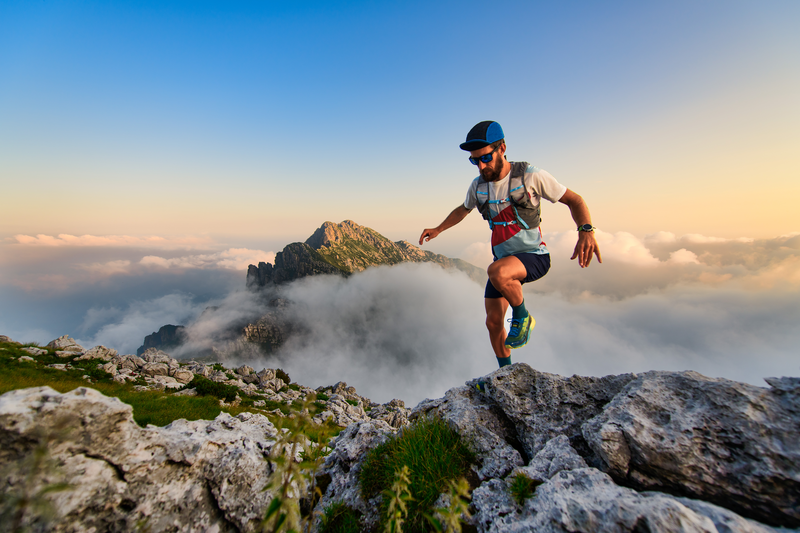
94% of researchers rate our articles as excellent or good
Learn more about the work of our research integrity team to safeguard the quality of each article we publish.
Find out more
MINI REVIEW article
Front. Dent. Med , 20 April 2022
Sec. Dental Materials
Volume 3 - 2022 | https://doi.org/10.3389/fdmed.2022.851331
Conventional method for removal of carious tissue using low speed drills usually induce noise and vibration, in addition to thermal and pressure effects that can be harmful to the pulp tissue and cause fear in children. Therefore, several alternative methods are being developed to try to minimize the unpleasant perception of the patient during caries removal. Chemical-mechanical removal of carious tissue goal is to selectively remove the carious lesion, which reduces the amount of bacteria inside the cavity without removing the tissue susceptible to remineralization. This method is also able to minimize the tactile perception by the patient during the manipulation of the lesion compared to the conventional method, and, therefore, it has been widely accepted among phobic patients, children and special needs patients. Due to the close relationship between dentin and pulp tissue, all injuries imposed on this dentin may have repercussions on the underlying pulp connective tissue. The morphological aspects of remaining dentin favor the diffusion of chemical components of dental materials, which can be toxic to the pulp tissue or even negatively interfere in the reparative process. Thus, considering the proximity between the applied material and the underlying pulp tissue, especially in deep cavities, there is a need to assess the biological behavior of dental materials against pulp cells, since aggressions to the pulp tissue can be caused not only by metabolites from microorganisms involved in dental caries but also by components that are released from these products. This subject was explored in this narrative literature review.
Dental caries is a multifactorial disease caused by the imbalance between the processes of tooth demineralization and remineralization, which induces the progressive destruction of the mineralized structure by the microorganisms in the adhered biofilm. Bacteria in dental biofilm can be characterized as acidogenic and aciduric according to their abitily to produce, survive and proliferate under acidic conditions. The development of a cariogenic microbiota is associated with the consumption of fermentable carbohydrates, essential for colony development, not removed from the mouth by routine oral hygiene methods. The treatment of the disease depends on reducing the population of cariogenic bacteria through the control of diet and oral hygiene, associated with the control of sequelae—caries lesions (1).
In recent decades, scientific research has enabled a greater understanding of the functional and structural biology of dental tissues and caries disease, leading to a less invasive approach and focusing on disease prevention and health promotion (2–4). Minimal intervention dentistry is an approach aimed at maximum preservation of dental structures with a focus on diagnosis, prevention and less invasive interventions (2).
Conventional methods of caries removal, using low speed drills, usually induce pain, noise and vibration, in addition to thermal and pressure effects that can be harmful to the pulp tissue (5). Therefore, several alternatives are being developed to try to minimize the unpleasant perception of the patient during caries removal, in addition to an attempt to better preserve healthy tissues. In view of the vision of minimal intervention and maximum preservation of dental tissues, it is necessary to clinically distinguish the dentin that should be removed (infected dentin) from the remineralizable dentin that can be preserved (affected dentin) (6). Infected dentin can be defined as the outermost layer of the lesion and is characterized as soft, necrotic and extremely contaminated. On the other hand, the affected dentin is located more internally, below the necrotic dentin, and is less contaminated and disorganized, with a capacity for remineralization (7, 8). Some methods that selectively remove decayed dentin are: chemical-mechanical removal, atraumatic restorative treatment (ART), polymer drills and laser irradiation. The success of these therapies is based on the selectivity of carious dentin removal, which reduces the amount of bacteria inside the cavity and keeps the tissue susceptible to remineralization (9, 10).
The chemical-mechanical removal of caries has been studied and the success of this therapy is based on the selective removal of the lesion, which reduces the amount of bacteria inside the cavity without removing the tissue susceptible to remineralization (7, 9). This method is also able to minimize the unpleasant perception by the patient during the manipulation of the lesion by the conventional method, and, therefore, it has been widely accepted among phobic patients, children and special needs patients (11–13). In a research carried out recently in Egypt, the effectiveness of the treatment was evaluated in terms of stopping the caries activity and controlling pain, fear and anxiety during dental care. The public chosen for the research was a group of pregnant women who, due to pregnancy, had not received dental treatment for some time, a condition that could make them more susceptible to pain and risk of infection. In this scenario, the use chemical-mechanical removal of caries was effective in reducing pain and offered more satisfactory results compared to the excavation performed, comparatively, in the conventional method (14). In addition, this method promotes less generation of aerosols, which reduces the chances of transmitting viral infections, such as contamination by the SARS-CoV-2 (15).
Due to the close relationship between dentin and pulp tissue, all injuries imposed on dentin may have repercussions on the underlying pulp connective tissue. The morphological aspects of the remaining dentin favor the diffusion of the chemical components of the materials throughout the dentin, which can be toxic to the pulp tissue or even negatively interfere in the reparative process. Thus, considering the proximity between the applied material and the underlying pulp tissue, especially in deep cavities, there is a need to assess the biological behavior of dental materials against pulp cells, since aggressions to the pulp tissue can be caused not only by metabolites from microorganisms involved in dental caries but also by components that are released from these products. This will be the central theme of this literature review.
Studies on the chemical-mechanical removal of caries emerged in the United States of America in the 1970s when Goldman and Kronman (16), studying the effects of 5% sodium hypochlorite, found that it was capable of promote the dissolution of decayed dentin (12). Later, however, this material was noted to be too unstable and corrosive for use in healthy tissue (16). In an attempt to minimize these problems, a solution known as Sorensen that contained glycine, sodium chloride and sodium hydroxide was incorporated into 5% sodium hypochlorite and the resulting product, 0.05% N-monochloroglycine (NMG) also called GK101, was shown to be effective in removing decayed tissue. Attempts to improve this solution were made and this was succeeded by the GK101 E (12).
Solely in the 1980s, after advances in studies on chemical-mechanical caries removal, was the use of the GK101 E solution was authorized by regulation agencies, which became known as Caridex™ (National Patent Medical Products Inc., New Jersey, USA). However, due to disadvantages such as high cost, short clinical validity and the need for large volumes of material (making it impractical), this product is no longer found in the market (12).
Trying to overcome the disadvantages of the Caridex™ system, Carisolv™ (MediTeam Dentalutveckling AB, Savedelen Sweden) was developed in the 1990s in Sweden. It consisted of two tubes, one containing a gel of lysine, glycine, leucine, acid glutamic, sodium chloride, erythrosine and carboxymethylcellulose, and one containing 0.5% sodium hypochlorite (11). Later, Carisolv™ was commercially available in a single syringe, with the same components, making its use easier and increasing its durability. Despite its effectiveness in removing decayed tissue, Carisolv™ requires specific instruments, increasing the cost of the product (9).
Faced with the need for a more accessible product for the application of the chemical- mechanical method to remove caries, a new formula was developed in 2003 in Brazil (5) and improved over the years. Papacárie Duo® is a successor to the Caridex™ and Carisolv™ system and its composition is basically a proteolytic enzyme that interacts with the partially degraded collagen of the necrotic carious tissue (5). Papacárie Duo® (Formula & Ação, São Paulo, SP, Brazil) is composed of papain, chloramine, toluidine blue, salts and thickening vehicle, which provides anti-inflammatory, bactericidal and bacteriostatic actions to the product (5). The main component, papain, comes from the latex of papaya leaves and fruits (Carica papaya) and is widely used in food, beverages, medicines and in industries in general (17, 18). It has long been used in wound healing due to its chemical debridement capacity, stimulation of granulation tissue formation and superficial epithelialization (19). Chloramine is a fundamental component due to its bactericidal and antiseptic properties (20), in addition to its important ability to chemically smooth decayed dentin, facilitating its removal (21). Papacárie Duo® does not require specific instruments for its use, thus being a practical, accessible and low-cost product that has been shown to be effective for caries removal with less painful stimuli and superior patient acceptance when compared to conventional treatments (22). It is noteworthy that, currently, we have a wide variety of products available on the market in addition to those mentioned above. Among them, we can mention Brix3000 (Santa Fé, Argentina) and Carie-care™ (Uni-Biotech Pharmaceuticals Private Limited, Chennai, India in collaboration with Vittal Mallya Scientific Research Foundation). Brix3000 presents a higher papain concentration (3000 U/mg) and bioencapsulation by buffer emulsion (EBE) technology (23, 24). These characteristics allows to remove the compromised tissue more easily without causing damage or pulp cytotoxicity. In addition Brix3000 presents anti-inflammatory properties which may favor the recovery of pulp tissue (25). Carie-care™ is composed of papain and clove oil, it acts paralyzing the activity of localized caries and preventing the advancement of lesions to the dental pulp. In addition, the clove oil has analgesic, antiseptic and anti-inflammatory properties, which acts preventing the dental pulp damage (26).
Due to the close relationship between dentin and pulp tissue, all injuries imposed on this dentin may have repercussions on the underlying pulp connective tissue (27). Thus, materials applied on the pulp-dentin complex can be biocompatible if applied on shallow and medium depth cavities where the dentin permeability is lower, but they may have undesirable effects on the pulp tissue when in deeper cavities, because of the thickness and characteristics of dentin tubules (28). Thus, considering the proximity between the applied material and the underlying pulp tissue, especially in deep cavities, there is a need to assess the biological behavior of dental materials against pulp cells.
In caries disease, the pulp balance can be disrupted by inflammation (29). Once the bacteria demineralize the enamel through their products and reach the dentin, they can spread through the dentinal tubules and reach the dental pulp. Lipopolysaccharide (LPS), present in the cell wall of Gram-negative bacteria, can penetrate the pulp and stimulate an inflammatory response from a variety of tissue-resident cells, including macrophages (30).
During an inflammatory process, macrophages are recruited to participate in the defense reaction. Their function includes phagocytosis of particles and release of biochemical mediators. The main goal is to attract a cell-mediated defense supply to achieve tissue repair. However, if their actions are exacerbated or not controlled local tissue destruction occurs (31, 32). When stimulated by external factors, such as lipopolysaccharide (LPS) from the wall of gram- negative bacteria or by endogenous factors such as interferon gamma (IFN), produced by T lymphocytes, macrophages have their functions greatly increased, becoming activated cells. An activated macrophage increases in size and volume, adheres strongly to surfaces, and produces large amounts of pro- and anti-inflammatory biological mediators, increasing the capacity for phagocytosis (32, 33).
The pulp tissue, then, reveals itself as an environment with capacity for repair, especially by presenting progenitor cells and repair signaling pathways induced by the dissolution of carious dentin, generating the secretion of mediators that promote the deposition of reparative dentin, angiogenesis and innervation (34).
Papacárie Duo® has no cytotoxic effects on macrophages and dental pulp cells at 0.5% concentration (35). At same concentration when tested in stem cells from human exfoliated deciduous teeth (SHED), Carisolv™ and Papacarie caused no cytotoxic effects (36). For pulp cells from deciduous teeth and Human pulp fibroblasts (FP6), Papacarie Duo® and Brix 3000 exhibited no cytotoxic, however the viability of cells exposed to the Papacarie Duo® was considerably lower when compared with cells exposed to Brix 3000 (25, 37). The viability of cells exposed to the highest concentration of Papacárie Duo® was 19.8% in contrast to cells exposed to Brix 3000 which was 52.5% (37).
With regard to cell differentiation, Papacárie Duo® inhibited the expression of the Runx2 and Ibsp genes (35). Runx2 coordinates multiple signaling pathways, related to both osteoblastic (38) and odontoblastic differentiation (39–41). In the process of odontoblast differentiation, this factor is expressed in odontoblast-like cells and in dental pulp stem cells in the region of restorative dentin deposition, which makes it a promoter of pulp stem cell differentiation (41, 42). On the other hand, the Ibsp gene, which encodes a phosphorylated glycoprotein, expressed mainly in mineralized connective tissues, plays multiple and distinct roles in the development, volume and mineralization of bone and dentin tissue. The induction of this gene coincides with the initial formation of mineralized matrix, and the maximum induction concentration is achieved during bone formation (43, 44). Thus, considering the roles played in cell differentiation by both genes, Runx2 and Ibsp, it appears that the inhibition of their relative gene expression is capable, therefore, of causing an inhibitory effect on the differentiation of dental pulp cells.
Unlike these results found for Runx2 and Ibsp genes, Papacárie Duo® induced the expression of Spp1 (35), a gene that encodes the osteopontin (OPN), a highly phosphorylated glycoprotein, a component of the mineralized extracellular matrix of bone and important for inflammatory and mineralization events, as it is able to regulate several physiological and pathological processes, including wound healing, bone remodeling, tumorigenesis, inflammation and immune responses (45). Immune response cells such as macrophages and dendritic cells secrete OPN, which is deposited at the dentin-predentin interface before the differentiation of odontoblast-like cells, suggesting that OPN has a role in the differentiation of these cells (46), in addition to being involved in the organization and deposition of mineralized tissue matrix (47). This, once deposited at the pulp-dentin interface, then induces the formation of tertiary dentin, as well as type I collagen, which is essential for the mineralization process (48). Thus, further research must be carried out, since the expression of Spp1 is related not only to mineralization events, but also to inflammatory events (49, 50).
It is noteworthy that cytotoxicity tests are widely used (51, 52), aiming to simulate in vitro the processes that occur in vivo and thus allowing the response of a specific cell type specific products can be investigated in this experimental approach (53). The cytotoxicity of a material also depends on the thickness of the remaining dentin and its permeability (54–56). Therefore, deeper cavities require greater care regarding the use of some dental materials, whose incorrect handling can result in significant tissue and pulp damage.
In the study of García-Contreras (2014) (57), the authors investigated the production of the pro-inflammatory lipid mediator Prostaglandin E2 (PGE2) and found an increase in its production in gingival fibroblasts, in addition to the fact that PGE2 production was synergistically increased in the presence of IL−1β. Bastos et al. (35) demonstrated that Papacárie Duo® increased Ptgs2 gene expression. The Ptgs2 gene encodes the enzyme cyclooxygenase-2 (COX-2), which is responsible for the synthesis of prostaglandins involved in the inflammatory response (58). IL-10, which is also an anti-inflammatory cytokine and a modulating factor of macrophage activation during an infection (59), was also induced by Papacárie Duo®, in the presence or not of LPS (35).
Studies which evaluated the materials Carisolv™, Papacarie Duo® and Brix 3000 showed no statistical differences between effectiveness of this materials and caries removal with rotary instruments, when evaluated mean values of total viable bacterial count after caries removal (22, 24, 60–62). However, patients which were treated with chemical-mechanical materials related better treatment experiences and less discomfort during the consult (22, 63). Chemical-mechanical caries removal material interacts with the collagen and acts on the degradation of the infected dentin, which facilitates its excavation (21), beside that they have bactericidal and anti-inflammatory properties which might promote less pain during treatments (22).
Regarding to effectiveness between the materials, Papacarie and Carisolv™ demonstrated similar results in the reduction of bacteria as Streptococcus mutans and Lactobacillus sp (64). An in-vitro study showed that Carisolv™ is more biocompatible to SHEDs than Papacarie and a clinical study demonstrated that Papacarie was significantly more efficient than Carisolv™ (36, 63). When compared to Carie Care and Papacarie Duo®, Brix-3000 reduced significantly the bacterial count before caries excavation, and it was less cytotoxic than Papacarie Duo® to human pulp fibroblasts (25, 65). This gel might be more effective due to the higher papain concentration (30,000 IU/mg) and to the encapsulation technology it uses.
According to Moimaz et al. (64), the meantime for chemical-mechanical caries removal was slower when compared to the conventional mechanical methods, however it was not statistically significant (64). The differences in time over the studies might be duo to the lack of standardization and the time of action determinate by each fabricant.
The chemical-mechanical removal of carious tissue has received increasing attention in the literature, but its use is still controversial, especially in more extensive and deeper lesions, in addition to being unfeasible for some techniques due to its high cost. The advantages of this method are its speed, ease of execution and patient comfort, as it does not generate anxiety and pain. These materials can be an excellent alternative in the context of the COVID-19 pandemics, as they reduce aerosols in care.
Despite requiring greater investment compared to the conventional method, these materials are being explored in other functionalities and not just in the chemical removal of decayed tissue. In recent research, the effectiveness of the Papacárie Duo® and Carisolv™ products has been proven when used as an alternative irrigating solution in cases of endodontic treatment with necrotic pulp, aiming at reducing the bacterial contingent within the canal system (66).
The chemical-mechanical removal of carious tissue has been studied and the success of this therapy is based on the selective removal of the lesion, which reduces the amount of bacteria inside the cavity without removing the tissue susceptible to remineralization. The proximity between the applied material and the underlying pulp tissue should be considered, especially in deep cavities, there is a need to assess the biological behavior of dental materials against pulp cells. Nonetheless, this method is also able to minimize the perception by the patient during the manipulation of the lesion by the conventional method, and, therefore, it has been widely accepted among phobic patients, children and special needs patients.
All authors listed have made a substantial, direct, and intellectual contribution to the work and approved it for publication.
This study was supported by The São Paulo Research Foundation (Research grant 2019/00204-2 to FP-S and Research fellowship 2020/06607-8 to RL).
The authors declare that the research was conducted in the absence of any commercial or financial relationships that could be construed as a potential conflict of interest.
All claims expressed in this article are solely those of the authors and do not necessarily represent those of their affiliated organizations, or those of the publisher, the editors and the reviewers. Any product that may be evaluated in this article, or claim that may be made by its manufacturer, is not guaranteed or endorsed by the publisher.
1. Frencken JE, Peters MC, Manton DJ, Leal SC, Gordan VV, Eden E. Minimal intervention dentistry for managing dental caries - a review: report of a FDI task group. Int Dent J. (2012) 5:223–43. doi: 10.1111/idj.12007
2. Murdoch-Kinch CA, McLean ME. Minimally invasive dentistry. J Am Dent Assoc. (2003) 1:87–95. doi: 10.14219/jada.archive.2003.0021
3. Schwendicke F, Foster Page LA, Smith LA, Fontana M, Thomson WM, Baker SR. To fill or not to fill: a qualitative cross-country study on dentists' decisions in managing non- cavitated proximal caries lesions. Implement Sci. (2018) 1:54. doi: 10.1186/s13012-018-0744-7
4. Fernández CE, González-Cabezas C, Fontana M. Minimum intervention dentistry in the US: an update from a cariology perspective. Br Dent J. (2020) 7:483–6. doi: 10.1038/s41415-020-2219-x
5. Bussadori SK, Castro LC, Galvão AC. Papain gel: a new chemo-mechanical caries removal agent. J Clin Pediatr Dent. (2005) 2:115–9. doi: 10.17796/jcpd.30.2.xq641w720u101048
6. Massara ML, Alves JB, Brandão PR. Atraumatic restorative treatment: clinical, ultrastructural and chemical analysis. Caries Res. (2002) 6:430–6. doi: 10.1159/000066534
8. Kuhn E, Chibinski AC, Reis A, Wambier DS. The role of glass ionomer cement on the remineralization of infected dentin: an in vivo study. Pediatr Dent. (2014) 4:E118–124.
9. Martins MD, Fernandes KP, Motta LJ, Santos EM, Pavesi VC, Bussadori SK. Biocompatibility analysis of chemomechanical caries removal material Papacárie on cultured fibroblasts and subcutaneous tissue. J Dent Child. (2009) 2:123–9.
10. Hamama H, Yiu C, Burrow MF, King NM. Systematic review and meta-analysis of randomized clinical trials on chemomechanical caries removal. Oper Dent. (2015) 4:E167–78. doi: 10.2341/14-021-LIT
11. Ericson D, Zimmerman M, Raber H, Götrick B, Bornstein R, Thorell J. Clinical evaluation of efficacy and safety of a new method for chemo-mechanical removal of caries. A multi-centre study Caries Res. (1999) 3:171–7. doi: 10.1159/000016513
12. Beeley JA, Yip HK, Stevenson AG. Chemochemical caries removal: a review of the techniques and latest developments. Br Dent J. (2000) 8:427–30. doi: 10.1038/sj.bdj.4800501
13. Chowdhry S, Saha S, Samadi F, Jaiswal JN, Garg A, Chowdhry P. Recent vs conventional methods of caries removal: a comparative in vivo study in pediatric patients. Int J Clin Pediatr Dent. (2015) 1:6–11. doi: 10.5005/jp-journals-10005-1275
14. Adham MM, El Catalan MK, Abdelaziz WE, Rashad AS. The impact of minimally invasive restorative techniques on perception of dental pain among pregnant women: a randomized controlled clinical trial. BMC Oral Health. (2021) 1:76. doi: 10.1186/s12903-021-01432-3
15. Ge ZY, Yang LM, Xia JJ, Fu XH, Zhang YZ. Possible aerosol transmission of COVID-19 and special precautions in dentistry. J Zhejiang Univ Sci B. (2020) 5:361–8. doi: 10.1631/jzus.B2010010
16. Goldman M, Kronman JHA. preliminary report on a chemomechanical means of removing caries. J Am Dent Assoc. (1976) 6:1149–53. doi: 10.14219/jada.archive.1976.0249
17. Kimmel JR, Smith EL, Crystalline papain I. Preparation, specificity, and activation. J Biol Chem. (1954) 2:515–31. doi: 10.1016/S0021-9258(18)65669-8
18. Kimmel JR, Smith EL. The properties of papain. Adv Enzymol Relat Subj Biochem. (1957) 19:267–334. doi: 10.1002/9780470122648.ch4
19. Mandelbaum SH, Di Santis ÉP, Mandelbaum MHSA. Cicatrization: current concepts and auxiliary resources-Part II Anais Brasileiros de. Dermatologia. (2003) 5:521–2. doi: 10.1590/S0365-05962003000500002
20. Nagl M, Nguyen VA, Gottardi W, Ulmer H, Höpfl R. Tolerability and efficacy of N- chlorotaurine in comparison with chloramine T for the treatment of chronic leg ulcers with a purulent coating: a randomized phase II study. Br J Dermatol. (2003) 3:590–7. doi: 10.1046/j.1365-2133.2003.05432.x
21. Maragakis GM, Hahn P, Hellwig E. Chemomechanical caries removal: a comprehensive review of the literature. Int Dent J. (2001) 4:291–9. doi: 10.1002/j.1875-595X.2001.tb00841.x
22. Cardoso M, Coelho A, Lima R, Amaro I, Paula A, Marto CM, et al. Efficacy and patient's acceptance of alternative methods for caries removal-a systematic review. J Clin Med. (2020) 11:3407. doi: 10.3390/jcm9113407
23. Torresi F, Besereni L. Effectiveness method of chemomechanical removal of dental caries as papain in adults. J Rev Assoc Paul cir Dent. (2017) 266–9.
24. Ismail MMM, Al Haidar AHMJ. Evaluation of the efficacy of caries removal using papain gel (Brix 3000) and smart preparation bur (in vivo comparative study). J Pharma Sci and Res. (2019) 2:444–9.
25. Santos TML, Bresciani E, Matos FS, Camargo SEA, Hidalgo APT, Rivera LML, et al. Comparison between conventional and chemomechanical approaches for the removal of carious dentin: an in vitro study. Sci Rep. (2020) 1:8127. doi: 10.1038/s41598-020-65159-x
26. Ramamoorthi S, Nivedhitha MS, Vanajassun PP. Effect of two different chemomechanical caries removal agents on dentin microhardness: an in vitro study. J Conserv Dent. (2013) 5:429–33. doi: 10.4103/0972-0707.117520
27. Orchardson R, Cadden SW. An update on the physiology of the dentine-pulp complex. Dent Update. (2001) 4:200–6. doi: 10.12968/denu.2001.28.4.200
28. Marshall GW, Marshall SJ, Kinney JH, Balooch M. The dentin substrate: structure and properties related to bonding. J Dent. (1997) 6:441–58. doi: 10.1016/S0300-5712(96)00065-6
29. Farges JC, Alliot-Licht B, Renard E, Ducret M, Gaudin A, Smith AJ, et al. Dental pulp defence and repair mechanisms in dental caries. Mediators Inflamm. (2015) 2015:230251. doi: 10.1155/2015/230251
30. Cooper PR, Takahashi Y, Graham LW, Simon S, Imazato S, Smith AJ. Inflammation-regeneration interplay in the dentine-pulp complex. J Dent. (2010) 9:687–97. doi: 10.1016/j.jdent.2010.05.016
31. Okiji T, Kawashima N, Kosaka T, Matsumoto A, Kobayashi C, Suda H. An immunohistochemical study of the distribution of immunocompetent cells, especially macrophages and Ia antigen-expressing cells of heterogeneous populations, in normal rat molar pulp. J Dent Res. (1992) 5:1196–202. doi: 10.1177/00220345920710051201
32. Murray PJ, Wynn TA. Protective and pathogenic functions of macrophage subsets. Nat Rev Immunol. (2011) 11:723–37. doi: 10.1038/nri3073
33. Metzger Z. Macrophages in periapical lesions. Endod Dent Traumatol. (2000) 1:1–8. doi: 10.1034/j.1600-9657.2000.016001001.x
34. Chmilewsky F, Jeanneau C, Dejou J, About I. Sources of dentin-pulp regeneration signals and their modulation by the local microenvironment. J Endod. (2014) 4:S19–25. doi: 10.1016/j.joen.2014.01.012
35. Bastos LA, Silva FL, Thomé JPQ, Arnez MFM, Faccioli LH, Paula-Silva FWG. Effects of papain-based gel used for caries removal on macrophages and dental pulp cells. Braz Dent J. (2019) 5:484–90. doi: 10.1590/0103-6440201902560
36. Maru V, Madkaikar M, Shabrish S, Kambli P, Dalvi A, Setia P. Evaluation and comparison of cytotoxicity and bioactivity of chemomechanical caries removal agents on stem cells from human exfoliated deciduous teeth. Eur Arch Paediatr Dent. (2021). doi: 10.1007/s40368-021-00684-5
37. Guedes FR, Bonvicini JF, de Souza GL, da Silva WH, Moura CC, Paranhos LR, et al. Cytotoxicity and dentin composition alterations promoted by different chemomechanical caries removal agents: a preliminary in vitro study. J Clin Exp Dent. (2021) 8:e826–34. doi: 10.4317/jced.58208
38. Komori T. Regulation of bone development and extracellular matrix protein genes by RUNX2. Cell Tissue Res. (2010) 1:189–95. doi: 10.1007/s00441-009-0832-8
39. D'Souza RN, Aberg T, Gaikwad J, Cavender A, Owen M, Karsenty G, et al. Cbfa1 is required for epithelial-mesenchymal interactions regulating tooth development in mice. Development. (1999) 13:2911–20. doi: 10.1242/dev.126.13.2911
40. Yang F, Xu N, Li D, Guan L, He Y, Zhang Y, et al. Feedback loop between RUNX2 and the E3 ligase SMURF1 in regulation of differentiation of human dental pulp stem cells. J Endod. (2014) 10:1579–86. doi: 10.1016/j.joen.2014.04.010
41. Daltoé MO, Paula-Silva FW, Faccioli LH, Gatón-Hernández PM, De Rossi A, Bezerra Silva LA. Expression of mineralization markers during pulp response to biodentine and mineral trioxide aggregate. J Endod. (2016) 4:596–603. doi: 10.1016/j.joen.2015.12.018
42. Han N, Zheng Y, Li R, Li X, Zhou M, Niu Y, et al. β-catenin enhances odontoblastic differentiation of dental pulp cells through activation of Runx2. PLoS ONE. (2014) 9:e88890. doi: 10.1371/journal.pone.0088890
43. Ganss B, Kim RH, Sodek J. Bone sialoprotein. Crit Rev Oral Biol Med. (1999) 1:79–98. doi: 10.1177/10454411990100010401
44. Ogata Y. Bone sialoprotein and its transcriptional regulatory mechanism. J Periodontal Res. (2008) 2:127–35. doi: 10.1111/j.1600-0765.2007.01014.x
45. Pagel CN, Wasgewatte Wijesinghe DK, Taghavi Esfandouni N, Mackie EJ. Osteopontin, inflammation and myogenesis: influencing regeneration, fibrosis and size of skeletal muscle. J Cell Commun Signal. (2014) 2:95–103. doi: 10.1007/s12079-013-0217-3
46. Saito K, Nakatomi M, Ida-Yonemochi H, Kenmotsu S, Ohshima H. The expression of GM-CSF and osteopontin in immunocompetent cells precedes the odontoblast differentiation following allogenic tooth transplantation in mice. J Histochem Cytochem. (2011) 5:518–29. doi: 10.1369/0022155411403314
47. Bailey S, Karsenty G, Gundberg C, Vashishth D. Osteocalcin and osteopontin influence bone morphology and mechanical properties. Acad Sci. (2017) 1:79–84. doi: 10.1111/nyas.13470
48. Saito K, Nakatomi M, Ida-Yonemochi H, Ohshima H. Osteopontin is essential for type I collagen secretion in reparative dentin. J Dent Res. (2016). 95:1034–41. doi: 10.1177/0022034516645333
49. Scatena M, Liaw L, Giachelli CM. Osteopontin: a multifunctional molecule regulating chronic inflammation and vascular disease. Arterioscler Thromb Vasc Biol. (2007) 11:2302–9. doi: 10.1161/ATVBAHA.107.144824
50. Chatakun P, Núñez-Toldrà R, Díaz López EJ, Gil-Recio C, Martínez-Sarrà E, Hernández-Alfaro F, et al. The effect of five proteins on stem cells used for osteoblast differentiation and proliferation: a current review of the literature. Cell Mol Life Sci. (2014) 1:113–42. doi: 10.1007/s00018-013-1326-0
51. de Azevedo CL, Marques MM, Bombana AC. Cytotoxic effects of cyanoacrylates used as retrograde filling materials: an in vitro analysis. Pesqui Odontol Bras. (2003) 2:113–8. doi: 10.1590/S1517-74912003000200003
52. Cavalcanti BC, Costa-Lotufo LV, Moraes MO, Burbano RR, Silveira ER, Cunha KM, et al. Genotoxicity evaluation of kaurenoic acid, a bioactive diterpenoid present in Copaiba oil. Food Chem Toxicol. (2006) 3:388–92. doi: 10.1016/j.fct.2005.08.011
53. Schmalz G. Use of cell cultures for toxicity testing of dental materials–advantages and limitations. J Dent. (1994) 2:S6–11. doi: 10.1016/0300-5712(94)90032-9
54. Murray PE, Lumley PJ, Ross HF, Smith AJ. Tooth slice organ culture for cytotoxicity assessment of dental materials. Biomaterials. (2000) 16:1711–21. doi: 10.1016/S0142-9612(00)00056-9
55. Duque C, Hebling J, Smith AJ, Giro EM, Oliveira MF, de Souza Costa CA. Reactionary dentinogenesis after applying restorative materials and bioactive dentin matrix molecules as liners in deep cavities prepared in nonhuman primate teeth. J Oral Rehabil. (2006) 6:452–61. doi: 10.1111/j.1365-2842.2005.01585.x
56. Costa CA, Ribeiro AP, Giro EM, Randall RC, Hebling J. Pulp response after application of two resin modified glass ionomer cements (RMGICs) in deep cavities of prepared human teeth. Dent Mater. (2011) 7:e158–70. doi: 10.1016/j.dental.2011.04.002
57. Garcia-Contreras R, Scougall-Vilchis RJ, Contreras-Bulnes R, Kanda Y, Nakajima H, Sakagami H. Cytotoxicity and pro-inflammatory action of chemo-mechanical caries- removal agents against oral cells. In Vivo. (2014) 4:549–56.
58. Clària J. Cyclooxygenase-2 biology. Curr Pharm Des. (2003) 27:2177–90. doi: 10.2174/1381612033454054
59. Couper KN, Blount DG, Riley EM. IL-10: the master regulator of immunity to infection. J Immunol. (2008) 9:5771–7. doi: 10.4049/jimmunol.180.9.5771
60. Soni HK, Sharma A, Sood PB. A comparative clinical study of various methods of caries removal in children. Eur Arch Paediatr Dent. (2015) 1:19–26. doi: 10.1007/s40368-014-0140-1
61. AlHumaid J. Efficacy and efficiency of papacarie versus conventional method in caries removal in primary teeth: an sem study. Saudi J Med Med Sci. (2020) 1:41–45. doi: 10.4103/sjmms.sjmms_104_18
62. Asal MA, Abdellatif AM, Hammouda HE. Clinical and microbiological assessment of carisolv and polymer bur for selective caries removal in primary molars. Int J Clin Pediatr Dent. (2021) 3:357–63. doi: 10.5005/jp-journals-10005-1956
63. Hegde S, Kakti A, Bolar DR, Bhaskar SA. Clinical efficiency of three caries removal systems: rotary excavation, carisolv, and papacarie. J Dent Child. (2016) 1:22–8.
64. Moimaz SAS, Okamura AQC, Lima DC, Saliba TA, Saliba NA. Clinical and microbiological analysis of mechanical and chemomechanical methods of caries removal in deciduous teeth. Oral Health Prev Dent. (2019)3:283–8. doi: 10.3290/j.ohpd.a42659
65. Inamdar MS, Chole DG, Bakle SS, Gandhi NP, Hatte NR, Rao MP. Comparative evaluation of BRIX3000, CARIE CARE, and SMART BURS in caries excavation: An in vivo study. J Conserv Dent. (2020) 2:163–8. doi: 10.4103/JCD.JCD_269_20
Keywords: caries removal, chemical-mechanical methods, biocompatibility, dental pulp, dentin
Citation: Leme RD, Lamarque GdCC, Bastos LA, Arnez MFM and Paula-Silva FWG (2022) Minimal Intervention Dentistry: Biocompatibility and Mechanism of Action of Products for Chemical-Mechanical Removal of Carious Tissue. Front. Dent. Med. 3:851331. doi: 10.3389/fdmed.2022.851331
Received: 09 January 2022; Accepted: 16 March 2022;
Published: 20 April 2022.
Edited by:
Sirin Güner Onur, Altinbaş University, TurkeyReviewed by:
Aliye Tugce Gurcan, Altinbaş University, TurkeyCopyright © 2022 Leme, Lamarque, Bastos, Arnez and Paula-Silva. This is an open-access article distributed under the terms of the Creative Commons Attribution License (CC BY). The use, distribution or reproduction in other forums is permitted, provided the original author(s) and the copyright owner(s) are credited and that the original publication in this journal is cited, in accordance with accepted academic practice. No use, distribution or reproduction is permitted which does not comply with these terms.
*Correspondence: Francisco Wanderley Garcia Paula-Silva, ZnJhbmNpc2NvZ2FyY2lhQGZvcnAudXNwLmJy
Disclaimer: All claims expressed in this article are solely those of the authors and do not necessarily represent those of their affiliated organizations, or those of the publisher, the editors and the reviewers. Any product that may be evaluated in this article or claim that may be made by its manufacturer is not guaranteed or endorsed by the publisher.
Research integrity at Frontiers
Learn more about the work of our research integrity team to safeguard the quality of each article we publish.