- 1Department of Quantum Science and Technology, Research School of Physics, Australian National University, Canberra, ACT, Australia
- 2Oral Health Centre, The University of Queensland School of Dentistry, Brisbane, QLD Australia
- 3Dentroid (Emudent Technologies Pty Ltd), Canberra, ACT, Australia
- 4Faculty of Medicine and Health, The University of Sydney School of Dentistry, Sydney, NSW, Australia
- 5School of Engineering and Built Environment, Griffith University, Nathan, QLD, Australia
By leveraging ultrashort pulse laser and micro-electromechanical systems (MEMS) technologies, we are developing a miniaturized intraoral dental robotic device that clamps onto teeth, is remotely controlled, and is equipped with a focusing and scanning system to perform efficient, fast, and ultraprecise laser treatments of teeth and dental restorative materials. The device will be supported by a real-time monitoring system for visualization and diagnostic analysis with appropriate digital controls. It will liberate dentists from repetitive manual operations, physical strain and proximity to the patient's oropharyngeal area that potentially contains infectious agents. The technology will provide patients with high-accuracy, minimally invasive and pain-free treatment. Unlike conventional lasers, femtosecond lasers can ablate all materials without generating heat, thus negating the need for water irrigation, allowing for a clear field of view, and lowering cross-infection hazards. Additionally, dentists can check, analyze, and perform precise cutting of tooth structure with automatic correction, reducing human error. Performing early-stage diagnosis and intervention remotely will be possible through units installed at schools, rural health centers and aged care facilities. Not only can the combination of femtosecond lasers, robotics and MEMS provide practical solutions to dentistry's enduring issues by allowing more precise, efficient, and predictable treatment, but it will also lead to improving the overall access to oral healthcare for communities at large.
Introduction
Dentistry today is subject to an accelerating demand driven by people's health, oral diseases, aesthetics, and increased life expectancy. Untreated dental caries, severe periodontitis and tooth loss are among the ten most prevalent conditions, affecting more than 3.5 billion people worldwide in 2017. Globally, dental diseases accounted for US$356.80 billion in direct costs and US$187.61 billion in indirect costs in 2015 (1). While automation and robotic assistance are widely adopted in medicine, their application in dentistry remains limited. Hence, dentists still rely on their eyesight and dexterity to perform complex restorative and surgical procedures that require submillimeter precision.
In this paper, we discuss the development of an intraoral robotic device, which will liberate dentists from repetitive manual operations, the need for proximity to the microbe-laden oropharyngeal area and provide patients with minimally invasive pain-free treatment. The essential step forward is to introduce ultrafast, non-thermal regime of ablation to dentistry, which is offered by powerful ultrashort femtosecond lasers. Ultrafast femtosecond (1 fs = 10−15 s) laser micromachining is a versatile tool successfully applied in precise industrial micromachining of metals, ceramics, polymers, and glass, as well as in biological material for medical use in eye surgery (2, 3). The major difference offered by short pulse duration when compared to the conventional laser ablation with longer, nanosecond and microsecond laser pulses, is that optical energy is delivered on a timescale shorter than the rate of thermal diffusion into the surrounding area of the laser beam focus. As a result, ultrashort fs-lasers provide a unique technological opportunity to precisely and efficiently micromachine materials with minimal thermal collateral damage due to the significantly reduced heat transfer into the bulk and surrounding area. The results of our research demonstrate how femtosecond laser enabled robotics can revolutionize dentistry in a comparable way to the da Vinci surgical robot (4, 5). Thus, solving long-standing challenges in effectively treating patients with dental phobia and or residents in remote settings.
Challenges in dentistry
Patients
Anxiety and fear
For millions of people dental anxiety is a health-altering problem as delayed checkups and treatment change the trajectory of diseased teeth (6). The high-speed dental handpiece (“drill”) is often used with a diamond-coated bur (“bit”), which rotates at up to 400,000 revolutions per minute near the patient's lips, tongue, and throat (7). This creates a distinct noise that often triggers anxiety and a source of potential harm to the patient (8). Anxiety is a significant barrier to seeking necessary restorative dental treatment for conditions such as decayed, chipped, or broken teeth. Typically, up to 30% of adults actively avoid dental treatment because of anxiety (8).
Stressors
Dentists are seeing an increase in stress-related conditions among patients. These include teeth grinding, cracked and chipped teeth, and symptoms of temporomandibular joint dysfunction such as jaw and muscle pain (9, 10). A major challenge that has come to prominence from the COVID-19 pandemic is that routine restorative dental procedures generate aerosols, increasing the risk of viral transmission (11). As precautions, many clinics have limited the nature or procedures they offer, or have added space and time between appointments, reducing the number of patients they can see.
Barriers to care
Where dentistry is funded privately as an out-of-pocket personal expense, affordability is an issue. For example, in Australia, around 35 percent of the population delays or misses out on dental care each year because of cost (12, 13). Individual Australians were directly responsible for approximately 58 percent of all spending on dental care in Australia in 2016–2017, dwarfing government and private health insurance contributions. Many people without insurance avoided attending the dentist altogether. Approximately 27 percent of the population, who are most disadvantaged, delayed or did not see a dentist when they should have due to the cost.
Accessibility
Across the world, poorer oral health has been observed in people living in regional and remote areas compared to those in cities, and oral health status generally declines as remoteness increases (13). Rural populations have access to fewer dental practitioners than their city counterparts, which, when coupled with longer travel times and limited transport options to services, affects the range of oral health care that they can receive (14).
Dentists
Fatigue and ergonomics
Many oral health professionals, including dentists, dental hygienists, dental therapists, perform repetitive movements with their hands and wrists, grasping the same instruments, in a predominately static and awkward posture for most of their work time often resulting in musculoskeletal disorders. According to a systematic review by Hayes et al. (15), this results in a very high prevalence of occupational musculoskeletal disorders and pain.
Human error
Dentists aspire to provide high-quality and safe oral health care to their patients. Each year in the US alone, over 195,000 active dental practitioners provide care to more than 127 million patients, expending more than $117 billion annually (16, 17). While doing so, they routinely perform highly exacting and delicate procedures using many devices and tools in complex environments. To add to the complexity, they often perform these procedures using indirect mirror vision, in areas of the mouth where direct lighting is not possible. In response to these challenges, intense concentration is needed for sustained periods, with high burden on visual acuity and sensory processing. Despite best efforts, treatment can fall short of the goal of delivering precise procedures without error. Research has shown that adverse events (AE) and patient harm are commonplace in dentistry (18–20). Researchers have developed a data repository to organize dental AEs into a searchable database systematically together with novel dental AE type and severity classification systems (21).
Lack of tactile feedback
In comparison to the conventional drill where there is tactile feedback of applied forces through the handpiece, dentists using a laser handpiece in a non-contact procedure on teeth lack similar feedback. Consequently, they do not feel sufficiently in control when they are handling a laser beam for cutting teeth (ablation) (7).
Treatments
Limited precision
The hands of a dentist cannot hold any instrument, including a laser handpiece, perfectly still, due to normal microtremors (7). Further, the problem of movement is compounded as patients move as they breathe. Their jaw position can also change slowly or quickly, as they try to maintain a constant open jaw position. Other factors that affect precision include visual fatigue of the dentist due to intense and sustained concentration, and limited eye lens accommodation to near vision with advancing age (22).
Based on a limited understanding of the complexity of dental diseases, oral and dental care has been managing conditions like dental caries or periodontitis similarly for all patients and disease stages for centuries in a similar manner (23, 24). Building on a deeper understanding of oral conditions, their pathogenesis and trajectories, and, most importantly, the acknowledgment of the broader social, behavioral, and systemic determinants of oral health and their link with general health (25).
Limited access
Reduced mouth opening is a common clinical problem that poses an ergonomic challenge to instrumenting inside the mouth. This condition worsens when temporomandibular joint (TMJ) problems develop. Many individuals experience it at least once in their life. Limited mouth opening makes reaching posterior regions difficult or impossible. Restricted mouth opening can occur due to a variety of underlying conditions which may involve complex medical and psychological factors (26). Trismus is defined as an inability to open the mouth due to masticator muscle spasm or fibrosis (27).
Surface consistency
The undulating and complex morphology of teeth adds a further level of challenge. When treating caries or fractured dental fillings, the dentist must aim to achieve preparations of the teeth that are smooth and regular at the macroscopic scale, so that the margin of the filling material visually blends in with adjacent natural tooth structure. At the same time, it is necessary to achieve a surface that has particular microscopic features that enhance the bonding and adhesion with dental filling materials. When a drill is used, organic material from the tooth is smeared microscopically over the prepared tooth surface. This smear layer is poorly attached and is structurally weak, and hence a range of protocols must be followed to alter or remove it entirely. As well as forming smear layer, regular drills cause microscopic cracking of the tooth structure, as well as heat-induced stresses to the dental pulp.
Limited visibility
Adding to the above-mentioned factors that hinder precision is the limited visibility created by the splatter of coolant water sprays onto face shields, visors, and protective glasses. Conventional dental drills and hard tissue lasers all use a coolant water spray, to prevent excessive heating of the dental pulp when a tooth is being prepared. These devices generate a large amount of splashed particles that may contain water, saliva, blood and other patient materials (28). As these fluid particles splash across the working area, they build up on the protective eyewear worn by the dentist, and progressively reduce the quality of what they can see. Face shields are now used commonly to protect the face and eyes of dental staff from these splashed fluids (29).
The next technological step for dentistry
Ultrashort vs. conventional laser dentistry
In the dental clinic, hard tissue removal involves two typical methods: grinding using air turbine or electric micromotor-driven drills, and ablation by microsecond pulsed erbium lasers. These two methods generate mechanical and thermal stresses, and can create micro-cracks extending several tens of microns into the enamel. These are potential initiation sites for new carious attacks which must be avoided.
While erbium lasers reduce thermal effects, they cannot cut metals and ceramic fillings, and are lower in speed and cutting quality than mechanical drills (7, 30, 31). Microsecond pulsed erbium lasers have been used for the preparation of teeth for restorative procedures since the 1990s. They rely on a photomechanical process, whereby the absorption of laser energy in water and hydroxyl groups in the material creates explosions of steam which then detach particles from the tooth (30). The explosion is accompanied by shock waves that can create microscopic cracks while propagating through the tooth structure at the edges of individual craters. All erbium lasers require a water mist spray to operate effectively, and to prevent burning, carbonization, and thermal stress to the dental pulp (30, 31). On one hand, excessively high rates of water flow interfere with the laser ablation action by attenuating the beam, while on the other hand, an insufficient film of water will lead to burning, carbonization, cracking, and building in the tooth structure.
Recently, femtosecond (10−15 s) lasers have been used widely in medical areas such as vision correction surgery (LASIK), cataract surgery, ear surgery, and tattoo removal. Femtosecond pulses cause non-linear interactions with materials, breaking molecular bonds with minimal heat load in a process called “cold ablation”. For restorative dentistry they can overcome the drawbacks of traditional treatment methods (32, 33), and provide a solution to the challenge of attaining an “universal laser cutter” by allowing high precision cutting of metal, ceramic, and polymer dental materials as well as natural tooth structure. Femtosecond laser dentistry was demonstrated 20 years ago (34, 35) with prepared crack-free cavities in teeth and processing precision better than 10 µm, which is ideal for fillings, and far more precise than ablation by erbium or other lasers. Despite the clear advantages of femtosecond laser pulses for ablating teeth, at that time, the low speed together with the limited capability and prohibitively high cost of available lasers made it challenging to compete with mechanical drills.
Development of ultrashort pulse laser dentistry
While there has been a growing number of studies investigating the effects of femtosecond ablation on dental hard tissue, these studies were largely limited by the availability and parameters (such as power) of the then existing femtosecond laser devices which could not have been easily sourced, modified, or optimized for dental application. A very recent systemic review (36) that extensively assessed literature published in English during the last 25 years on the effectiveness and impact of in vitro femtosecond laser irradiation on enamel and dentine was only able to identify 11 studies that met criteria. Interestingly, only 3 out of the 11 studies were considered of “high quality” by the authors who accordingly concluded that “The limited number of high-quality studies and the heterogeneity between the protocols used and the variations in the formatting of the reported data prevented a meta-analysis”. Limitations aside, previous studies indicated, overall, that femtosecond laser ablation could be a viable alternative to both high-speed rotary drills and conventional lasers with the added advantages of unprecedented precision, lack of collateral thermal damage and no requirement for water irrigation and cooling (33–35, 37–39)
With the recent emergence of powerful femtosecond lasers generating high peak energy and high repetition rate, the new generation of ultrashort pulse (USP) lasers can now outperform conventional dental high-speed drills. Such an approach can be a major paradigm shift, as it opens up new perspectives for precise and conservative dental treatment, instead of focusing on merely increasing the speed of rotating drills as has been the case during the last decades.
While USP lasers are used in some medical procedures [such as in vision correction surgery to cut and flop lamellae from the cornea surface and re-shaping cornea (40)] and may be used in dental implant manufacturing stages (41), USP lasers are not used currently in the clinical setting in dentistry for tooth cutting procedures. The current state-of-the-art for tooth cutting is to employ middle infrared lasers such as the Er:YAG and Er,Cr:YSGG lasers operating at wavelengths of 2940 nm and 2780 nm respectively (7, 8). These lasers are used with pulse durations from 50–400 μsec, in combination with a water mist spray for cooling. Such pulse durations are between one million and one billion times longer than those in the picosecond and femtosecond range used by USP lasers (40). Their effect on dental hard tissues is thermo-mechanical in nature, and they have limited ability to ablate metals and ceramics (42).
Using USP mitigates problems caused by thermo-mechanical actions. The instantaneous peak power of the pulses is very high, which means that it is not necessary to use a laser wavelength strongly absorbed in either water or the mineral component of tooth structure, i.e., hydroxyapatite. Femtosecond lasers have demonstrated their capabilities to cut through any type of material, for example, all types of dental restorations and ceramics (7). The ability to control the ablation process much more precisely by altering the pulse energy and the scanning pattern allows the tooth to be machined to give a smooth surface without the accumulation of heat within the tooth itself. The prepared surfaces are very smooth because only a very thin layer of material is removed with each pulse, but up to a million pulses per second can be delivered, without requiring use of any irrigation or cooling system. Accurate control of the extent to which pulses overlap requires going beyond hand-held handpieces. A growing number of studies have demonstrated that femtosecond laser ablation represents the first viable alternative to high-speed dental drills operated by air turbines or by electric micromotors (37). Finally, the femtosecond laser “drill” is much quieter and causes little to no direct pain during its operation (30).
The authors have undergone a series of experiments using a state-of-the-art femtosecond laser machine capable of delivering a range of parameters suitable for dental ablation. The device is a Carbide 40 W femtosecond laser (CB3-40 W from Light Conversion, Lithuania). The laser wavelength was 1029 nm (fundamental), and the pulse duration was set to 275 fs. The repetition rate was 100 kHz and the pulse energy 400 µJ. Our results demonstrated the benefits of femtosecond laser dentistry with high ablation rate and efficiency for drilling enamel and dentin without requiring any cooling system (43). Our observations of the laser processed areas by optical microscopy, scanning electron microscopy and Raman spectroscopy confirmed crack-free cavities in teeth, no change of the chemical composition and a processing precision better than 10 µm. This radically exceeds the precision that handheld high-speed drills can achieve and is significantly more precise than ablation by erbium or other middle / far infrared lasers. This ultra-precision provides a high degree of control of the materials and the quantity removed allowing to preserve as much precious tooth structure as possible following principles of Minimal Intervention Dentistry.
Miniaturization: a device fit for the mouth
Intraoral miniature robotic device
Developing a miniature robotic device for dentistry enables hands-free manipulation of a femtosecond laser beam inside the mouth to painlessly modify and remove tooth structure, decayed carious material and dental filling materials, and to shape and prepare a tooth for subsequent restoration. The intraoral device, by this aspect, will have specific space constraints to fit inside the mouth of most patients without inducing any discomfort. It is required to be as small as possible, without exceeding 25 × 25 mm2 in extent laterally and 15 mm in height, based on studies and the size of existing dental handpieces. Figure 1 presents a conceptual representation of the intraoral robotic device with a width of 22 mm, a depth of 20 mm and a maximum height of 15 mm.
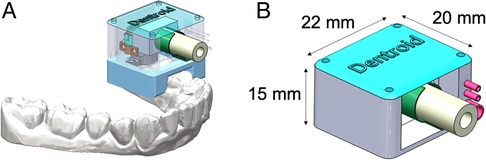
Figure 1. (A) Conceptual representation of the intraoral device on teeth including a MEMS mirror, a fiber optic connector for laser delivery, camera, diagnostics system, and external packaging, and (B) a schematic view with dimensions.
Next generation scanning system
Due to its compact size, the intraoral robotic device needs to take advantage of a miniaturized beam deflection device. A Micro Electro-Mechanical Systems (MEMS) mirror is a tiny integrated system that combines mechanical and electrical components. MEMS has been identified as one of the most promising technologies for the 21st Century, and it has the potential to revolutionize both industrial and consumer products by combining silicon-based microelectronics with micromachining technology. While commercial MEMS mirrors are available, their compatibility with high instantaneous peak and average power lasers are uncertain. Specialized MEMS mirrors with large deflection angles and highly reflective dielectric coatings suitable for high-power laser are required for these types of applications. MEMS mirrors used in laser-surgical instrument for neurosurgery have been successfully tested with a high-power picosecond-laser at 532 nm up to 20 W average laser power, to cut skull tissues (44), and several demonstrations of deflection angles beyond ∼20° have been reported (45). However, none of the existing MEMS mirrors meet all the requirements for the intraoral high-power laser manipulation, including a low driving voltage of less than 10 volts, a high mechanical reflection angle of over ±10°, a high reflectivity of over 99.99%, a high resonant frequency over 1 kHz, a and small size. Therefore, robust MEMS mirrors need to be developed specifically for this device (46, 47). We fabricated successfully micromirrors that satisfied some key parameters, such as small size, and a large deflection angle at low driving voltage (less than 10 volts), as seen in Figure 2. Optimization of these MEMS mirrors will be necessary to meet the remaining technical requirements mentioned above. Another key aspect is the mirror coating. A delicate balance between high reflection, low stress/stress compensation, and very high reflectivity must be achieved. This requires special developments for both the coating technique and the subsequent post processing. For this, we demonstrated coatings with 99.9997% peak reflectivity with high-end Ion Beam Sputtering (IBS) coating equipment, making this well positioned for the required coatings on the miniature MEMS mirrors.
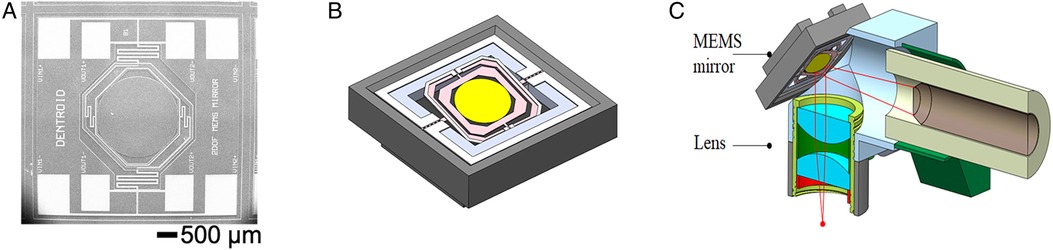
Figure 2. (A) Scanning electron microscopy image of a 2DOF MEMS mirror with (B) its schematic representation, and (C) the intraoral head of the device with MEMS mirror position indication.
Beam delivery and focusing
A key consideration is transmission of ultrashort pulses from the laser system to the tooth without degradation in pulse shape, energy, or beam quality. Articulated arms are a mature technology and are used widely in medical applications. With the recent demonstrations of efficient femtosecond pulse transmission through hollow-core/photonic crystal fibers specifically designed for fs-pulses [e.g., 48], their low weight and excellent flexibility is immensely advantageous compared to articulated arms.
The ideal approach to scanning is to use a so-called telecentric f-theta lens after the beam scanner that provides linear displacement of the focus spot with scan angle. Telecentricity provides low distortion scanning, and normal beam incidence providing magnification/scan scale invariance. Such lenses provide the highest performance laser scanning systems, which cannot be achieved with a spherical lens which can only image along a circular plane, having spot distortion and scan scale variation with processing plane position. These lenses can realize tighter spot sizes, which translate into higher resolution, as well as higher intensity.
Dental applications require ∼8 mm processing depth of focus and a laser spot likely <50 µm diameter due to pulse energy limits and the required fluence. Achieving this depth of focus requires a variable optical focusing system, ideally with a real-time response that can adapt during the tooth ablation. There are three different ways of accomplishing this: moving the scan lens, a variable focus lens (zoom/liquid adaptive lens) before the scanner, or moveable optics before the scan lens. With a variable focus system, focus detection is required to ensure the focus is on the processing plane. We should note here that this problem is made much more difficult by the complex topology and homogenous appearance of the teeth. There are many possible approaches, such as confocal, critical angle prism sensing, and camera-based approaches, to name a few.
Heat considerations
Thermal load into the tooth body during ablation is one of the major concerns of any ablation or cutting process, first, because it is one of the causes of pain of the patient during dental treatment, and second, as an excessive temperature rise can lead to permanent injuries to the dental pulp at the histological level (49). The generally accepted painless limit due to heating human nerves is the increase by 5 °C relative to the temperature of the environment. A temperature rise above 5.5 °C that is sustained for more than 10 s has been considered one such threshold. Above this temperature, soft tissue irritation and inflammation (pulpitis) occur. In approximately 15% of cases this has led to dental pulp necrosis (50). A human in vivo study showed that transient temperature increases of as much as 8.9–14.7 °C could be tolerated without adverse outcomes since they did not cause permanent damage (51, 52). We demonstrated that femtosecond laser ablation of a tooth only led to an increase of 1.3 °C in dental pulp for a thermally isolated tooth (and likely much lower for one in vivo) for a processing time shorter than 1 min (53). The laser treatment was undertaken without using water spray for cooling the tooth. Using compressed air during laser processing will mitigate any thermal changes at the power levels used.
A tooth cutting technology with low thermal load and no significant vibration should have a low “annoyance factor” and be tolerated well by patients. Formal studies of the level of anxiety and pain experienced by patients with this new approach need to be undertaken. One can expect that feelings of pain and discomfort will have an adverse psychological impact on patients and will also impact negatively on their oral health related quality of life (54). On the other hand, it could predict that a procedure which is quiet, comfortable, and free of thermal stress should have a high level of acceptability.
Clamping system - strength, robustness, and durability
The clamping system needs to be attached to teeth of various shapes and sizes, ranging from molar teeth (which are wide in their horizontal buccolingual dimensions) to incisor teeth (much narrower). The clamping system is required to stabilize the device in relation to the biting (occlusal) surfaces of the tooth in question, using an arrangement similar to a dental dam clamp that attaches onto the area below the natural horizontal bulge in the tooth. The outer casing of the intraoral device needs to resist the regular compressive forces applied during jaw closing. An attachment, used as a bite block, needs to stabilize the jaw position, and thus protect the device and the patient attempts to close their jaws during the procedure.
Real-time visualization and diagnostic imaging system
Feedback during the femtosecond laser ablation is essential, and several possibilities exist. A high-resolution color microcamera is needed for precise remote robotic control to give an accurate visualization and targeting of the areas to be processed with the laser. Dentists will have a clear view in real-time of the area to process and constant visual control of the machining of the tooth. It is also vital for the diagnostic system to direct the laser to be carious (decayed) and selected regions accurately. The simplest is a fluorescent diagnostic approach using a 405 nm light. The fluorescence-assisted caries excavation (FACE) method highlights infected decayed tooth structure (in red), allowing for its precise removal, and has been proven with conventional rotary dental drills (53–56). This diagnostic minimizes the problem of overtreatment when decay extends deeply into a tooth, since only the highly fluorescent red-emitting areas will be removed. It also allows clear identification of tooth-colored filling materials, that are otherwise difficult to identify, so these can be removed without the risk of removing healthy tooth structure (56). Fluorescence information could be viewed alongside conventional white light imaging of the target area on the tooth or could be superimposed digitally on the white light image to provide a type of augmented reality for the clinician. Based on what is known regarding augmented reality in complex dental procedures (57), the enhanced information should increase confidence for the clinician that the work they are doing is technically correct.
A different approach to achieving the selective removal of fillings and their various liners and bases that sit beneath the restoration surface is to use laser-induced breakdown spectroscopy (LIBS) or in-situ Raman spectroscopy. This approach leverages the diagnostic value of light given off in bursts when very short-duration laser pulses are applied to the surface and a plasma is generated. The light emissions from that plasma give information regarding the composition of the surface. By sampling the surface using a lower pulse energy, the system can build up a representation of which materials are present, to guide the dentist for their selective removal. These benefits are essential to the overall philosophy of precision dentistry and Minimal Intervention Dentistry principles. Other optical imaging modalities such as optical coherence tomography, and transillumination could also be worth considering as adjuncts to diagnosis, since they will allow identification of tooth-colored restorations and aspects of the internal structure of teeth (56, 58).
Remotely controlled hands-free robotic device
As the robotic device will be inserted inside the mouth of the patient, dentists will be able to control it with a specialized computer and perform treatments from an ergonomically designed desk or workstation. A dental assistant and dentists can install the device will be able to work remotely, from the desk at the side of the patient, from a room next door, or even from another clinic or a remote site. This brings the expertise of dentists to remote locations.
While this paper has emphasized applications for hard tissue restorative procedures, this type of device may also have applications for hard tissue surgery (e.g., preparing bone for the placement of dental implants) or for soft tissue surgery such as gingivoplasty. A key consideration for these procedures will be distinguishing between the various hard and soft tissue components that could be encountered, so that surgery is safe and effective.
Conclusion
This paper presents how to deliver the first intraoral robotic device based on a femtosecond laser and the foundational knowledge for future robotic dentistry. The benefits of femtosecond laser dentistry are heat-free, shock-free material removal with sub-micron precision, combined with the absence of collateral damage to underlying structures, the absence of a smear layer of particle debris, the elimination of water irrigation during the process, the ability to cut through all types of dental restorations and ceramics, and elimination of the need for injections of local anesthetics in 80%–90% of procedures.
Harnessing the synergy of the newly available affordable femtosecond laser systems, the complementary advanced technologies in MEMS, femtosecond pulse compatible optical fibers, and the support from real-time monitoring systems for visualization and diagnostic analysis, intraoral robotic dentistry will liberate dental surgeons from repetitive manual operations. It will allow checking, analyzing, and performing skilled procedures with high precision and automatic correction, lowering human error. The final restoration steps can be built by pairing the device with any commercially available dental milling machine or 3D printer.
Automation lessens workplace physical and mental stresses and thus the physical and mental burnout of dentists and increases their productivity. The treatment can be done in a smaller number of visits, reducing the costs of dental treatments. At the same time, better precision and standardization will lower human errors and consequently medico-legal complications. Early-stage diagnosis and intervention will be performed through units installed at schools, rural health centers and aged care facilities, saving critical time by avoiding waiting lists, and thus facilitating access to care for most of the population. Following the Learning Health System principles, all data gathered will be used for continuous system improvement, to increase our understanding of disease pathogenesis, and improve risk-assessment strategies, diagnostic accuracy, and disease prediction.
Robotic dentistry is crucial for rural and remote communities that suffer from reduced access to oral healthcare. Moreover, intraoral robotic dentistry will offer a platform for mobile and remotely controlled dentistry and has the potential to address the extreme challenge of dental care during space missions.
Intraoral robotic dentistry based on ultrashort pulse lasers will allow the development of the world's first dental device that can automate the workflow of teeth treatments, from diagnosis and planning through performing procedures and building restorations, both locally and as a remote service, that can increase our understanding of disease pathogenesis, diagnosis accuracy and prediction, and that provide patients with minimally invasive pain-free treatment.
Data availability statement
The original contributions presented in the study are included in the article/Suplementary Material, further inquiries can be directed to the corresponding author/s.
Author contributions
LR wrote the manuscript with input from SM, AVR, LJW, HS, QN, VD, PW, DD, OZ, AH, TRH. All authors contributed to the article and approved the submitted version.
Funding
This study received funding from Dentroid (Emudent Technologies Pty Ltd). The funder was not involved in the study design, collection, analysis, interpretation of data, or the decision to submit it for publication. All authors declare no other competing interests.
Acknowledgments
Financial support from the Department of Industry, Innovation and Science (Innovative Manufacturing CRC Ltd - IMCRC/DNT/230921) towards the work on MEMS mirrors is acknowledged. Part of the laser research was financially supported by the Advanced Manufacturing Growth Centre under Project E108 and is hereby acknowledged. ANFF Optofab ACT is also acknowledged for the development of thin film coatings for the MEMS mirrors.
Conflict of interest
Authors OZ, AH, TH and LJW are employed by Dentroid (Emudent Technologies Pty Ltd). This study received funding from Dentroid (Emudent Technologies Pty Ltd). All authors declare no other competing financial interests.
Publisher's note
All claims expressed in this article are solely those of the authors and do not necessarily represent those of their affiliated organizations, or those of the publisher, the editors and the reviewers. Any product that may be evaluated in this article, or claim that may be made by its manufacturer, is not guaranteed or endorsed by the publisher.
References
1. Listl S, Quiñonez C, Vujicic M. Including oral diseases and conditions in universal health coverage. Bull World Health Organ. (2021) 99:407. doi: 10.2471/BLT.21.285530
2. Gattass RR, Mazur E. Femtosecond laser micromachining in transparent materials. Nat Photonics. (2008) 2:219. doi: 10.1038/nphoton.2008.48
3. Sugioka K, Cheng Y. Ultrafast lasers - reliable tools for advanced materials processing. Light Sci Appl. (2014) 3:e149. doi: 10.1038/lsa.2014.30
4. Da Vinci System: https://www.davincisurgery.com/da-vinci-systems/about-da-vinci-systems.
5. Lippross S, Jünemann KP, Osmonov D, Peh S, Alkatout I, Finn J, et al. Robot assisted spinal surgery- a technical report on the use of DaVinci in orthopaedics. J Orthop. (2019) 19:50–3. doi: 10.1016/j.jor.2019.11.045
6. Silveira ER, Cademartori MG, Schuch HS, Armfield JA, Demarco FF. Estimated prevalence of dental fear in adults: a systematic review and meta-analysis. J Dent. (2021) 108:103632. doi: 10.1016/j.jdent.2021.103632
7. Mount GJ, Walsh LJ, Brostek AM. Instruments used in cavity preparation (chapter 8). In: Mount GJ, Hume WR, Ngo HC, Wolff MS, editors. Preservation and restoration of tooth structure, 3rd edn. Chichester UK: John Wiley (2016). p. 117–38.
8. Hmud R, Walsh LJ. Dental anxiety: causes, complications and management approaches. Indent Engl. (2007) 9(5):6–16.
9. Melo G, Duarte J, Pauletto P, Porporatti AL, Stuginski-Barbosa J, Winocur E, et al. Bruxism: an umbrella review of systematic reviews. J Oral Rehabil. (2019) 46(7):666–90. doi: 10.1111/joor.12801
10. Goldstein G, DeSantis L, Goodacre C. Bruxism: best evidence consensus statement. J Prosthodont. (2021) 30(S1):91–101. doi: 10.1111/jopr.13308
11. Amato A, Caggiano M, Amato M, Moccia G, Capunzo M, De Caro F. Infection control in dental practice during the COVID-19 pandemic. Int J Environ Res Public Health. (2020) 17(13):4769. doi: 10.3390/ijerph17134769
12. Teusner DN, Brennan DS, Gnanamanickam ES. Individual dental expenditure by Australian adults. Aust Dent J. (2013) 58(4):498–506. doi: 10.1111/adj.12108
13. Kamil W, Kruger E, Tennant M. Utilisation of dental services of older people in Australia: an economic explanatory model based on cost and geographic location. Geriatrics. (2021) 6(4):102. doi: 10.3390/geriatrics6040102
14. Jean G, Kruger E, Tennant M. The distribution of allied dental practitioners in Australia: socio-economics and rurality as a driver of better health service accessibility. Aust Dent J. (2019) 64(2):153–60. doi: 10.1111/adj.12679
15. Hayes MJ, Cockrell D, Smith DR. A systematic review of musculoskeletal disorders among dental professionals. Int J Dent Hyg. (2009) 7(3):159–65. doi: 10.1111/j.1601-5037.2009.00395.x
16. Munson B, Vujicic M. Supply of dentists in the United States is likely to grow. Health Policy Institute Research Brief. American Dental Association (2014).
17. Franklin A, Gantela S, Shifarraw S, Johnson TR, Robinson DJ, King BR, et al. Dashboard visualizations: supporting real-time throughput decision-making. J Biomed Inform. (2017) 71:211–21. doi: 10.1016/j.jbi.2017.05.024
18. Obadan EM, Ramoni RB, Kalenderian E. Lessons learned from dental patient safety case reports. J Am Dent Assoc (1939). (2015) 146(5):318–326.e312. doi: 10.1016/j.adaj.2015.01.003
19. Hebballi NB, Ramoni R, Kalenderian E, Delattre VF, Stewart DCL, Kent K, et al. The dangers of dental devices as reported in the food and drug administration manufacturer and user facility device experience database. J Am Dent Assoc. (2015) 146(2):102–10. doi: 10.1016/j.adaj.2014.11.015
20. Yansane A, Tokede O, Walji M, Obadan-Udoh E, Riedy C, White J, et al. Burnout, engagement, and dental errors among U.S. Dentists. J Patient Saf. (2021) 17(8):e1050–6. doi: 10.1097/PTS.0000000000000673
21. Maramaldi P, Walji MF, White J, Etolue J, Kahn M, Vaderhobli R, et al. How dental team members describe adverse events. J Am Dent Assoc. (2016) 147(10):803–11. doi: 10.1016/j.adaj.2016.04.015
22. Eichenberger M, Perrin P, Neuhaus KW, Bringolf U, Lussi A. Influence of loupes and age on the near visual acuity of practicing dentists. J Biomed Opt. (2011) 16(3):035003. doi: 10.1117/1.3555190
23. Schwendicke F, Krois J. Precision dentistry—what it is, where it fails (yet), and how to get there. Clin Oral Investig. (2022) 26:3395–403. doi: 10.1007/s00784-022-04420-1
24. Innes NPT, Chu CH, Fontana M, Lo ECM, Thomson WM, Uribe S, et al. A century of change towards prevention and minimal intervention in cariology. J Dent Res. (2019) 98(6 ):611–7. doi: 10.1177/0022034519837252
25. Peres MA, Macpherson LMD, Weyant RJ, Daly B, Venturelli R, Mathur MR, et al. Oral diseases: a global public health challenge. Lancet. (2019) 394(10194):249–60. doi: 10.1016/S0140-6736(19)31146-8
26. Kumar Gupta SK, Rana AS, Gupta D, Jain G, Kalra P. Unusual causes of reduced mouth opening and it's Suitable surgical management: our experience. Natl J Maxillofac Surg. (2010) 1(1):86–90. doi: 10.4103/0975-5950.69150
28. Han P, Li H, Walsh LJ, Ivanovski S. Splatters and aerosols contamination in dental aerosol generating procedures. Appl Sci 2021. (2021) 11(4):1914. doi: 10.3390/app11041914
29. Singh P, Pal K, Chakravraty A, Ikram S. Execution and viable applications of face shield “a safeguard” against viral infections of cross-protection studies: a comprehensive review. J Mol Struct. (2021) 1238:130443. doi: 10.1016/j.molstruc.2021.130443
30. Walsh LJ. The current status of laser applications in dentistry. Aust Dent J. (2003) 48(3):146–55. doi: 10.1111/j.1834-7819.2003.tb00025.x
31. Al-Batayneh OB, Seow WK, Walsh LJ. Assessment of Er: YAG laser for cavity preparation in primary and permanent teeth: a scanning electron microscopic and thermographic study. Pediatr Dent. (2014) 36(3):90–4.24960377
32. Chung SH, Mazur E. Surgical applications of femtosecond lasers. J Biophotonics. (2009) 2:557–72. doi: 10.1002/jbio.200910053
33. Niemz MH. Laser-Tissue interactions: fundamentals and applications. Heidelberg: Springer-Verlag, Berlin (2004).
34. Rode AV, Gamaly EG, Luther-Davies B, Taylor BT, Dawes J, Chan A, et al. Subpicosecond laser ablation of dental enamel. J Appl Phys. (2002) 92:2153. doi: 10.1063/1.1495896
35. Rode AV, Gamaly EG, Luther-Davies B, Taylor BT, Graessel M, Dawes JM, et al. Precision ablation of dental enamel using a subpicosecond pulsed laser. Aust Dent J. (2003) 48:233. doi: 10.1111/j.1834-7819.2003.tb00036.x
36. Lagunov V, Rybachuck M, Itthagarun A, Walsh LJ, George R. Modification of enamel, dentin surfaces by ultra-fast femtosecond laser ablation: a systematic review. Opt Laser Technol. (2022) 155:1084399. doi: 10.1016/j.optlastec.2022.108439
37. Gamaly EG, Rode AV, Luther-Davies B, Tikhonchuk VT. Ablation of solids by femtosecond lasers: ablation mechanism and ablation thresholds for metals and dielectrics. Phys Plasmas. (2002) 9:949–57. doi: 10.1063/1.1447555
38. Chen H, Liu J, Li H, Ge W, Sun Y, Wang Y, et al. Femtosecond laser ablation of dentin and enamel: relationship between laser fluence and ablation efficiency. J Biomed Opt. (2015) 20(2):0208004. doi: 10.1117/1.JBO.20.2.028004
39. Chen H, Li H, Sun YC, Wang Y, Lu PJ. Femtosecond laser for cavity preparation in enamel and dentin: ablation efficiency related factors. Sci Rep. (2016) 6:20950. doi: 10.1038/srep20950
40. Pietilä J, Huhtala A, Mäkinen P, Uusitalo H. Flap characteristics, predictability, and safety of the Ziemer FEMTO LDV femtosecond laser with the disposable suction ring for LASIK. Eye. (2014) 28:66–71. doi: 10.1038/eye.2013.244
41. Ghinassi B, Di Baldassarre A, D'Addazio G, Traini T, Andrisani M, Di Vincenzo G, et al. Gingival response to dental implant: comparison study on the effects of new nanopored laser-treated vs. traditional healing abutments. Int J Mol Sci. (2020) 21(17):6056. doi: 10.3390/ijms21176056
42. Fenelon T, Bakr M, Walsh LJ, George R. Effects of lasers and their delivery characteristics on machined and micro-roughened titanium dental implant surfaces. Bioengineering. (2020) 7(3):93. doi: 10.3390/bioengineering7030093
43. Rapp L, Madden S, Brand J, Walsh LJ, Spallek H, Zuaiter O, et al. Femtosecond laser dentistry for precise and efficient cavity preparation in teeth. Biomed Opt Express. (2022) 13:8. doi: 10.1364/BOE.463756
44. Sandner T, Kimme S, Grasshoff T, Todt U, Graf A, Tulea C, et al. Micro-scanning mirrors for high-power laser applications in laser surgery. Proc. SPIE. 8977, MOEMS and Miniaturized Systems XIII, (2014). p. 897705.
45. Wang D, Watkins C, Xie H. MEMS Mirrors for LiDAR: a review. Micromachines (Basel). (2020) 11:456. doi: 10.3390/mi11050456
46. Amaya S, Dao D, Sugiyama S. Novel fabrication process for a monolithic PMMA torsion mirror and vertical comb actuator. J Micromech Microeng. (2011) 21:065032. doi: 10.1088/0960-1317/21/6/065032
47. Dau V, Bui T, Dinh TX, Dao D, Yamada T, Hata K, et al. A micromirror with CNTs hinge fabricated by the integration of CNTs film into a MEMS actuator. J Micromech Microeng. (2013) 23:075024. doi: 10.1088/0960-1317/23/7/075024
48. Debord B, Alharbi M, Vincetti L, Husakou A, Fourcade-Dutin C, Hoenninger C, et al. Multi-meter fiber-delivery and pulse self-compression of milli-Joule femtosecond laser and fiber-aided laser-micromachining. Opt Express. (2014) 22(9):10735–46. doi: 10.1364/OE.22.010735
49. Baldissara P, Catapano S, Scotti R. Clinical and histological evaluation of thermal injury thresholds in human teeth: a preliminary study. J Oral Rehabil. (1997) 24:791–801. doi: 10.1046/j.1365-2842.1997.00566.x
50. Zach L, Cohen G. Pulp response to externally applied heat. Oral Surg Oral Med Oral Pathol. (1965) 19:515–30. doi: 10.1016/0030-4220(65)90015-0
51. Kwon S-J, Park Y-J, Jun S-H, Ahn J-S, Lee I-B, Cho B-H, et al. Thermal irritation of teeth during dental treatment procedures. Restor Dent Endod. (2013) 38(3):105–12. doi: 10.5395/rde.2013.38.3.105
52. König K, Kasenbacher A. Thermal damage behaviour of human dental pulp stem cells” International Dentistry 7,2 and Laser 4 (2016).
53. Walsh LJ. Caries diagnosis aided by fluorescence. In: Arkanslan Z, editors. Dental caries - diagnosis and management. Croatia: InTech Publishers (2018). p. 97–115. doi:10.5772/intechopen.75459.
54. Lajolo C, Gioco G, Rupe C, Patini R, Rizzo I, Romeo U, et al. Patient perception after oral biopsies: an observational outpatient study. Clin Oral Invest. (2021) 25:5687–97. doi: 10.1007/s00784-021-03870-3
55. Mandikos MN, Walsh LJ. Illuminating dental instrument, coupling and method of use. US patent 9,028,251 (2015).
56. Kiran R, Chapman J, Tennant M, Forrest A, Walsh LJ. Fluorescence-aided selective removal of resin-based composite restorative materials: an in vitro comparative study. J Esthet Restor Dent. (2020) 32(3):310–6. doi: 10.1111/jerd.12536
57. Fahim S, Maqsood A, Das G, Ahmed N, Saquib S, Lal A, et al. Augmented reality and virtual reality in dentistry: highlights from the current research. Appl Sci. (2022) 12:3719. doi: 10.3390/app12083719
Keywords: ultrashort (femtosecond) laser pulses, dentistry, intraoral, micro-electromechanical systems (MEMS), dental treatment, robotic dentistry, laser ablation, ergonomic
Citation: Rapp L, Madden S, Rode AV, Walsh LJ, Spallek H, Nguyen Q, Dau V, Woodfield P, Dao D, Zuaiter O, Habeb A and Hirst TR (2022) Anesthetic-, irrigation- and pain-free dentistry? The case for a femtosecond laser enabled intraoral robotic device. Front. Dent. Med 3:976097. doi: 10.3389/fdmed.2022.976097
Received: 23 June 2022; Accepted: 8 August 2022;
Published: 23 August 2022.
Edited by:
Kunaal Dhingra, All India Institute of Medical Sciences, IndiaReviewed by:
Maria Contaldo, University of Campania L. Vanvitelli, ItalyDinesh Rokaya, Walailak University International College of Dentistry, Thailand
© 2022 Rapp, Madden, Rode, Walsh, Spallek, Nguyen, Dau, Woodfield, Dao, Zuaiter, Habeb and Hirst. This is an open-access article distributed under the terms of the Creative Commons Attribution License (CC BY). The use, distribution or reproduction in other forums is permitted, provided the original author(s) and the copyright owner(s) are credited and that the original publication in this journal is cited, in accordance with accepted academic practice. No use, distribution or reproduction is permitted which does not comply with these terms.
*Correspondence: Ludovic Rapp, bHVkb3ZpYy5yYXBwQGFudS5lZHUuYXU=
Specialty Section: This article was submitted to Systems Integration, a section of the journal Frontiers in Dental Medicine