- 1Faculty of Dentistry, Oral and Craniofacial Sciences, King’s College London, London, United Kingdom
- 2Laboratory of Odontogenesis and Osteogenesis, Institute of Animal Physiology and 21 Genetics, Brno, Czech Republic
Management of the growing adult orthodontic patient population must contend with challenges particular to orthodontic treatment in adults. These include a limited rate of tooth movement, increased incidence of periodontal complications, higher risk of iatrogenic root resorption and pulp devitalisation, resorbed edentulous ridges, and lack of growth potential. The field of regenerative dentistry has evolved numerous methods of manipulating cellular and molecular processes to rebuild functional oral and dental tissues, and research continues to advance our understanding of stem cells, signalling factors that stimulate repair and extracellular scaffold interactions for the purposes of tissue engineering. We discuss recent findings in the literature to synthesise our understanding of current and prospective approaches based on biological repair that has the potential to improve orthodontic treatment outcomes in adult patients. Methods such as mesenchymal stem cell transplantation, biomimetic scaffold manipulation, and growth factor control may be employed to overcome the challenges described above, thereby reducing adverse sequelae and improving orthodontic treatment outcomes in adult patients. The overarching goal of such research is to eventually translate these regenerative techniques into clinical practice, and establish a new gold standard of safe, effective, autologous therapies.
Introduction
Engagement with orthodontic treatment amongst adults is on the rise, with a noticeable shift in the orthodontic patient demographic over the past two decades (1, 2). This may be attributed to improved dental awareness amongst the general public, better accessibility of dental services, and increased social acceptability (2, 3, 4). The physiological, pathological and behavioural differences between adult orthodontic patients and their child and adolescent counterparts create unique considerations and challenges in their treatment (4–6). Almost all current treatments rely on introducing foreign materials into or onto the dentofacial tissues, whereas the ideal therapy would utilise our understanding of tissue biology to regenerate natural tissues.
Regenerative dentistry encompasses repair and regeneration of functional dental tissues by understanding tissue development, growth, underlying mechanisms of molecular signalling, that drive repair process, and employment of biocompatible scaffolds. Recent years have seen remarkable progress towards the goal of regenerating autologous dental and craniofacial tissues for therapeutic use (7), and these techniques can be applied to some of the clinical problems faced in orthodontic treatment of adult patients.
This review summarises current and cutting-edge research in the field of regenerative dentistry and presents the perspective of its translation into therapeutic approaches for adult orthodontic patients.
We critically discuss six themes relevant to adult orthodontic challenges which may be tackled with regenerative approaches (Figure 1). Cell turnover and healing potential are diminished with age, limiting the speed of orthodontic tooth movements (4–6). Completion of bony growth in adolescence results in increased reliance on surgical corrections (4, 6). Adults experience a higher incidence of periodontal disease which affects the reaction of the periodontium to orthodontic forces, and tooth loss leaves edentulous alveolar ridges that resorb and impair orthodontic tooth movement into the space (4, 5). Root resorption, a common adverse consequence of orthodontic treatment, shows greater risk in adult patients (8), as does tooth devitalisation (9).
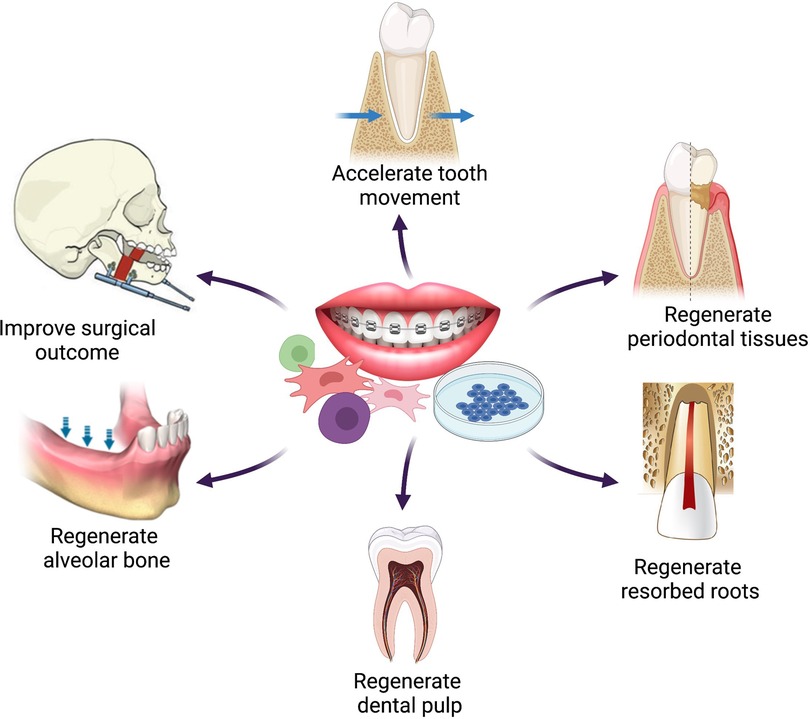
Figure 1 Schematic representation of the six themes of potential applications of regenerative techniques in adult orthodontics. Figure created with BioRender.com.
Accelerating tooth movement
Orthodontic tooth movement (OTM) in the absence of adverse sequelae becomes limited with aging as periodontal remodelling potential reduces, but this may be augmented by manipulation of regenerative techniques. OTM arises from coordinated remodelling events in the alveolus and periodontal structures surrounding a tooth root, stimulated by mechanical force. Its efficiency depends on cellular and extracellular activity, establishment of local molecular gradients, periodontal and dental health and stability (10, 11). The pressure-tension theory states that orthodontic forces create differential compression and tension on different sides of the periodontal ligament (PDL), generating local disruptions in vascularity, a mild inflammatory response, and an altered biochemical environment which enables movement of the root (Figure 2) (12). OTM is slower and delayed in adults compared to children due to age-related physiological changes: cellular activity and turnover decrease, the PDL becomes more fibrous and less vascular, and alveolar bone becomes denser and more calcified (4, 11, 13).
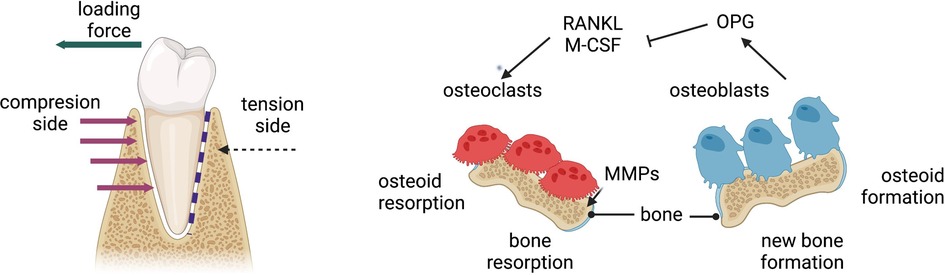
Figure 2 Mechanism of orthodontic tooth movement due to compression and tension generated from orthodontic loading. MMPs, matrix metalloproteinases; OPG, osteoprotegrin; M-CSF, macrophage colony-stimulating factor; RANKL, receptor activator of nuclear factor kappa-Β ligand. Figure created with BioRender.com.
Modern methods to accelerate OTM include corticotomy, piezosurgery, microperforations, low-level laser therapy, photobiomodulation, and vibration-based strategies (14, 15). Corticotomies and other surgical techniques produce a regional acceleratory phenomenon and stimulate a surge in bone remodelling, but may lead to lower bone density in that region (11, 16). There is a lack of high-quality evidence to rigorously evaluate the efficacy of the other newer techniques, which are still being keenly studied (14).
To meet the need for alternative, effective, non-invasive methods, stem cell therapy may draw inspiration from the periodontium's natural response to orthodontic forces: endogenous mesenchymal and haematopoietic stem cells are activated to differentiate into osteoblasts and osteoclasts, respectively, within hours of force application (17). Studies have demonstrated that transplanting adipose-derived stem cells (ASCs) into the PDL can increase the rate of OTM by stimulating resident PDL stem cells (PDLSCs) to accelerate PDL reorganisation and bone remodelling, or by direct effects of the MSCs on the tissues (18). ASCs have high osteogenic and periodontal differentiation potential (19) and can be transplanted into the PDL in a minimally invasive manner via infiltrative or intraligamentary injections (10). PDLSCs are also mechanosensitive and contribute to periodontal and osseous remodelling during OTM (20, 21).
On a molecular level, inflammatory markers such as interleukin-11 (IL-11) are produced by PDLSCs in response to mechanical forces, and these molecules regulate the proliferation and differentiation of osteoclasts and osteoblasts (22). Thus, influence over the availability and activity of such molecules may allow more predictable control of alveolar remodelling associated with OTM. Similarly, in vivo and in vitro studies have demonstrated relationships between prostaglandins, particularly Prostaglandin E2, and accelerated tooth movement when subjected to forces as in orthodontic therapy (23). The highly conserved Wnt/β-catenin pathway, which is ubiquitous in embryonic development and many self-renewing adult cells, is activated by mechanical stimulation and maintains bone homeostasis during OTM (21). With further research, future therapies may be able to accelerate OTM by transplanting autologous MSCs into the periodontium, or by controlling resident endogenous MSCs through pharmacological manipulation of relevant signalling pathways.
Regenerating periodontal tissues
The periodontium is a complex functional unit consisting of cementum, alveolar bone, gingivae, and PDL. Periodontitis, characterised by host inflammatory responses causing irreversible destruction of periodontal tissues, affects approximately 20%–50% of the global population (7) and adults significantly more than adolescents (24). Individuals undergoing orthodontic treatment are not at higher risk of developing periodontitis, even with reduced periodontal attachment, provided active disease is stabilised and plaque is controlled (4, 25). Periodontitis frequently causes unfavourable tooth migration which may benefit from orthodontic repositioning (26, 27). Loss of periodontal support reduces the PDL surface area bearing the orthodontic forces, so a certain force will generate larger moments and more extrusive movements than if applied to a tooth with physiological periodontal levels (28).
Damaged periodontal tissues may be repaired (whereby the lost tissues are replaced by a different structure from the original, for example following non-surgical root scaling) or regenerated (whereby damaged tissues are restored to their original form and function). Currently employed regenerative techniques have had some success in clinical applications for severe defects (27): guided tissue regeneration (GTR) obstructs ingress of epithelial and gingival cells into the defect, tissue grafts may come from autogenous tissues or animal-derived bone particles, and biologic agents (30) stimulate regenerative cellular processes. However, the search for more clinically efficient autologous periodontal regenerative techniques continues, with keen interest in stem cell therapy (31).
Experiments in animal models have shown that implantation of MSCs from various sources into periodontal defects can contribute to periodontal tissue regeneration, including from the dental pulp, the apical papilla, and Hertwig's epithelial root sheath (7, 29). However, most interest lies in unlocking the potential of MSCs and progenitor cells in the PDL to regenerate the periodontium, as the local resident stem cells. Human PDLSCs can differentiate into osteoblast-, fibroblast- and cementoblast-like cells and synthesise bone and collagen fibres when transplanted into periodontal defects, indicating a potential to regenerate the whole bone-PDL-cementum complex (32–34). Engineered cell sheets aid transplantation by preventing unwanted cell diffusion away from the defect (7, 35–37); but 3-dimensional (3D) scaffolds (such as hydroxyapatite-tricalcium phosphate or collagen scaffolds) (27, 38, 39) are superior in reconstituting the complex architecture of the periodontium; they allow cell mobility and interaction with the scaffold biomaterial and release growth and differentiation factors (7). Natural extracellular matrix (ECM) scaffolds may be obtained non-invasively, from MSCs isolated from human urine: PDLSCs cultured on this ECM show superior proliferation, osteogenesis and angiogenesis compared to controls (40). Indeed, MSC-derived ECM is more effective than other kinds of ECM at improving desirable stem cell characteristics, whilst maintaining stemness and even rejuvenating aged stem cells (40). This is valuable since PDLSCs are known to lose proliferation and differentiation capacity and increase apoptotic tendencies with age (41).
Additional techniques can enhance stem cells' natural capabilities, for example, PDLSCs treated in a conditioned medium from developing apical tooth germs showed increased capacity to generate cementum and periodontal-like structures, in contrast to untreated PDLSCs which generated more connective tissue (42). Furthermore, epigenetics may also play a role: increased deoxyribonucleic acid (DNA) methylation (for example in uncontrolled diabetes) was shown to affect the osteogenic differentiation capacity of PDLSCs, which was rescued in vitro by applying a DNA methyltransferase inhibitor (43). Such techniques gender optimism that a clinically useful strategy will be available to regenerate periodontal defects in the near future.
Regenerating resorbed roots
Orthodontically-induced inflammatory root resorption (OIIRR) is the most common sequela of orthodontic treatment (44, 45). The occurrence of OIIRR depends on biological host factors, genetic predisposition, and mechanical force factors (46, 47). Adults are at higher risk of OIIRR than their adolescent counterparts due to the adult PDL being mostly quiescent (8). Excessive forces damage and expose the root surfaces which are resorbed by osteoclast attachment and activity in a pro-inflammatory environment (48, 49). Physiologically, locally resident stem cells are integral in the normal maintenance and repair of dentine and cementum in the root. Stem and precursor cells in the PDL are capable of differentiating into the cementoblastic cells needed to repair resorption lacunae and are crucial for periodontal homeostasis and maintaining the cementum-PDL interface (20, 38).
Future therapies may seek to promote PDLSC activity to counteract and repair root resorption during OTM. So far, experiments in animal models have demonstrated beneficial effects of transferring ASCs into the PDL of teeth subjected to orthodontic forces: a significant protective effect against OIIRR was found in rats (49), suggesting that the transferred MSCs not only differentiated into osteoblasts or cementoblasts themselves, but also activated endogenous PDLSCs to contribute to repair. A variety of MSC types [including from bone marrow, PDL, dental follicles (DFCs) and dental pulp] have been successfully differentiated into cementoblast-like cells and increased cementum formation (50). Odontoblasts have been successfully derived from a range of dental-derived MSCs (51, 52); DFCs show superior dentine regeneration capacity to PDLSCs, producing more complete dentine tissues and capable of growing root and periodontal tissue in pre-clinical in vitro and in vivo studies (53, 54). Stem cells from human exfoliated deciduous teeth (SHEDs) have been shown to possess similar odontogenic differentiation capacity to DFCs in in vivo experiments, with the additional advantage of simple harvesting methods; consequently, much research interest is focused on exploring SHEDs' potential for bio-root regeneration (55, 56).
Stem cells can be delivered to root defects via transplantation on scaffolds, in cellular pellets, or directly injected. Materials such as calcium aluminate can be used to enrich porous scaffolds, increasing mineral matrix deposition and enhancing the odontogenic potential of seeded stem cells (57). Additionally, research may exploit cytokines and other signalling molecules to improve tissue formation: mechanical force activates IL-11 to stimulate osteoblastic and cementoblast-specific markers in MSCs, indicating their role in inducing MSC differentiation into osteoblasts or cementoblasts during tooth movement (49). Further elucidation of naturally occurring intercellular and inter-tissue communication will allow finer control of the dental tissue repair and regeneration.
Regenerating vital dental pulp
Orthodontic forces produce cellular changes not only in the periodontal structures but also in the dental pulp: prolonged force application may result in vascular stasis and eventually necrosis (58, 59). Data on the prevalence of orthodontic-related pulp damage is limited, but reports estimate that a range of 1%–14% of teeth in the adolescent patient population devitalise (60). Adults are likely to be at higher risk of devitalisation due to having more mature roots and narrower apical foramina (9, 59). Current endodontic therapy entails removal of all necrotic pulp tissue and replacement with a synthetic material; this is commonly done with good outcomes, however, endodontically treated teeth become weakened and more brittle (60), and subsequent cuspal coverage restorations necessitate removal of more coronal tooth tissue. The ability to restore the dental pulp to its original vitality, form and function could avoid these problems and revolutionise endodontics.
Regeneration of vital dental pulp is an area of great research interest, and progress in this field of regenerative endodontics is encouraging. As discussed in earlier sections of this review, several types of MSCs derived from teeth and other organs can differentiate into odontoblasts and produce dentine-like material (7). This capacity can be enhanced by modulating the biochemical environment; for example, porous 3D scaffolds which mimic the tubular structure of dentine induce differentiation of human dental pulp stem cells (DPSCs) and bone marrow MSCs (BMMSCs) into odontoblasts (61). Injectable scaffolds are advantageous over hard polymer scaffolds because they can be inserted into the pulp chamber of an intact tooth through a conservative access point. SHEDs have been seeded onto such injectable scaffolds of self-assembly peptides or collagen and injected into root canals, successfully forming pulp-like tissue and functional odontoblast-like cells (62). Some self-assembly peptide hydrogels are capable of forming nano-fibre meshes which are conducive to the growth and differentiation of DPSCs, and relatively economical (58).
Re-vascularisation is essential in providing a functional dental pulp tissue capable of limited dentine repair, since most progenitor cells are recruited from the blood (63). The pulp chamber, encased in a rigid structure, presents a unique challenge since blood supply is provided solely through the narrow apical foramen. Various techniques have been explored, including impregnating scaffolds with pro-angiogenic factors such as vascular endothelial growth factor (VEGF) (58, 64). Endothelial cells can be transplanted in scaffolds to induce vascular differentiation of stem cells due to their intrinsic vasculogenic potential; however, this has had limited success due to their low proliferative capacity (65, 66). Alternatively, DPSCs can induce angiogenesis in other cell types, as well as differentiate into vascular endothelial cells themselves (67). Extracellular vesicles produced by PDLSCs were recently co-cultured with endothelial cells and DPSCs in injectable fibrin hydrogels, and they promoted angiogenesis, cell growth and cell migration, thus demonstrating potential for pulp regeneration (68).
Regenerating alveolar ridges
The incidence of tooth loss increases with age, and subsequent bone remodelling results in reduction in height and width of the alveolar ridge (69). As the ridge resorbs, the bone envelope into which teeth can be moved orthodontically is reduced (70). Adults are at no higher risk of orthodontic-induced bone loss than younger patients, contingent on the absence of plaque and application of appropriate orthodontic forces (71). However, restoring the dimensions of alveolar ridges with pre-existing resorption is particularly helpful in adults in order to allow tooth movement into that edentulous space.
Most current strategies for alveolar augmentation involve bone grafting, with no definitive conclusion as to which graft material is superior or most suitable for which clinical situation. Various materials have been used including autogenous grafts, xenografts, and synthetic or alloplastic bone substitutes (72). These may be performed alone or in conjunction with GTR or corticotomy (73, 74). The gold standard is an autogenous bone graft, but limitations include pain and morbidity of the donor site, variable quality and quantity of the grafted bone, and limitation in shape and size of the graft (63).
Tissue engineering in the context of ridge augmentation bypasses these concerns by providing a minimally invasive method of replacing lost bone: it endeavours to utilise and optimise the properties of autologous osteogenic cells, osteoconductive scaffolds, and osteoinductive molecular signals. Studies employing ex vivo expanded stem or progenitor cells and whole-tissue fractions have not yet concluded the most superior method (69), but each offers advantages over current practice. Many different types of MSCs have been evaluated for their osteogenic potential in animal models and some human studies, including BMMSCs, ASCs and dental-derived MSCs. Each demonstrates positive osteogenic capacity, but with variable efficacy and potency (63).
BMMSCs have been extensively studied in repairing craniofacial bony defects in animal models and more recently in human trials, which showed clinical and histological success in regenerating resorbed mandibular ridges via a less invasive method than conventional bone grafting (75–78). Perivascular stem cells (PSCs), which are easily acquired from well-vascularised tissues and can be rapidly isolated and purified (79), were also shown to induce bone formation in mouse calvarial defects (80, 81). Of the dental-derived MSCs, PDLSCs and gingivae-derived MSCs were tested in preclinical studies and successfully regenerated calvarial defects (82, 83), although the PDLSC osteogenic capacity is less than that of BMMSCs (84). DPSCs are also able to regenerate orofacial bony defects in animals and humans (85, 86) and in fact compares favourably to BMMSCs and PSCs with regards to osteogenic potential (87). SHEDs are also capable of inducing angiogenesis and growing mature bone in mice (88) and have superior proliferative and osteogenic ability to DPSCs and BMMSCs (89, 90).
As the ECM is critical in directing osteogenic lineage specification in utero, scaffolds aim to mimic the natural bone ECM. Regeneration of bone defects by MSCs is greatly enhanced when combined with scaffolds (72), and extensive research continues to discover the optimal conditions for osteogenesis. A wide variety of biomaterials have been tried and tested, including β-tricalcium phosphate, hydroxyapatite-tricalcium phosphate, collagen, bovine freeze-dried tooth-derived protein, and decellularized dental pulp matrix (85, 86, 91, 92). Advances in 3D technology have enabled creation of electrospun nanofibrous scaffolds which improve osteogenic differentiation of BMMSCs (93), and 3D-printed titanium alloy scaffolds for MSC seeding and bone formation (94). Supplementary factors which enrich the osteogenic extracellular and cellular environment include aspirin, which modulates gene expression and inflammation to increase the proliferative and osteogenic potential of PDLSCs (95, 96). Pulsed ultrasound stimulation can also upregulate osteogenic differentiation of PDLSCs (97), as can encapsulation in gelatin methacrylate hydrogels (98), and treatment with gold nanoparticles (99).
Improving dentofacial orthopaedic surgical outcomes
Dentofacial orthopaedics aims to guide the growth and balance the final positions of the facial bones and jaws. A key difference between adults and children or adolescents is their growth potential; on average, the maxilla and mandible achieve maturity at approximately 16–17 years of age. Although subtle allometric changes continue throughout adulthood, the growth rate is far less than in adolescence (100). The teenage growth spurt is often exploited by orthodontists via functional appliances and dentofacial orthopaedics; although the true long-term skeletal impact of functional appliances is debated (101), it is accepted that they are not clinically effective once growth is diminished in adulthood. Therefore, adults presenting with such skeletal discrepancies have the sole option of surgery.
Conventional orthognathic surgeries entail osteotomy and repositioning of the maxilla, mandible, or both. These surgeries have a long and largely successful history but are not without risks: patients may experience severe haemorrhage, neurological damage, and non-union of the separated bone segments, and clinical limits must be imposed on the distance that the jaws can be displaced (102, 103). Bone grafting can improve ossification in the osteotomy site but is hindered by the limitations of harvesting autogenous bone and developing predictable, effective biomaterials (104). Distraction osteogenesis (DO) is another surgical approach which lengthens bone by separation of the fragments to promote gradual new bone formation: tension exerted on the bone fragments induces recruitment of MSCs and osteogenic progenitors, which then form a neocallus in the distraction gap (105). Patients must wear external fixators for an average of 12 months, risking infection and osteomyelitis of the jaws (106); thus, efforts to reduce treatment time are valuable.
Surgical outcomes may be improved by facilitating wound healing, both from a histological and clinical perspective. Stem cell therapy can be extremely useful in this context, as MSCs are effective in stimulating angiogenesis, healing and tissue repair (107, 108). Skin wounds showed significantly faster closure, re-epithelialisation and angiogenesis when engrafted with BMMSCs (109). As discussed earlier, the osteogenic ability of various types of MSCs and the potential to add scaffolds and growth factors would be instrumental in enhancing ossification at the orthognathic surgical site, and therefore accelerating patient recovery (110).
New bone formation in DO can also be facilitated by stem cell application (Figure 3) (111). Several animal studies show that BMMSCs upregulate osteogenesis and neocallus consolidation, therefore not only shortening treatment time but also improving the quality of the bone formed (112–114). Other sources of MSCs have also been successfully used to aid DO, including ASCs and SHEDs (115). As well as differentiating into osteoblastic cells themselves, MSCs release important pro-osteogenic factors which signal to and affect activity of adjacent cells. Adding BMMSC-conditioned media to mice undergoing rapid DO was shown to accelerate healing by promoting recruitment of bone marrow stromal cells and endothelial cells, inducing osteoblastic differentiation and angiogenesis, and inhibiting inflammation and apoptosis (106). Neoangiogenesis is essential as ischaemia usually limits healing and treatment time. Endothelial progenitor cells from blood vessels contribute to increased blood flow in the mandible during DO, and therefore protect from ischaemia (116). MSCs can also differentiate into neuronal and vascular endothelial progenitors, but the challenge is expanding the appropriate populations and simultaneously coordinating control of osteoblastic, neuronal and vascular tissue lineage specification.
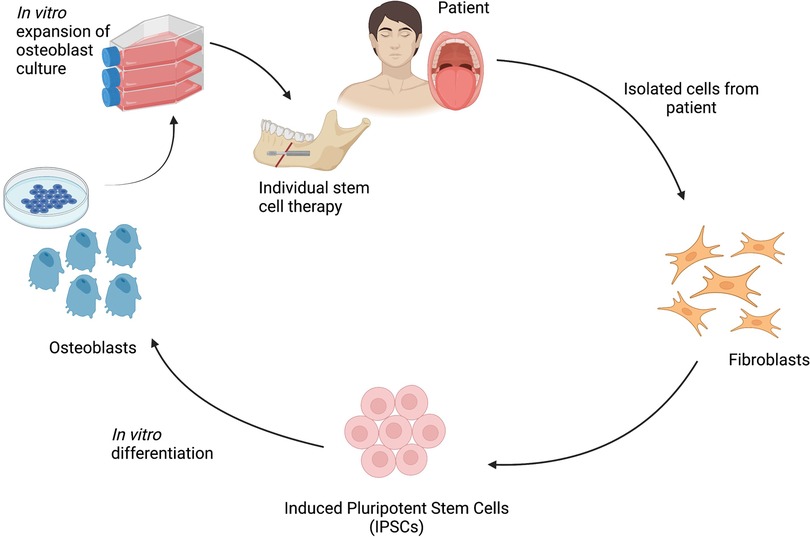
Figure 3 Schematic representation of the process of isolation and programming of iPSCs for bone regeneration in dentofacial orthopaedic surgery. Figure created with BioRender.com.
Discussion
There are several clinical challenges in adult orthodontics that may be improved by harnessing regenerative approaches and techniques. Limited cell turnover and rate of OTM, loss of periodontal support, OIIRR, iatrogenic pulp devitalisation, alveolar ridge resorption, and surgical orthodontic treatment outcomes have potential to benefit from applications of current and future approaches based on biological repair and regeneration.
In particular, directing research efforts down the route of improving efficacy, efficiency, and safety of MSC acquisition and differentiation could give valuable results for clinical therapies. Broadening and deepening our understanding of the underlying mechanisms that drive the process of repair will enable more predictable ways of stimulating biological repair and regeneration. However, barriers to translation of regenerative approaches and stem cell-based therapies are high due to practical and ethical issues, and these must be addressed before considering clinical use. Synthetic biomaterials used to generate biomimetic scaffolds are more straightforward in their translation, but their application may be limited, and cellular factors and conditions should first be optimised.
Most regenerative techniques are still being developed in pre-clinical stages, and it is important to note the differences in dental anatomy, cellular behaviour, and progression of diseases in the animals used when modelling human processes. There is a need to establish protocols for reliable stem cell expansion and differentiation, ascertain compatibility with appropriate biomaterials, and assess the quality of the tissues produced when tissue engineering techniques are being translated into therapeutic solutions. These important steps must be taken before such techniques can be translated into clinical applications for adult orthodontic patients and the practice of regenerative therapies becomes reality.
Author contributions
CL conducted all aspects of the review and writing of the manuscript. AAV and PS conducted review of and amendments to the manuscript. All authors contributed to the article and approved the submitted version.
Funding
Research from Paul Sharpe's laboratory was funded in part by Czech Science 760 Foundation, project 21-21409S.
Conflict of interest
The authors declare that the research was conducted in the absence of any commercial or financial relationships that could be construed as a potential conflict of interest.
Publisher's note
All claims expressed in this article are solely those of the authors and do not necessarily represent those of their affiliated organizations, or those of the publisher, the editors and the reviewers. Any product that may be evaluated in this article, or claim that may be made by its manufacturer, is not guaranteed or endorsed by the publisher.
References
1. Khan RS, Horrocks EN. A study of adult orthodontic patients and their treatment. Br J Orthod. (1991) 18:183–94. doi: 10.1179/bjo.18.3.183
2. Nattrass C, Sandy JR. Adult orthodontics–a review. Br J Orthod. (1995) 22:331–7. doi: 10.1179/bjo.22.4.331
3. McMorrow SM, Millett DT. Adult orthodontics in the republic of Ireland: specialist orthodontists’ opinions. J Orthod. (2017) 44:277–86. doi: 10.1080/14653125.2017.1403144
4. Scott P, Fleming P, DiBiase A. An update in adult orthodontics. Dent Update. (2007) 34:427–8; 431–24, 436 passim. doi: 10.12968/denu.2007.34.7.427
5. Cedro MK, Moles DR, Hodges SJ. Adult orthodontics–who's doing what? J Orthod. (2010) 37:107–17. doi: 10.1179/14653121042966
6. Thilander B. Indications for orthodontic treatment in adults. Eur J Orthod. (1979) 1(4):227–41. doi: 10.1093/ejo/1.4.227
7. Matichescu A, Ardelean LC, Rusu LC, Craciun D, Bratu EA, Babucea M, et al. Advanced biomaterials and techniques for oral tissue engineering and regeneration - A review. Materials (Basel). (2020) 13(22):5303. doi: 10.3390/ma13225303
8. Reitan K. Initial tissue behavior during apical root resorption. Angle Orthod. (1974) 44(1):68–82. doi: 10.1043/0003-3219(1974)044%3C0068:ITBDAR%3E2.0.CO;2
9. Hamersky PA, Weimer AD, Taintor JF. The effect of orthodontic force application on the pulpal tissue respiration rate in the human premolar. Am J Orthod. (1980) 77(4):368–78. doi: 10.1016/0002-9416(80)90103-7
10. Amuk N G, Kurt G, Karsli E, Ozcan S, Acar MB, Amuk M, et al. Effects of mesenchymal stem cell transfer on orthodontically induced root resorption and orthodontic tooth movement during orthodontic arch expansion protocols: an experimental study in rats. Eur J Orthod. (2020) 42(3):305–16. doi: 10.1093/ejo/cjz035
11. Li Y, Jacox LA, Little SH, Ko CC. Orthodontic tooth movement: the biology and clinical implications. Kaohsiung J Med Sci. (2018) 34(4):207–14. doi: 10.1016/j.kjms.2018.01.007
12. Krishnan V, Davidovitch Z. Cellular, molecular, and tissue level reactions to orthodontic force. Am J Orthod Dentofac Orthop. (2006) 129(4):469.e1–32. doi: 10.1016/j.ajodo.2005.10.007
13. Baumrind S. A reconsideration of the property of the pressure tension hypothesis. Am J Orthod. (1969) 55:12–22. doi: 10.1016/S0002-9416(69)90170-5
14. Shroff B. Accelerated orthodontic tooth movement: recommendations for clinical practice. Semin Orthod. (2020) 26(3):157–61. doi: 10.1053/j.sodo.2020.06.013
15. Kau CH, Kantarci A, Shaughnessy T, Vachiramon A, Santiwong P, de la Fuente A, et al. Photobiomodulation accelerates orthodontic alignment in the early phase of treatment. Prog Orthod. (2013) 14:30. doi: 10.1186/2196-1042-14-30
16. Frost HM. The regional acceleratory phenomenon: a review. Henry Ford Hosp Med J. (1983) 31(1):3–9.6345475
17. Zainal Ariffin SH, Yamamoto Z, Zainol Abidin IZ, Megat Abdul Wahab R, Zainal Ariffin Z. Cellular and molecular changes in orthodontic tooth movement. Sci World J. (2011) 11:1788–803. doi: 10.1100/2011/761768
18. Feng L, Yang R, Liu D, Wang X, Song Y, Cao H, et al. PDL progenitor-mediated PDL recovery contributes to orthodontic relapse. J Dent Res. (2016) 95:1049–56. doi: 10.1177/0022034516648604
19. Venkataiah VS, Handa K, Njuguna MM, Hasegawa T, Maruyama K, Nemotoet E, et al. Periodontal regeneration by allogeneic transplantation of adipose tissue derived multi-lineage progenitor stem cells in vivo. Sci Rep. (2019) 9:921. doi: 10.1038/s41598-018-37528-0
20. Huang H, Yang R, Zhou Y. Mechanobiology of periodontal ligament stem cells in orthodontic tooth movement. Stem Cells Int. (2018) 11:1–7. doi: 10.1155/2018/6531216
21. Zhang L, Liu W, Zhao J, Ma X, Shen L, Zhang Y, et al. Mechanical stress regulates osteogenic differentiation and RANKL/OPG ratio in periodontal ligament stem cells by the Wnt/β-catenin pathway. Biochim Biophys Acta. (2016) 1860(10):2211–9. doi: 10.1016/j.bbagen.2016.05.003
22. Liu J, Li Q, Liu S, Gao J, Qin W, Song Y, et al. Periodontal ligament stem cells in the periodontitis microenvironment are sensitive to static mechanical strain. Stem Cells Int. (2017) 3:1–13. doi: 10.1155/2017/1380851
23. Nimeri G, Kau CH, Abou-Kheir NS, Corona R. Acceleration of tooth movement during orthodontic treatment–a frontier in orthodontics. Prog Orthod. (2013) 14:42. doi: 10.1186/2196-1042-14-42
24. Proffit WR, Fields HM, Sarver DM. Contemporary orthodontics. 4th ed. St. Louis: CV Mosby (2007). 768 p.
25. Ericsson I, Thilander B. Orthodontic forces and recurrence of periodontal disease. An experimental study in the dog. Am J Orthod. (1978) 74(1):41–50. doi: 10.1016/0002-9416(78)90044-1
26. Feu D. Orthodontic treatment of periodontal patients: challenges and solutions, from planning to retention. Dental Press J Orthod. (2020) 25(6):79–116. doi: 10.1590/2177-6709.25.6.079-116.sar
27. Nuñez J, Sanz-Blasco S, Vignoletti F, Muñoz F, Arzate H, Villalobos C, et al. Periodontal regeneration following implantation of cementum and periodontal ligament-derived cells. J Periodontal Res. (2012) 47(1):33–44. doi: 10.1111/j.1600-0765.2011.01402.x
28. Gkantidis N, Christou P, Topouzelis N. The orthodontic-periodontic interrelationship in integrated treatment challenges: a systematic review. J Oral Rehabil. (2010) 37(5):377–90. doi: 10.1111/j.1365-2842.2010.02068.x
29. Guo Y, Weihua G, Jie C, Guoqing C, Weidong T, Ding B. Are hertwig's epithelial root sheath cells necessary for periodontal formation by dental follicle cells? Arch Oral Biol. (2018) 94:1–9. doi: 10.1016/j.archoralbio.2018.06.014
30. Tavelli L, McGuire MK, Zucchelli G, Rasperini G, Feinberg SE, Wang HL, et al. Biologics-based regenerative technologies for periodontal soft tissue engineering. J Periodontol. (2020) 91(2):147–54. doi: 10.1002/JPER.19-0352
31. Chen FM, Jin Y. Periodontal tissue engineering and regeneration: current approaches and expanding opportunities. Tissue Eng Part B Rev. (2010) 16(2):219–55. doi: 10.1089/ten.teb.2009.0562
32. Yamato M, Okano T. Cell sheet engineering. In: Yamato M, Okano T, editors. Materials today. UK: Elsevier Ltd (2004). Vol. 7. p. 42–7.
33. Grimm WD, Dannan A, Becher S, Gassmann G, Arnold W, Varga G, et al. The ability of human periodontium-derived stem cells to regenerate periodontal tissues: a preliminary in vivo investigation. Int J Periodontics Restorative Dent. (2011) 31:e94–101.22140674
34. Liu J, Zhao Z, Ruan J, Weir MD, Ma T, Ren K, et al. Stem cells in the periodontal ligament differentiated into osteogenic, fibrogenic and cementogenic lineages for the regeneration of the periodontal complex. J Dent. (2020) 92:103259. doi: 10.1016/j.jdent.2019.103259
35. Yang H, Li J, Hu Y, Sun J, Guo W, Li H, et al. Treated dentin matrix particles combined with dental follicle cell sheet stimulate periodontal regeneration. Dent Mater. (2019) 35(9):1238–53. doi: 10.1016/j.dental.2019.05.016
36. Hasegawa M, Yamato M, Kikuchi A, Okano T, Ishikawa I. Human periodontal ligament cell sheets can regenerate periodontal ligament tissue in an athymic rat model. Tissue Eng. (2005) 11(3–4):469–78. doi: 10.1089/ten.2005.11.469
37. Iwata T, Yamato M, Washio K, Yoshida T, Tsumanuma Y, Yamada A, et al. Periodontal regeneration with autologous periodontal ligament-derived cell sheets - A safety and efficacy study in ten patients. Regen Ther. (2018) 9:38–44. doi: 10.1016/j.reth.2018.07.002
38. Seo BM, Miura M, Gronthos S, Bartold PM, Batouli S, Brahim J, et al. Investigation of multipotent postnatal stem cells from human periodontal ligament. Lancet (London, England). (2004) 364:149–55. doi: 10.1016/S0140-6736(04)16627-0
39. Yamamoto S, Masuda H, Shibukawa Y, Yamada S. Combination of bovine-derived xenografts and enamel matrix derivative in the treatment of intrabony periodontal defects in dogs. Int J Periodontics Restorative Dent. (2007) 27:471–9.17990444
40. Xiong X, Yang X, Dai H, Feng G, Zhang Y, Zhou J, et al. Extracellular matrix derived from human urine-derived stem cells enhances the expansion, adhesion, spreading, and differentiation of human periodontal ligament stem cells. Stem Cell Res Ther. (2019) 10(1):396. doi: 10.1186/s13287-019-1483-7
41. Li X, Zhang B, Wang H, Zhao X, Zhang Z, Ding G, et al. The effect of aging on the biological and immunological characteristics of periodontal ligament stem cells. Stem Cell Res Ther. (2020) 11(1):326. doi: 10.1186/s13287-020-01846-w
42. Yang ZH, Zhang XJ, Dang NN, Ma ZF, Xu L, Wu JJ, et al. Apical tooth germ cell-conditioned medium enhances the differentiation of periodontal ligament stem cells into cementum/periodontal ligament-like tissues. J Periodontal Res. (2009) 44(2):199–210. doi: 10.1111/j.1600-0765.2008.01106.x
43. Liu Z, Chen T, Sun W, Yuan Z, Yu M, Chen G, et al. DNA Demethylation rescues the impaired osteogenic differentiation ability of human periodontal ligament stem cells in high glucose. Sci Rep. (2016) 6:27447. doi: 10.1038/srep27447
44. McNab S, Battistutta D, Taverne A, Symons AL. External apical root resorption following orthodontic treatment. Angle Orthod. (2000) 70:227–32. doi: 10.1043/0003-3219(2000)070%3C0227:EARRFO%3E2.0.CO;2
45. Sameshima GT, Sinclair PM. Predicting and preventing root resorption: part II. Treatment factors. Am J Orthod Dentofacial Orthop. (2001) 119:511–5. doi: 10.1067/mod.2001.113410
46. Weltman B, Vig KW, Fields HW, Shankar S, Kaizar EE. Root resorption associated with orthodontic tooth movement: a systematic review. Am J Orthod Dentofac Orthop. (2010) 137:462–76. doi: 10.1016/j.ajodo.2009.06.021
47. Zahrowski J, Jeske A. Apical root resorption is associated with comprehensive orthodontic treatment but not clearly dependent on prior tooth characteristics or orthodontic techniques. J Am Dent Assoc. (2011) 142:66–8. doi: 10.14219/jada.archive.2011.0030
48. Trope M. Root resorption due to dental trauma. Endod Topics. (2002) 1:79–100. doi: 10.1034/j.1601-1546.2002.10106.x
49. Monnouchi S, Maeda H, Yuda A, Hamano S, Wada N, Tomokiyo A, et al. Mechanical induction of interleukin-11 regulates osteoblastic/cementoblastic differentiation of human periodontal ligament stem/progenitor cells. J Periodontal Res. (2015) 50(2):231–9. doi: 10.1111/jre.12200
50. Shinagawa-Ohama R, Mochizuki M, Tamaki Y, Suda N, Nakahara T. Heterogeneous human periodontal ligament-committed progenitor and stem cell populations exhibit a unique cementogenic property under in vitro and in vivo conditions. Stem Cells Dev. (2017) 26(9):632–45. doi: 10.1089/scd.2016.0330
51. Hakki SS, Kayis SA, Hakki EE, Bozkurt SB, Duruksu G, Unal ZS, et al. Comparison of mesenchymal stem cells isolated from pulp and periodontal ligament. J Periodontol. (2015) 86(2):283–91. doi: 10.1902/jop.2014.140257
52. Yang X, Ma Y, Guo W, Yang B, Tian W. Stem cells from human exfoliated deciduous teeth as an alternative cell source in bio-root regeneration. Theranostics. (2019) 9(9):2694–711. doi: 10.7150/thno.31801
53. Fuloria S, Jain A, Singh S, Hazarika I, Salile S, Fuloria NK. Regenerative potential of stem cells derived from human exfoliated deciduous (SHED) teeth during engineering of human body tissues. Curr Stem Cell Res Ther. (2021) 16(5):507–17. doi: 10.2174/1574888X16999201231213206
54. Bluteau G, Luder HU, De Bari C, Mitsiadis TA. Stem cells for tissue engineering. Eur Cells Mater. (2008) 16:1–9. doi: 10.22203/eCM.v016a01
55. Tian Y, Bai D, Guo W, Li J, Zeng J, Yang L, et al. Comparison of human dental follicle cells and human periodontal ligament cells for dentin tissue regeneration. Regen Med. (2015) 10(4):461–79. doi: 10.2217/rme.15.21
56. Han C, Yang Z, Zhou W, Jin F, Song Y, Wang Y, et al. Periapical follicle stem cell: a promising candidate for cementum/periodontal ligament regeneration and bio-root engineering. Stem Cells Dev. (2010) 19(9):1405–15. doi: 10.1089/scd.2009.0277
57. Soares DG, Rosseto HL, Scheffel DS, Basso FG, Huck C, Hebling J, et al. Odontogenic differentiation potential of human dental pulp cells cultured on a calcium-aluminate enriched chitosan-collagen scaffold. Clin Oral Investig. (2017) 21(9):2827–39. doi: 10.1007/s00784-017-2085-3
58. Demarco FF, Conde MC, Cavalcanti BN, Casagrande L, Sakai VT, Nör JE. Dental pulp tissue engineering. Braz Dent J. (2011) 22(1):3–13. doi: 10.1590/S0103-64402011000100001
59. Javed F, Al-Kheraif AA, Romanos EB, Romanos GE. Influence of orthodontic forces on human dental pulp: a systematic review. Arch Oral Biol. (2015) 60(2):347–56. doi: 10.1016/j.archoralbio.2014.11.011
60. Faria AC, Rodrigues RC, de Almeida Antunes RP, de Mattos Mda G, Ribeiro RF. Endodontically treated teeth: characteristics and considerations to restore them. J Prosthodont Res. (2011) 55(2):69–74. doi: 10.1016/j.jpor.2010.07.003
61. Miyashita S, Ahmed NE, Murakami M, Iohara K, Yamamoto T, Horibe H, et al. Mechanical forces induce odontoblastic differentiation of mesenchymal stem cells on three-dimensional biomimetic scaffolds. J Tissue Eng Regen Med. (2017) 11(2):434–46. doi: 10.1002/term.1928
62. Rosa V. Stem cells from exfoliated deciduous teeth and self-assembling nanofiber and recombinant human collagen I scaffolds allows for the engineering of a functional dental pulp [doctoral thesis]. São Paulo: Faculdade de Odontologia da Universidade de São Paulo, Universidade de São Paulo (2010).
63. Asatrian G, Pham D, Hardy WR, James AW, Peault B. Stem cell technology for bone regeneration: current status and potential applications. Stem Cells Cloning. (2015) 8:39–48. doi: 10.2147/SCCAA.S48423
64. Sakai VT, Zhang Z, Dong Z, Neiva KG, Machado MA, Shi S, et al. SHED Differentiate into functional odontoblasts and endothelium. J Dent Res. (2010) 89:791–6. doi: 10.1177/0022034510368647
65. Cordeiro MM, Dong Z, Kaneko T, Zhang Z, Miyazawa M, Shi S, et al. Dental pulp tissue engineering with stem cells from exfoliated deciduous teeth. J Endod. (2008) 34:962–9. doi: 10.1016/j.joen.2008.04.009
66. Melero-Martin JM, Khan ZA, Picard A, Wu X, Paruchuri S, Bischoff J. In vivo vasculogenic potential of human blood-derived endothelial progenitor cells. Blood. (2007) 109:4761–8. doi: 10.1182/blood-2006-12-062471
67. d’Aquino R, Graziano A, Sampaolesi M, Laino G, Pirozzi G, De Rosa A, et al. Human postnatal dental pulp cells co-differentiate into osteoblasts and endotheliocytes: a pivotal synergy leading to adult bone tissue formation. Cell Death Differ. (2007) 14:1162–71. doi: 10.1038/sj.cdd.4402121
68. Zhang S, Thiebes AL, Kreimendahl F, Ruetten S, Buhl EM, Wolf M, et al. Extracellular vesicles-loaded fibrin gel supports rapid neovascularization for dental pulp regeneration. Int J Mol Sci. (2020) 21(12):4226. doi: 10.3390/ijms21124226
69. Shanbhag S, Suliman S, Pandis N, Stavropoulos A, Sanz M, Mustafa K. Cell therapy for orofacial bone regeneration: a systematic review and meta-analysis. J Clin Periodontol. (2019) 46(Suppl 21):162–82. doi: 10.1111/jcpe.13049
70. Sakkas A, Wilde F, Heufelder M, Winter K, Schramm A. Autogenous bone grafts in oral implantology—is it still a “gold standard”? A consecutive review of 279 patients with 456 clinical procedures. Int J Implant Dent. (2017) 3:23. doi: 10.1186/s40729-017-0084-4
71. Eliasson L, Hugoson A, Kurol J, Siwe H. The effects of orthodontic treatment of periodontal tissues in patients with reduced periodontal support. Eur J Orthod. (1982) 4:1–9. doi: 10.1093/ejo/4.1.1
72. Shanbhag S, Pandis N, Mustafa K, Nyengaard JR, Stavropoulos A. Alveolar bone tissue engineering in critical-size defects of experimental animal models: a systematic review and meta-analysis. J Tissue Eng Regen Med. (2017) 11(10):2935–49. doi: 10.1002/term.2198
73. Wilcko MT, Wilcko WM, Bissada NF. An evidence-based analysis of periodontally accelerated orthodontic and osteogenic techniques: a synthesis of scientific perspectives. Semin Orthod. (2008) 14(4):305–16. doi: 10.1053/j.sodo.2008.07.007
74. Lee K-B, Lee D-Y, Ahn H-W, Kim S-H, Kim E-C, Roitman I. Tooth movement out of the bony wall using augmented corticotomy with nonautogenous graft materials for bone regeneration. BioMed Res Int. (2014) 2014:347508. doi: 10.1155/2014/347508
75. Zaky SH, Cancedda R. Engineering craniofacial structures: facing the challenge. J Dent Res. (2009) 88:1077–91. doi: 10.1177/0022034509349926
76. Zhang L, Wang P, Mei S, Li C, Cai C, Ding Y. In vivo alveolar bone regeneration by bone marrow stem cells/fibrin glue composition. Arch Oral Biol. (2012) 57:238–44. doi: 10.1016/j.archoralbio.2011.08.025
77. Gjerde C, Mustafa K, Hellem S, Rojewski M, Gjengedal H, Yassin MA, et al. Cell therapy induced regeneration of severely atrophied mandibular bone in a clinical trial. Stem Cell Res. (2018) 9:213. doi: 10.1186/s13287-018-0951-9
78. Kaigler D, Pagni G, Park CH, Braun TM, Holman LA, Yi E, et al. Stem cell therapy for craniofacial bone regeneration: a randomized, controlled feasibility trial. Cell Transplant. (2013) 22:767–77. doi: 10.3727/096368912X652968
79. Corselli M, Crisan M, Murray IR, West CC, Scholes J, Codrea F, et al. Identification of perivascular mesenchymal stromal/stem cells by flow cytometry. Cytometry A. (2013) 83(8):714–20. doi: 10.1002/cyto.a.22313
80. Crisan M, Yap S, Casteilla L, Chen CW, Corselli M, Park TS, et al. A perivascular origin for mesenchymal stem cells in multiple human organs. Cell Stem Cell. (2008) 3(3):301–13. doi: 10.1016/j.stem.2008.07.003
81. James AW, Zara JN, Corselli M, Askarinam A, Zhou AM, Hourfar A, et al. An abundant perivascular source of stem cells for bone tissue engineering. Stem Cells Transl Med. (2012) 1(9):673–84. doi: 10.5966/sctm.2012-0053
82. Moshaverinia A, Chen C, Xu X, Akiyama K, Ansari S, Zadeh HH, et al. Bone regeneration potential of stem cells derived from periodontal ligament or gingival tissue sources encapsulated in RGD-modified alginate scaffold. Tissue Eng Part A. (2014) 20(3–4):611–21. doi: 10.1089/ten.TEA.2013.0229
83. Menicanin D, Mrozik KM, Wada N, Marino V, Shi S, Bartold PM, et al. Periodontal-ligament-derived stem cells exhibit the capacity for long-term survival, self-renewal, and regeneration of multiple tissue types in vivo. Stem Cells Dev. (2014) 23(9):1001–11. doi: 10.1089/scd.2013.0490
84. Kim SH, Kim KH, Seo BM, Koo KT, Kim TI, Seol YJ, et al. Alveolar bone regeneration by transplantation of periodontal ligament stem cells and bone marrow stem cells in a canine peri-implant defect model: a pilot study. J Periodontol. (2009) 80(11):1815–23. doi: 10.1902/jop.2009.090249
85. Zheng Y, Liu Y, Zhang CM, Zhang HY, Li WH, Shi S, et al. Stem cells from deciduous tooth repair mandibular defect in swine. J Dent Res. (2009) 88(3):249–54. doi: 10.1177/0022034509333804
86. d'Aquino R, De Rosa A, Lanza V, Tirino V, Laino L, Graziano A, et al. Human mandible bone defect repair by the grafting of dental pulp stem/progenitor cells and collagen sponge biocomplexes. Eur Cell Mater. (2009) 18:75–83. doi: 10.22203/ecm.v018a07
87. Ito K, Yamada Y, Nakamura S, Ueda M. Osteogenic potential of effective bone engineering using dental pulp stem cells, bone marrow stem cells, and periosteal cells for osseointegration of dental implants. Int J Oral Maxillofac Implants. (2011) 26:947–54.22010075
88. Hiraki T, Kunimatsu R, Nakajima K, Abe T, Yamada S, Rikitake K, et al. Stem cell-derived conditioned media from human exfoliated deciduous teeth promote bone regeneration. Oral Dis. (2020) 26(2):381–90. doi: 10.1111/odi.13244
89. Nakajima K, Kunimatsu R, Ando K, Ando T, Hayashi Y, Kihara T, et al. Comparison of the bone regeneration ability between stem cells from human exfoliated deciduous teeth, human dental pulp stem cells and human bone marrow mesenchymal stem cells. Biochem Biophys Res Commun. (2018) 497(3):876–82. doi: 10.1016/j.bbrc.2018.02.156
90. Kunimatsu R, Nakajima K, Awada T, Tsuka Y, Abe T, Ando K, et al. Comparative characterization of stem cells from human exfoliated deciduous teeth, dental pulp, and bone marrow-derived mesenchymal stem cells. Biochem Biophys Res Commun. (2018) 501(1):193–8. doi: 10.1016/j.bbrc.2018.04.213
91. Sari DS, Maduratna E, Ferdiansyah, Latief FDE, Satuman, Nugraha AP, et al. Osteogenic differentiation and biocompatibility of bovine teeth scaffold with rat adipose-derived mesenchymal stem cells. Eur J Dent. (2019) 13(2):206–12. doi: 10.1055/s-0039-1694305
92. Lee DJ, Miguez P, Kwon J, Daniel R, Padilla R, Min S, et al. Decellularized pulp matrix as scaffold for mesenchymal stem cell mediated bone regeneration. J Tissue Eng. (2020) 11:2041731420981672. doi: 10.1177/2041731420981672
93. Ye K, Liu D, Kuang H, Cai J, Chen W, Sun B, et al. Three-dimensional electrospun nanofibrous scaffolds displaying bone morphogenetic protein-2-derived peptides for the promotion of osteogenic differentiation of stem cells and bone regeneration. J Colloid Interface Sci. (2019) 534:625–36. doi: 10.1016/j.jcis.2018.09.071
94. Kim JH, Kim MY, Knowles JC, Choi S, Kang H, Park SH, et al. Mechanophysical and biological properties of a 3D-printed titanium alloy for dental applications. Dent Mater. (2020) 36(7):945–58. doi: 10.1016/j.dental.2020.04.027
95. Abd Rahman F, Mohd Ali J, Abdullah M, Abu Kasim NH, Musa S. Aspirin enhances osteogenic potential of periodontal ligament stem cells (PDLSCs) and modulates the expression profile of growth factor-associated genes in PDLSCs. J Periodontol. (2016) 87(7):837–47. doi: 10.1902/jop.2016.150610
96. Zhang Y, Ding N, Zhang T, Sun Q, Han B, Yu T. A tetra-PEG hydrogel based aspirin sustained release system exerts beneficial effects on periodontal ligament stem cells mediated bone regeneration. Front Chem. (2019) 7:682. doi: 10.3389/fchem.2019.00682
97. Hu B, Zhang Y, Zhou J, Li J, Deng F, Wang Z, et al. Low-intensity pulsed ultrasound stimulation facilitates osteogenic differentiation of human periodontal ligament cells. PLoS One. (2014) 9(4):e95168. doi: 10.1371/journal.pone.0095168
98. Pan J, Deng J, Yu L, Wang Y, Zhang W, Han X, et al. Investigating the repair of alveolar bone defects by gelatin methacrylate hydrogels-encapsulated human periodontal ligament stem cells. J Mater Sci Mater Med. (2019) 31(1):3. doi: 10.1007/s10856-019-6333-8
99. Zhang Y, Wang P, Wang Y, Li J, Qiao D, Chen R, et al. Gold nanoparticles promote the bone regeneration of periodontal ligament stem cell sheets through activation of autophagy. Int J Nanomedicine. (2021) 16:61–73. doi: 10.2147/IJN.S282246
100. Ross A, Williams S. Craniofacial growth, maturation, and change. J Craniofac Surg. (2010) 21:458–61. doi: 10.1097/SCS.0b013e3181cfea34
101. DiBiase A, Cobourne M, Lee R. The use of functional appliances in contemporary orthodontic practice. Br Dent J. (2015) 218:123–8. doi: 10.1038/sj.bdj.2015.44
102. Zanettini LM, Fritscher GG, De Marco RG, de Oliveira Andriola F, Pagnoncelli RM. Bone substitutes in Le fort I osteotomy to promote bone union and skeletal stability. J Craniofac Surg. (2017) 28(2):492–5. doi: 10.1097/SCS.0000000000003349
103. Kim YK. Complications associated with orthognathic surgery. J Korean Assoc Oral Maxillofac Surg. (2017) 43(1):3–15. doi: 10.5125/jkaoms.2017.43.1.3
104. Haas Junior OL, da Silva Meirelles L, Scolari N, Emmel Becker O, Fernandes Santos Melo M, Belle de Oliveira R. Bone grafting with granular biomaterial in segmental maxillary osteotomy: a case report. Int J Surg Case Rep. (2016) 25:238–42. doi: 10.1016/j.ijscr.2016.06.034
105. Dheeraj K, Rastogi N, Singh M. Modern practice in orthognathic and reconstructive surgery- craniofacial distraction osteogenesis. J Public Health Epidemiol. (2011) 3:129–37. doi: 10.5897/JPHE.9000072
106. Ando Y, Matsubara K, Ishikawa J, Fujio M, Shohara R, Hibi H, et al. Stem cell-conditioned medium accelerates distraction osteogenesis through multiple regenerative mechanisms. Bone. (2014) 61:82–90. doi: 10.1016/j.bone.2013.12.029
107. Semedo P, Burgos-Silva M, Donizetti-Oliveira C, Camara NO. How do mesenchymal stem cells repair? In: Gholamrezanezhad A, editors. Stem cells in clinic and research. Brazil: InTech (2011). p. 83–104.
108. Caplan AI, Dennis JE. Mesenchymal stem cells as trophic mediators. J Cell Biochem. (2006) 98(5):1076–84. doi: 10.1002/jcb.20886
109. Wu Y, Chen L, Scott PG, Tredget EE. Mesenchymal stem cells enhance wound healing through differentiation and angiogenesis. Stem Cells. (2007) 25(10):2648–59. doi: 10.1634/stemcells.2007-0226
110. Oliver JD, Madhoun W, Graham EM, Hendrycks R, Renouard M, Hu MS. Stem cells regenerating the craniofacial Skeleton: current state-of-the-art and future directions. J Clin Med. (2020) 9(10):3307. doi: 10.3390/jcm9103307
111. Tee BC, Sun Z. Mandibular distraction osteogenesis assisted by cell-based tissue engineering: a systematic review. Orthod Craniofac Res. (2015) 18(Suppl 1(01)):39–49. doi: 10.1111/ocr.12087
112. Lai QG, Yaun KF, Xu X, Li DR, Li GJ, Wei FL, et al. Transcription factor osterix modified bone marrow mesenchymal stem cells enhance callus formation during distraction osteogenesis. Oral Surg Med Oral Pathol Oral Radiol Endod. (2011) 111:412–9. doi: 10.1016/j.tripleo.2010.05.012
113. El Hadidi YN, El Kassaby M, El Fatah Ahmed SA, Khamis NS. Effect of mesenchymal stem cell application on the distracted bone microstructure: an experimental study. J Oral Maxillofac Surg. (2016) 74(7):1463.e1–1463.e11. doi: 10.1016/j.joms.2016.03.021
114. El Kassaby M, El Kader KA, Khamis N, Al Hammoud A, Talb AB, El Hadidi YN. The effect of bone marrow mesenchymal stem cells application on distracted bone quality during rapid rate of distraction osteogenesis. Craniomaxillofac Trauma Reconstr. (2018) 11(3):192–8. doi: 10.1055/s-0037-1604070
115. Volponi AA, Pang Y, Sharpe PT. Stem cell-based biological tooth repair and regeneration. Trends Cell Biol. (2010) 20(12):715–22. doi: 10.1016/j.tcb.2010.09.012
Keywords: orthodontics, adult, stem cell, tissue engineering, regenerative dentistry
Citation: Liu C, Sharpe P and Volponi AA (2023) Applications of regenerative techniques in adult orthodontics. Front. Dent. Med 3:1100548. doi: 10.3389/fdmed.2022.1100548
Received: 16 November 2022; Accepted: 7 December 2022;
Published: 9 January 2023.
Edited by:
Quamarul Hassan, University of Alabama at Birmingham, United StatesReviewed by:
Marianna Bei, Harvard Medical School, United StatesRuili Yang, Peking University Hospital of Stomatology, China
Chung How Kau, University of Alabama at Birmingham, United States
© 2023 Liu, Sharpe and Volponi. This is an open-access article distributed under the terms of the Creative Commons Attribution License (CC BY). The use, distribution or reproduction in other forums is permitted, provided the original author(s) and the copyright owner(s) are credited and that the original publication in this journal is cited, in accordance with accepted academic practice. No use, distribution or reproduction is permitted which does not comply with these terms.
*Correspondence: Catherine Liu, Y2F0aGVyaW5lLjEubGl1QGtjbC5hYy51aw==
Specialty Section: This article was submitted to Regenerative Dentistry, a section of the journal Frontiers in Dental Medicine