- Department of Pulp Biology and Endodontics, Division of Oral Health Sciences, Graduate School of Medical and Dental Sciences, Tokyo Medical and Dental University (TMDU), Tokyo, Japan
Dental pulp is a mesenchymal connective tissue located inside the rigid encasement of the dentin. When bacteria or bacterial products invade the dental pulp, inflammation known as pulpitis is induced in this tissue. Various mediators produced during the course of pulpitis profoundly modify the pathophysiology of the inflammation. Typical mediators include cytokines, chemokines, nitric oxide, reactive oxygen species, matrix metalloproteinases, proteases, neutrophil extracellular traps, neuropeptides, and eicosanoids. Controlling these mediators may potentially lead to the healing of pulpitis and the preservation of pulp tissue. This review discusses these mediators and further explores the possibility of controlling them.
1 Introduction
Dental pulp is a nerve- and vascular-rich mesenchymal connective tissue surrounded by a mineralized tissue called dentin (1). Cells with fibroblastic characteristics (known as dental pulp cells) are the major cellular constituents of the pulp tissue. Along the most peripheral portion of the pulp tissue subjacent to the pulp−dentin border, a layer of cells responsible for dentin formation, known as odontoblasts, are located. The pulp tissue also contains dental pulp stem cells, which are mesenchymal stem cells that have the ability to differentiate into a new generation of odontoblast-like cells responsible for reparative dentin formation when the original odontoblasts are lost as a result of severe damage to the pulp (2). Various types of immunocompetent cells are also present in the dental pulp tissue. In particular, dendritic cells constitutively expressing major histocompatibility complex class II molecules are abundant just below the odontoblast layer and respond as antigen-presenting cells to bacterial stimuli derived via the dentin tubules (3, 4). Class II-negative resident macrophages, another major immune cell population of the pulp, are diffusely distributed throughout the pulp tissue. These cells express M2 macrophage markers such as CD163 and may be associated with the repair of injured pulp tissue by promoting angiogenesis (5). The nerves and blood vessels that are abundant in the pulp tissue also characterize the pathophysiology of this tissue.
Following invasion of bacterial stimuli into the pulp tissue, typically through a carious lesion or traumatically exposed dentin, localized pulp inflammation is initiated in the area around the site of the initial challenge in the coronal pulp. Pulp inflammation then spreads to the root pulp tissue, exhibiting variable histology and clinical manifestations. Clinically, pulpitis is classified as reversible pulpitis or irreversible pulpitis, the latter being further classified into symptomatic and asymptomatic irreversible pulpitis (6). Reversible pulpitis usually progresses to irreversible pulpitis, but the acute manifestation of irreversible pulpitis depends on the balance between the degree of bacterial invasion and the activity of the defense systems present in the pulp tissues (7).
Although the clinical classification of pulpitis cannot be strictly applied to the histopathologic classification, the nature of the inflammatory reaction occurring in pulpal inflammation is essentially no different from inflammation in other parts of the body. However, because the pulp is situated in a low-compliance environment surrounded by mineralized tissues, intrapulpal pressure increases with the progression of exudation causing compression of blood vessels to be shunted (8), impeding circulation in the pulp. In other words, the impaired circulation and accompanying hypoxia cause deep modifications of pulpal inflammation (9, 10).
To cope with exogenous noxious stimuli, the pulp tissue has the ability to self-protect against dentinal tubule-derived bacterial invasion by forming reparative dentin, and resident and recruited immune cells eliminate bacterial products that have invaded the pulp (1). Dense sensory innervation of the pulp indicates that, in addition to evoking pain as a warning signal, neurogenic inflammation may be deeply involved in the pathophysiological responses of this tissue; the neurogenic inflammation involves vasodilation and vascular permeability increases through the release of neuropeptides, such as substance P, from the endings of sensory nerve fibers (11). However, if bacterial infection from the oral cavity persists, pulpitis spreads from the coronal region to the apex. The pulp eventually becomes necrotic and the defense mechanisms of the pulp tissue are no longer triggered, ultimately leading to tooth loss.
Various inflammatory mediators produced during the course of pulpitis profoundly modify the pathophysiology of the inflammation. Many of these mediators are also produced in healthy pulp tissue, where they are associated with physiological functions and contribute to the maintenance of homeostasis of this tissue. This article aims to give an overview of the properties and roles of the key mediators of pulpitis and to explore the possibility of therapeutic control of these mediators.
2 Mediators in pulpitis
Mediators discussed in this review are listed in Figure 1.
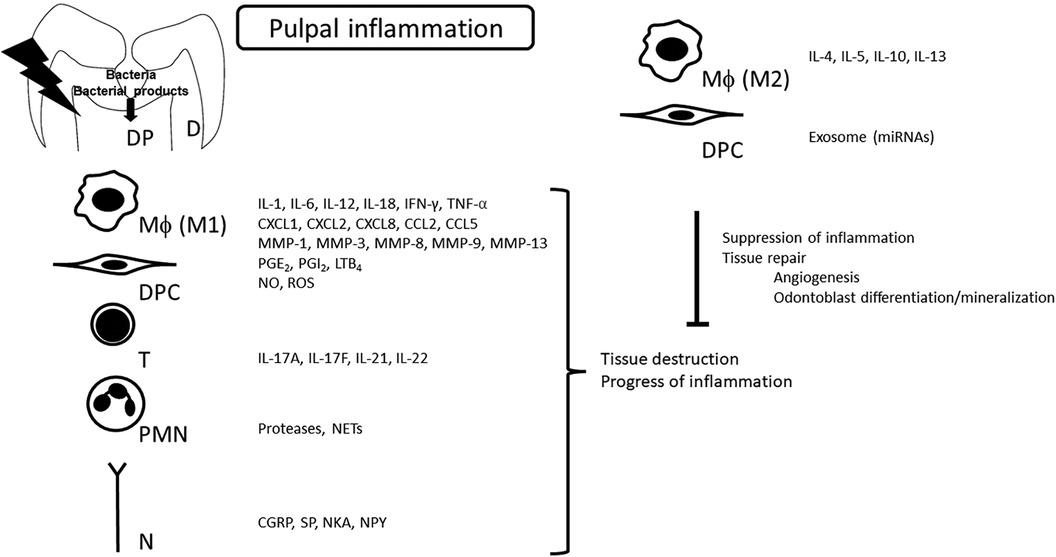
Figure 1. Synthesis of mediators in pulpal inflammation. Various mediators produced during the pulpal inflammatory process profoundly modulate the pathogenesis of pulpitis. At the same time, as inflammation progresses, the production of anti-inflammatory mediators is induced as a result of negative feedback, which is another characteristic of pulpitis. D, dentin; DP, dental pulp; Mϕ, macrophage; DPC, dental pulp cells; T, T cells; PMN, polymorphonuclear leukocyte; N, nerve; IL, interleukin; IFN, interferon; TNF, tumor necrosis factor; MMP, matrix metalloproteinase; PG, prostaglandin; LT, leukotriene; NETs, neutrophil extracellular traps; CGRP, calcitonin gene-related peptide; SP, substance P; NKA, neurokinin A; NPY, neuropeptide Y.
3 Cytokines and chemokines
3.1 Proinflammatory cytokines
Immunocompetent cells such as macrophages eliminate bacteria and bacterial products that invade the pulp through the dentinal tubules, and activated macrophages produce various kinds of proinflammatory mediators (7). Typical proinflammatory cytokines such as interleukin (IL)-1, IL-6, IL-12, IL-18, interferon (IFN)-γ, and tumor necrosis factor (TNF)-α are predominantly synthesized by M1 macrophages in pulpal inflammation. Among these cytokines, elevated levels of IL-6 have been detected in various types of samples, including pulp tissue (12), pulp blood (13, 14), and dentinal fluid (15) obtained from inflamed pulps. This indicates that IL-6 is a candidate biomarker that can discriminate the condition of the pulp between healthy and irreversibly inflamed (16).
Clinically, pulpitis is often regarded as a chronic inflammatory lesion that may acutely transform when the body’s defense capabilities are compromised (17). Because of the chronic nature of pulpitis, a large number of T lymphocytes are found to accumulate in the inflamed pulp (18), and T lymphocyte-derived cytokines are also involved in the pathogenesis of pulpitis (7, 19). The principal Th1 effector cytokine is IFN-γ, which activates macrophages (20). Th17-derived cytokines include IL-17A, IL-17F, IL-21, and IL-22 (21), and the presence of IL-17 has been reported in inflamed pulp tissue (22).
3.2 Anti-inflammatory cytokines
Key Th2 cytokines include IL-4, IL-5, IL-10, and IL-13 (23). In particular, IL-10 possesses potent anti-inflammatory properties, and it plays a central role in limiting the host immune response to pathogens (24). IL-10 is also produced by macrophages of the M2 type, which are primarily involved in wound healing and tissue repair and are classified into several subtypes (M2a-d) (25). The dynamics of these cells in pulpitis have not yet been fully elucidated. Levels of IL-10 in pulp blood are significantly higher in samples from cariously exposed pulps and irreversibly inflamed pulps compared with those from normal pulps (Elsalhy et al., 2013). These higher levels may indicate that IL-10 acts to dampen any excessive inflammatory reaction before the establishment of irreversibly inflamed pulp status.
3.3 Chemokines
Cytokines with the property of inducing migration of inflammatory/immunocompetent cells are specifically referred to as chemokines. Chemokines are classified into four subclasses (CCL, CXCL, CX3CL, and XCL), and bind to G protein-coupled heptahelical chemokine receptors and induce migration of their target cells (26). Chemokines also possess key properties of macrophage activation and polarization to different functional phenotypes (M1, M2a, M2b, M2c) (27). Increased expression of IL-8, which is classified as a CXCL chemokine and is a powerful neutrophil chemoattractant, is detected in irreversibly inflamed human dental pulps (13, 28–31) indicating that IL-8 may be used as a biomarker for the diagnosis of irreversible pulpitis (16). In experimentally induced rat pulpitis, the kinetics of CXCL1 (GROa), CXCL2 (GROb), and CCL2 (MCP1) mRNA expression correlates with the infiltration of neutrophils, and CCL5 (RANTES) mRNA expression correlates with the infiltration of macrophages (32).
4 Nitric oxide, reactive oxygen species, and matrix metalloproteinases
Nitric oxide (NO), a small free radical, and reactive oxygen species (ROS) including superoxide and hydrogen peroxide, are effectors of the immune response and possess critical signaling roles in physiology and pathophysiology (33). The expression of nicotinamide adenine dinucleotide phosphate-diaphorase (NADPH-d), an indicator of nitric oxide synthase, is significantly higher in inflamed pulp tissues than in normal healthy pulp tissues (34). Acute pulpal inflammation in human teeth enhances the mRNA and protein levels of inducible nitric oxide synthase (iNOS) (35, 36), which acts as a key enzyme in inflammation and immune activation processes through the production of NO from L-arginine (37). ROS are molecules or ions formed by the incomplete one-electron reduction of oxygen and are key signaling molecules in the progress of inflammation (38). In particular, superoxide is involved in the regulation of autophagy (39).
Macrophage-derived proteases include subclasses of matrix metalloproteinases (MMPs), disintegrins and metalloproteinases (ADAMs), and TNF-α converting enzymes (TACE, ADAM17). MMPs are zinc-dependent proteases and are secreted by various types of cells, including fibroblasts, osteoblasts, endothelial cells, vascular smooth muscle cells, macrophages, neutrophils, lymphocytes, and cytotrophoblasts (40). MMPs are key enzymes in matrix degradation, and a functional balance between MMPs and tissue inhibitors of metalloproteinases regulates tissue degradation (41). An increase of MMP-1, MMP-8, and MMP-13 levels in chronic inflamed pulp has been observed (42). MMP-9, a neutrophil-derived MMP, is proposed as a local biomarker useful for distinguishing reversible and irreversible pulpitis because higher levels of MMP-9/total protein in pulpal fluid were significantly associated with the failure of direct pulp capping (43). However, the application of MMP-3 had anti-inflammatory effects on experimentally induced pulpitis in canines (44) and rats (45).
5 Proteases, neutrophil extracellular traps
Various proteases are produced during inflammation. When acute inflammation occurs, neutrophils infiltrate the front line of infection and are responsible for removing exogenous stimuli. Neutrophil-derived proteases include neutrophil-derived serine proteases (NSPs), neutrophil elastase (NE), and proteinase-3 (PR-3). Neutrophil proteases are mainly responsible for the intracellular killing of pathogens, but their extracellular release upon neutrophil activation is involved in tissue damage at the sites of inflammation (46). Neutrophils are equipped with bactericidal devices in the form of neutrophil extracellular traps (NETs). NETs consist of a meshwork of chromatin fibers composed of granule-derived antimicrobial peptides and enzymes such as neutrophil elastase, cathepsin G, and myeloperoxidase (47). NETs have been detected in inflamed pulp, and may contribute to disease progression (48).
6 Neuropeptides
Sensory nerves are abundantly distributed in the dental pulp tissue. Neuropeptides released from peripheral nerve terminals include calcitonin gene-related peptide (CGRP), substance P (SP), neurokinin A (NKA), vasoactive intestinal peptide (VIP), and neuropeptide Y (NPY). Increased expression of CGRP, SP, NKA, and NPY is detected in inflamed human pulp compared with that in healthy pulp (49). Furthermore, the mRNA expression of SP and its receptor, neurokinin-1 receptor, is detected in pulp fibroblasts (50). The local release of these vasoactive peptides is thought to cause neurogenic inflammation (51).
7 Eicosanoids
The eicosanoid family comprises 20-carbon polyunsaturated fatty acid metabolites (52). The classical eicosanoids, including prostaglandins (PGs), thromboxanes, leukotrienes (LTs), and hydroxy-, hydroperoxy-, epoxy- and oxo-eicosanoids, play a critical role in the regulation of inflammation (52). Elevated synthesis of PGE2, 6-keto-PGF1α (a stable metabolite of PGI2) (53), and LTB4 (54) is detected in experimentally induced rat dental pulp inflammation. An increase in the PGE2 level was detected in human pulps diagnosed as having reversible pulpitis (55). Eicosanoids are thought to essentially exacerbate inflammation, and the cyclooxygenase pathway involved in eicosanoid production is a major target for nonsteroidal anti-inflammatory drugs (NSAIDs) (56). Recently, the potential of these eicosanoids to induce the healing of pulpitis, including hard tissue induction, has been a focus for research. PGE2 alone is cytotoxic, but its inclusion in microspheres induces hard tissue marker expression in pulp cells (57). PGE2 induces cAMP production, which is mainly mediated by the EP2 receptor (58). Furthermore, EP2/EP4 agonists promote the generation of endothelial cell filopodia and upregulation of genes related to odontoblast differentiation (59). PGI2 induces MMP-9 production, which is expected to be involved in pulp tissue healing (60). Incorporation of LTB4 into the microsphere induces odontoblast differentiation and mineralization (61). Additionally, resolvin E1, an ω-3 polyunsaturated fatty acid (PUFA) metabolite and not an arachidonic acid metabolite, suppresses inflammatory mediator production and induces hard tissue marker expression in human dental pulp stem cells (DPSCs) (62).
Discussion
In pulpal inflammation, macrophages are mainly responsible for the production of inflammatory and anti-inflammatory cytokines. Macrophages are classified as M1 and M2 (63), and M2 macrophages are attracting attention for their function in healing and the termination of inflammation (64). Conditioned medium from M2 macrophages induces odontoblast marker expression in DPSCs (65), and hard tissue marker expression in dental pulp cells mainly through TGF-β (66). M2 polarization is reported to be induced by conditioned medium from human DPSCs (67), GSK-3β inhibitor small molecules (68), and an injectable dental pulp-derived decellularized matrix hydrogel (69). Calcium silicate materials, including mineral trioxide aggregate (MTA), which is currently the most popular direct pulp capping material, are reported to cause M2 polarization in vitro (70) and in vivo (71). Further studies on the development of methods and materials to induce M2 macrophage polarization and migration in pulp tissue are expected to be conducted.
MicroRNAs (miRNAs), which comprise a highly conserved group of small, non-coding RNA molecules, regulate gene expression primarily by silencing, and they play essential roles in the physiology and development of cells and tissues (72). We revealed that microRNA (miR)-21 and miR-146b are highly expressed in experimentally induced rat pulpitis and lipopolysaccharide-stimulated human dental pulp cells, and both miRNAs possess anti-inflammatory effects via downregulation of the NF-kB signaling pathway, a major cascade of proinflammatory mediator synthesis (73, 74). Various miRNAs involved in pulpal inflammation, including miR-21, have been reviewed by Muñoz-Carrillo et al. (75). Application of these miRNA mimics induces downregulation of proinflammatory signals/medications, which are the targets of pulpal inflammation control. Currently, the use of exosomal miRNAs is widely debated in relation to their therapeutic potential (76). Exosomes are cell-derived extracellular vesicles that promote cell−cell communication, and they may be used as suitable carriers for miRNA delivery.
Detecting mediators may allow us to infer the state of inflammation (77). The amount of MMP-9 in the leachate from the pulp surface is reported to be a promising biomarker that can indicate the inflammatory state of the pulp tissue (43). However, the relationship between the MMP-9 levels and pathological changes in the pulp is still unclear. Further research should be directed to identify appropriate biomarkers to evaluate the inflammatory state of the pulp and clearly show that the amount of the biomarker(s) correlates with the pathology of pulpitis.
Suppression of proinflammatory mediator production accompanied by elimination of bacterial infection seems to induce healing of pulpal inflammation. In experimentally induced rat pulpitis, application of a specific iNOS inhibitor can reduce macrophage infiltration into the pulp tissue and decrease the mRNA expression of pro-inflammatory cytokines and cyclooxygenase-2 (78, 79). However, inflammatory mediators may play a positive role as well as a negative one; some induce healing and promote the differentiation of odontoblasts (80). The transition to healing may be induced by the suppression of excessive production rather than complete suppression. Optimal regulation of mediator synthesis opens the way for healing of pulpal inflammation and recovery of the integrity of pulpal tissue (Figure 1).
Author contributions
NK: Writing – original draft, Writing – review & editing. TO: Writing – review & editing.
Funding
The author(s) declare financial support was received for the research, authorship, and/or publication of this article.
Grants-in-Aid for Scientific Research (KAKENHI) from the Japan Society for the Promotion of Science (JSPS), #24K12909ZA (N.K.), and #22K09960ZA (T.O.).
Acknowledgments
We thank Helen Jeays, BDSc, from Edanz (https://jp.edanz.com/ac) for editing a draft of this manuscript.
Conflict of interest
The authors declare that the research was conducted in the absence of any commercial or financial relationships that could be construed as a potential conflict of interest.
Publisher's note
All claims expressed in this article are solely those of the authors and do not necessarily represent those of their affiliated organizations, or those of the publisher, the editors and the reviewers. Any product that may be evaluated in this article, or claim that may be made by its manufacturer, is not guaranteed or endorsed by the publisher.
References
1. Kawashima N, Okiji T. Odontoblasts: specialized hard-tissue-forming cells in the dentin-pulp complex. Congenit Anom (Kyoto). (2016) 56(4):144–53. doi: 10.1111/cga.12169
2. Kawashima N. Characterisation of dental pulp stem cells: a new horizon for tissue regeneration? Arch Oral Biol. (2012) 57(11):1439–58. doi: 10.1016/j.archoralbio.2012.08.010
3. Okiji T, Kawashima N, Kosaka T, Matsumoto A, Kobayashi C, Suda H. An immunohistochemical study of the distribution of immunocompetent cells, especially macrophages and Ia antigen-expressing cells of heterogeneous populations, in normal rat molar pulp. J Dent Res. (1992) 71(5):1196–202. doi: 10.1177/00220345920710051201
4. Zhang J, Kawashima N, Suda H, Nakano Y, Takano Y, Azuma M. The existence of CD11c+ sentinel and F4/80+ interstitial dendritic cells in dental pulp and their dynamics and functional properties. Int Immunol. (2006) 18(9):1375–84. doi: 10.1093/intimm/dxl070
5. Kieu TQ, Tazawa K, Kawashima N, Noda S, Fujii M, Nara K, et al. Kinetics of LYVE-1-positive M2-like macrophages in developing and repairing dental pulp in vivo and their pro-angiogenic activity in vitro. Sci Rep. (2022) 12(1):5176. doi: 10.1038/s41598-022-08987-3
6. Endodontic Diagnosis (2013). Available online at: https://www.aae.org/specialty/wp-content/uploads/sites/2/2017/07/endodonticdiagnosisfall2013.pdf
7. Farges JC, Alliot-Licht B, Renard E, Ducret M, Gaudin A, Smith AJ, et al. Dental pulp defence and repair mechanisms in dental caries. Mediat Inflamm. (2015) 2015:230251. doi: 10.1155/2015/230251
8. Heyeraas KJ, Berggreen E. Interstitial fluid pressure in normal and inflamed pulp. Crit Rev Oral Biol Med. (1999) 10(3):328–36. doi: 10.1177/10454411990100030501
9. Fujii M, Kawashima N, Tazawa K, Hashimoto K, Nara K, Noda S, et al. HIF1α inhibits LPS-mediated induction of IL-6 synthesis via SOCS3-dependent CEBPβ suppression in human dental pulp cells. Biochem Biophys Res Commun. (2020) 522(2):308–14. doi: 10.1016/j.bbrc.2019.11.032
10. Fujii M, Kawashima N, Tazawa K, Hashimoto K, Nara K, Noda S, et al. Hypoxia-inducible factor 1α promotes interleukin 1β and tumour necrosis factor α expression in lipopolysaccharide-stimulated human dental pulp cells. Int Endod J. (2020) 53(5):636–46. doi: 10.1111/iej.13264
11. Marek-Jozefowicz L, Nedoszytko B, Grochocka M, Żmijewski MA, Czajkowski R, Cubała WJ, et al. Molecular mechanisms of neurogenic inflammation of the skin. Int J Mol Sci. (2023) 24(5):5001. doi: 10.3390/ijms24055001
12. Giuroiu CL, Vataman M, Melian G, Bularda D, Lozneanu L, Salceanu M, et al. Detection and assessment of interleukin 6 in irreversible pulp inflamation. Rev Chim-Bucharest. (2017) 68(2):323–7. doi: 10.37358/RC.17.2.5445
13. Elsalhy M, Azizieh F, Raghupathy R. Cytokines as diagnostic markers of pulpal inflammation. Int Endod J. (2013) 46(6):573–80. doi: 10.1111/iej.12030
14. Nakanishi T, Matsuo T, Ebisu S. Quantitative analysis of immunoglobulins and inflammatory factors in human pulpal blood from exposed pulps. J Endod. (1995) 21(3):131–6. doi: 10.1016/S0099-2399(06)80438-3
15. Brizuela C, Meza G, Mercade M, Inostroza C, Chaparro A, Bravo I, et al. Inflammatory biomarkers in dentinal fluid as an approach to molecular diagnostics in pulpitis. Int Endod J. (2020) 53(9):1181–91. doi: 10.1111/iej.13343
16. Karrar RN, Cushley S, Duncan HF, Lundy FT, Abushouk SA, Clarke M, et al. Molecular biomarkers for objective assessment of symptomatic pulpitis: a systematic review and meta-analysis. Int Endod J. (2023) 56(10):1160–77. doi: 10.1111/iej.13950
17. Giuroiu C, Căruntu I-D, Lozneanu L, Melian A, Vataman M, Andrian S. Dental pulp: correspondences and contradictions between clinical and histological diagnosis. BioMed Res Int. (2015) 2015:1–7. doi: 10.1155/2015/960321
18. Hahn CL, Falkler WA Jr., Siegel MA. A study of T and B cells in pulpal pathosis. J Endod. (1989) 15(1):20–6. doi: 10.1016/S0099-2399(89)80093-7
19. Annunziato F, Cosmi L, Liotta F, Maggi E, Romagnani S. Human Th1 dichotomy: origin, phenotype and biologic activities. Immunology. (2014) 144(3):343–51. doi: 10.1111/imm.12399
20. Gattorno M, Martini A. Chapter 3—the immune system and the inflammatory response. In: Cassidy JT, Petty RE, Laxer RM, Lindsley CB, editors. Textbook of Pediatric Rheumatology (Fifth Edition). Philadelphia: W.B. Saunders (2005). p. 19–63.
21. Eisenstein EM, Williams CB. The Treg/Th17 cell balance: a new paradigm for autoimmunity. Pediatr Res. (2009) 65(7):26–31. doi: 10.1203/PDR.0b013e31819e76c7
22. Liu M, Zhao Y, Wang C, Luo H AP, Ye L. Interleukin-17 plays a role in pulp inflammation partly by WNT5A protein induction. Arch Oral Biol. (2019) 103:33–9. doi: 10.1016/j.archoralbio.2019.05.003
23. Hohl TM. 6-Cell-mediated defense against infection. In: Bennett JE, Dolin R, Blaser MJ, editors. Mandell, Douglas, and Bennett’s Principles and Practice of Infectious Diseases (Eighth Edition). Philadelphia: W.B. Saunders (2015). p. 50–69.e6.
24. Iyer SS, Cheng G. Role of interleukin 10 transcriptional regulation in inflammation and autoimmune disease. Crit Rev Immunol. (2012) 32(1):23–63. doi: 10.1615/CritRevImmunol.v32.i1.30
25. Colin S, Chinetti-Gbaguidi G, Staels B. Macrophage phenotypes in atherosclerosis. Immunol Rev. (2014) 262(1):153–66. doi: 10.1111/imr.12218
26. Hughes CE, Nibbs RJB. A guide to chemokines and their receptors. FEBS J. (2018) 285(16):2944–71. doi: 10.1111/febs.14466
27. Mantovani A, Sica A, Sozzani S, Allavena P, Vecchi A, Locati M. The chemokine system in diverse forms of macrophage activation and polarization. Trends Immunol. (2004) 25(12):677–86. doi: 10.1016/j.it.2004.09.015
28. Silva AC, Faria MR, Fontes A, Campos MS, Cavalcanti BN. Interleukin-1 beta and interleukin-8 in healthy and inflamed dental pulps. J Appl Oral Sci. (2009) 17(5):527–32. doi: 10.1590/S1678-77572009000500031
29. Wu QQ, Li SS, Li R, Chen XJ, Guo LZ, Zheng Y. The detection of pro-inflammatory cytokines in exudates from dental pulp tissues. Cytokine. (2022) 153:155846. doi: 10.1016/j.cyto.2022.155846
30. Al-Natour B, Rankin R, McKenna R, McMillan H, Zhang S-D, About I, et al. Identification and validation of novel biomarkers and therapeutics for pulpitis using connectivity mapping. Int Endod J. (2021) 54(9):1571–80. doi: 10.1111/iej.13547
31. Dincer G A, Erdemir A, Kisa U. Comparison of neurokinin A, substance P, interleukin 8, and matrix metalloproteinase-8 changes in pulp tissue and gingival crevicular fluid samples of healthy and symptomatic irreversible pulpitis teeth. J Endod. (2020) 46(10):1428–37. doi: 10.1016/j.joen.2020.07.013
32. Kawashima N, Takahashi S, Ohi C, Okuhara S, Kawanishi HN, Suda H. Kinetics of chemokine mRNA expression in experimentally-induced rat pulitis. Jpn J Conserv Dent. (2005) 48(5):586–93.
33. Tejero J, Shiva S, Gladwin MT. Sources of vascular nitric oxide and reactive oxygen species and their regulation. Physiol Rev. (2019) 99(1):311–79. doi: 10.1152/physrev.00036.2017
34. da Silva LP, Issa JP, Del Bel EA. Action of nitric oxide on healthy and inflamed human dental pulp tissue. Micron (Oxford, England: 1993). (2008) 39(7):797–801. doi: 10.1016/j.micron.2008.01.018
35. Zamora R, Vodovotz Y, Billiar TR. Inducible nitric oxide synthase and inflammatory diseases. Mol Med. (2000) 6(5):347–73. doi: 10.1007/BF03401781
36. Di Nardo Di Maio F, Lohinai Z, D'Arcangelo C, De Fazio PE, Speranza L, De Lutiis MA, et al. Nitric oxide synthase in healthy and inflamed human dental pulp. J Dent Res. (2004) 83(4):312–6. doi: 10.1177/154405910408300408
37. Caldwell RW, Rodriguez PC, Toque HA, Narayanan SP, Caldwell RB. Arginase: a multifaceted enzyme important in health and disease. Physiol Rev. (2018) 98(2):641–65. doi: 10.1152/physrev.00037.2016
38. Mittal M, Siddiqui MR, Tran K, Reddy SP, Malik AB. Reactive oxygen species in inflammation and tissue injury. Antioxid Redox Signal. (2014) 20(7):1126–67. doi: 10.1089/ars.2012.5149
39. Chen Y, Azad MB, Gibson SB. Superoxide is the major reactive oxygen species regulating autophagy. Cell Death Differ. (2009) 16(7):1040–52. doi: 10.1038/cdd.2009.49
40. Wang X, Khalil RA. Chapter eight—matrix metalloproteinases, vascular remodeling, and vascular disease. In: Khalil RA, editor. Advances in Pharmacology. Academic Press (2018). 81. p. 241–330.
41. Cabral-Pacheco GA, Garza-Veloz I, Castruita-De la Rosa C, Ramirez-Acuña JM, Perez-Romero BA, Guerrero-Rodriguez JF, et al. The roles of matrix metalloproteinases and their inhibitors in human diseases. Int J Mol Sci. (2020) 21(24):9739. doi: 10.3390/ijms21249739
42. Evrosimovska B, Dimova C, Kovacevska I, Panov S. Concentration of collagenases (MMP-1, -8, -13) in patients with chronically inflamed dental pulp tissue. Prilozi. (2012) 33(2):191–204.23425881
43. Ballal NV, Duncan HF, Wiedemeier DB, Rai N, Jalan P, Bhat V, et al. MMP-9 levels and NaOCl lavage in randomized trial on direct pulp capping. J Dent Res. (2022) 101(4):414–9. doi: 10.1177/00220345211046874
44. Eba H, Murasawa Y, Iohara K, Isogai Z, Nakamura H, Nakamura H, et al. The anti-inflammatory effects of matrix metalloproteinase-3 on irreversible pulpitis of mature erupted teeth. PloS one. (2012) 7(12):e52523. doi: 10.1371/journal.pone.0052523
45. Takimoto K, Kawashima N, Suzuki N, Koizumi Y, Yamamoto M, Nakashima M, et al. Down-regulation of inflammatory mediator synthesis and infiltration of inflammatory cells by MMP-3 in experimentally induced rat pulpitis. J Endod. (2014) 40(9):1404–9. doi: 10.1016/j.joen.2014.04.001
46. Pham CTN. Neutrophil serine proteases fine-tune the inflammatory response. Int J Biochem Cell Biol. (2008) 40(6):1317–33. doi: 10.1016/j.biocel.2007.11.008
47. Kaplan MJ, Radic M. Neutrophil extracellular traps: double-edged swords of innate immunity. J Immunol. (2012) 189(6):2689–95. doi: 10.4049/jimmunol.1201719
48. Holder MJ, Wright HJ, Couve E, Milward MR, Cooper PR. Neutrophil extracellular traps exert potential cytotoxic and proinflammatory effects in the dental pulp. J Endod. (2019) 45(5):513–20. doi: 10.1016/j.joen.2019.02.014
49. Caviedes-Bucheli J, Lombana N, Azuero-Holguín MM, Munoz HR. Quantification of neuropeptides (calcitonin gene-related peptide, substance P, neurokinin A, neuropeptide Y and vasoactive intestinal polypeptide) expressed in healthy and inflamed human dental pulp. Int Endod J. (2006) 39(5):394–400. doi: 10.1111/j.1365-2591.2006.01093.x
50. Killough SA, Lundy FT, Irwin CR. Substance P expression by human dental pulp fibroblasts: a potential role in neurogenic inflammation. J Endod. (2009) 35(1):73–7. doi: 10.1016/j.joen.2008.10.010
51. Matsuda M, Huh Y, Ji RR. Roles of inflammation, neurogenic inflammation, and neuroinflammation in pain. J Anesth. (2019) 33(1):131–9. doi: 10.1007/s00540-018-2579-4
52. Yamaguchi A, Botta E, Holinstat M. Eicosanoids in inflammation in the blood and the vessel. Front Pharmacol. (2022) 13. doi: 10.3389/fphar.2022.997403
53. Okiji T, Morita I, Kobayashi C, Sunada I, Murota S. Arachidonic-acid metabolism in normal and experimentally-inflamed rat dental pulp. Arch Oral Biol. (1987) 32(10):723–7. doi: 10.1016/0003-9969(87)90116-6
54. Okiji T, Morita I, Sunada I, Murota S. The role of leukotriene B4 in neutrophil infiltration in experimentally-induced inflammation of rat tooth pulp. J Dent Res. (1991) 70(1):34–7. doi: 10.1177/00220345910700010501
55. Petrini M, Ferrante M, Ciavarelli L, Brunetti L, Vacca M, Spoto G. Prostaglandin E2 to diagnose between reversible and irreversible pulpitis. Int J Immunopathol Pharmacol. (2012) 25(1):157–63. doi: 10.1177/039463201202500118
56. Khanapure SP, Garvey DS, Janero DR, Letts LG. Eicosanoids in inflammation: biosynthesis, pharmacology, and therapeutic frontiers. Curr Top Med Chem. (2007) 7(3):311–40. doi: 10.2174/156802607779941314
57. Lorencetti-Silva F, Pereira PAT, Meirelles AFG, Faccioli LH, Paula-Silva FWG. Prostaglandin E2 induces expression of mineralization genes by undifferentiated dental pulp cells. Braz Dent J. (2019) 30(3):201–7. doi: 10.1590/0103-6440201902542
58. Chang MC, Lin SI, Lin LD, Chan CP, Lee MS, Wang TM, et al. Prostaglandin E2 stimulates EP2, adenylate cyclase, phospholipase C, and intracellular calcium release to mediate cyclic adenosine monophosphate production in dental pulp cells. J Endod. (2016) 42(4):584–8. doi: 10.1016/j.joen.2015.12.011
59. Ohkura N, Yoshiba K, Yoshiba N, Oda Y, Edanami N, Ohshima H, et al. Prostaglandin E(2)-transporting pathway and its roles via EP2/EP4 in cultured human dental pulp. J Endod. (2023) 49(4):410–8. doi: 10.1016/j.joen.2023.01.009
60. Seang S, Pavasant P, Everts V, Limjeerajarus CN. Prostacyclin analog promotes human dental pulp cell migration via a matrix metalloproteinase 9-related pathway. J Endod. (2019) 45(7):873–81. doi: 10.1016/j.joen.2019.03.020
61. da Silva FL, de Campos Chaves Lamarque G, de Oliveira F, Nelson-Filho P, da Silva LAB, Segato RAB, et al. Leukotriene B(4) loaded in microspheres regulate the expression of genes related to odontoblastic differentiation and biomineralization by dental pulp stem cells. BMC Oral Health. (2022) 22(1):45. doi: 10.1186/s12903-022-02083-8
62. Chen J, Xu H, Xia K, Cheng S, Zhang Q. Resolvin E1 accelerates pulp repair by regulating inflammation and stimulating dentin regeneration in dental pulp stem cells. Stem Cell Res Ther. (2021) 12(1):75. doi: 10.1186/s13287-021-02141-y
63. Martinez FO, Gordon S. The M1 and M2 paradigm of macrophage activation: time for reassessment. F1000Prime Rep. (2014) 6:13. doi: 10.12703/P6-13
64. Krzyszczyk P, Schloss R, Palmer A, Berthiaume F. The role of macrophages in acute and chronic wound healing and interventions to promote pro-wound healing phenotypes. Front Physiol. (2018) 9:419. doi: 10.3389/fphys.2018.00419
65. Kadowaki M, Yoshida S, Itoyama T, Tomokiyo A, Hamano S, Hasegawa D, et al. Involvement of M1/M2 macrophage polarization in reparative dentin formation. Life (Basel). (2022) 12(11):1812.36362965
66. Park HC, Quan H, Zhu T, Kim Y, Kim B, Yang HC. The effects of M1 and M2 macrophages on odontogenic differentiation of human dental pulp cells. J Endod. (2017) 43(4):596–601. doi: 10.1016/j.joen.2016.11.003
67. Yang Z, Ma L, Du C, Wang J, Zhang C, Hu L, et al. Dental pulp stem cells accelerate wound healing through CCL2-induced M2 macrophages polarization. iScience. (2023) 26(10):108043. doi: 10.1016/j.isci.2023.108043
68. Neves VCM, Yianni V, Sharpe PT. Macrophage modulation of dental pulp stem cell activity during tertiary dentinogenesis. Sci Rep. (2020) 10(1):20216. doi: 10.1038/s41598-020-77161-4
69. Yi P, Chen S, Zhao Y, Ku W, Lu H, Yu D, et al. An injectable dental pulp-derived decellularized matrix hydrogel promotes dentin repair through modulation of macrophage response. Biomater Adv. (2024) 161:213883. doi: 10.1016/j.bioadv.2024.213883
70. Abuarqoub D, Aslam N, Zaza R, Jafar H, Zalloum S, Atoom R, et al. The immunomodulatory and regenerative effect of biodentine on human THP-1 cells and dental pulp stem cells: In vitro study. Biomed Res Int. (2022) 2022:2656784. doi: 10.1155/2022/2656784
71. Ito T, Kaneko T, Yamanaka Y, Shigetani Y, Yoshiba K, Okiji T. M2 macrophages participate in the biological tissue healing reaction to mineral trioxide aggregate. J Endod. (2014) 40(3):379–83. doi: 10.1016/j.joen.2013.11.011
72. Ranganathan K, Sivasankar V. MicroRNAs—biology and clinical applications. J Oral Maxillofac Pathol. (2014) 18(2):229–34. doi: 10.4103/0973-029X.140762
73. Han P, Sunada-Nara K, Kawashima N, Fujii M, Wang S, Kieu TQ, et al. MicroRNA-146b-5p suppresses pro-inflammatory mediator synthesis via targeting TRAF6, IRAK1, and RELA in lipopolysaccharide-stimulated human dental pulp cells. Int J Mol Sci. (2023) 24(8):7433. doi: 10.3390/ijms24087433
74. Nara K, Kawashima N, Noda S, Fujii M, Hashimoto K, Tazawa K, et al. Anti-inflammatory roles of microRNA 21 in lipopolysaccharide-stimulated human dental pulp cells. J Cell Physiol. (2019) 234(11):21331–41. doi: 10.1002/jcp.28737
75. Muñoz-Carrillo JL, Vázquez-Alcaraz SJ, Vargas-Barbosa JM, Ramos-Gracia LG, Alvarez-Barreto I, Medina-Quiroz A, et al. The role of microRNAs in pulp inflammation. Cells. (2021) 10(8):2142. doi: 10.3390/cells10082142
76. Hu G, Drescher KM, Chen X. Exosomal miRNAs: biological properties and therapeutic potential. Front Genet. (2012) 3.22529849
77. Rechenberg DK, Galicia JC, Peters OA. Biological markers for pulpal inflammation: a systematic review. PLoS One. (2016) 11(11):e0167289. doi: 10.1371/journal.pone.0167289
78. Kawashima N, Nakano-Kawanishi H, Suzuki N, Takagi M, Suda H. Effect of NOS inhibitor on cytokine and COX2 expression in rat pulpitis. J Dent Res. (2005) 84(8):762–7. doi: 10.1177/154405910508400815
79. Kawanishi HN, Kawashima N, Suzuki N, Suda H, Takagi M. Effects of an inducible nitric oxide synthase inhibitor on experimentally induced rat pulpitis. Eur J Oral Sci. (2004) 112(4):332–7. doi: 10.1111/j.1600-0722.2004.00139.x
Keywords: inflammation, mediators, dental pulp, inflammatory cells, inflammatory control
Citation: Kawashima N and Okiji T (2024) Characteristics of inflammatory mediators in dental pulp inflammation and the potential for their control. Front. Dent. Med 5:1426887. doi: 10.3389/fdmed.2024.1426887
Received: 2 May 2024; Accepted: 24 July 2024;
Published: 6 August 2024.
Edited by:
Carla Sipert, University of São Paulo, BrazilReviewed by:
Emi Shimizu, The State University of New Jersey, United StatesFrancisco W. G. Paula-Silva, University of São Paulo, Brazil
© 2024 Kawashima and Okiji. This is an open-access article distributed under the terms of the Creative Commons Attribution License (CC BY). The use, distribution or reproduction in other forums is permitted, provided the original author(s) and the copyright owner(s) are credited and that the original publication in this journal is cited, in accordance with accepted academic practice. No use, distribution or reproduction is permitted which does not comply with these terms.
*Correspondence: Nobuyuki Kawashima, a2F3YXNoaW1hLm4uZW5kb0B0bWQuYWMuanA=