via the MGBA-related alterations in the expression of receptors for the neurotransmitter GABA and/or glutamate (Cryan and Dinan, 2012; Varesi et al., 2023) and/or through biosynthesis of pantothenate, coenzyme A, and adenosine (Ke et al., 2022). Up till now, only one study has shown that better emotion regulation skills (i.e. greater use of reappraisal, and lower use of suppression) were associated with distinct compositional profiles of the gut microbiome at the species level in healthy women (Ke et al., 2022). Specifically, their findings revealed that Bacteroides xylanisolvens, F. bacterium CAG 95, and Parabacteroides distasonis were correlated with higher levels of cognitive reappraisal but lower levels of suppression. Moreover, cognitive reappraisal was associated with lower abundance of Anaeromassilibacillus sp. An250, Bacteroides faecis, Blautia hydrogenotrophica, Clostridium bolteae CAG 59, Clostridium leptum, F. bacterium CAG 94, R. bacterium D16, Sellimonas intestinalis, and Streptococcus parasanguinis, while suppression was associated with higher abundance of these species.
Importantly, to date, only a few behavioral and neural outcomes (e.g., temperament, cognitive and brain function) have been linked to the MGBA in infants—albeit inconsistently (Alving-Jessep et al., 2022). For instance, in newborn infants (mean age = 25 days), negative emotionality and regulation/orienting were marked by an enrichment of Bifidobacterium (Kelsey et al., 2021). Whereas, in 2.5- month-old infants, increased abundance of Bifidobacterium and Streptococcus were linked to higher positive emotionality, and higher diversity were linked to negative emotionality (Aatsinki et al., 2019). Similarly, Bifidobacterium was positively correlated with “soothability” and Hungatella was negatively correlated with “cuddliness” in 12-month-old infants (Wang et al., 2020), while higher surgency (positive emotionality) was associated with a more diverse gut microbiome in toddlers aged between 18–27 months (Christian et al., 2015). Recent longitudinal evidence has shown that Bifidobacterium and Lachnospiraceae abundance at 1–3 weeks of age was positively associated with surgency/extraversion at 12 months of age (Fox et al., 2022); Prevotella abundance at 12 months of age was negatively associated with internalizing problems at 2 years of age (Loughman et al., 2020), highlighting the role of the infant gut microbiota for neurodevelopment and mental health in later life. However, no clear evidence of associations between gut microbiome diversity (as well as taxonomies or functions) and mental health problems were found at 10 years of age (Kraaij et al., 2023).
Within this rapidly expanding evidence base, key gaps remain. First of all, current studies have been based almost entirely on either infants or school-aged children, even though psychiatric disorders typically manifest earlier in childhood as emotional and behavioral problems (Solmi et al., 2022). The only exception to this is a study by Flannery et al. (2020), who recruited 5-to-7-year-olds with a range of adverse experiences and caregiver stressors and relationships, and found that Bacteroides fragilis was associated with reduced levels of aggressive behavior, emotional reactivity, externalizing behavior, sadness, and impulsivity, as well as an increased inhibitory control. As such, it remains unclear whether associations reported in other published studies are evident during early childhood in healthy samples. Additionally, it appears that mother's mental wellbeing plays interdependent role and may impede the child's emotion regulation (Morelen et al., 2016), as previous research has repeatedly shown associations between maternal mental health and emotion regulation and children's emotion regulation abilities (Zimmer-Gembeck et al., 2022). However, apart from Querdasi et al. (2023) controlling for prenatal and preconception adversity by measuring childhood trauma and anxiety in mothers, no research has accounted for parental mental health while assessing the association between microbial and behavioral/temperamental outcomes of the offspring.
To address these gaps, in the current study, our aim was to examine cross-sectional associations between the gut microbiome (diversity and composition) and emotion regulation in 2-to-6-year-old children, while accounting for maternal mental health, as well as other known and potential confounds.
2 Methods
2.1 Participants and study design
One hundred and eleven mothers with 2-to-6-year-old children responded to online advertisements and completed an online survey (delivered on Qualtrics; https://www.qualtrics.com) assessing their child's and their own demographic and clinical outcomes (please see Section 2.2. Behavioral Outcomes for details). Out of the initial sample of participants, only 81 mothers agreed to provide their child's stool sample and were sent at-home microbiome testing kits. However, only 73 kits were returned for stool analysis. Therefore, the final sample consisted of 73 participants. By using the Emotion Regulation Skills Questionnaire parent form (ERSQ) (Mirabile, 2014), Low (n = 37) and High ( = n = 36) Maladaptive Emotion Regulation (MA ER) groups were established based on median splits.
Children were excluded if they had a significant acute or chronic co-existing illness (including functional gastrointestinal disorders, inflammatory bowel disease, coeliac disease, lactose intolerance etc.), immunological, psychiatric, neurodevelopmental disorders, and metabolic disorders. Additionally, children were not included if they had taken any prebiotic or probiotic supplements or antibiotics 4 weeks prior to enrolment in the study. Mothers received £60 for participation. All participants provided informed consent. The study was conducted in accordance with the Declaration of Helsinki, and approved by the Ethical Committee at the University of Roehampton (Reference: PSYC 23/452).
2.2 Behavioral outcomes
All mothers completed a demographics form (developed in-house) that assessed their child's age, sex, birth, current weight and height, number of siblings, living arrangements (i.e., who they are living with), physical activity [via Parent-reported Physical Activity and Sedentary Time in Young Children Questionnaire (Sarker et al., 2015)], stay in neonatal intensive care >24 h, breastfeeding status at some point, delivery type, as well as their own age, weight and height, education, ethnicity, employment, age during delivery, and relationship to child, as well as household income. These measures were used to ensure that High and Low MA ER groups did not differ for these demographic and environmental/lifestyle factors.
Emotion Regulation Skills Questionnaire (ERSQ) (Mirabile, 2014), a 52-item questionnaire, that measures child's maladaptive and adaptive ER skills was used. Mothers rated each item on a 5-point Likert scale by the mothers (from 0 = never to 4 = almost always), and scores averaged to create component indicators of adaptive (consists of children's self-directed speech, instrumental coping, information gathering, social distraction, object distraction, self-soothing, comfort seeking, and support seeking strategies) and maladaptive (consists of children's focus on the distressing object, venting, aggression, avoidance, and suppression strategies) ER. In the current paper, MA ER subscale scores were used. The ERSQ MA ER subscale demonstrates good internal consistency (Cronbach's alpha ranging from 0.61 to 0.85) (Mirabile and Thompson, 2011; Mirabile, 2014).
Beck Depression Inventory (BDI) (Beck et al., 1996), a 21-item questionnaire, was used to assess maternal depression. Each item was rated on a 5-point Likert scale (from 0 = not at all to 4 = severely). A score of 0–13 indicate none or minimal depression, 14–19 mild depression, 20–28 moderate depression, and 29–63 severe depression. The BDI demonstrates good internal consistency (Cronbach's alpha of 0.86 and 0.81 for clinical and subclinical samples, respectively) (Beck et al., 1988).
Spielberger State Trait Anxiety Inventory (STAI) (Spielberger et al., 1983), a 40-item measure of trait and state anxiety, was used to assess maternal anxiety. Each item was rated on a 5-point Likert scale (from 1 = not at all to 4 = very much so) and scores range from 20- to 80, where higher scores denote severe levels of anxiety. The STAI demonstrates good internal consistency (Cronbach's alpha), ranging from 0.86 to 0.95 (Spielberger et al., 1983).
Difficulties in Emotion Regulation Scale (DERS) (Gratz and Roemer, 2004), a 36-item questionnaire, was used to measure maternal emotional regulation skills. Each item was rated on a 5-point Likert scale (from 1 = almost never to 4 = almost always). This measure comprises of 6 subscales: lack of emotional awareness, lack of emotional clarity, limited access to emotion regulation strategies, non-acceptance of emotional responses, difficulty in engaging in goal-directed behaviors and difficulties in controlling impulses. All items are summed to create a total score, with higher scores reflecting greater difficulties in emotion regulation. The DERS demonstrates good internal consistency (Cronbach's alpha) of 0.93 (Gratz and Roemer, 2004).
2.3 Fecal sample collection and pre-processing
A few days after completing the online questionnaires, mothers were sent at-home gut microbiome testing kits. Microbiome sample collection was performed using the Omnigene Gut OM-200 kit (DNA Genotek, Ottawa, CA), provided by Atlas Biomed in ISO 13485:2016-accredited packaging (medical device). The OM-200 kit facilitated the DNA extraction and stabilization (Chen et al., 2020) prior to being sequenced by the Illumina MiSeq platform (Caporaso et al., 2012; Ravi et al., 2018). The raw data were then analyzed using the Deblur algorithm (Amir et al., 2017). The taxonomic classification of the denoised reads was performed with the QIIME2 Naive-Bayes classifier (Bolyen et al., 2019). The classifier was trained on a 16S rRNA sequence database (Greengenes database v.13.5) (DeSantis et al., 2006). ASVs (amplicon sequencing variants) detected in negative control samples that were known to be skin or environmental dwellers were removed from the analysis. For alpha-diversity analysis, the classified reads were randomly rarefied to the same number (3,000 reads per sample), for each sample. Estimation of alpha-diversity for each sample was performed using the Shannon Index (Shannon, 1948). Read counts of microbial species, genera, and families were calculated as the sum of reads assigned to the ASVs within each taxonomic group, and then the taxonomic table was normalized to represent the relative abundances across different taxonomic levels. Additionally, for vitamin synthesis analysis, based on the representation of specific bacteria in the gut (please see the Supplementary material for a list of these bacteria that was created based on prior work showing the role of them in the production of specific vitamins), vitamin synthesis scores (ranging from 1 to 10; higher scores representing above-average microbiome ability to produce vitamin) for Vitamins B1, B2, B3, B5, B6, B7, B9, K were created.
2.4 Dietary recall
Mothers were also asked to report on their child's dietary intake (everything eaten the day before stool sample collection, between midnight and midnight) via Intake241 (https://intake24.co.uk) (Bradley et al., 2016). Data from Intake24 were used to estimate energy, macro- and micro-nutrient intakes.
2.5 Statistical analyses
Statistical analyses were carried out using IBM® Statistical Package for Social Sciences (SPSS Version 29) (SPSS Inc., Chicago, Illinois). High vs. Low MA ER groups were compared on demographic and clinical measures by using independent-samples t-tests or chi-squared tests where appropriate. Separate ANCOVAs [adjusted for child's sex, age, calorie intake,2 physical activity, breastfeeding status, and delivery method, as well as mothers depression (see text footnote 2) and BMI] were used to compare High vs. Low MA ER groups on (i) alpha diversity, (ii) relative abundance of the 30 most abundant genera (iii) relative abundance of butyrate-producing microbes (Faecalibacterium, Roseburia, Anaerostipes, Odoribacter, Eubacterium hallii group, Flavonifractor, Butyricicoccus, Butyricimonas, Butyrivibrio, and Coprococcus), and (iv) vitamin synthesis scores. A statistical significance threshold of p < 0.05 (two-tailed) was applied throughout. Multiple comparisons were adjusted using Bonferroni correction.
3 Results
3.1 Participant characteristics
Participant characteristics in the total sample and separately in high and low MA ER groups are reported in Table 1. Groups did not differ in terms of child's age, sex, birth, current weight, number of siblings, living arrangements (i.e. who they are living with), physical activity, energy, carbohydrate, fat, and protein intake, stay in neonatal intensive care >24 h, breastfeeding status, delivery type, as well as maternal age, body-mass-index, education, ethnicity, employment, age during delivery, and relationship to child, depression, trait and state anxiety, and household income. By design, groups differed on child's MA ER scores. Additionally, groups differed on child's current height, fiber intake (Englyst method), and maternal DERS scores; a trend in group difference on fiber intake (AOAC method) was also observed. For micronutrient comparisons, please see the Supplementary material.
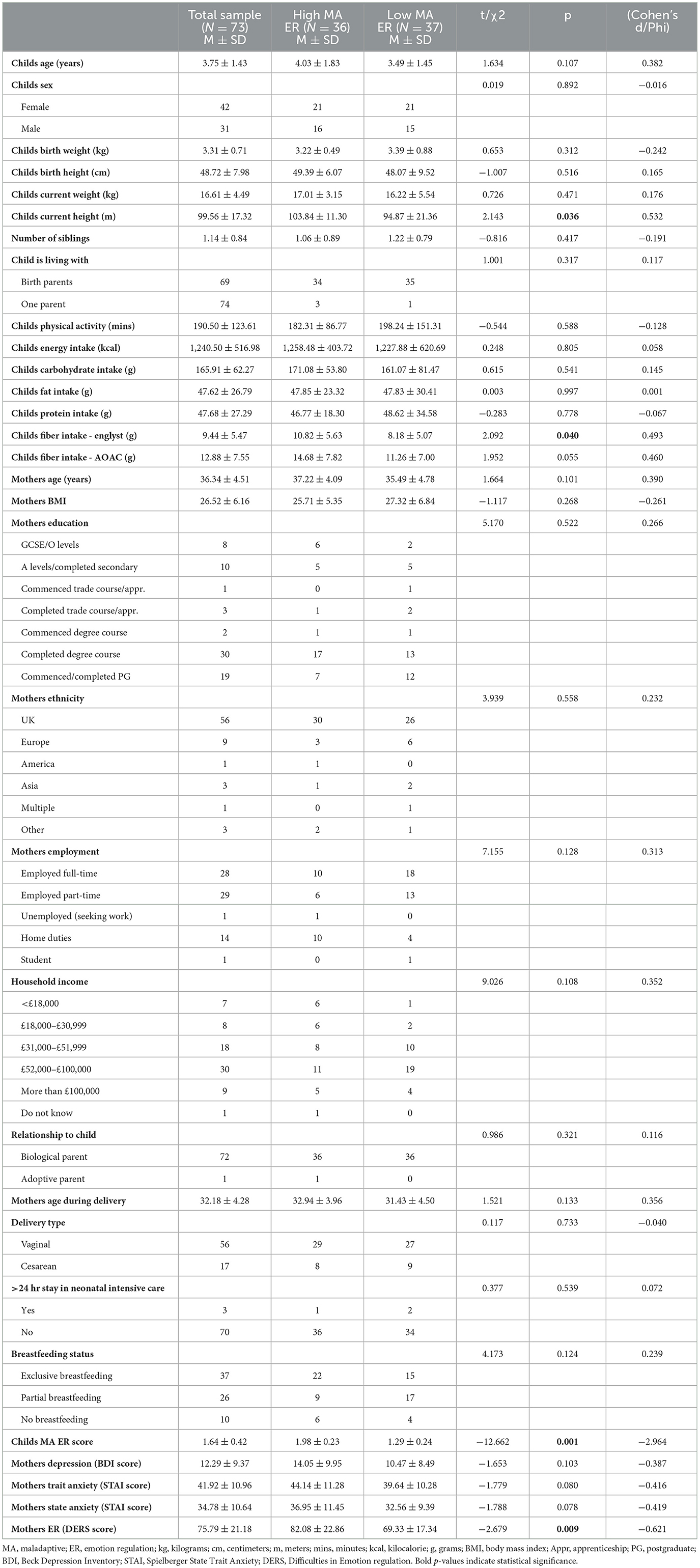
Table 1. Descriptive characteristics of the total sample and high and low maladaptive emotion regulation groups.
3.2 Gut microbiome diversity and composition
There was a significant main effect of group on alpha diversity [F(1, 71) = 4.299, p = 0.042, η2 = 0.066], such that, High MA ER group (M = 5.12, SD = 0.72) had reduced alpha diversity compared to Low MA ER group (M = 5.48, SD = 0.72) (Figure 1A).
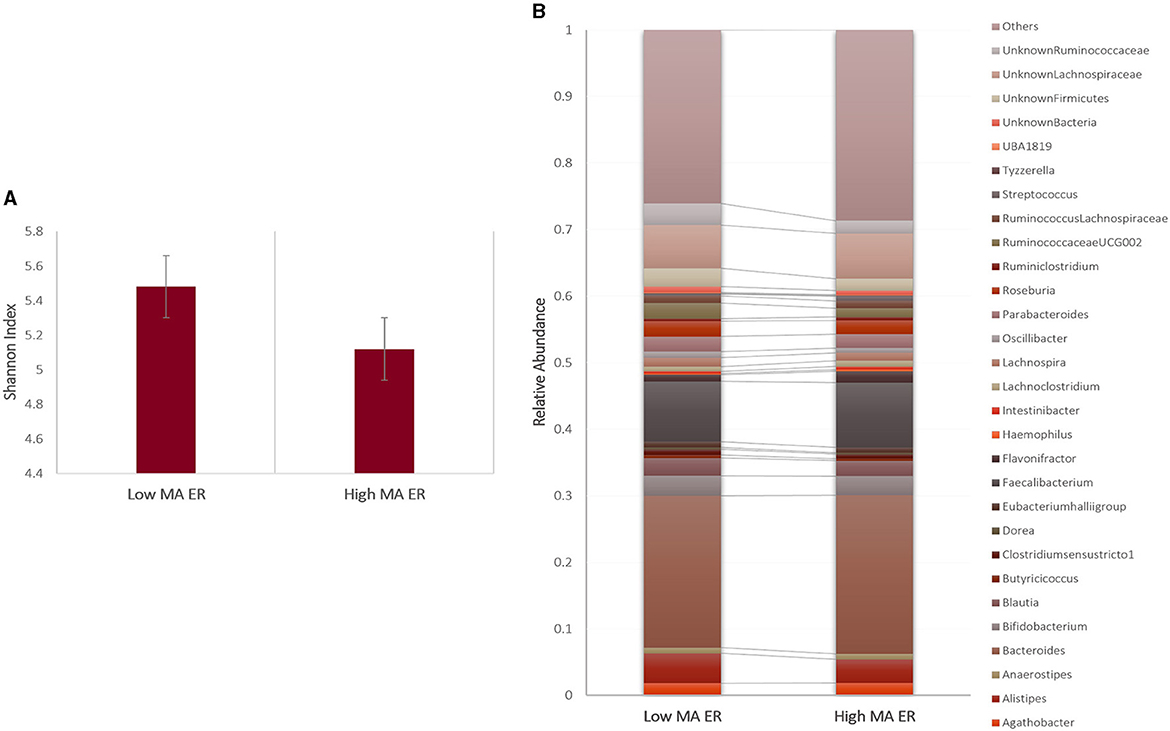
Figure 1. (A) Alpha diversity and (B) summary of the 30 most abundant genera in the Low and High MA ER groups. Bars represent error rates.
The bacterial composition results showed that sequences from the High MA ER group were mainly assigned to Bacteroides, Faecalibacterium, undefined Veillonellaceae, and undefined Prevotellaceae at the genus level; the most abundant genus in the Low MA ER group was also Bacteroides, followed by Faecalibacterium, undefined Lachnospiraceae, and Alistipes (Figure 1B). Compared to those in the Low MA ER group (M = 0.0045, SD = 0.003), the relative abundance of Butyricicoccus [F(1, 69) = 5.217, p = 0.026, η2 = 0.081] was significantly lower in the High MA ER group (M = 0.0026, SD = 0.003) (Figure 2A). Additionally, the relative abundances of Ruminococcaceae UCG-002 [F(1, 58) = 3.402, p = 0.07, η2 = 0.066; Low MA ER M = 0.025, SD = 0.022, High MA ER M = 0.013, SD = 0.022], and undefined Ruminococcaceae [F(1, 58) = 2.816, p = 0.100, η2 = 0.055; Low MA ER M = 0.031, SD = 0.020, High MA ER M = 0.021, SD = 0.020] were nominally lower in the High (vs. Low) MA ER group (Figure 2A). No significant or nominal group differences were observed for other genera (all p > 0.1).
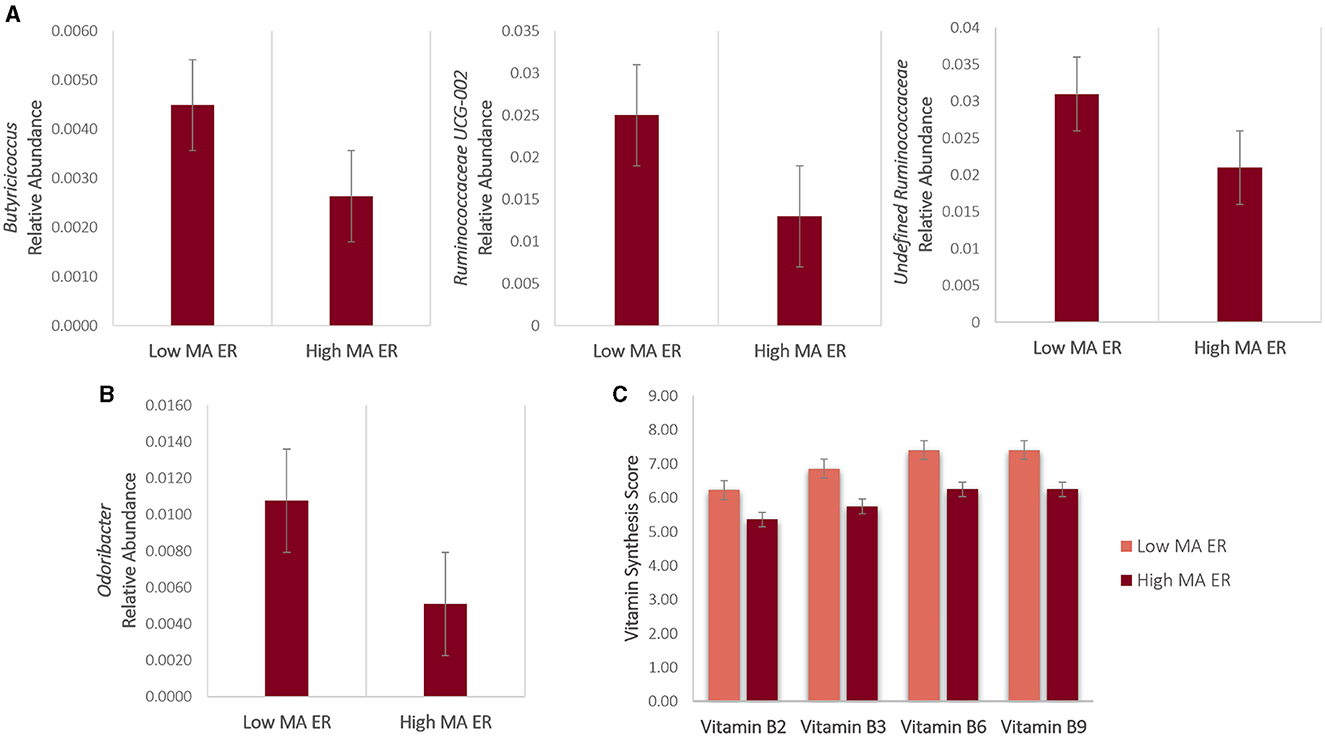
Figure 2. (A, B) Bacterial genera and (C) vitamin synthesis scores that were significantly or nominally different between the Low and High MA ER groups. Bars represent error rates.
3.3 Butyrate and vitamin synthesis capacity
In addition to Butyricicoccus results reported above, the relative abundance of butyrate-producing microbe Odoribacter was significantly lower in the High (M = 0.001, SD = 0.003) [vs. Low (M = 0.010, SD = 0.0135)] MA ER group [F(1, 40) = 4.414, p = 0.044, η2 = 0.128] (Figure 2B). No significant or nominal group differences were observed for other butyrate-producing microbes (all p > 0.1).
Compared to those in the Low MA ER group, vitamin synthesis scores for Vitamin B2 [F(1, 71) = 4.382, p = 0.040, η2 = 0.067; High MA ER M = 5.36, SD = 1.93; Low MA ER M = 6.23, SD = 1.91], Vitamin B3 [F(1, 71) = 7.466, p = 0.008, η2 = 0.109; High MA ER M = 5.75, SD = 1.99; Low MA ER M = 6.85, SD = 1.82], Vitamin B6 [F(1, 71) = 8.160, p = 0.006, η2 = 0.118, High MA ER M = 6.25, SD = 1.87; Low MA ER M = 7.40, SD = 1.58], and Vitamin B9 [F(1, 71) = 4.334, p = 0.042, η2 = 0.066; High MA ER M = 6.25, SD = 1.98; Low MA ER M = 7.40, SD = 1.51] were significantly lower in the High MA ER group (Figure 2C). No significant or nominal group differences were observed for other vitamin synthesis scores (all p > 0.1).
4 Discussion
In the current cross-sectional study, we examined the associations between the gut microbiome (diversity and composition) and emotion regulation in 2-to-6-year-old children and observed that high MA ER skills were associated with (i) a less diverse gut microbiota, (ii) distinct compositional profiles of the gut microbiome, and (iii) alterations in vitamin synthesis scores.
4.1 Gut microbiome diversity and composition
Our finding of an association between higher MA ER skills and reduced alpha diversity is consistent with prior studies that identified significant associations between increased alpha diversity and (i) reduced suppression (a maladaptive emotional regulation strategy that is involved in inhibiting emotional behavior after emotions occur) in adults (Ke et al., 2023), and (ii) higher surgency/positive emotionality in toddlers (Christian et al., 2015). As it has been suggested that MA ER during childhood is associated with risk for developing depressive and anxiety symptoms later in life (Aldao et al., 2010), our finding highlights that reduced microbial diversity is associated with host behavior, operationalised as maternal ratings, and a possible early biomarker of MA ER, hence, adolescence/adulthood depression. However, more biologically meaningful descriptors are required, as microbial diversity provides a summary of a highly dimensional dataset.
We also considered microbiome associations at the genus level and showed that those with high maladaptive ER tendencies had significantly lower relative abundance of Butyricicoccus and Odoribacter. Our findings also revealed that the relative abundances of Ruminococcaceae UCG-002 and undefined Ruminococcaceae were nominally lower in the high (vs. low) MA ER group. Consistently, studies in infants have also shown that various behavioral outcomes (such as temperament) were associated with distinct compositional profiles of the gut microbiome at the species level (Christian et al., 2015; Aatsinki et al., 2019; Loughman et al., 2020; Wang et al., 2020; Kelsey et al., 2021; Fox et al., 2022). However, it is important to highlight that the bacterial signatures of behavioral outcomes are different across these studies, which is not surprising given the vast variation in the developing gut microbiome composition early in life (Bäckhed et al., 2015). Nevertheless, these species were previously reported as being associated with psychopathological conditions that have also been linked with emotional distress and dysregulation (Guimond et al., 2021). For instance, low preponderance of Butyricicoccus, Odoribacter, Ruminococcaceae UCG002 genera as well as Oscillospiraceae and Ruminococcaceae family has been observed in individuals with generalized anxiety, obsessive compulsive, and depressive disorders (Jiang et al., 2018; Cheung et al., 2019; Valles-Colomer et al., 2019; Barandouzi et al., 2020; Turna et al., 2020; Simpson et al., 2021; Radjabzadeh et al., 2022; Kim et al., 2023).
In terms of mechanisms, both Butyricicoccus and Odoribacter are known butyrate producers (Geirnaert et al., 2014; Petra et al., 2015; Gomez-Arango et al., 2016; Rivière et al., 2016). Butyrate is a short-chain fatty acid produced by bacterial fermentation of fiber in the colon, and it is the main energy source for colonic epithelial cells, and it is known to (i) enhance epithelial barrier integrity, (ii) prevent “leaky gut” syndrome (increased gut permeability), and (iii) inhibit inflammation (Hamer et al., 2008). As such, lower levels of butyrate-producing bacteria in children with high MA ER skills may be associated with lower colonic butyrate, increased intestinal permeability, and hence, microbial dysbiosis that is known to have a role in psychiatric conditions (Clapp et al., 2017; Andrioaie et al., 2022; Grau-Del Valle et al., 2023). Given that children in the high MA ER group consumed less dietary fiber, our findings showing reduced relative abundance of butyrate-producing bacteria in the gut are not surprising, however, future studies are warranted to measure colonic butyrate levels to confirm our results. Ruminococcaceae, on the other hand, was reported to be correlated with energy metabolism pathways, including gluconeogenesis, glycolysis, and the pentose phosphate pathways in animal studies (Zhang et al., 2019). Therefore, lower Ruminococcaceae in children with high MA ER skills could be related to altered energy metabolism, which is also a common finding in patients with depression, bipolar disorder, schizophrenia, and autism (Gu et al., 2021; Kolar et al., 2021; Oyarzábal et al., 2021), possibly due to the role of glucose in regulating glutamate, acetylcholine, gamma-aminobutyric acid (Dienel, 2019). It is also important to note that, aforementioned bacteria show potential involvement in the synthesis of glutamate, serotonin and gamma amino butyric acid, key neurotransmitters for mental health disorders (see Supplementary Data of Valles-Colomer et al., 2019) potentially directly by passing the blood-brain-barrier (BBB) [e.g., via gut dysbiosis affecting BBB permeability (Tang et al., 2020)] or indirectly via peripheral routes [e.g., via the vagus nerve (Cryan et al., 2019)].
4.2 Vitamin synthesis
Ccompared to those in the Low MA ER group, vitamin synthesis scores for Vitamin B2, Vitamin B3, Vitamin B6, and Vitamin B9 were significantly lower in the High MA ER group. Our results are consistent with prior work that found lower serum levels and/or intakes of Vitamins B2, B3, B6, and B9 was associated with increased mental health complaints and/or risk of psychopathology (Cornish and Mehl-Madrona, 2008) that is known to be linked with emotional dysregulation (Guimond et al., 2021).
Vitamin B2 derivatives, specifically flavin adenine dinucleotide and flavin mononucleotide (Zempleni et al., 2013), possess antioxidant properties and are cofactors in the metabolism of fatty acids in brain lipids, hence dysregulation of this process could be associated with broad negative consequences for brain function (Kennedy, 2016). Additionally, Vitamin B2 is known to inhibit inflammation in adipocyte and macrophage co-cultures (Mazur-Bialy and Pocheć, 2016) and similarly, Vitamin B3 is known to decrease the expression of the pro-inflammatory cytokines, IL-1, IL-6, and TNF-α by the macrophages (Bialonska et al., 2010). Thus, dysregulation of inflammatory processes may mediate the connection between synthesis of Vitamins B2 and B3 and maladaptive emotion regulation, due to the link between inflammation and mental health outcomes (Goldsmith et al., 2016; Yuan et al., 2019; Hepsomali and Coxon, 2022). Moreover, both Vitamin B3 and serotonin are synthesized from tryptophan, and altered Vitamin B3 synthesis can lead to lower levels of serotonin (Fukuwatari and Shibata, 2013), which may, subsequently, contribute to maladaptive emotion regulation skills, as serotonin is a crucial modulator of emotional behavior (Lesch, 2007). Vitamin B6, on the other hand, is particularly well known for its role in the synthesis of dopamine from L-DOPA, serotonin from 5-HTP, and gamma-aminobutyric acid from glutamate (Calderón-Ospina and Nava-Mesa, 2020). Similarly, Vitamin B9 is also involved in the synthesis of dopamine, norepinephrine, and serotonin (Bottiglieri et al., 2000; Lam et al., 2022) and by converting homocysteine to methionine, Vitamin B9 also facilitates the production of S-adenosyl methionine, which influences the synthesis of these neurotransmitters (Bottiglieri et al., 1994). Alterations in the aforementioned neurotransmitters are known to be associated with exacerbations of psychiatric and neurodevelopmental disorders (Szabo et al., 2004; Ramamoorthi and Lin, 2011; Moretto et al., 2018; Carvajal-Oliveros and Campusano, 2021).
4.3 Limitations
Certain limitations in the current study should be noted. Firstly, although small sample sizes are common in human microbiome research, in the current study a relatively small sample size was used. Secondly, the cross-sectional nature of this study does not allow us to test for causality and/or directionality (especially considering the role of nutrition in modulating the gut microbiome), hence, reverse causation is also possible. Thirdly, emotion regulation was reported only by the mother, and parental reports might not always correlate strongly with observer reports in standardized conditions (Mangelsdorf et al., 2000) and might be influenced by maternal temperament and other characteristics such as age, gender, stress and depression (Bayly and Gartstein, 2013), as well as parents' ability to read their children's emotions and monitor and report dietary intake accurately. Fourthly, gut microbiome data was processed using 16S rRNA sequencing instead of using the more precise whole genome sequencing (Laudadio et al., 2018), which provides information on microbial composition but not function (e.g., bacterial gene content or metabolite levels). However, as 16S rRNA sequencing is currently the most widely used application to study microbiota, this makes the current study comparable with others in the field. Fifthly, we utilized median splits to artificially categorized MA ER, and although it is not an uncommon and unacceptable practice (Iacobucci et al., 2015), future studies are warranted to examine the associations between MA ER and gut microbiome markers in a continuous manner in a larger sample. Sixthly, although we excluded participants if their child had a significant acute or chronic disorder based on maternal reports, we cannot guarantee a psychopathological problem-free sample. Finally, both emotion regulation and gut microbiome were assessed only at a single time point. Although it is important to note that prior work highlighted significant temporal variation in the adult gut microbiome (Davenport et al., 2014; Riddle and Connor, 2016), intraindividual variability in key groups of microorganisms was found to remain relatively low during infancy (Subramanian et al., 2014; Raman et al., 2019). These limitations are balanced by considerable strengths which include a detailed consideration of potential confounding factors, information to estimate dietary intake, and the use of validated questionnaires.
5 Conclusion
In conclusion, our study contributes to a growing literature that links gut microbiota to host behavior and physiology, by demonstrating that microbial diversity and composition are associated with host behavior, operationalized as maternal ratings of emotion regulation skills. As maladaptive emotion regulation skills might precede psychopathology and adaptive emotion regulation skills, on the other hand, might present as resilience factors, the results may have implications on early prevention of child mental health problems in the future. Future studies in larger cohorts, utilizing designs that allow causality/directionality, ideally by incorporating metagenomics, metabolomics, as well as neuroimaging (at multiple timepoints to involve serial and concurrent sampling and measurements) will help begin to elucidate the bidirectional association between early life microbiota and developmental trajectories of early life behavioral and cognitive risk factors and potential development of psychopathology.
Data availability statement
The raw data supporting the conclusions of this article will be made available by the authors, without undue reservation.
Ethics statement
The studies involving humans were approved by University of Roehampton Ethical Committee. The studies were conducted in accordance with the local legislation and institutional requirements. Written informed consent for participation in this study was provided by the participants' legal guardians/next of kin.
Author contributions
PF: Conceptualization, Methodology, Writing – original draft, Writing – review & editing. AC: Writing – review & editing. FI: Formal analysis, Investigation, Writing – review & editing. NP: Writing – review & editing. PH: Conceptualization, Formal analysis, Funding acquisition, Methodology, Project administration, Supervision, Writing – original draft, Writing – review & editing.
Funding
The author(s) declare financial support was received for the research, authorship, and/or publication of this article. The authors declare that this study received funding from Reckitt/Mead Johnson Nutrition. The funder was not involved in the study design, collection, analysis, interpretation of data, the writing of this article, or the decision to submit it for publication.
Conflict of interest
PH and AC have received research funding, consultancy, travel support, and speaking honoraria from various industrial companies. NP is employed at Reckitt/Mead Johnson Nutrition.
The remaining authors declare that the research was conducted in the absence of any commercial or financial relationships that could be construed as a potential conflict of interest.
Publisher's note
All claims expressed in this article are solely those of the authors and do not necessarily represent those of their affiliated organizations, or those of the publisher, the editors and the reviewers. Any product that may be evaluated in this article, or claim that may be made by its manufacturer, is not guaranteed or endorsed by the publisher.
Supplementary material
The Supplementary Material for this article can be found online at: https://www.frontiersin.org/articles/10.3389/fdpys.2024.1445642/full#supplementary-material
Footnotes
1. ^Intake24 is an open-source web-based dietary assessment research tool based on the 24-hour recall method, primarily designed for self-completion. Intake24 was created by Newcastle University (UK), funded by Food Standards Agency, Scotland and is now maintained and developed through a collaboration between Cambridge University (UK), Monash University (Australia) and Newcastle University (UK).
2. ^Due to multicollinearity, only children's calorie intake and mother's depression scores (but not other nutrients and mental health parameters) were added as covariates. Correlations between (i) calorie and nutrient intakes, and, (ii) depression and anxiety and emotion regulation, please see the Supplementary material.
References
Aatsinki, A.-K., Lahti, L., Uusitupa, H.-M., Munukka, E., Keskitalo, A., Nolvi, S., et al. (2019). Gut microbiota composition is associated with temperament traits in infants. Brain Behav. Immun. 80, 849–858. doi: 10.1016/j.bbi.2019.05.035
Aldao, A., Nolen-Hoeksema, S., and Schweizer, S. (2010). Emotion-regulation strategies across psychopathology: a meta-analytic review. Clin. Psychol. Rev. 30, 217–237. doi: 10.1016/j.cpr.2009.11.004
Allen, A. P., Dinan, T. G., Clarke, G., and Cryan, J. F. (2017). A psychology of the human brain-gut-microbiome axis. Soc. Personal. Psychol. Compass 11:e12309. doi: 10.1111/spc3.12309
Alving-Jessep, E., Botchway, E., Wood, A. G., Hilton, A. C., and Blissett, J. M. (2022). The development of the gut microbiome and temperament during infancy and early childhood: a systematic review. Dev. Psychobiol. 64:e22306. doi: 10.1002/dev.22306
Amir, A., McDonald, D., Navas-Molina, J., Kopylova, E., Morton, J., and Zech Xu, Z. (2017). Deblur rapidly resolves single-nucleotide community sequence patterns. mSystems 2, e00191–e00116. doi: 10.1128/mSystems.00191-16
Andrioaie, I. M., Duhaniuc, A., Nastase, E. V., Iancu, L. S., Luncă, C., Trofin, F., et al. (2022). The role of the gut microbiome in psychiatric disorders. Microorganisms 10:2436. doi: 10.3390/microorganisms10122436
Bäckhed, F., Roswall, J., Peng, Y., Feng, Q., Jia, H., Kovatcheva-Datchary, P., et al. (2015). Dynamics and stabilization of the human gut microbiome during the first year of life. Cell Host Microbe 17, 690–703. doi: 10.1016/j.chom.2015.04.004
Barandouzi, Z. A., Starkweather, A. R., Henderson, W. A., Gyamfi, A., and Cong, X. S. (2020). Altered composition of gut microbiota in depression: a systematic review. Front. Psychiatry 11:541. doi: 10.3389/fpsyt.2020.00541
Bayly, B., and Gartstein, M. (2013). Mother's and father's reports on their child's temperament: does gender matter? Infant Behav. Dev. 36, 171–175. doi: 10.1016/j.infbeh.2012.10.008
Beck, A. T., Steer, R. A., Ball, R., and Ranieri, W. (1996). Comparison of Beck Depression Inventories -IA and -II in psychiatric outpatients. J. Pers. Assess. 67, 588–597. doi: 10.1207/s15327752jpa6703_13
Beck, A. T., Steer, R. A., and Carbin, M. G. (1988). Psychometric properties of the beck depression inventory: twenty-five years of evaluation. Clin. Psychol. Rev. 8, 77–100. doi: 10.1016/0272-7358(88)90050-5
Bialonska, D., Ramnani, P., Kasimsetty, S. G., Muntha, K. R., Gibson, G. R., and Ferreira, D. (2010). The influence of pomegranate by-product and punicalagins on selected groups of human intestinal microbiota. Int. J. Food Microbiol. 140, 175–182. doi: 10.1016/j.ijfoodmicro.2010.03.038
Bolyen, E., Rideout, J. R., Dillon, M. R., Bokulich, N. A., Abnet, C. C., Al-Ghalith, G. A., et al. (2019). Reproducible, interactive, scalable and extensible microbiome data science using QIIME 2. Nat. Biotechnol. 37, 852–857. doi: 10.1038/s41587-019-0209-9
Boonchooduang, N., Louthrenoo, O., Chattipakorn, N., and Chattipakorn, S. C. (2020). Possible links between gut-microbiota and attention-deficit/hyperactivity disorders in children and adolescents. Eur. J. Nutr. 59, 3391–3403. doi: 10.1007/s00394-020-02383-1
Bottiglieri, T., Hyland, K., and Reynolds, E. H. (1994). The clinical potential of ademetionine (S-adenosylmethionine) in neurological disorders. Drugs 48, 137–152. doi: 10.2165/00003495-199448020-00002
Bottiglieri, T., Laundy, M., Crellin, R., Toone, B. K., Carney, M. W., and Reynolds, E. H. (2000). Homocysteine, folate, methylation, and monoamine metabolism in depression. J. Neurol. Neurosurg. Psychiatry 69, 228–232. doi: 10.1136/jnnp.69.2.228
Bradley, J., Simpson, E., Poliakov, I., Matthews, J. N., Olivier, P., Adamson, A. J., et al. (2016). Comparison of INTAKE24 (an Online 24-h Dietary Recall Tool) with Interviewer-Led 24-h Recall in 11-24 Year-Old. Nutrients 8:358. doi: 10.3390/nu8060358
Calderón-Ospina, C. A., and Nava-Mesa, M. O. (2020). B Vitamins in the nervous system: Current knowledge of the biochemical modes of action and synergies of thiamine, pyridoxine, and cobalamin. CNS Neurosci. Therap. 26, 5–13. doi: 10.1111/cns.13207
Caporaso, J. G., Lauber, C. L., Walters, W. A., Berg-Lyons, D., Huntley, J., Fierer, N., et al. (2012). Ultra-high-throughput microbial community analysis on the Illumina HiSeq and MiSeq platforms. ISME J. 6, 1621–1624. doi: 10.1038/ismej.2012.8
Carvajal-Oliveros, A., and Campusano, J. M. (2021). Studying the contribution of serotonin to neurodevelopmental disorders. can this fly? Front. Behav. Neurosci. 14:601449. doi: 10.3389/fnbeh.2020.601449
Chen, C.-C., Wu, W.-K., Chang, C.-M., Panyod, S., Lu, T.-P., Liou, J.-M., et al. (2020). Comparison of DNA stabilizers and storage conditions on preserving fecal microbiota profiles. J. Formosan Med. Assoc. 119, 1791–1798. doi: 10.1016/j.jfma.2020.01.013
Cheung, S. G., Goldenthal, A. R., Uhlemann, A. C., Mann, J. J., Miller, J. M., and Sublette, M. E. (2019). Systematic review of gut microbiota and major depression. Front. Psychiatry 10:34. doi: 10.3389/fpsyt.2019.00034
Christian, L. M., Galley, J. D., Hade, E. M., Schoppe-Sullivan, S., Kamp Dush, C., and Bailey, M. T. (2015). Gut microbiome composition is associated with temperament during early childhood. Brain Behav. Immun. 45, 118–127. doi: 10.1016/j.bbi.2014.10.018
Cisler, J. M., Olatunji, B. O., Feldner, M. T., and Forsyth, J. P. (2010). Emotion regulation and the anxiety disorders: an integrative review. J. Psychopathol. Behav. Assess. 32, 68–82. doi: 10.1007/s10862-009-9161-1
Clapp, M., Aurora, N., Herrera, L., Bhatia, M., Wilen, E., and Wakefield, S. (2017). Gut microbiota's effect on mental health: the gut-brain axis. Clin. Pract. 7:987. doi: 10.4081/cp.2017.987
Compare, A., Zarbo, C., Shonin, E., Van Gordon, W., and Marconi, C. (2014). Emotional regulation and depression: a potential mediator between heart and mind. Cardiovasc. Psychiatry Neurol. 2014:324374. doi: 10.1155/2014/324374
Cornish, S., and Mehl-Madrona, L. (2008). The role of vitamins and minerals in psychiatry. Integr. Med. Insights 3:117863370800300003. doi: 10.4137/117863370800300003
Cryan, J. F., and Dinan, T. G. (2012). Mind-altering microorganisms: the impact of the gut microbiota on brain and behaviour. Nat. Rev. Neurosci. 13, 701–712. doi: 10.1038/nrn3346
Cryan, J. F., O'Riordan, K. J., Cowan, C. S. M., Sandhu, K. V., Bastiaanssen, T. F. S., Boehme, M., et al. (2019). The microbiota-gut-brain axis. Physiol. Rev. 99, 1877–2013. doi: 10.1152/physrev.00018.2018
Davenport, E. R., Mizrahi-Man, O., Michelini, K., Barreiro, L. B., Ober, C., and Gilad, Y. (2014). Seasonal variation in human gut microbiome composition. PLoS ONE 9:e90731. doi: 10.1371/journal.pone.0090731
Derakhshan, N. (2020). “Attentional control and cognitive biases as determinants of vulnerability and resilience in anxiety and depression,” in Cognitive biases in health and psychiatric disorders (Academic Press), 261–274. doi: 10.1016/B978-0-12-816660-4.00012-X
DeSantis, T. Z., Hugenholtz, P., Larsen, N., Rojas, M., Brodie, E. L., Keller, K., et al. (2006). Greengenes, a chimera-checked 16S rRNA gene database and workbench compatible with ARB. Appl. Environ. Microbiol. 72, 5069–5072. doi: 10.1128/AEM.03006-05
Dienel, G. A. (2019). Brain glucose metabolism: integration of energetics with function. Physiol. Rev. 99, 949–1045. doi: 10.1152/physrev.00062.2017
Evans, S. J., Bassis, C. M., Hein, R., Assari, S., Flowers, S. A., Kelly, M. B., et al. (2017). The gut microbiome composition associates with bipolar disorder and illness severity. J. Psychiatr. Res. 87, 23–29. doi: 10.1016/j.jpsychires.2016.12.007
Eysenck, M. W., Derakshan, N., Santos, R., and Calvo, M. G. (2007). Anxiety and cognitive performance: attentional control theory. Emotion 7, 336–353. doi: 10.1037/1528-3542.7.2.336
Flannery, J. E., Stagaman, K., Burns, A. R., Hickey, R. J., Roos, L. E., Giuliano, R. J., et al. (2020). Gut feelings begin in childhood: the gut metagenome correlates with early environment, caregiving, and behavior. MBio 11:10-1128. doi: 10.1128/mBio.02780-19
Foster, J. A., Rinaman, L., and Cryan, J. F. (2017). Stress and the gut-brain axis: regulation by the microbiome. Neurobiol. Stress 7, 124–136. doi: 10.1016/j.ynstr.2017.03.001
Fox, M., Lee, S. M., Wiley, K. S., Lagishetty, V., Sandman, C. A., Jacobs, J. P., et al. (2022). Development of the infant gut microbiome predicts temperament across the first year of life. Dev. Psychopathol. 34, 1914–1925. doi: 10.1017/S0954579421000456
Fukuwatari, T., and Shibata, K. (2013). Nutritional aspect of tryptophan metabolism. Int. J. Tryptophan Res. 6, 3–8. doi: 10.4137/IJTR.S11588
Garcia-Gutierrez, E., Narbad, A., and Rodríguez, J. M. (2020). Autism spectrum disorder associated with gut microbiota at immune, metabolomic, and neuroactive level. Front. Neurosci. 14:578666. doi: 10.3389/fnins.2020.578666
Geirnaert, A., Steyaert, A., Eeckhaut, V., Debruyne, B., Arends, J. B. A., Van Immerseel, F., et al. (2014). Butyricicoccus pullicaecorum, a butyrate producer with probiotic potential, is intrinsically tolerant to stomach and small intestine conditions. Anaerobe 30, 70–74. doi: 10.1016/j.anaerobe.2014.08.010
Goldsmith, D. R., Rapaport, M. H., and Miller, B. J. (2016). A meta-analysis of blood cytokine network alterations in psychiatric patients: comparisons between schizophrenia, bipolar disorder and depression. Mol. Psychiatry 21, 1696–1709. doi: 10.1038/mp.2016.3
Gomez-Arango, L. F., Barrett, H. L., McIntyre, H. D., Callaway, L. K., Morrison, M., and Nitert, M. D. (2016). Increased systolic and diastolic blood pressure is associated with altered gut microbiota composition and butyrate production in early pregnancy. Hypertension 68, 974–981. doi: 10.1161/HYPERTENSIONAHA.116.07910
Gratz, K. L., and Roemer, L. (2004). Multidimensional assessment of emotion regulation and dysregulation: development, factor structure, and initial validation of the difficulties in emotion regulation scale. J. Psychopathol. Behav. Assess. 26, 41–54. doi: 10.1023/B:JOBA.0000007455.08539.94
Grau-Del Valle, C., Fernández, J., Solá, E., Montoya-Castilla, I., Morillas, C., and Bañuls, C. (2023). Association between gut microbiota and psychiatric disorders: a systematic review. Front. Psychol. 14:1215674. doi: 10.3389/fpsyg.2023.1215674
Gu, X., Ke, S., Wang, Q., Zhuang, T., Xia, C., Xu, Y., et al. (2021). Energy metabolism in major depressive disorder: Recent advances from omics technologies and imaging. Biomed. Pharmacother. 141:111869. doi: 10.1016/j.biopha.2021.111869
Guimond, A.-J., Kubzansky, L. D., and Lee, L. O. (2021). “Emotion and illness,” in Emotion in the Clinical Encounter, eds. R. Schwartz, J. A. Hall, and L. G. Osterberg (New York: McGraw Hill).
Hamer, H. M., Jonkers, D., Venema, K., Vanhoutvin, S., Troost, F. J., and Brummer, R. J. (2008). Review article: the role of butyrate on colonic function. Aliment. Pharmacol. Therapeut. 27, 104–119. doi: 10.1111/j.1365-2036.2007.03562.x
Hepsomali, P., and Coxon, C. (2022). Inflammation and diet: Focus on mental and cognitive health. Adv. Clin. Exp. Med. 31, 821–825. doi: 10.17219/acem/152350
Iacobucci, D., Posavac, S. S., Kardes, F. R., Schneider, M. J., and Popovich, D. L. (2015). The median split: robust, refined, and revived. J. Consumer Psychol. 25, 690–704. doi: 10.1016/j.jcps.2015.06.014
Jiang, H., Ling, Z., Zhang, Y., Mao, H., Ma, Z., Yin, Y., et al. (2015). Altered fecal microbiota composition in patients with major depressive disorder. Brain Behav. Immun. 48, 186–194. doi: 10.1016/j.bbi.2015.03.016
Jiang, H.-y., Zhang, X., Yu, Z.-h., Zhang, Z., Deng, M., Zhao, J.-h., et al. (2018). Altered gut microbiota profile in patients with generalized anxiety disorder. J. Psychiatr. Res. 104, 130–136. doi: 10.1016/j.jpsychires.2018.07.007
Ke, S., Guimond, A.-J., Tworoger, S. S., Huang, T., Chan, A. T., Liu, Y.-Y., et al. (2022). Gut feelings: Associations of emotions and emotion regulation with the gut microbiome in women. bioRxiv 2022.2005.2026.493641. doi: 10.1101/2022.05.26.493641
Ke, S., Guimond, A.-J., Tworoger, S. S., Huang, T., Chan, A. T., Liu, Y.-Y., et al. (2023). Gut feelings: associations of emotions and emotion regulation with the gut microbiome in women. Psychol. Med. 53, 7151–7160. doi: 10.1017/S0033291723000612
Kelly, J. R., Minuto, C., Cryan, J. F., Clarke, G., and Dinan, T. G. (2017). Cross talk: the microbiota and neurodevelopmental disorders. Front. Neurosci. 11:490. doi: 10.3389/fnins.2017.00490
Kelsey, C. M., Prescott, S., McCulloch, J. A., Trinchieri, G., Valladares, T. L., Dreisbach, C., et al. (2021). Gut microbiota composition is associated with newborn functional brain connectivity and behavioral temperament. Brain Behav. Immun. 91, 472–486. doi: 10.1016/j.bbi.2020.11.003
Kennedy, D. O. (2016). B vitamins and the brain: mechanisms, dose and efficacy–a review. Nutrients 8:68. doi: 10.3390/nu8020068
Kim, S.-Y., Woo, S.-Y., Raza, S., Ho, D., Jeon, S. W., Chang, Y., et al. (2023). Association between gut microbiota and anxiety symptoms: a large population-based study examining sex differences. J. Affect. Disord. 333, 21–29. doi: 10.1016/j.jad.2023.04.003
Kolar, D., Kleteckova, L., Brozka, H., and Vales, K. (2021). Mini-review: brain energy metabolism and its role in animal models of depression, bipolar disorder, schizophrenia and autism. Neurosci. Lett. 760:136003. doi: 10.1016/j.neulet.2021.136003
Kraaij, R., Schuurmans, I. K., Radjabzadeh, D., Tiemeier, H., Dinan, T. G., Uitterlinden, A. G., et al. (2023). The gut microbiome and child mental health: a population-based study. Brain Behav. Immun. 108, 188–196. doi: 10.1016/j.bbi.2022.12.006
Lam, N. S. K., Long, X. X., Li, X., Saad, M., Lim, F., Doery, J. C. G., et al. (2022). The potential use of folate and its derivatives in treating psychiatric disorders: a systematic review. Biomed. Pharmacother. 146:112541. doi: 10.1016/j.biopha.2021.112541
Laudadio, I., Fulci, V., Palone, F., Stronati, L., Cucchiara, S., and Carissimi, C. (2018). Quantitative assessment of shotgun metagenomics and 16s rDNA amplicon sequencing in the study of human gut microbiome. OMICS 22, 248–254. doi: 10.1089/omi.2018.0013
Leigh, S.-J., Uhlig, F., Wilmes, L., Sanchez-Diaz, P., Gheorghe, C. E., Goodson, M. S., et al. (2023). The impact of acute and chronic stress on gastrointestinal physiology and function: a microbiota–gut–brain axis perspective. J. Physiol. 601, 4491–4538. doi: 10.1113/JP281951
Lesch, K. P. (2007). Linking emotion to the social brain. The role of the serotonin transporter in human social behaviour. EMBO Rep. 8, S24–29. doi: 10.1038/sj.embor.7401008
Li, S., Song, J., Ke, P., Kong, L., Lei, B., Zhou, J., et al. (2021). The gut microbiome is associated with brain structure and function in schizophrenia. Sci. Rep. 11:9743. doi: 10.1038/s41598-021-89166-8
Loughman, A., Ponsonby, A. L., O'Hely, M., Symeonides, C., Collier, F., Tang, M. L. K., et al. (2020). Gut microbiota composition during infancy and subsequent behavioural outcomes. EBioMedicine 52:102640. doi: 10.1016/j.ebiom.2020.102640
Mangelsdorf, S. C., Schoppe, S. J., and Buur, H. (2000). “The meaning of parental reports: A contextual approach to the study of temperament and behavior problems in childhood,” in Temperament and personality development across the life span (Lawrence Erlbaum Associates Publishers), 121–140.
Mazur-Bialy, A. I., and Pocheć, E. (2016). Riboflavin reduces pro-inflammatory activation of adipocyte-macrophage co-culture potential application of vitamin B2 enrichment for attenuation of insulin resistance and metabolic syndrome development. Molecules 21:1724. doi: 10.3390/molecules21121724
Mirabile, S. (2014). Parents' inconsistent emotion socialization and children's socioemotional adjustment. J. Appl. Dev. Psychol. 35, 392–400. doi: 10.1016/j.appdev.2014.06.003
Mirabile, S. P., and Thompson, B. M. (2011). “Skills-based assessment of emotion regulation in early childhood: description and validation of a novel measure,” in Poster presented at the 2011 Biennial Meeting of the Society for Research in Child Development (Montreal, QC).
Morelen, D., Shaffer, A., and Suveg, C. (2016). Maternal emotion regulation: links to emotion parenting and child emotion regulation. J. Fam. Issues 37, 1891–1916. doi: 10.1177/0192513X14546720
Moretto, E., Murru, L., Martano, G., Sassone, J., and Passafaro, M. (2018). Glutamatergic synapses in neurodevelopmental disorders. Progr. Neuro-Psychopharmacol. Biol. Psychiat. 84, 328–342. doi: 10.1016/j.pnpbp.2017.09.014
Oyarzábal, A., Musokhranova, U., Barros, L. F., and García-Cazorla, A. (2021). Energy metabolism in childhood neurodevelopmental disorders. EBioMedicine 69:103474. doi: 10.1016/j.ebiom.2021.103474
Petra, A. I., Panagiotidou, S., Hatziagelaki, E., Stewart, J. M., Conti, P., and Theoharides, T. C. (2015). Gut-microbiota-brain axis and its effect on neuropsychiatric disorders with suspected immune dysregulation. Clin. Ther. 37, 984–995. doi: 10.1016/j.clinthera.2015.04.002
Querdasi, F., Enders, C., Karnani, N., Broekman, B., Chong, Y. S., Gluckman, P., et al. (2023). Multigenerational adversity impacts on human gut microbiome composition and socioemotional functioning in early childhood. Proc. Natl. Acad. Sci. USA. 120:e2213768120. doi: 10.1073/pnas.2213768120
Radjabzadeh, D., Bosch, J. A., Uitterlinden, A. G., Zwinderman, A. H., Ikram, M. A., van Meurs, J. B. J., et al. (2022). Gut microbiome-wide association study of depressive symptoms. Nat. Commun. 13:7128. doi: 10.1038/s41467-022-34502-3
Ramamoorthi, K., and Lin, Y. (2011). The contribution of GABAergic dysfunction to neurodevelopmental disorders. Trends Mol. Med. 17, 452–462. doi: 10.1016/j.molmed.2011.03.003
Raman, A. S., Gehrig, J. L., Venkatesh, S., Chang, H. W., Hibberd, M. C., Subramanian, S., et al. (2019). A sparse covarying unit that describes healthy and impaired human gut microbiota development. Science 365:eaau4735. doi: 10.1126/science.aau4735
Ravi, R. K., Walton, K., and Khosroheidari, M. (2018). “MiSeq: a next generation sequencing platform for genomic analysis,” in Disease Gene Identification: Methods and Protocols, 223–232. doi: 10.1007/978-1-4939-7471-9_12
Riddle, M. S., and Connor, B. A. (2016). The traveling microbiome. Curr. Infect. Dis. Rep. 18:29. doi: 10.1007/s11908-016-0536-7
Rivière, A., Selak, M., Lantin, D., Leroy, F., and De Vuyst, L. (2016). Bifidobacteria and butyrate-producing colon bacteria: importance and strategies for their stimulation in the human gut. Front. Microbiol. 7:979. doi: 10.3389/fmicb.2016.00979
Sarker, H., Anderson, L. N., Borkhoff, C. M., Abreo, K., Tremblay, M. S., Lebovic, G., et al. (2015). Validation of parent-reported physical activity and sedentary time by accelerometry in young children. BMC Res. Notes 8:735. doi: 10.1186/s13104-015-1648-0
Shannon, C. E. (1948). A mathematical theory of communication. Bell Syst. Tech. J. 27, 379–423. doi: 10.1002/j.1538-7305.1948.tb01338.x
Simpson, C. A., Diaz-Arteche, C., Eliby, D., Schwartz, O. S., Simmons, J. G., and Cowan, C. S. M. (2021). The gut microbiota in anxiety and depression – A systematic review. Clin. Psychol. Rev. 83:101943. doi: 10.1016/j.cpr.2020.101943
Solmi, M., Radua, J., Olivola, M., Croce, E., Soardo, L., Salazar de Pablo, G., et al. (2022). Age at onset of mental disorders worldwide: large-scale meta-analysis of 192 epidemiological studies. Mol. Psychiatry 27, 281–295. doi: 10.1038/s41380-021-01161-7
Spielberger, C. D., Gorusch, R. L., Lushene, R., Vagg, P. R., and Jacobs, G. A. (1983). Manual for State-Trait Anxiety Inventory. Palo Alto: Consulting Psychologists Press.
Subramanian, S., Huq, S., Yatsunenko, T., Haque, R., Mahfuz, M., Alam, M. A., et al. (2014). Persistent gut microbiota immaturity in malnourished Bangladeshi children. Nature 510, 417–421. doi: 10.1038/nature13421
Szabo, S. T., Gould, T. D., and Manji, H. K. (2004). “Neurotransmitters, receptors, signal transduction, and second messengers in psychiatric disorders,” in The American Psychiatric Publishing textbook of psychopharmacology, 3rd ed. (American Psychoanalytic Association), 3–52.
Tang, W., Zhu, H., Feng, Y., Guo, R., and Wan, D. (2020). The impact of gut microbiota disorders on the blood-brain barrier. Infect. Drug Resist. 13, 3351–3363. doi: 10.2147/IDR.S254403
Turna, J., Grosman Kaplan, K., Anglin, R., Patterson, B., Soreni, N., Bercik, P., et al. (2020). The gut microbiome and inflammation in obsessive-compulsive disorder patients compared to age- and sex-matched controls: a pilot study. Acta Psychiatr. Scand. 142, 337–347. doi: 10.1111/acps.13175
Valles-Colomer, M., Falony, G., Darzi, Y., Tigchelaar, E. F., Wang, J., Tito, R. Y., et al. (2019). The neuroactive potential of the human gut microbiota in quality of life and depression. Nat. Microbiol. 4, 623–632. doi: 10.1038/s41564-018-0337-x
Varesi, A., Campagnoli, L. I. M., Chirumbolo, S., Candiano, B., Carrara, A., Ricevuti, G., et al. (2023). The brain-gut-microbiota interplay in depression: a key to design innovative therapeutic approaches. Pharmacol. Res. 192:106799. doi: 10.1016/j.phrs.2023.106799
Wang, Y., Chen, X., Yu, Y., Liu, Y., Zhang, Q., and Bai, J. (2020). Association between gut microbiota and infant's temperament in the first year of life in a chinese birth cohort. Microorganisms 8:753. doi: 10.3390/microorganisms8050753
Yuan, N., Chen, Y., Xia, Y., Dai, J., and Liu, C. (2019). Inflammation-related biomarkers in major psychiatric disorders: a cross-disorder assessment of reproducibility and specificity in 43 meta-analyses. Transl. Psychiatry 9:233. doi: 10.1038/s41398-019-0570-y
Zempleni, J., Suttie, J. W., Gregory, J. F. III, and Stover, P. J. (2013). Handbook of Vitamins. New York: CRC Press. doi: 10.1201/b15413
Zhang, F., Zheng, W., Xue, Y., and Yao, W. (2019). Suhuai suckling piglet hindgut microbiome-metabolome responses to different dietary copper levels. Appl. Microbiol. Biotechnol. 103, 853–868. doi: 10.1007/s00253-018-9533-0
Keywords: microbiome, emotion regulation, alpha diversity, Shannon Index, butyrate
Citation: Faulkner P, Costabile A, Imakulata F, Pandey N and Hepsomali P (2024) A preliminary examination of gut microbiota and emotion regulation in 2- to 6-year-old children. Front. Dev. Psychol. 2:1445642. doi: 10.3389/fdpys.2024.1445642
Received: 14 June 2024; Accepted: 05 August 2024;
Published: 21 August 2024.
Edited by:
Ghizlane Bendriss, Weill Cornell Medicine- Qatar, QatarReviewed by:
Marina Fuertes, Instituto Politécnico de Lisboa, PortugalIrina Jarvers, University of Regensburg, Germany
Copyright © 2024 Faulkner, Costabile, Imakulata, Pandey and Hepsomali. This is an open-access article distributed under the terms of the Creative Commons Attribution License (CC BY). The use, distribution or reproduction in other forums is permitted, provided the original author(s) and the copyright owner(s) are credited and that the original publication in this journal is cited, in accordance with accepted academic practice. No use, distribution or reproduction is permitted which does not comply with these terms.
*Correspondence: Piril Hepsomali, P.Hepsomali@reading.ac.uk