- 1Nunn Biostatistical Solutions, Omaha, NE, United States
- 2Private Practitioner, Omaha, NE, United States
- 3Tokyo Dental College, Tokyo, Japan
- 4Private Practitioner, Tokyo, Japan
- 5Department of Periodontics, Creighton University School of Dentistry, Omaha, NE, United States
The objectives of this study are to provide a systematic review of a novel alloplastic hard-tissue grafting material, carbonate apatite granules (CO3Ap-granules), to provide a clinical case presentation of CO3Ap-granules in periodontal surgery. The following three electronic databases were searched independently by two of the authors (MN) and (CR): National Library of Medicine [MEDLINE (PubMed) and ClinicalTrials.gov], EMBASE (OVID) and the Cochrane Central Register of Controlled Trials (CENTRAL). After searching electronic databases, select journals in periodontics and implantology were also manually searched. Of the 43 studies identified from the systematic review, the following classifications were determined: (1) in vitro studies – 5 studies, (2) animal studies – 28 studies, (3) clinical studies – 7 studies, (4) reviews – 3 studies. Results from selected animal studies and all human studies were summarized. These results demonstrate that the novel alloplast CO3Ap-granules has the potential ability to stimulate new bone formation while CO3Ap-granules simultaneously resorb over time. Replacement of CO3Ap-granules with new bone formation has been shown to be comparable to autogenous bone grafting with one study showing superior results to a bovine-derived xenograft.
1 Introduction
Hard-tissue grafting is integral to the practice of periodontics, including repair of alveolar bone (1), periodontal pocket elimination, gain in periodontal attachment, sinus augmentations, alveolar ridge preservation (2, 3), alveolar ridge augmentation (4), an adjunct to implant placement (5), to facilitate socket healing with placement of immediate implants (5, 6), and in treatment of peri-implantitis (7). Different types of hard-tissue grafts include autografts, allografts, xenografts, and alloplasts (6, 8, 9). Autografts are defined as tissue moved from one location to another site within the same species. Allografts are defined as tissue moved from one person to another genetically dissimilar person, such as freeze-dried bone allografts (FDBA) or demineralized freeze-dried bone (DFDBA). While allografts are widely used in the field of periodontics, risks from allografts include antigenicity and risk of infection (8), although freeze-drying markedly reduces these risks (10, 11). Xenografts are defined as tissue transferred from one species to another species, such as bovine-derived hard-tissue graft. The deproteinized bovine bone matrix (DBBM) is mainly xenograft materials used in alveolar bone defects and in sinus floor augmentation (included citations). DBBM consists of hydroxyapatite prepared by alkaline and heat treatment for eliminating the organics of the bone. However, bovine-derived graft biomaterials may theoretically carry a risk of prion/bovine spongiform encephalopathy transmission to patients (6, 12).
In contrast to autografts, allografts, and xenografts, an alloplast is completely artificial. Hence, alloplasts are defined as artificial tissue, and since alloplasts are not dependent on tissues derived from living beings, such as allografts from other humans or xenografts from animals, the availability of alloplasts is virtually unlimited since the single limiting factor is the least available material used to fabricate an alloplast. Alloplastic graft is usually made from hydroxyapatite, which is a natural mineral component of bone. Thus, they have no risk for cross infection or disease transmission, which might be a possibility with the use of allografts and xenografts (6, 8, 9). If an alloplast has similar properties to an autograft, such as osteoconductivity and osteoinductivity, that alloplast would be the perfect solution for bone grafting. Early bone substitutes included hydroxyapatite [HAp: Ca10(PO4)6-(OH)2], β-tricalcium phosphate [β-TCP: Ca3(PO4)2], biphasic calcium phosphate, BCP; a mixture of HAp and β-TCP, calcium sulfate [CaSO4·2H2O], and bioglass (13–15).
CO3Ap-granules are a novel alloplastic material that are capable of inducing new bone generation, while also resorbing from the graft site, leaving an area of newly formed bone and blood vessels, without any remaining graft material (15–17). One of the unique ways CO3Ap-granules accomplish this is through the activation of both osteoclasts and osteoblasts from osteoprogenitor cells within the graft site (18). CO3Ap-granules induce the transcription of osteoclast differentiation markers such as cathepsin K and tartrate-resistant acid phosphatase allowing osteoclasts to develop in direct contact with the CO3Ap-granules without any intermediary layers of fibrous tissue (19). Both the carbonate apatite and newly differentiated osteoclasts in turn activate osteoblast differentiation via upregulation of alkaline phosphatase, osteopontin, and osteocalcin (16–18, 20). Osteoblasts near CO3Ap-granules lay down new type I collagen, the most abundant bone matrix protein expressed in both mature bone and as the main organic component of immature bone, indicating the osteoinductive capabilities of the granules (17, 18). The presence of osteocalcin and osteopontin found in the newly formed bone around CO3Ap-granules indicates extracellular matrix synthesis and bone matrix mineralization necessary for strong replacement bone (18). The CO3Ap-granules act as a scaffold for both osteogenesis and angiogenesis to promote vascularization while allowing autogenous bone to grow adjacent to, and eventually replace, the CO3Ap-granules (17, 20). In addition to inducing osteoclast differentiation, CO3Ap-granules release certain ions in aqueous solutions, namely carbonic acid, phosphoric acid, and calcium, which reduce the pH of the immediate area promoting osteoclast activity. Because CO3Ap-granules are stable at physiologic pH, but dissolve at low pH, the release of ions allows both bone remodeling and granule dissolution to occur at the same time (16, 17, 20).
A successful hard-tissue graft involves applying the hard-tissue grafting material, a period of healing, the hard-tissue grafting material becoming incorporated into the bone, revascularization, and finally, assuming the form desired by the clinician (6). Early hard-tissue grafts were viewed as lattice structures with the success of these bone-like lattice structures judged primarily by the mechanical stress that could be applied to the area containing a hard-tissue graft (16, 17, 20). Modern hard-tissue grafts are treated as biological structures. Mechanical stress, shear stress, contouring, and regeneration of new bone in the remodeling stage are all part of the long-term healing of modern hard-tissue grafts (8).
The purpose of this paper is to provide a systematic review of a novel alloplast, CO3Ap-granules (Cytrans Granules®: GC America, Alsip, IL), and to demonstrate a clinical case with long term outcome utilizing carbonate apatite granules in periodontal regeneration. For background, a brief overview of the different types of hard-tissue grafts used in periodontics and implantology is provided as Supplementary Material.
2 Materials and methods
This systematic review was conducted in accordance with the Preferred Reporting Items for Systematic Reviews and Meta-Analysis (PRISMA) and Cochrane guidelines. Supplementary Diagram 1 shows the PRISMA flowchart and PRISMA checklist. The following focus question is based on population, intervention, comparison, and outcome (PICO) as shown in Table 1.
What is the effect of CO3Ap-granules intraoral graft when compared to unassisted healing or other intraoral bone graft materials?
An initial electronic systematic search was performed independently by two of the authors (MN) and (CR). The following electronic databases were searched: National Library of Medicine [MEDLINE (PubMed) and ClinicalTrials.gov], EMBASE (OVID) and the Cochrane Central Register of Controlled Trials (CENTRAL).
The following search strategy was designed for the MEDLINE (PubMed) database and then modified accordingly for other database engines: (“carbonate apatite” or “carbonated apatite” or “Cytrans”) and (“periodontal surgery” or “periodontology” or “implant” or “oral surgery”). The most recent electronic database search was conducted on November 1, 2023. In addition to the electronic search, further manual search of the following journals from 2012 to November 1, 2023 was also conducted: Journal of Periodontology, Journal of Clinical Periodontology, Clinical Oral Implants Research, Journal of Dental Research, International Journal of Oral and Maxillofacial Implants, International Journal of Oral and Maxillofacial Surgery, and International Journal of Periodontics and Restorative Dentistry.
Following the initial systematic search, all the titles and abstracts were scanned independently by two investigators (MN and CR), followed by the full-text assessment of the potentially eligible studies. In case of any disagreement regarding study selection, a third investigator (TM) was contacted. Studies unrelated to using CO3Ap-granules as a hard-tissue grafting material were eliminated.
3 Results
3.1 Types of publications
Publications we encountered in our systematic review are classified into four categories: (1) in vitro studies (5 studies), (2) animal studies (28 studies), (3) human clinical studies (7 studies), and (4) review articles (3 studies). For the purposes of this systematic review, we will focus on the second and third categories with particular attention to human clinical studies. Tables of all studies involving CO3Ap-granules, used as alloplast grafting material, are shown in Table 2 (in vitro studies), Table 3 (animal studies), Table 4 (human clinical studies), and Table 5 (review articles). Normally, systematic reviews are restricted to human clinical studies. However, because of the large number of animal studies and a very limited number of human clinical studies, all animal studies and all human clinical studies are presented in Tables 3, 4. A subset of the most significant animal studies (10 of 28 animal studies) and all human clinical studies are presented in the results.
3.2 Significant animal studies (10 of 28 animal studies presented)
In a study comparing CO3Ap-granules to sintered hydroxyapatite in defects created in the tibia of rats, it was found that CO3Ap-granules more closely mimicked the bone matrix with direct apposition of new bone via osteoblasts and osteoclastic resorption with new bone replacing CO3Ap-granules (21). In another study that compared the healing sockets of mandibular incisors in rats where CO3Ap-granules with and without statin were compared to hydroxyapatite (Neobone®, CoorsTek, Tokyo, Japan), with and without statin and an untreated control, CO3Ap-granules combined with statins produced the greatest bone mineral density, which suggests that CO3Ap-granules with statins may promote bone healing in the socket and may be applicable to alveolar bone preservation following tooth extraction (22). In another study of the molar sockets of rats, CO3Ap-granules was compared to autogenous bone and control. After 5 days, osteoclasts were observed near the CO3Ap-granules and the bone thickness observed for the CO3Ap-granules bone sockets was similar to autogenous bone sockets. And as observed from previous rat studies, new bone completely replaced CO3Ap-granules (17). In another study of the soft-tissue healing of molar sockets of rats where either hydroxyapatite or carbonate apatite was placed in the sockets, carbonate apatite was found to promote soft-tissue healing by accelerating wound closure with connective tissue compared to hydroxyapatite (23). In a 2023 study on the healing of rat extraction sockets, researchers focused on mesenchymal stem cell activity, which is thought to have a key role in wound healing. In this study of rat extraction sockets, they compared CO3Ap-granules, hydroxyapatite, and β-tricalcium phosphate (Cerasorb®, Curasan Inc., Frankfurt, Germany), with CO3Ap-granules producing overall greater mesenchymal stem cell activity, which suggests that CO3Ap-granules promote wound healing in the oral cavity (24).
In a study comparing CO3Ap-granules to a bovine-derived xenograft in rabbit femur defects, at 4 weeks, CO3Ap-granules outperformed the bovine-derived xenograft with a significantly larger amount of new bone deposited while CO3Ap-granules spontaneously resorbed as it was replaced by new bone. At 8 weeks, bovine-derived granules showed no change in size compared to CO3Ap-granules that gradually decreased in size because they were replaced with bone. However, the difference in new bone formation between CO3Ap-granules and bovine-derived granules at 8 weeks was not statistically significant (25). In a 2019 study of rabbit femur defects by Hayashi, et al., CO3Ap-granules, hydroxyapatite, and β-tricalcium phosphate were compared. Again, CO3Ap-granules outperformed both hydroxyapatite and β-tricalcium phosphate with CO3Ap-granules having 14.3-fold and 4.3-fold greater mature bone at 4 weeks compared to hydroxyapatite and β-tricalcium phosphate, respectively, and 7.5-fold and 1.4-fold higher mature bone at 12 weeks. In addition, CO3Ap-granules readily resorbed and were replaced by new bone while hydroxyapatite never resorbed (26). In a 2019 study by Ishikawa, et al., where a rabbit femur defect was filled with carbonate apatite honeycomb blocks, new bone completely penetrated the carbonate apatite honeycomb blocks. Osteoclasts and osteoblasts were also found on the newly formed bone (27).
In a hybrid dog mandible defect model, a comparison was made among CO3Ap-granules, hydroxyapatite, and β-tricalcium phosphate hard-tissue grafts. As in the rabbit model, CO3Ap-granules demonstrated the highest level of bone formation (28). A comparison of healing of vertical bone defects in beagle dogs was conducted to compare CO3Ap-granules, bovine-derived xenograft, β-tricalcium phosphate, hydroxyapatite, and a sham control. At 4 weeks, CO3Ap-granules demonstrated significantly greater bone formation than either the sham control group or the bovine-derived xenograft group. CO3Ap-granules also tended to have greater bone formation than either β-tricalcium phosphate or hydroxyapatite, although these differences did not achieve statistical significance. This study indicates that CO3Ap-granules initially promoted rapid bone formation, and hence, CO3Ap-granules may be a reliable bone substitute in the treatment of vertical bone defects as the result of periodontitis (29).
3.3 Human clinical studies
In the first human clinical trial in 2019, two sizes of CO3Ap-granules were used in 8 subjects for a sinus floor augmentation while simultaneously placing implants. The initial mean maxillary molar bone height was 5.2 ± 0.8 mm preoperatively with the post-augmentation height being 14.0 ± 1.9 mm. At 12 months, the mean maxillary bone height was 11.7 ± 0.6 mm so that total resorption after a year was 16.4%, which compares favorably with historical controls of autogenous bone augmentation and autogenous bone, bovine-derived xenograft mixture augmentation with resorption of 23.1% and 18.9%, respectively (30). This first clinical trial suggests that CO3Ap-granules is a safe, useful bone substitute for sinus floor augmentation (20).
In a two-stage sinus floor augmentation clinical study, 13 subjects with 17 implants were enrolled. The mean residual bone height was 3.5 ± 1.3 mm with a mean post-augmentation height of 13.3 ± 1.7 mm achieved with CO3Ap-granules. Mean bone height was found to be 10.7 ± 1.9 mm at 7 ± 2 months and 9.6 ± 1.4 mm at 18 ± 2 months for mean resorption of 19.5% and 27.8% at 7 months and 18 months, respectively. Histological examination demonstrated new bone and residual CO3Ap-granules of 33.8% ± 15.1% and 15.3% ± 11.9%, respectively, at 7 months (19). The rate of new bone formation from autogenous cortical bone alone is approximately 40%–50% (31, 32) and from bovine-derived xenograft is approximately 20%–30% (32–34). Hence, results from two-stage sinus floor augmentation compare positively with autogenous bone augmentation and bovine-derived xenograft only sinus augmentation. At 10 ± 2 months, sinus floor augmentation with CO3Ap-granules exhibited a reduction in elevated bone height (EBH) of 10.3% (19). This compares favorably with reduction in EBH for autogenous cortical bone augmentation of 14.4% in the iliac crest group at 5.5 months (35), reduction in EBH for autogenous bone in the mandible group of 8.4% at 5.5 months (35), and reduction in EBH for bovine-derived xenograft augmentation alone at 10 months of 6.5% (36).
A 3-year follow-up of the initial 2-stage sinus augmentation with CO3Ap-granules above was conducted with results revealing 100% implant survival without complications. Histological analyses at 7-month follow-up revealed mature new bone of 36.8% ± 17.3% and residual CO3Ap-granules of 16.2% ± 10.1% (37). These results compared quite favourably with a 9-month follow-up of bovine-derived xenograft with mature new bone of 19% and residual bovine-derived xenograft of 40% (38).
A clinical trial was conducted that compared CO3Ap-granules to deproteinized bovine-derived xenograft for sinus lift augmentation with 6 subjects receiving CO3Ap-granules sinus lift augmentation and 8 subjects receiving deproteinized bovine-derived xenograft sinus lift augmentation. At 6 months, the CO3Ap-granules group demonstrated significantly less bone resorption (p < 0.001) compared to the deproteinized bovine-derived xenograft group with 14.2% bone resorption for the CO3Ap-granules group and 24.2% bone resorption for the deproteinized bovine-derived xenograft group (39). Future clinical trials should be conducted to compare the relative efficacy of CO3Ap-granules and bovine-derived xenograft in other periodontal applications, such as immediate implant placement and as an adjunct to extraction site healing for eventual implant placement.
A clinical study was conducted to test the safety and efficacy of 0.3% basic fibroblast growth factor (REGROTH®, Kaken Pharmaceutical Co., Ltd., Tokyo, Japan) in combination with CO3Ap-granules in the treatment of severe alveolar bone defects as the result of moderate-to-severe periodontitis in 10 subjects. Currently, a flap operation (FO) in conjunction with fibroblast growth factor (FGF) has been the standard procedure for periodontal regenerative therapy in the treatment of alveolar bone defects in Japan. However, FGF has been inadequate in the treatment of severe alveolar bone defects. Hence, Kitamura, et al., conducted a clinical study of FGF and CO3Ap-granules to test the safety and efficacy of these two materials used together to treat severe alveolar bone defects using flap operations in these procedures. With the primary endpoint of safety, there were no adverse effects. In terms of secondary clinical endpoints at 36 weeks, significant increase in alveolar bone (p = 0.003), significant increase in CAL (p = 0.001), significant decrease in periodontal probing depth (p = 0.002), and significant decrease in bleeding on probing (p = 0.016) were observed compared to preoperative values. Future clinical studies comparing the combination of FGF and CO3Ap-granules to FGF alone and CO3Ap-granules alone need to be conducted to validate that the addition of CO3Ap-granules to FGF is advantageous in regenerating alveolar bone in the treatment of severe alveolar bone defects (40).
In a study published in 2023, a one-arm, single-center prospective trial was conducted to test the safety and efficacy of CO3Ap-granules in patients with periodontitis. Four patients with seven periodontitis sites were included in the study with the following sites included in the study: 3 deep intrabony defects, 2 class II furcation involvements, and 2 class III furcation involvements. At 9 months, reductions in probing depth were 5.0 ± 1.0 mm, 4.5 ± 0.7 mm, and 1.5 ± 0.7 mm for intrabony defects, class II furcation involvements, and class III furcation involvements, respectively. At 9 months, neither class III furcation involvements changed in terms of furcation classification. At 9 months, one class II furcation involvement improved to class I furcation involvement while the other class II furcation involvement was completely closed to eliminate furcation involvement (41).
Finally, the short-term histological results from three case reports for (1) an immediate implant placement, (2) a lateral guided bone regeneration (GBR), and (3) a vertical guided bone regeneration (GBR) demonstrated the efficacy of CO3Ap-granules in these three applications. In all three applications, new bone was produced while the original grafts of CO3Ap-granules were resorbed. In the limited results of these three short-term case reports, the novel alloplast CO3Ap-granules appears to be safe and effective. However, larger, long-term human clinical trials need to be conducted to validate results observed here and in the other human studies described previously (42).
3.4 Human case report of carbonate apatite crystals
In this case report, a 65-year-old male patient was seen by the same periodontal office (MN) over a 10-year period for supportive periodontal regenerative therapy. The patient was presented with a 10-mm periodontal probing depth localized to the facial of tooth number 8 – upper right central incisor (Figures 1–3). Initially, the etiology for this deep probing depth was thought to be a root fracture, which would have required the tooth to be extracted. However, the patient reported no other symptoms of a potential root fracture, so the area was examined using a periodontal endoscope. The periodontal endoscopy showed cemental tears resent with one large portion embedded in the facial gingiva adjacent to the bone defect (Figure 4). The separated cementum was removed using an ultra-sonic scaler (Figure 5). The area was then treated surgically to regenerate the alveolar bony defect. After flap elevation, additional cementum particles were removed, and the area was thoroughly debrided. FGF was applied over the root surface to expedite the keratinocyte epithelial-mesenchymal transition, and CO3Ap-granules were grafted into the area. In this case, CO3Ap-granules were held in place by the sutured gingiva with no additional material necessary for support or retention. At 6 months periodontal reevaluation visit, the periodontal probing depth had been reduced to 4 mm with no bleeding on probing. At the same appointment, a CT scan confirmed newly generated labial bone formation from the resorbed CO3Ap-granules at tooth number 8 (Figure 6). After 12 months, the periodontal probing depth remained stable, and a new zirconia crown was placed accordingly.
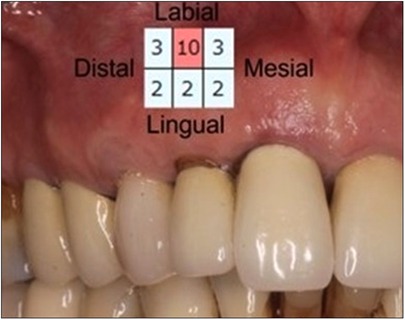
Figure 1. Clinical photograph of patient at regular supportive periodontal therapy appointment when 10 mm probing depth was detected. Insert shows recorded probing depths for all surfaces of #8.
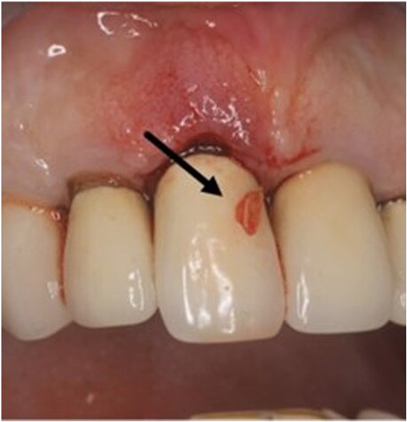
Figure 2. Clinical photograph of one of the cemental tear particles (black arrow) removed during ultrasonic scaling.
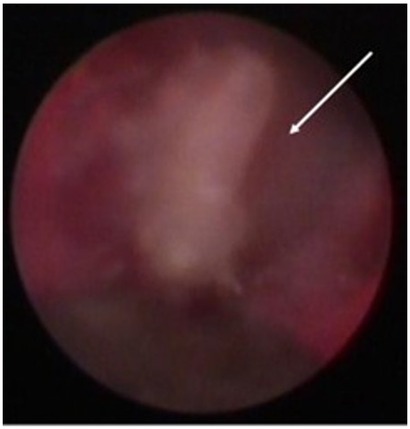
Figure 3. Screen grab from video taken with the periodontal endoscope showing a cemental tear particle (white arrow) embedded in the inner lining of the periodontal pocket causing inflammation and bone loss.
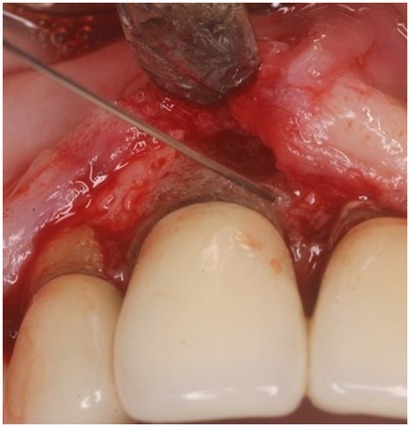
Figure 4. Clinical photograph showing vertical bone defect of #8 after flap resection and prior to debridement or grafting.
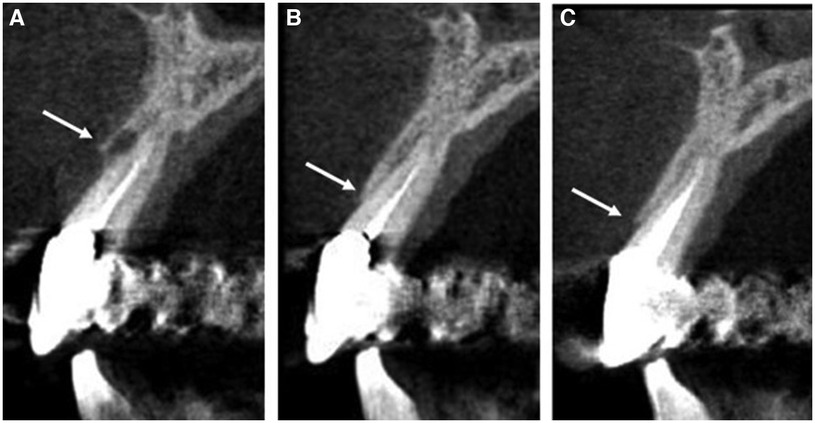
Figure 6. Computed tomography images showing bone levels (indicated by white arrows) at (A) initial presentation of pocketing during supportive periodontal therapy appointment with notable bone loss on the facial surface of #8, (B) 6-month follow up with visible bone regeneration and resorption of the carbonate apatite granules, (C) additional growth of regenerated bone at 18-month follow-up appointment.
4 Discussion
The brief overview of existing hard-tissue grafting materials used in periodontics (shown in Supplementary Material), the systematic review of CO3Ap-granules, and the case report presented here demonstrate the potential advantages of the novel alloplastic hard-tissue grafting material, CO3Ap-granules, in periodontal regeneration. As pointed out in the brief overview of hard tissue grafting materials used in periodontics, autogenous bone grafts, previous allografts, and xenografts all have significant drawbacks when applied to periodontal surgery. Hence, a need for a safe, effective alloplastic osseous grafting material that can be applied to a wide variety of procedures within the field of periodontics has long existed (6, 8, 9).
Since the beginning of hard-tissue grafting in dentistry, clinicians and scientists have attempted to develop a hard-tissue grafting material with the most benefit and least risk to the patient. Currently, autografts are the ideal material because of its excellent osteoinductive and osteoconductive properties without the minimum risk of graft rejection. However, the primary drawbacks to autografts are limited source material, increased morbidity, and prolonged healing time for the patient from the harvesting site (6, 8, 9). The next best option to autografts would be an alloplastic material with the following properties: (1) has little to no risk of antigenicity or graft rejection, (2) can induce the generation of new bone, and (3) resorbs as new bone is formed. Previously, an alloplast with all these properties did not exist. Prior alloplasts failed to stimulate the formation of sufficient new bone while the alloplastic hard-tissue graft simultaneously remained in the grafting site to create problems (19). The lack of suitable alloplasts has left the dental profession with only two clinically viable options for hard-tissue grafts: xenografts and allografts, both of which have limitations. If an alloplastic material were developed which could induce both osteoclastic and osteoblastic function to promote the creation of new bone, could resorb over time as new bone is generated, and could promote angiogenesis to maintain the health and viability of the newly formed bone, the need for xenografts and allografts would virtually be eliminated, which, in turn, would drastically limit the risks to patients in need of alveolar bone regeneration.
Following Japan government approval in December, 2017 and market introduction in February, 2018, this novel alloplast, CO3Ap-granules, has been shown to be a consistently reliable hard-tissue grafting material in Japan with CO3Ap-granules becoming the most widely accepted dominant hard-tissue grafting material in Japan in 2022 (43). In addition, CO3Ap-granules received the prestigious “1st Japan Open Innovation Prize” in March, 2019, with only 12 out of 212 projects obtaining that recognition, which was established to promote and accelerate innovations in Japan (44). In August, 2020, the U.S. Food and Drug Administration (FDA) approved CO3Ap-granules as a hard-tissue grafting material with multiple dental applications (45). The official introduction of CO3Ap-granules to the United States market occurred in February, 2022 (43). The results of both animal and human studies demonstrate that CO3Ap-granules provide clinical results that are at least comparable to all other types of available hard-tissue grafting material (21, 23, 24). One of the clinical advantages of CO3Ap-granules is its ability to simultaneously resorb as new bone is deposited to replace the alloplastic hard-tissue graft. Both histologic studies and radiographic studies using CT radiography have demonstrated the utility of CO3Ap-granules in resorbing as new bone is acquired over time (19, 20).
There has long been a need for an effective, safe alloplastic osseous grafting material in periodontics. The applications for such an alloplast include repair of lost alveolar bone, elimination of periodontal pockets, clinical attachment regeneration, sinus floor augmentation, alveolar ridge augmentation, and as an adjunct in implant placement, particularly with sinus lifts, alveolar ridge augmentation, and to facilitate socket healing with placement of immediate implants. Previously, both xenografts, such as bovine-derived xenografts, and alloplasts, such as hydroxyapatite, have been limited in terms of resorption so that a high percentage of the original hard-tissue grafting material often remained in the surgical site. Based on both animal studies and clinical studies in humans, the amount of resorption of CO3Ap-granules and replacement with new bone is comparable to autogenous bone grafts with an average of 33.8% new bone at CO3Ap-granules sites at 7 months (19) compared to an average of 41.0% new bone at autogenous bone sites at 6 months (31) in human studies. In contrast, bovine-derived xenografts sites demonstrated an average of only 22.9% new bone at 8 months (34).
Taken together, the cumulative evidence provided by animal studies, human clinical studies, and the overwhelming acceptance of CO3Ap-granules in the Japanese dental market with steady growth in market share since 2018 demonstrates that CO3Ap-granules may be a hard-tissue grafting material worthy of clinical use and further clinical investigation (43). Overall, CO3Ap-granules can be promising allograft with multiple potential applications in periodontal surgery and oral surgery where a hard-tissue graft is potentially needed, including the repair of lost alveolar bone, periodontal alveolar reconstruction, gain in periodontal clinical attachment, alveolar ridge augmentation, as an adjunct in implant placement, and in the treatment of peri-implant disease.
The single greatest drawback of CO3Ap-granules is the lack of large randomized controlled clinical trials that would test the effectiveness of CO3Ap-granules compared to allografts, xenografts, and sham controls in a variety of periodontal hard-tissue grafting applications. It is suggested that future study is needed to demonstrate clinical efficacy and effectiveness of CO3Ap-granules through multi-center randomized controlled clinical trials.
Data availability statement
The original contributions presented in the study are included in the article/Supplementary Material, further inquiries can be directed to the corresponding author.
Author contributions
MN: Conceptualization, Data curation, Formal Analysis, Funding acquisition, Investigation, Methodology, Project administration, Resources, Software, Supervision, Validation, Visualization, Writing – original draft, Writing – review & editing. CR: Data curation, Investigation, Validation, Writing – original draft, Writing – review & editing. MN: Data curation, Resources, Writing – original draft, Writing – review & editing. TM: Conceptualization, Data curation, Investigation, Methodology, Supervision, Validation, Writing – original draft, Writing – review & editing.
Funding
The author(s) declare that no financial support was received for the research, authorship, and/or publication of this article.
Acknowledgments
The authors gratefully acknowledge Mark Carlascio, Mark Heiss, and Yusuke Shigemitsu of GC Dental for information and assistance they provided during the preparation of this manuscript.
Conflict of interest
The authors declare that the research was conducted in the absence of any commercial or financial relationships that could be construed as a potential conflict of interest.
Publisher's note
All claims expressed in this article are solely those of the authors and do not necessarily represent those of their affiliated organizations, or those of the publisher, the editors and the reviewers. Any product that may be evaluated in this article, or claim that may be made by its manufacturer, is not guaranteed or endorsed by the publisher.
Supplementary material
The Supplementary Material for this article can be found online at: https://www.frontiersin.org/articles/10.3389/fdmed.2024.1418039/full#supplementary-material
References
1. de Almeida CS, Sartoretto SC, Durte IM, Alves ATNN, Barreto HV, Resende RFB, et al. In vivo evaluation of bovine Xenograft associated with oxygen therapy in alveolar bone repair. J Oral Implantol. (2021) 47(6):465–71. doi: 10.1563/aaid-joi-D-20-00110
2. Atieh MA, Alsabeeha NH, Payne AG, Duncan W, Faggion CM, Esposito M. Interventions for replacing missing teeth: alveolar ridge preservation techniques for dental implant site development. Cochrane Database Syst Rev. (2015) 5:10176. doi: 10.1002/14651858.CD010176.pub2
3. Majzoub J, Ravida A, Starch-Jensen T, Tattan M, Suárez-López Del Amo F. The influence of different grafting materials on alveolar ridge preservation: a systematic review. J Oral Maxillofac Res. (2019) 10(3):e6. doi: 10.5037/jomr.2019.10306
4. Smeets R, Matthies L, Windisch P, Gosau M, Jung R, Brodala N, et al. Horizontal augmentation techniques in the mandible: a systematic review. Int J Implant Dent. (2022) 8(1):23. doi: 10.1186/s40729-022-00421-7
5. Seyssens L, Eeckhout C, Cosyn J. Immediate implant placement with or without socket grafting: a systematic review and meta-analysis. Clin Implant Dent Relat Res. (2022) 24(3):339–51. doi: 10.1111/cid.13079
6. Kumar J, Jain V, Kishore S, Pal H. Journey of bone graft materials in periodontal therapy: a chronological review. J Dent Allied Sci. (2016) 5(1):30. doi: 10.4103/2277-4696.185195
7. Noelken R, Al-Nawas B. Bone regeneration as treatment of peri-implant disease: a narrative review. Clin Implant Dent Relat Res. (2023) 25(4):696–709. doi: 10.1111/cid.13209
8. Balaji VR, Manikandan D, Ramsundar A. Bone grafts in periodontics. Matrix Sci Med. (2020) 4(3):57–63. doi: 10.4103/MTSM.MTSM_2_19
9. Cornelius Timothius CJ, Kilic HN, Gandi KK, Kakar A, John V. Particulate bone graft materials for periodontal and implant surgery: a narrative review and case series. Dent Rev. (2023) 3(2):100068. doi: 10.1016/j.dentre.2023.100068
10. Mellonig JT. Autogenous and allogeneic bone grafts in periodontal therapy. Crit Rev Oral Biol Med. (1992) 3(4):333–52. doi: 10.1177/10454411920030040201
11. Turner DW, Mellonig JT. Antigenicity of freeze-dried bone allograft in periodontal osseous defects. J Periodontal Res. (1981) 16(1):89–99. doi: 10.1111/j.1600-0765.1981.tb00952.x
12. Kim Y, Nowzari H, Rich SK. Risk of prion disease transmission through bovine-derived bone substitutes: a systematic review. Clin Implant Dent Relat Res. (2013) 15(5):645–53. doi: 10.1111/j.1708-8208.2011.00407.x
13. Hollinger JO, Brekke J, Gruskin E, Lee D. Role of bone substitutes. Clin Orthop Relat Res. (1996) 324:55–65. doi: 10.1097/00003086-199603000-00008
14. Nandi SK, Roy S, Mukherjee P, Kundu B, De DK, Basu D. Orthopaedic applications of bone graft & graft substitutes: a review. Indian J Med Res. (2010) 132:15–30.20693585
15. Ishikawa K. Carbonate apatite bone replacement: learn from the bone. J Ceram Soc Jpn. (2019) 127(9):595–601. doi: 10.2109/jcersj2.19042
16. Fujioka-Kobayashi M, Miyamoto Y, Ishikawa K, Satomi T, Schaller B. Osteoclast behaviors on the surface of deproteinized bovine bone mineral and carbonate apatite substitutes in vitro. J Biomed Mater Res A. (2022) 110(8):1524–32. doi: 10.1002/jbm.a.37392
17. Zhang X, Atsuta I, Narimatsu I, Ueda N, Takahashi R, Egashira Y, et al. Replacement process of carbonate apatite by alveolar bone in a rat extraction socket. Materials (Basel, Switzerland). (2021) 14(16):4457. doi: 10.3390/ma14164457
18. Nagai H, Kobayashi-Fujioka M, Fujisawa K, Ohe G, Takamaru N, Hara K, et al. Effects of low crystalline carbonate apatite on proliferation and osteoblastic differentiation of human bone marrow cells. J Mater Sci: Mater Med. (2015) 26(2):99. doi: 10.1007/s10856-015-5431-5
19. Nakagawa T, Kudoh K, Fukuda N, Kasugai S, Tachikawa N, Koyano K, et al. Application of low-crystalline carbonate apatite granules in 2-stage sinus floor augmentation: a prospective clinical trial and histomorphometric evaluation. J Periodontal Implant Sci. (2019) 49(6):382–96. doi: 10.5051/jpis.2019.49.6.382
20. Kudoh K, Fukuda N, Kasugai S, Tachikawa N, Koyano K, Matsushita Y, et al. Maxillary sinus floor augmentation using low-crystalline carbonate apatite granules with simultaneous implant installation: first-in-human clinical trial. J Oral Maxillofac Surg. (2019) 77(5):985.e1–e11. doi: 10.1016/j.joms.2018.11.026
21. Ayukawa Y, Suzuki Y, Tsuru K, Koyano K, Ishikawa K. Histological comparison in rats between carbonate apatite fabricated from gypsum and sintered hydroxyapatite on bone remodeling. BioMed Res Int. (2015) 2015:579541. doi: 10.1155/2015/579541
22. Rakhmatia YD, Ayukawa Y, Furuhashi A, Koyano K. Carbonate apatite containing statin enhances bone formation in healing incisal extraction sockets in rats. Materials (Basel, Switzerland). (2018) 11(7):1201. doi: 10.3390/ma11071201
23. Egashira Y, Atsuta I, Narimatsu I, Zhang X, Takahashi R, Koyano K, et al. Effect of carbonate apatite as a bone substitute on oral mucosal healing in a rat extraction socket: in vitro and in vivo analyses using carbonate apatite. Int J Implant Dent. (2022) 8(1):11. doi: 10.1186/s40729-022-00408-4
24. Takahashi R, Atsuta I, Narimatsu I, Yamaza T, Zhang X, Egashira Y, et al. Evaluation of carbonate apatite as a bone substitute in rat extraction sockets from the perspective of mesenchymal stem cells. Dent Mater J. (2023) 42(2):282–90. doi: 10.4012/dmj.2022-040
25. Fujisawa K, Akita K, Fukuda N, Kamada K, Kudoh T, Ohe G, et al. Compositional and histological comparison of carbonate apatite fabricated by dissolution-precipitation reaction and bio-Oss®. J Mater Sci: Mater Med. (2018) 29(8):121. doi: 10.1007/s10856-018-6129-2
26. Hayashi K, Kishida R, Tsuchiya A, Ishikawa K. Honeycomb blocks composed of carbonate apatite, β-tricalcium phosphate, and hydroxyapatite for bone regeneration: effects of composition on biological responses. Mater Today Bio. (2019) 4:100031. doi: 10.1016/j.mtbio.2019.100031
27. Ishikawa K, Munar ML, Tsuru K, Miyamoto Y. Fabrication of carbonate apatite honeycomb and its tissue response. J Biomed Mater Res A. (2019) 107(5):1014–20. doi: 10.1002/jbm.a.36640
28. Ishikawa K, Miyamoto Y, Tsuchiya A, Hayashi K, Tsuru K, Ohe G. Physical and histological comparison of hydroxyapatite, carbonate apatite, and β-tricalcium phosphate bone substitutes. Materials (Basel, Switzerland). (2018) 11(10):1993. doi: 10.3390/ma11101993
29. Sato N, Handa K, Venkataiah VS, Hasegawa T, Njuguna MM, Yahata Y, et al. Comparison of the vertical bone defect healing abilities of carbonate apatite, β-tricalcium phosphate, hydroxyapatite and bovine-derived heterogeneous bone. Dent Mater J. (2020) 39(2):309–18. doi: 10.4012/dmj.2019-084
30. Peng W, Kim IK, Cho HY, Pae SP, Jung BS, Cho HW, et al. Assessment of the autogenous bone graft for sinus elevation. J Korean Assoc Oral Maxillofac Surg. (2013) 39(6):274–82. doi: 10.5125/jkaoms.2013.39.6.274
31. Zerbo IR, Zijderveld SA, de Boer A, Bronckers AL, de Lange G, ten Bruggenkate CM, et al. Histomorphometry of human sinus floor augmentation using a porous beta-tricalcium phosphate: a prospective study. Clin Oral Implants Res. (2004) 15(6):724–32. doi: 10.1111/j.1600-0501.2004.01055.x
32. John HD, Wenz B. Histomorphometric analysis of natural bone mineral for maxillary sinus augmentation. Int J Oral Maxillofac Implants. (2004) 19(2):199–207.15101590
33. Sartori S, Silvestri M, Forni F, Icaro Cornaglia A, Tesei P, Cattaneo V. Ten-year follow-up in a maxillary sinus augmentation using anorganic bovine bone (bio-Oss). A case report with histomorphometric evaluation. Clin Oral Implants Res. (2003) 14(3):369–72. doi: 10.1034/j.1600-0501.2003.140316.x
34. Tadjoedin ES, de Lange GL, Bronckers AL, Lyaruu DM, Burger EH. Deproteinized cancellous bovine bone (bio-Oss) as bone substitute for sinus floor elevation. A retrospective, histomorphometrical study of five cases. J Clin Periodontol. (2003) 30(3):261–70. doi: 10.1034/j.1600-051x.2003.01099.x
35. Deppe H, Mücke T, Wagenpfeil S, Hölzle F. Sinus augmentation with intra- vs extraorally harvested bone grafts for the provision of dental implants: clinical long-term results. Quintessence Int (Berl). (2012) 36(6):469–81.
36. Hieu PD, Chung JH, Yim SB, Hong KS. A radiographical study on the changes in height of grafting materials after sinus lift: a comparison between two types of xenogenic materials. J Periodontal Implant Sci. (2010) 40(1):25–32. doi: 10.5051/jpis.2010.40.1.25
37. Ogino Y, Ayukawa Y, Tachikawa N, Shimogishi M, Miyamoto Y, Kudoh K, et al. Staged sinus floor elevation using novel low-crystalline carbonate apatite granules: prospective results after 3-year functional loading. Materials (Basel, Switzerland). (2021) 14(19):5760. doi: 10.3390/ma14195760
38. Lee DZ, Chen ST, Darby IB. Maxillary sinus floor elevation and grafting with deproteinized bovine bone mineral: a clinical and histomorphometric study. Clin Oral Implants Res. (2012) 23(8):918–24. doi: 10.1111/j.1600-0501.2011.02239.x
39. Nagata K, Fuchigami K, Kitami R, Okuhama Y, Wakamori K, Sumitomo H, et al. Comparison of the performances of low-crystalline carbonate apatite and bio-Oss in sinus augmentation using three-dimensional image analysis. Int J Implant Dent. (2021) 7(1):24. doi: 10.1186/s40729-021-00303-4
40. Kitamura M, Yamashita M, Miki K, Ikegami K, Takedachi M, Kashiwagi Y, et al. An exploratory clinical trial to evaluate the safety and efficacy of combination therapy of REGROTH® and cytrans® granules for severe periodontitis with intrabony defects. Regen Ther. (2022) 21:104–13. doi: 10.1016/j.reth.2022.06.001
41. Fukuba S, Okada M, Iwata T. Clinical outcomes of periodontal regenerative therapy with carbonate apatite granules for treatments of intrabony defects, class II and class III furcation involvements: a 9-month prospective pilot clinical study. Regen Ther. (2023) 24:343–50. doi: 10.1016/j.reth.2023.08.002.1
42. Funato A, Katayama A, Moroi H. Novel synthetic carbonate apatite as bone substitute in implant treatments: case reports. Int J Periodontics Restorative Dent. (2024) 44(3):257–66. doi: 10.11607/prd.6535
44. GC received the Selection Committee Special Award of the 1 Japan Open Innovation Prize! - GC Global (gc-dental.com) (2019). (accessed March 10, 2024).
Keywords: alloplast, hard-tissue graft, carbonate apatite granules, cytrans granules, artificial bone
Citation: Nunn ME, Rudick C, Nikaido M and Miyamoto T (2024) A systematic review of a novel alloplast carbonate apatite granules. Front. Dent. Med 5:1418039. doi: 10.3389/fdmed.2024.1418039
Received: 15 April 2024; Accepted: 18 July 2024;
Published: 16 August 2024.
Edited by:
Carlos M. Ardila, University of Antioquia, ColombiaReviewed by:
Tania Cestari, University of São Paulo, BrazilAntoine Nicolas Berberi, Lebanese University, Lebanon
Copyright: © 2024 Nunn, Rudick, Nikaido and Miyamoto. This is an open-access article distributed under the terms of the Creative Commons Attribution License (CC BY). The use, distribution or reproduction in other forums is permitted, provided the original author(s) and the copyright owner(s) are credited and that the original publication in this journal is cited, in accordance with accepted academic practice. No use, distribution or reproduction is permitted which does not comply with these terms.
*Correspondence: Martha E. Nunn, bWVudW5uQGdtYWlsLmNvbQ==