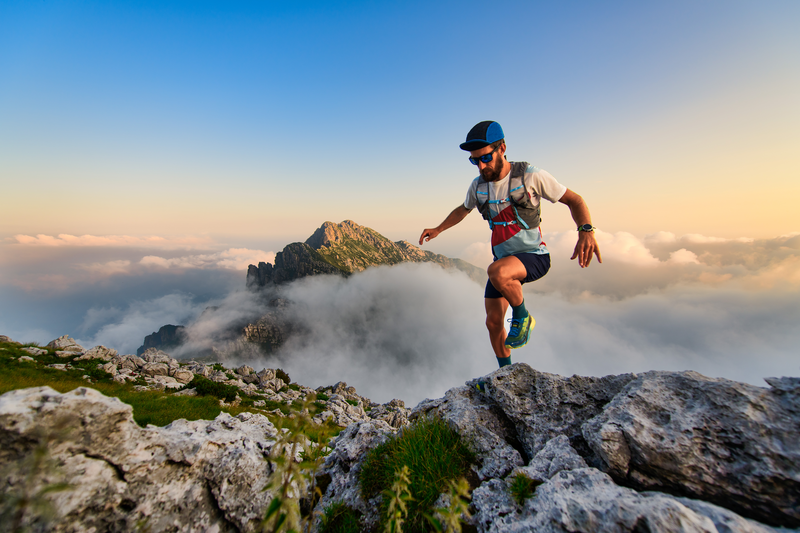
94% of researchers rate our articles as excellent or good
Learn more about the work of our research integrity team to safeguard the quality of each article we publish.
Find out more
REVIEW article
Front. Dent. Med , 21 April 2022
Sec. Dental Materials
Volume 3 - 2022 | https://doi.org/10.3389/fdmed.2022.868651
This article is part of the Research Topic Next-Generation Dental Materials Engineered for Mineralized Tissue Reconstruction: Advances, Challenges and Opportunities View all 5 articles
Resin-based dental materials are popular restorative materials especially in direct adhesive restoration because of the excellent mechanical and esthetic properties. Toward the realization of minimally invasive dental procedures, direct composite resin adhesive restoration has become the main treatment for dental defects. In addition, for caries-affected dentin close to the pulp, conservation remineralization has been advocated to save the living pulp. However, the resin–dentin interface can be destabilized by various factors, especially the enzymatic degradation of collagen fibrils within the hybrid layer and polymer hydrolysis. Furthermore, for resin-based restorative materials, the marginal gap remains a major problem that can lead to the occurrence of secondary caries. To address these issues, research efforts have focused on the remineralization of mineral-depleted dental hard tissues using remineralizing bioactive substances. In this review, we first described various bioactive agents with remineralizing properties. Furthermore, we discussed recent advances in resin-based dental materials for enamel or dentin remineralization. Finally, we examined the current challenges and prospects of these emerging materials. This work aims to provide a theoretical foundation for the future development of resin-based dental materials in direct adhesive restoration with remineralizing agents.
Teeth are highly mineralized organs in the human body. After tooth eruption, a natural remineralization process occurs, in which mineral crystals generated by calcium, phosphorus, fluorine, and other inorganic ions repair or replace the hard tissue of the tooth. Teeth undergo a lifelong process of demineralization and remineralization, which reaches an equilibrium under physiological conditions (1). When demineralization occurs before remineralization, the minerals in the teeth are lost, which is the first step of caries. Thus, accelerating the remineralization process is a promising approach for preventing and treating caries.
The development of resin-based dental materials and the improved understanding of caries and remineralization development have catalyzed the evolution of caries management from the “preventive extension” concept put forward by G.V. Black to a “minimally invasive” approach (2). Caries can stimulate pulpitis and eventually cause pulp infection and necrosis. Traditionally, the complete removal of carious dentin is adopted in deep caries management. Minimally invasive dentistry works by removing the caries-infected dentin while preserving the caries-affected dentin (CAD). Remineralizing fillers in resin-based dental materials including adhesives and composite resins may promote the remineralization of residual CAD to prevent the occurrence of pulp exposure, thus maintaining a healthy dental structure and preserving living pulp (3).
The most critical problem in resin–dentin adhesives is their limited durability. The resin-sparse, water-rich zones in resin-bonded interfaces are thought to degrade over 1–2 years. Degradation is partly due to the hydrolysis of the hydrophilic resin components in such adhesives and the degeneration of collagen fibers by matrix metalloproteinases (MMPs) generated by demineralized dentin (4). Furthermore, these adhesives with low stiffness may result in fatigue failure when exposed to excessive cyclic strain under daily use (5). Biomimetic remineralizing bonding approaches can be applied to solve this problem when the average bonding interface contains 30%−50% residual water, which should be slowly displaced by nano-sized crystals of apatite generated using biomimetic remineralization. Remineralization, seen as a dehydration process, can remove free water to prevent MMPs from being activated (4, 6, 7). In recent years, exogenous sources of mineralizing ions have been introduced into the bonding interface to encourage the remineralization of exposed collagen. In this approach, the newly formed mineral replaces water and bridges the interlayer gap, thus improving the durability of the bonding interface. Despite numerous improvements in multifaceted approaches for caries prevention, caries remain prevalent worldwide. Since the 1960s, when Bowen introduced bisphenol A glycerolate dimethacrylate (BisGMA) (8), methacrylate-based dental resin composites have gradually become the mainstream choice for restoration. However, the longevity of composite restorations is not ideal. Recurrent dental caries along the margins of dental fillings is the most common cause of failed restorations, accounting for 50%−70% of all failures (9).
Dental remineralization for carious tissue has begun to be attention as early as half a century ago.The use of remineralizing agents in resin-based materials could prolong the service life of resin-based composites (RBCs), remineralize CAD, or prevent caries lesions from advancing. However, the development of resin-based materials with remineralizing agents is not straight forward. The initial remineralization method was to provide large amounts of fluoride and calcium and phosphorus substrates around the caries lesions to facilitate the occurring of remineralization (10). Later, fluoride-releasing composite resins (11) and adhesives containing bioactive glass (BG) (12) were used to promote remineralization of the bonding interface. In order to better simulate the structure of natural teeth, some scholars have proposed the concept of biomimetic mineralization. Current development of biomimetic remineralization strategies in in vitro experiments focuses on remineralizing bonding interface (13) and CAD (14). The technical obstacle to overcome in clinical application lies in how to continuously supplement the effective components required for remineralization, and how to maintain the mechanical properties while the components are slowly released. Researchers have proposed using rechargeable nanomaterials (15) for constant ion release and some transportation system such as mesoporous nanomaterials (16), provides a preliminary in vitro basis for the transformation of biomimetic remineralization strategy to clinical application. In this review, we discuss the remineralizing active components that have been added to resin-based dental materials in recent years. Furthermore, we provide an overview of recent approaches for the remineralization of enamel and dentin by resin-based dental materials and the corresponding biological remineralization mechanisms.
The release and deposition of calcium and phosphorus directly impact tooth development (17). The most common calcium phosphate components in dental composites are hydroxyapatite (HA) and amorphous calcium phosphate (ACP). HA is an essential component of enamel, dentin, and cementum tissues in the human body (18). The composition and crystal structure of HA are similar to those of natural apatite found in human dental hard tissues and the human skeletal system (19). The inclusion of HA particles can enhance the properties of RBCs. Furthermore, the remineralizing capabilities of HA particles are enhanced in the presence of calcium and phosphate ions, resulting in stronger bonding at the adhesive–dentin interface and consequently improving the microhardness (20, 21).
ACP, which is the first solid phase formed in highly supersaturated calcium phosphate solutions, can be easily converted into stable crystalline phases. Distinctly, ACP lacks the long-range, periodic, atomic-scale order found in crystalline calcium phosphates (22). Furthermore, in biomineralization processes, ACP is a direct precursor of biological apatite. Owing to this bioactivity, ACP can be used to encourage remineralization via RBCs. In low pH environments, RBCs containing ACP particles release more calcium and phosphate ions (23, 24). In the process, a supersaturated ion reservoir is created that can deposit apatitic minerals on the tooth surface. As ACP lacks a regular crystalline order and is highly soluble, it can quickly form apatite and thus increase the pH of acidic solutions that demineralize the tooth structure (25).
Calcium and fluoride are essential ions that can remineralize the tooth structure (26). Fluoride is the most common prophylactic agent for reducing and preventing enamel demineralization (21). In the presence of fluoride, HA behaves as fluorapatite, a mineral phase more resistant to dissolution. Fluoride can preferentially replace OH− ions in the HA lattice, which promotes remineralization (27). Moreover, fluoride inhibits the metabolic and physiological pathways of microorganisms in cariogenic biofilms that produce organic acids capable of demineralizing dental tissue. Thus, the inclusion of CaF2 nanoparticles imparts materials with antibacterial properties (28) and stimulates fluoride release (29).
BGs are calcium-sodium-phosphosilicate-based materials. The first BG, Bioglass 45S5, was developed by L. Hench in 1969; it was composed of SiO2, CaO, Na2O, and P2O5. Consequently, using varying ratios of the same components, different BGs have been produced. Other components, such as CaF2, K2O, and MgO, have also been used (30). Upon the addition of BG filler particles, ions like calcium and phosphate are released and the pH rises (31), resulting in the remineralization of the adhesive–dentin interface (32). Hydrated silica in BGs can provide nucleation sites for the formation of HA-like layers on the restoration surface, thus preventing bacterial penetration and development of secondary caries (33).
Traditional remineralization is usually performed by adding different concentrations of fluoride and immersing demineralized dentin in a solution containing calcium and phosphate ions. This process involves the growth of the local remaining nuclei in demineralized dentin in the presence of sufficient mineral ions. The composition of the mineralizing solution affects the subsequent remineralization process, including the location and density (34). This traditional remineralization approach can only be applied to the continuous growth of single crystals with a good surface structure, not to the early formation of crystals in the matrix stage (35). Thus, the classical ion-based crystallization concept may only be suitable for remineralizing the basal parts of partially demineralized dentin created by milder self-etching adhesives. In addition, this approach is unlikely to be effective for remineralizing completely demineralized dentin within hybrid layers created with etch-and-rinse adhesives, or the superficial parts of CAD lesions that remain after minimally invasive removal of caries (36, 37).
Enamel, the structure covering the exterior of the anatomical crown, is composed of 96% inorganic materials, 3% water, and 1% organic materials, without cells or vascular tissue. The absence of cells prevents enamel from repairing or replacing itself once damaged. To achieve enamel-like layered structures and chemical properties based on non-classical crystallization mechanisms, materials such as amelogenin, peptides, extracellular matrix analogs, poly(amidoamine) (PAMAM), and chitosan have been investigated using in vivo or in vitro experiments. However, the applied biomimetic pathway does not fully mimic the temporal and spatial synchronous assembly of amelogenin, non-amelogenin, and minerals, which may also hinder the formation of enamel column-like structures in vitro.
The normal dentin matrix is composed of an ordered woven collagen matrix, iteratively arranged inorganic apatite crystals, and a small amount of noncollagenous proteins (NCPs). Minerals account for 70% of the dentin matrix, with the remaining 30% contributed by organic collagen, NCPs, and water (38). Dentin is supported by collagen in the form of protofibrillar scaffolds, in which apatite is embedded in an extrafibrillar and intrafibrillar manner. Researchers have discovered that intrafibrillar mineralization is critical for the mechanical properties of dentin (39). Thus, biomimetic mineralization is an important concept for dentin remineralization.
An improved understanding of dentin and biomimetic remineralization has promoted significant progress in dentin remineralization research. In the natural state, the dental hard tissue mineralization process is regulated by various proteins, such as the enamel matrix protein and dentin phosphoprotein (DPP). These protein-derived peptides can regulate the HA crystal nucleation growth (40). The occurrence of this process may be related to the induction of the structural orientation of the HA crystals after the acidic groups in the polypeptide are combined with calcium ions. The key peptide sequences of these proteins function mainly as a stabilizer functional analog (41) in biomimetic remineralization; and these proteins are adsorbed to the surface of the amorphous mineralization precursor to stabilize it in the nano-amorphous phase and ensure its mobility. NCP analogs such as polyacrylic acid (PAA) (42), phosphoric acid polyethylene (PVPA) (40), casein phosphopeptide amorphous calcium phosphate (CPP-ACP) (43, 44), and synthetic peptides (45) have been applied to promote dentin remineralization through the addition of dental restorative materials. In addition, graphene oxide (46), which can attract calcium ions for HA formation, and metal ions (47) including Zn, Cu, and Ag, which can reduce collagen degradation, have been used to promote the remineralization of the resin–dentin interface and sustain bonding efficacy.
Nevertheless, remineralized and natural dentin have been found to differ significantly in mineralization degree and mineralized particle ordering. In addition, most remineralization experiments have been performed in the liquid phase, which may hinder direct clinical applications. Therefore, subsequent biomimetic mineralization experiments should not be limited to the laboratory and should place more emphasis on clinically relevant conditions.
The theory of remineralization along with knowledge about the clinical application of resin-based dental materials has been applied to design the following agents, with a focus on their effectiveness in remineralizing demineralized dentin, preventing caries, and improving the durability of bonding interfaces.
Secondary caries is the main reason for restoration failure, which are usually caused by demineralization at the restoration margins. To solve this problem, fluoride-releasing components have been incorporated into bioactive dental composites with remineralization capabilities. Various studies have shown that inorganic phosphate can increase the ability of fluoride to promote caries resistance. The greatest effect was observed for sodium trimetaphosphate (TMP), which appeared to act on the lesion area by facilitating the flux of calcium into the deeper enamel layers and reducing acid diffusion inside the enamel (48). Once adsorbed on enamel, TMP likely retains CaF2 and Ca ions by replacing Na in its cyclic structure to enhance remineralization (49). Tiveron et al. (50) found that the addition of 14.1% TMP and 1.6% NaF to a composite resin improved the enamel remineralization ability. The CaF2 nanocomposite showed high F ion release, but due to its opacity, it may not be suitable for anterior dental aesthetic restoration (51, 52).
Calcium phosphate (CaP) composite resins can release calcium and phosphate ions and oversaturate the surrounding medium, resulting in the deposition of HAP crystals on enamel in vitro. However, the mechanical properties of these resins are too weak for restoration. Hence, a silanized filler was incorporated into a nanocomposite containing ACP to achieved improved mechanical properties (53). However, their mechanical properties were still weak as the flexural strength is approximately half of the unfilled resin (53, 54).
More recently, nanoparticles of ACP (NACP) with particle size of approximately 100 nm was synthesized. Compared with traditional CaP, the flexural strength and elastic modulus of the NACP composite were 2-fold higher (55). The composite material exhibited greatly increased ion release under acidic conditions and was able to rapidly neutralize acids. Indeed, the NACP composite was shown to promote tooth remineralization (56). In an in vitro study, the NACP composite performed better than a commercial fluoride-releasing composite and a commercial nanocomposite in enamel and dental remineralization (56, 57). Acidic monomer adding in the composite is believed to impart ion recharge and re-release capabilities and stabilizers such as P2 are commonly added (58, 59). Zhang et al. (59) developed the first generation of rechargeable CaP composites using pyromellitic glycerol dimethacrylate (PMGDM) and bis[2-(methacryloyloxy)ethyl] phosphate as monomers, which showed continuous re-release of Ca and P ions for 42 days without a need for further recharge. Recently, PAMAM and NACP composites were combined to remineralize dental hard tissues, which can induce a layer of dense needle-like mineral crystals on the surface of demineralized dentin with hardness similar to that of a healthy dentin (60). However, the combination can only work well in non-acidic media.
BGs have also been introduced into composite resins as fillers for various purposes (31, 33, 61, 62). However, BGs are hydrophilic and tend to aggregate in the hydrophobic resin matrix, which often leads to severe deterioration of the resin's mechanical properties (63). To avoid this problem, BG fillers were hydrophobically modified or even allowed to form chemical bonds with the resin matrix. However, the BG surface needs direct contact with saliva to induce HA formation, which limits the extent of hydrophobic modification. Using amphiphilic bioactive raspberry-like composite particles (BRPs) embedded in the hydrophobic matrix resin may solve this dilemma (64). Such structure possessed a hydrophobic polymer domain compatible with the resin matrix and a hydrophilic bioactive domain to promote mineral precipitation. Comparing traditional RBCs with BG, BRP-containing RBCs showed greater mechanical properties and a dense mineral layer was formed on the dentin. Current research has mainly focused on the formation of apatite rather than the mechanical properties of dentin treated by BGs. Therefore, it is still unclear whether BGs can remineralize dentin (65).
As restorative materials for caries, RBCs require various properties that will allow them to function for a long time. Adding remineralizing fillers, usually at low dosages, make it possible for RBCs to have new functions. However, several unavoidable problems still need to be tackled. Reinforcing fillers is usually accompanied by low filler loading, which may affect the mechanical properties of RBCs. This can be resolved by using fillers with various sizes and morphologies. The added amounts should be controlled cautiously as the remineralizing fillers are usually detrimental to the mechanical properties. With these, sustainable and stable RBCs with improved biocompatibility and remineralization are expected to evolve.
There is a high incidence of enamel demineralization during orthodontic treatment. Generally, enamel is relatively stable with a dynamic balance between demineralization and remineralization at the dental–pellicle and plaque–saliva interfaces (66). However, due to the lengthy duration of the orthodontic treatment, the occlusion of the appliance, and the accumulation of food residues, enamel is vulnerable to permanent damage caused by cariogenic biofilms (67). This damage can lead to subsurface enamel lesions with partial demineralization, clinically presenting as white spot lesions (WSLs). WSLs can be remineralized through saliva, but when natural remineralization is not sufficient for the enamel to retain a normal morphological structure, surface demineralization gradually progresses to subsurface demineralization (68). It is necessary to accelerate the remineralization process to prevent enamel lesions from developing to cavities. Thus, in recent years, remineralizing adhesives that are easy to use, close to the enamel, and independent of patient cooperation have been developed.
Two common types of conventional adhesives are methacrylate resins and glass ionomer cement (GIC) derivatives. Resin-modified GICs (RMGICs), combining the acid-based chemical reaction of a GIC with the light-activated addition polymerization of hydroxyethyl methacrylate, have considerably developed over the past two decades. Through the use of RMGICs, a certain amount of fluoride ions can be released to promote remineralization. Furthermore, the carboxyl groups in polyoleic acid can form ionic bonds with HA in untreated dental hard tissues to avoid the side effects of acid etching on enamel and effectively prevent the occurrence of caries around brackets (69). To achieve sustained fluoride release, Shah et al. (70) modified an RMGIC with chitosan (CH-RMGIC). The modification did not affect the bonding strength; however, the amount of fluoride released by CH-RMGIC was greater than that of the unmodified RMGIC by 8.47% after 15 days and by 39.68% after 30 days. Furthermore, in reviewed trials, in a treatment period of >14 months, the composite resin may have a higher clinical efficacy than the unmodified RMGIC (71).
Most resin-based adhesives are either enamel-bonding agents or dentin-bonding agents. However, seventh-generation bonding agents have been updated to include one-component, self-etching bonding agents for simultaneous enamel and dentin bonding (72). The use of synthetic-resin-based fluoride adhesives can significantly increase the concentration of absorbed fluorine ions in tooth surface enamel (73), improve the microhardness of enamel near the restoration after demineralization and remineralization treatment, and promote enamel repair (74). Placing fluoride-releasing adhesives near brackets appears to be an effective way to reduce the risk of WSL development in patients wearing fixed orthodontic devices. However, it seems to have no impact on the extent of lesions when WSLs are present (75). To achieve improved remineralizing effects, Fan et al. (76) added NACP to bonding agents. These modified bonding agents were found to be more effective in remineralizing enamel in the S. mutans biofilm model than commercial fluoride bonding agents. To promote enamel remineralization, Gao et al. (77) added a statherin-based dendrimer (SN15)-PAMAM-NACP mixture to bonding agents, as statherin can bond to the enamel surface and thus provide protection from demineralization under acidic conditions.
The direct addition of fluoride or NACP has some drawbacks, in that the initial “sudden release effect” of ions is not easy to control and concentrations vary with time. A new composite incorporating PMGDM and ethoxylated bisphenol A dimethacrylate (EBPADMA) into the resin matrix can chelate inorganic ions in solution. This composite exhibited a strong recharging ability, allowing the continuous release of calcium and phosphate ions over 14 days after a single recharge. Furthermore, PEHB + 30% nanoparticles of CaF2 (nCaF2) was shown to release more F ions than a commercial RMGIC (78).
RMGICs are self-adhesive, fluoride-releasing materials used for fillings and cosmetic restorations as well as for bonding interfaces (79). Surface pre-reacted glass ionomer (S-PRG) fillers can release different ions such as aluminum, silicon, and strontium in addition to fluoride. Advantageously, S-PRG fillers can release and replenish fluoride in acidic environments, thus forming acid-resistant zones, strengthening tooth structure, and imparting antiplaque and antibacterial effects. In addition, S-PRG fillers have a pH-buffering capacity under acidic conditions, which can transform the acidic oral environment into a neutral alkaline environment and promote the remineralization of dental tissues. ThanNaing et al. (80) showed that resin-bonded cementum containing a S-PRG filler reduced mineral loss and promoted dentin remineralization.
Zinc oxide (ZnO) particles have also been added in adhesive systems as zinc ions can protect collagen from degradation. Other metallic oxides besides ZnO have also been proved to induce the precipitation of weakly crystallized apatite (81). The addition of zirconium oxide nanoparticles (4.8 or 9.1 wt.% nano-zirconia groups) to a bonding resin material was found to enhance surface mineral deposition significantly, indicating improved remineralization performance (82). Balbinot et al. (83) incorporated SiNb particles (1 or 2 wt.%) into an adhesive resin and found that the experimental bonding agent containing 2% SiNb promoted mineral deposition and enhanced bonding strength.
Minimally invasive dentistry advocates the prevention and reversal of initial caries (non-cavitated lesions) using contemporary nanotechnology and nanomaterials. However, few materials and bonding approaches have achieved the full remineralization of a demineralized dentin matrix with complete recovery of its original mechanical properties and functionality. More than 10 different NCPs have been found to have a key role in stabilizing NACP to induce remineralization (42, 84, 85), including PAA, PVPA, sodium trimetaphosphate (STMP), and other peptides. In most studies, these analogs were added in simulated body fluid or artificial saliva; however, in a few studies, they were employed as water-based primers. An experiment, incorporating PAA-ACP nanoparticles into a mild self-etch adhesive, successfully induced the extrafibrillar and intrafibrillar remineralization of demineralized enamel and dentin substrate, proving that a self-etching adhesive can act as a carrier for other nanoprecursors to promote remineralization (86).
Some identified peptides specific to the hydroxyapatite mineral can mediate ACP mineralization and promote dental remineralization such as DMP-derived peptides. These relatively shorter peptide sequences that mimic the role of natural proteins may allow for better control. Self-assembled peptide binding at the adhesive-dentin interface could lead to peptide diffusion and limited active conformations due to the challenges associated at the hybrid layer. To address this limitation, Spencer et al. (87) prepared a peptide-functionalized adhesive to allow in situ presentation of the bioactive cues at the adhesive-dentin interface. Antimicrobial peptides can promote remineralization or prevent demineralization by binding calcium to HA. HA affinity increases the substantivity of antimicrobial peptides with HA and hence increases their activity against cariogenic biofilm. Yuca et al. (88) synthetized a co-tethered dual peptide-polymer adhesive system, which incorporates an antimicrobial peptide to inhibit bacterial attack and a hydroxyapatite-binding peptide to promote the remineralization of damaged tooth structure. This demonstrated both strong metabolic inhibition of S. mutans and localized calcium phosphate remineralization.
Abundant in vitro evidence has demonstrated that the use of adhesives containing ion-releasing fillers and analogs can promote remineralization and reduce collagen degradation. However, no current restorative materials can achieve the complete extrafibrillar and intrafibrillar remineralization and the complete recovery of the elastic modulus at the bonding interfaces through biomimetic remineralization. The hydrophilicity of the adhesive and susceptibility to hydrolysis also affect the long-term bonding strength (89). Finally, the different specific roles of remineralized agents as resin formulations have not yet been elucidated. Nevertheless, further research into the identification of their clinical usage and the verification of their long-term effectiveness is necessary.
Pit-and-Fissure sealants are well-established materials and their use in dental treatments is effective. Sealants can serve as physical barriers on the complex morphology of occlusal surfaces, preventing plaque accumulation and bacterial growth. Sealants are minimally invasive and are considered as an effective preventive method for arresting non-cavitated carious lesions (90). However, sealant retention, material longevity, and technical sensitivity affect the clinical success rate (91). Fluoride sealants have the dual effects of sealing and slowly releasing low concentrations of fluoride ions into the dental surface and saliva around the material. These fluorine storage properties provide fluoride sealants an advantage over general fluorine preparations, such as fluoride toothpastes, mouthwashes, and coatings, which can only release fluoride for a short time.
Currently, resin-based sealants are the most common sealing materials. Fourth-generation resin-based sealants can be divided into two categories according to the fluoride binding mode (92). In the first category, soluble fluoride is released by dissolution. However, the sealant itself may be affected by the formation of pores after fluoride dissolution, which can increase the absorption of water and reduce durability. Representative sealants of this type include Fluro Shield (Dentsplay), Delton Plus (Johnson), and Helioseal-F (Vivadent). In the second category, fluorine ions are released from organic fluoride through ion exchange. The representative sealant of this type is Teethmate-F (Kuraray), which shows relatively stable, long-term, low-level fluorine release. In a 1-year clinical trial performed by Mathew et al. (93), the preservation rate of Helioseal-F (Vivadent) was 88%, and no secondary caries occurred. Another study showed that traditional fluoride sealants release very low concentrations of fluoride, which are insufficient for realizing antibacterial properties (94). Fei et al. (95) synthesized nCaF2 with a high surface area via a spray-drying technique. These particles were subsequently combined with dimethylaminohexadecyl methacrylate (DMAHDM) antibacterial agents to achieve high levels of fluoride ion release and improve remineralization. Furthermore, Ibrahim et al. (96) designed a pH-dependent sealant that allowed NACP to release more calcium and phosphate at low pH values. This approach could drive ion diffusion to promote remineralization when the calcium and phosphate levels (subsaturation concentrations) in the enamel are insufficient.
By utilizing new trends in materials science, such as introducing nanostructures and antibacterial properties, pit-and-fissure sealants with novel characteristics can be realized. Although many in vitro results are available for fluoride-releasing sealants, long-term clinical observations are required to confirm their superiority to traditional sealants. Therefore, the application of such sealants in oral clinical practice needs to be advanced through long-term clinical verification to achieve enhanced remineralizing effects.
Root canal sealers are mainly used to fill gaps between the core filling material and the dentinal wall. Most studies have focused on identifying ideal root canal sealers for use in combination with gutta-percha. Antibacterial agents were added to enhance the antimicrobial properties (97). An ideal root canal sealer should also induce the formation of mineralized tissue aside from having antimicrobial properties. Root canal irrigants and chelating solutions such as NaClO and ethylenediaminetetraacetic acid (EDTA) can alter the chemical structure of dentin, resulting in damage, mineral loss, and reduced root dentin hardness, thus leading to tooth fracture (98). Therefore, developing remineralizing root canal sealers that reverse the adverse effects produced by irrigants and chelating solutions is necessary.
Several root canal sealers containing calcium phosphate and calcium silicate have been developed to promote mineralized tissue formation by producing a high pH and inducing the release of calcium and phosphate ions (99). Recently, inorganic fillers such as dicalcium phosphate dihydrate (DCPD), dicalcium phosphate anhydrous (DCPA), tetracalcium phosphate (TTCP), alpha tricalcium phosphate (α-TCP), ACP, and NACP have been tested for their capability to release high levels of calcium and phosphorus and remineralize dental lesions when added in resin matrices. A new therapeutic root canal sealer with three bioactive agents, DMAHDM, Ag nanoparticles (NAg), and NACP, was found to neutralize acid, promote dentin remineralization, and exhibit antibacterial properties. Its highly desirable antibacterial and remineralization properties makes this sealer a good candidate strengthen the structure of dentin in the root and improve the success of endodontic treatment (100).
Over the last few decades, restorative dentistry has become more conservative. Remineralization procedures have proven to be the most effective methods for regrowing lost tooth structures. With the advancement of mineralization theory, numerous new technologies have been developed, and important breakthroughs have been made in the field of dental hard tissue restoration. However, a gap remains between remineralized dentin and natural dentin in terms of the degree of mineralization and particle ordering.
Various technical problems are encountered in the clinical application of dentin bionic remineralization. For example, an adequate supply of the ingredients required for mineralization must be available. Furthermore, approaches must be developed to maintain the mechanical properties of the parent material during the slow release of each component in the mixture. Most studies on remineralization systems have been conducted in the liquid phase. Therefore, it is also necessary to determine how these systems can be incorporated into clinical dental materials. By addressing these issues, we hope that more clinically applicable products will be identified in the future.
QZ, ML, XM, and YZ contributed to conception, data collection, wrote, and critically revised the manuscript. SM contributed to provide helpful comments on a previous draft and guide the manuscript revision. All authors gave an approval to the submitted version.
This work was funded by National Natural Science Foundation of China (81100743) and Guangdong Financial Fund for High-Caliber Hospital Construction (174-2018-XMZC-0001-03-0125/D-15).
The authors declare that the research was conducted in the absence of any commercial or financial relationships that could be construed as a potential conflict of interest.
All claims expressed in this article are solely those of the authors and do not necessarily represent those of their affiliated organizations, or those of the publisher, the editors and the reviewers. Any product that may be evaluated in this article, or claim that may be made by its manufacturer, is not guaranteed or endorsed by the publisher.
1. Ensanya AN, Anas A, Adam S, Salwa I, Melanie C, Anne Y, et al. Demineralization–remineralization dynamics in teeth and bone. Int J Nanomed. (2016) 11:4743–63. doi: 10.2147/IJN.S107624
2. Murdoch-Kinch Anne C., McLean, Ellen M. Minimally invasive dentistry. J Am Dent Assoc. (2003) 134:87–95. doi: 10.14219/jada.archive.2003.0021
3. Espinoza CAV, Aguilera JFO, Rizzante FAP, Maenosono RM, Ishikiriama SK. Preservation in young patients of pulp vitality and anatomical-functional restoration of the lost structure. Compend Contin Educ Dent. (2017) 38:144–9.
4. Tay FR, Pashley DH. Biomimetic remineralization of resin-bonded acid-etched dentin. J Dent Res. (2009) 88:719–724. doi: 10.1177/0022034509341826
5. Chen NL, Zhang YZ, Zhou Q, Jiao L, Chen H, Pashley J. Bonding of universal adhesives to dentine - old wine in new bottles? J Dent. (2015) 43:525–36. doi: 10.1016/j.jdent.2015.03.004
6. Sauro S, Osorio R, Watson TF, Toledano M. Influence of phosphoproteins' biomimetic analogs on remineralization of mineral-depleted resin–dentin interfaces created with ion-releasing resin-based systems. Dent Mater. (2015) 31:759–77. doi: 10.1016/j.dental.2015.03.013
7. Niu LN, Zhang W, Pashley DH, Breschi L, Mao J, Chen JH, et al. Biomimetic remineralization of dentin. Dent Mater. (2014) 30:77–96. doi: 10.1016/j.dental.2013.07.013
8. Fugolin APP, Pfeifer CS. New resins for dental composites. J Dent Res. (2017) 96:133549217. doi: 10.1177/0022034517720658
9. Makvandi P, Gu JT, Zare EN, Ashtari B, Moeini A, Tay FR, et al. Polymeric and inorganic nanoscopical antimicrobial fillers in dentistry. Acta Biomater. (2020) 101:69–101. doi: 10.1016/j.actbio.2019.09.025
10. Rodrigues MC, Natale LC, Arana-Chaves VE, Braga RR. Calcium and phosphate release from resin-based materials containing different calcium orthophosphate nanoparticles. J Biomed Mater Res B Appl Biomater. (2015) 103:1670–8. doi: 10.1002/jbm.b.33327
11. Parkinson CR, Hughes N, Hall C, Whelton H, Gallob J, Mason S. Three randomized clinical trials to assess the short-term efficacy of anhydrous 0.454% w/w stannous fluoride dentifrices for the relief of dentin hypersensitivity. Am J Dent. (2016) 29:25–32.
12. Freda NM, Veitz-Keenan A. Calcium sodium phosphosilicate had some benefit on dentine hypersensitivity. Evid Based Dent. (2016) 17:12. doi: 10.1038/sj.ebd.6401148
13. Gu L, Qi Y, Sui M, Qi H, Ling J. An in-situ remineralization design of hybrid layer with the use of sodium tripolyphosphate as a therapeutic primer. Chin J Stomatol Res. (2014) 8:97–103. doi: 10.3877/cma.j.issn.1674-1366.2014.02.003
14. Neha S, Cremer MA, Dolling ES, Hamid N, Stefan H, Marshall GW, et al. Influence of fluoride on the mineralization of collagen via the polymer-induced liquid-precursor (PILP) process. Dent Mater. (2018) 34:1378–90. doi: 10.1016/j.dental.2018.06.020
15. Xie XJ, Xing D, Wang L, Zhou H, Weir MD, Bai YX, et al. Novel rechargeable calcium phosphate nanoparticle-containing orthodontic cement. Int J Oral Sci. (2017) 9:24–32. doi: 10.1038/ijos.2016.40
16. Zhang W, Luo XJ, Niu LN, Yang HY, Yiu C, Wang TD, et al. Biomimetic intrafibrillar mineralization of type I collagen with intermediate precursors-loaded mesoporous carriers. Sci Rep. (2015) 5:11199. doi: 10.1038/srep11199
17. Zhang K, Zhang N, Weir MD, Reynolds MA, Bai Y, Xu HHK. Bioactive dental composites and bonding agents having remineralizing and antibacterial characteristics. Dent Clin N Am. (2017) 61:669–87. doi: 10.1016/j.cden.2017.05.002
18. Pajor K, Pajchel L, Kolmas J. Hydroxyapatite and fluorapatite in conservative dentistry and oral implantology—a review. Materials. (2019) 12:2683. doi: 10.3390/ma12172683
19. Moheet IA, Luddin N, Rahman IA, Kannan TP, Nik Abd Ghani NR, Masudi SM. Modifications of glass ionomer cement powder by addition of recently fabricated nano-fillers and their effect on the properties: a review. Eur J Dent. (2019) 13:470–7. doi: 10.1055/s-0039-1693524
20. Farooq I, Ali S, Al-Saleh S, AlHamdan EM, AlRefeai MH, Abduljabbar T, et al. Synergistic effect of bioactive inorganic fillers in enhancing properties of dentin adhesives—a review. Polymers. (2021) 13:2169. doi: 10.3390/polym13132169
21. Iafisco M, Degli Esposti L, Ramírez-Rodríguez GB, Carella F, Gómez-Morales J, Ionescu AC, et al. Fluoride-doped amorphous calcium phosphate nanoparticles as a promising biomimetic material for dental remineralization. Sci Rep. (2018) 8:17016. doi: 10.1038/s41598-018-35258-x
22. Zhao J, Liu Y, Sun W, Zhang H. Amorphous calcium phosphate and its application in dentistry. Chem Cent J. (2011) 5:1–7. doi: 10.1186/1752-153X-5-40
23. Melo MAS, Cheng L, Zhang K, Weir MD, Rodrigues LKA, Xu HHK. Novel dental adhesives containing nanoparticles of silver and amorphous calcium phosphate. Dent Mater. (2013) 29:199–210. doi: 10.1016/j.dental.2012.10.005
24. Chen C, Weir MD, Cheng L, Lin NJ, Lin-Gibson S, Chow LC, et al. Antibacterial activity and ion release of bonding agent containing amorphous calcium phosphate nanoparticles. Dent Mater. (2014) 30:891–901. doi: 10.1016/j.dental.2014.05.025
25. Balhaddad AA, Kansara AA, Hidan D, Weir MD, Xu HHK, Melo MAS. Toward dental caries: exploring nanoparticle-based platforms and calcium phosphate compounds for dental restorative materials. Bioactive Mater. (2019) 4:43–55. doi: 10.1016/j.bioactmat.2018.12.002
26. Dai Z, Liu M, Ma Y, Cao L, Xu HHK, Zhang K, et al. Effects of fluoride and calcium phosphate materials on remineralization of mild and severe white spot lesions. Biomed Res Int. (2019) 2019:1–13. doi: 10.1155/2019/1271523
27. Amaechi BT, Loveren CV. Fluorides and non-fluoride remineralization systems. Monogr Oral. (2013) 23:15–26. doi: 10.1159/000350458
28. Kulshrestha S, Khan S, Hasan S, Khan ME, Misba L, Khan AU. Calcium fluoride nanoparticles induced suppression of Streptococcus mutans biofilm: an in vitro and in vivo approach. Appl Microbiol Biot. (2016) 100:1901–14. doi: 10.1007/s00253-015-7154-4
29. Cheng L, Weir MD, Xu HHK, Kraigsley AM, Lin NJ, Lin-Gibson S, et al. Antibacterial and physical properties of calcium–phosphate and calcium–fluoride nanocomposites with chlorhexidine. Dent Mater. (2012) 28:573–83. doi: 10.1016/j.dental.2012.01.006
30. Brauer, Delia S. Bioactive glasses—structure and properties. Angew Chem Int Ed. (2015) 54:4160–81. doi: 10.1002/anie.201405310
31. Par M, Attin T, Tarle Z, Tauböck TT. A new customized bioactive glass filler to functionalize resin composites: acid-neutralizing capability, degree of conversion, and apatite precipitation. J Clin Med. (2020) 9:1173. doi: 10.3390/jcm9041173
32. Sauro S, Osorio R, Osorio E, Watson TF, Toledano M. Novel light-curable materials containing experimental bioactive micro-fillers remineralise mineral-depleted bonded-dentine interfaces. J Biomater Sci. (2013) 24:940–56. doi: 10.1080/09205063.2012.727377
33. Khvostenko D, Hilton TJ, Ferracane JL, Mitchell JC, Kruzic JJ. Bioactive glass fillers reduce bacterial penetration into marginal gaps for composite restorations. Dent Mater. (2016) 32:73–81. doi: 10.1016/j.dental.2015.10.007
34. Kawasaki K, Ruben J, Tsuda H, Huysmans MCDN, Takagi O. Relationship between mineral distributions in dentine lesions and subsequent remineralization in vitro. Caries Res. (2015) 34:395–403. doi: 10.1159/000016614
35. Simmer JP, Richardson AS, Hu YY, Smith CE, Hu CC. A post-classical theory of enamel biomineralization… and why we need one. Int J Oral Sci. (2012) 4:129–34. doi: 10.1038/ijos.2012.59
36. Kim J, Arola DD, Gu L, Kim YK, Sui M, Liu Y, et al. Functional biomimetic analogs help remineralize apatite-depleted demineralized resin-infiltrated dentin via a bottom–up approach. Acta Biomater. (2010) 6:2740–50. doi: 10.1016/j.actbio.2009.12.052
37. Dai L, Liu Y, Salameh Z, Khan S, Tay FR. Can caries-affected dentin be completely remineralized by guided tissue remineralization? Dental Hypotheses. (2011) 2:74–82. doi: 10.5436/j.dehy.2010.1.00011
38. Cao Y, Liu W, Ning T, Mei ML, Li QL, Lo EC, et al. A novel oligopeptide simulating dentine matrix protein 1 for biomimetic mineralization of dentine. Clin Oral Investig. (2014) 18:873–81. doi: 10.1007/s00784-013-1035-y
39. Zhong B, Peng C, Wang G, Tian L, Cai Q, Cui F. Contemporary research findings on dentine remineraliza-tion. J Tissue Eng Regen M. (2015) 9:1004–16. doi: 10.1002/term.1814
40. Tay F R., Pashley D H. Guided tissue remineralisation of partially demineralised human dentine. Biomaterials. (2008) 29:1127–37. doi: 10.1016/j.biomaterials.2007.11.001
41. George A, Veis A. Phosphorylated proteins and control over apatite nucleation, crystal growth, and inhibition. Chem Rev. (2008) 108:4670–93. doi: 10.1021/cr0782729
42. Wu S, Gu L, Huang Z, Sun Q, Chen H, Ling J, et al. Intrafibrillar mineralization of polyacrylic acid-bound collagen fibrils using a two-dimensional collagen model and Portland cement-based resins. Eur J Oral Sci. (2017) 125:72–80. doi: 10.1111/eos.12319
43. Cao Y, Mei ML, Xu J, Lo E, Li Q, Chu CH. Biomimetic mineralisation of phosphorylated dentine by CPP-ACP. J Dent. (2013) 41:818–25. doi: 10.1016/j.jdent.2013.06.008
44. Cochrane NJ, Cai F, Huq NL, Burrow MF, Reynolds EC. New approaches to enhanced remineralization of tooth enamel. J Dent Res. (2010) 89:1187–97. doi: 10.1177/0022034510376046
45. Xiao Z, Que K, Wang H, An R, Chen Z, Qiu Z, et al. Rapid biomimetic remineralization of the demineralized enamel surface using nano-particles of amorphous calcium phosphate guided by chimaeric peptides. Dent Mater. (2017) 33:1217–28. doi: 10.1016/j.dental.2017.07.015
46. Alshahrani A, Bin-Shuwaish MS, Al-Hamdan RS, Almohareb T, Maawadh AM, Al Deeb M, et al. Graphene oxide nano-filler based experimental dentine adhesive. A SEM / EDX, Micro-Raman and microtensile bond strength analysis. J Appl Biomater Func. (2020) 18:58809909. doi: 10.1177/2280800020966936
47. Toledano M, Aguilera FS, Osorio E, Cabello I, Toledano-Osorio M, Osorio R. Bond strength and bioactivity of ZN-doped dental adhesives promoted by load cycling. Microsc Microanal. (2015) 21:214–30. doi: 10.1017/S1431927614013658
48. Takeshita EM, Exterkate RAM, Delbem ACB, Ten Cate JM. Evaluation of different fluoride concentrations supplemented with trimetaphosphate on enamel de- and remineralization in vitro. Caries Res. (2011) 45:494–7. doi: 10.1159/000331209
49. Manarelli MM, Delbem ACB, Lima TMT, Castilho FCN, Pessan JP. In vitro remineralizing effect of fluoride varnishes containing sodium trimetaphosphate. Caries Res. (2014) 48:299–305. doi: 10.1159/000356308
50. Tiveron ARF, Delbem ACB, Gaban G, Sassaki KT, Pedrini D. In vitro enamel remineralization capacity of composite resins containing sodium trimetaphosphate and fluoride. Clin Oral Invest. (2015) 19:1899–904. doi: 10.1007/s00784-015-1404-9
51. Xu HHK, Moreau JL, Sun L, Chow LC. Novel CaF2 nanocomposite with high strength and fluoride ion release. J Dent Res. (2010) 89:739–45. doi: 10.1177/0022034510364490
52. Yang B, Flaim G, Dickens SH. Remineralization of human natural caries and artificial caries-like lesions with an experimental whisker-reinforced ART composite. Acta Biomater. (2011) 7:2303–9. doi: 10.1016/j.actbio.2011.01.002
53. Marovic D, Tarle Z, Hiller KA, Müller R, Ristic M, Rosentritt M, et al. Effect of silanized nanosilica addition on remineralizing and mechanical properties of experimental composite materials with amorphous calcium phosphate. Clin Oral Invest. (2014) 18:783–92. doi: 10.1007/s00784-013-1044-x
54. Langhorst SE, O Donnell JNR, Skrtic D. In vitro remineralization of enamel by polymeric amorphous calcium phosphate composite: quantitative microradiographic study. Dent Mater. (2009) 25:884–91. doi: 10.1016/j.dental.2009.01.094
55. Xu HHK, Moreau JL, Sun L, Chow LC. Nanocomposite containing amorphous calcium phosphate nanoparticles for caries inhibition. Dent Mater. (2011) 27:762–9. doi: 10.1016/j.dental.2011.03.016
56. Weir MD, Ruan J, Zhang N, Chow LC, Zhang K, Chang X, et al. Effect of calcium phosphate nanocomposite on in vitro remineralization of human dentin lesions. Dent Mater. (2017) 33:1033–44. doi: 10.1016/j.dental.2017.06.015
57. Weir MD, Chow LC, Xu HHK. Remineralization of demineralized enamelvia calcium phosphate nanocomposite. J Dent Res. (2012) 91:979–84. doi: 10.1177/0022034512458288
58. Braga, Ruggiero R. Calcium phosphates as ion-releasing fillers in restorative resin-based materials. Dent Mater. (2019) 35:3–14. doi: 10.1016/j.dental.2018.08.288
59. Zhang L, Weir MD, Chow LC, Antonucci JM, Chen J, Xu HHK. Novel rechargeable calcium phosphate dental nanocomposite. Dent Mater. (2016) 32:285–93. doi: 10.1016/j.dental.2015.11.015
60. Liang K, Weir MD, Xie X, Wang L, Reynolds MA, Li J, et al. Dentin remineralization in acid challenge environment via PAMAM and calcium phosphate composite. Dent Mater. (2016) 32:1429–40. doi: 10.1016/j.dental.2016.09.013
61. Jang JH, Lee MG, Ferracane JL, Davis H, Bae HE, Choi D, et al. Effect of bioactive glass-containing resin composite on dentin remineralization. J Dent. (2018) 75:58–64. doi: 10.1016/j.jdent.2018.05.017
62. Tezvergil-Mutluay A, Seseogullari-Dirihan R, Feitosa VP, Cama G, Brauer DS, Sauro S. Effects of composites containing bioactive glasses on demineralized dentin. J Dent Res. (2017) 96:999–1005. doi: 10.1177/0022034517709464
63. Par M, Tarle Z, Hickel R, Ilie N. Mechanical properties of experimental composites containing bioactive glass after artificial aging in water and ethanol. Clin Oral Invest. (2019) 23:2733–41. doi: 10.1007/s00784-018-2713-6
64. Li A, Cui Y, Gao S, Li Q, Xu L, Meng X, et al. Biomineralizing dental resin empowered by bioactive amphiphilic composite nanoparticles. ACS Appl Bio Mater. (2019) 2:1660–6. doi: 10.1021/acsabm.9b00051
65. Fernando D, Attik N, Pradelle-Plasse N, Jackson P, Grosgogeat B, Colon P. Bioactive glass for dentin remineralization: a systematic review. Mater Sci Eng C. (2017) 76:1369. doi: 10.1016/j.msec.2017.03.083
66. Aoba T. Solubility properties of human tooth mineral and pathogenesis of dental caries. Oral Dis. (2004) 10:249–57. doi: 10.1111/j.1601-0825.2004.01030.x
67. Jiang YL, Qiu W, Zhou XD, Li H, Lu JZ, Xu HH, et al. Quaternary ammonium-induced multidrug tolerant Streptococcus mutans persisters elevate cariogenic virulence in vitro. Int J Oral Sci. (2017) 9:e7. doi: 10.1038/ijos.2017.46
68. Featherstone JDB. Remineralization, the natural caries repair process—the need for new approaches. Adv Dental Res. (2009) 21:4–7. doi: 10.1177/0895937409335590
69. Tuncer C, Tuncer BB, Ulusoy Ç. Effect of fluoride-releasing light-cured resin on shear bond strength of orthodontic brackets. Am J Orthod Dentofac. (2009) 135:11–14. doi: 10.1016/j.ajodo.2008.09.016
70. Shah P, Jajoo SS, Chaudhary S, Patel A, Dhupar JK. Evaluation of adhesive bond strength, and the sustained release of fluoride by chitosan-infused resin-modified glass ionomer cement: an in vitro study. Int J Clin Pediatr Dent. (2021) 14:254–7. doi: 10.5005/jp-journals-10005-1943
71. Mickenautsch S, Yengopal V, Banerjee A. Retention of orthodontic brackets bonded with resin-modified GIC versus composite resin adhesives—a quantitative systematic review of clinical trials. Clin Oral Invest. (2012) 16:1–14. doi: 10.1007/s00784-011-0626-8
72. Cocco AR, de Oliveira Da Rosa WL, Da Silva AF, Lund RG, Piva E. A systematic review about antibacterial monomers used in dental adhesive systems: current status and further prospects. Dent Mater. (2015) 31:1345–62. doi: 10.1016/j.dental.2015.08.155
73. Capilouto ML, Depaola PF, Gron P. In vivo study of slow-release fluoride resin and enamel uptake. Caries Res. (1990) 24:441–5. doi: 10.1159/000261306
74. Samuel SM, Rubinstein C. Microhardness of enamel restored with fluoride and non-fluoride releasing dental materials. Braz Dent J. (2001) 12:35–8.
75. Nascimento PLDM, Fernandes MTG, Figueiredo FEDD, Faria-e-Silva AL. Fluoride-releasing materials to prevent white spot lesions around orthodontic brackets: a systematic review. Brazilian Dent J. (2016) 27:101–7. doi: 10.1590/0103-6440201600482
76. Fan M, Yang J, Xu HHK, Weir MD, Tao S, Yu Z, et al. Remineralization effectiveness of adhesive containing amorphous calcium phosphate nanoparticles on artificial initial enamel caries in a biofilm-challenged environment. Clin Oral Invest. (2021) 25:5375–90. doi: 10.1007/s00784-021-03846-3
77. Gao Y, Liang K, Weir MD, Gao J, Xu H. Enamel remineralization via poly(amido amine) and adhesive resin containing calcium phosphate nanoparticles. J Dent. (2019) 92:103262. doi: 10.1016/j.jdent.2019.103262
78. Yi J, Weir MD, Melo M, Li T, Xu H. Novel rechargeable nano-CaF 2 orthodontic cement with high levels of long-term fluoride release. J Dent. (2019) 90:103214. doi: 10.1016/j.jdent.2019.103214
79. Van Meerbeek B, De Munck J, Yoshida Y, Inoue S, Vargas M, Vijay P, et al. Adhesion to enamel and dentin: current status and future challenges (2003) 28:215–35.
80. ThanNaing S, Abdou A, Sayed M, Sumi Y, Tagami J, Hiraishi N. Dentin anti-demineralization potential of surface reaction-type pre-reacted glass-ionomer filler containing self-adhesive resin cement. Clin Oral Investig. (2022) 26:1333–42. doi: 10.1007/s00784-021-04107-z
81. Toledano M, Vallecillo-Rivas M, Aguilera FS, Osorio MT, Osorio E, Osorio R. Polymeric zinc-doped nanoparticles for high performance in restorative dentistry. J Dent. (2021) 107:103616. doi: 10.1016/j.jdent.2021.103616
82. Provenzi C, Collares FM, Cuppini M, Samuel SMW, Leitune VCB. Effect of nanostructured zirconium dioxide incorporation in an experimental adhesive resin. Clin Oral Invest. (2018) 22:1–10. doi: 10.1007/s00784-017-2311-z
83. Balbinot GS, Leitune VCB, Ogliari FA, Collares FM. Niobium silicate particles promote in vitro mineral deposition on dental adhesive resins. J Dent. (2020) 101:103449. doi: 10.1016/j.jdent.2020.103449
84. Cao C, Mei M, Li Q, Lo E, Chu C. Methods for biomimetic remineralization of human dentine: a systematic review. Int J Mol Sci. (2015) 16:4615–27. doi: 10.3390/ijms16034615
85. Huang Z, Qi Y, Zhang K, Gu L, Guo J, Wang R, et al. Use of experimental-resin-based materials doped with carboxymethyl chitosan and calcium phosphate microfillers to induce biomimetic remineralization of caries-affected dentin. J Mech Behav Biomed. (2019) 89:81–8. doi: 10.1016/j.jmbbm.2018.09.008
86. Zhe W, Yan O, Wu Z, Zhang L, Shao C, Fan J, et al. A novel fluorescent adhesive-assisted biomimetic mineralization. Nanoscale. (2018) 10:18980–7. doi: 10.1039/C8NR02078G
87. Spencer P, Ye Q, Kamathewatta NJB, Woolfolk SK, Bohaty BS, Misra A, Tamerler C. Chemometrics-assistedRaman spectroscopy characterization of tunable polymer-peptide hybrids for dental tissue repair. Front Mater. (2021) 8:681415. doi: 10.3389/fmats.2021.681415
88. Yuca E, Xie SX, Song L, Boone K, Tamerler C. Reconfigurable dual peptide tethered polymer system offers a synergistic solution for next generation dental adhesives. Int J Mol Sci. (2021) 22:6552. doi: 10.3390/ijms22126552
89. Pro Eta AC, Mannocd F, Foxton R, Watson TF, Feitosa VP, Carlo BD, et al. Experimental etch-and-rinse adhesives doped with bioactive calcium silicate-based micro-fillers to generate therapeutic resin–dentin interfaces - dental Materials. Dent Mater. (2013) 29:729–41. doi: 10.1016/j.dental.2013.04.001
90. Griffin SO, Oong E, Kohn W, Vidakovic B, Gooch BF, Bader J, et al. The effectiveness of sealants in managing caries lesions. J Dent Res. (2008) 87:169–74. doi: 10.1177/154405910808700211
91. Tellez M, Gray SL, Gray S, Lim S, Ismail AI. Sealants and dental caries: dentists' perspectives on evidence-based recommendations. J Am Dent Assoc. (2011) 142:1033–40. doi: 10.14219/jada.archive.2011.0324
92. Khatri SG, Madan KA, Srinivasan SR, Acharya S. Retention of moisture-tolerant fluoride-releasing sealant and amorphous calcium phosphate-containing sealant in 6-9-year-old children: a randomized controlled trial. J Indian Soc Pedod Prev Dent. (2019) 37:92–8. doi: 10.4103/JISPPD.JISPPD_173_18
93. Mathew SR, Narayanan RK, Vadekkepurayil K, Puthiyapurayil J. One-year clinical evaluation of retention ability and anticaries effect of a glass ionomer-based and a resin-based fissure sealant on permanent first molars: an in vivo study. Int J Clin Pediatr Dent. (2019) 12:553–9. doi: 10.5005/jp-journals-10005-1702
94. AlKahtani, Rawan N. The implications and applications of nanotechnology in dentistry: a review. Saudi Dental J. (2018) 30:107–16. doi: 10.1016/j.sdentj.2018.01.002
95. Fei X, Li Y, Weir MD, Baras BH, Wang H, Wang S, et al. Novel pit and fissure sealant containing nano-CaF2 and dimethylaminohexadecyl methacrylate with double benefits of fluoride release and antibacterial function. Dent Mater. (2020) 36:1241–53. doi: 10.1016/j.dental.2020.05.010
96. Ibrahim MS, Balhaddad AA, Garcia IM, Collares FM, Melo M. pH-responsive calcium and phosphate-ion releasing antibacterial sealants on carious enamel lesions in vitro. J Dent. (2020) 97:103323. doi: 10.1016/j.jdent.2020.103323
97. Gonçalves LS, Rodrigues RC, Andrade Junior CV, Soares RG, Vettore MV. The effect of hypochlorite and chlorhexidine as irrigant solutions for root canal disinfection: a systematic review of clinical trials. J Endodont. (2016) 42:527–32. doi: 10.1016/j.joen.2015.12.021
98. Baldasso FER, Roleto L, Silva VDD, Morgental RD, Kopper PMP. Effect of final irrigation protocols on microhardness reduction and erosion of root canal dentin. Braz Oral Res. (2017) 31:e40. doi: 10.1590/1807-3107bor-2017.vol31.0040
99. Al-Haddad A, Che Ab Aziz ZA. Bioceramic-based root canal sealers: a review. Int J Biomater. (2016) 2016:1–10. doi: 10.1155/2016/9753210
100. Baras BH, Sun J, Melo MAS, Tay FR, Oates TW, Zhang K, et al. Novel root canal sealer with dimethylaminohexadecyl methacrylate, nano-silver and nano-calcium phosphate to kill bacteria inside root dentin and increase dentin hardness. Dent Mater. (2019) 35:1479–89. doi: 10.1016/j.dental.2019.07.014
Keywords: remineralization, biomimetic pathway, calcium phosphate, adhesive, composite resin, resin-based dental materials, direct adhesive restoration
Citation: Mai S, Zhang Q, Liao M, Ma X and Zhong Y (2022) Recent Advances in Direct Adhesive Restoration Resin-Based Dental Materials With Remineralizing Agents. Front. Dent. Med. 3:868651. doi: 10.3389/fdmed.2022.868651
Received: 03 February 2022; Accepted: 17 March 2022;
Published: 21 April 2022.
Edited by:
Jingwei He, South China University of Technology, ChinaReviewed by:
Hao Yu, Fujian Medical University, ChinaCopyright © 2022 Mai, Zhang, Liao, Ma and Zhong. This is an open-access article distributed under the terms of the Creative Commons Attribution License (CC BY). The use, distribution or reproduction in other forums is permitted, provided the original author(s) and the copyright owner(s) are credited and that the original publication in this journal is cited, in accordance with accepted academic practice. No use, distribution or reproduction is permitted which does not comply with these terms.
*Correspondence: Sui Mai, bWFpc3VpQG1haWwuc3lzdS5lZHUuY24=
†These authors have contributed equally to this work
Disclaimer: All claims expressed in this article are solely those of the authors and do not necessarily represent those of their affiliated organizations, or those of the publisher, the editors and the reviewers. Any product that may be evaluated in this article or claim that may be made by its manufacturer is not guaranteed or endorsed by the publisher.
Research integrity at Frontiers
Learn more about the work of our research integrity team to safeguard the quality of each article we publish.