- Division of Orthodontics and Dentofacial Deformities, Centre for Dental Education and Research, All India Institute of Medical Sciences, New Delhi, India.
The inception of laboratory work for the construction of a removable, tooth-moving appliance by sectioning teeth from the malocclusion model to align them with wax and achieve minor dental correction has evolved into a state of digital planning and appliance manufacturing for a wide spectrum of malocclusions. The groundbreaking technology of directly printing clear aligners has drawn the interest of clinicians and researchers in the orthodontic community today. This workflow enables the development of an in-house aligner system with complete control over desired aligner thickness, extent, and attachments; it is also technically resource-efficient with greater accuracy by excluding all the intermediate steps involved in the thermoforming method of manufacturing. This promising exploratory subject demands to be well-received with further research-based improvements. This article intends to summarize the digital orthodontic workflow and the literature evidence.
1. Introduction
In an era with attention directed toward enhancement technology, orthodontic intervention is closely linked to improving the quality of life (1). With a focus on providing a more acceptable dentofacial appearance and comfort “during the treatment”, not just considering “after the treatment” phase, esthetic-centered orthodontic appliances are being endorsed.
Clear aligners are tailored removable appliances intended for efficient comprehensive cosmetic orthodontic treatment of mild to moderate malocclusions (2). The appliance brings about incremental tooth movement by sequential sets of plastic aligner trays that are used and replaced at specific times throughout treatment. The transparent retainer worn by Zia Chisti after the completion of his conventional braces treatment was the brainchild of a global medical device company (3). In 1997, his team of Stanford University graduates launched a start-up in Silicon Valley called “Align Technology” that used Computer Aided Designing and Manufacturing (CAD/CAM). Over time, patient interest and the entry of a large number of companies have made it a thriving field in orthodontics (4).
As the primary biomechanical basis of the clear aligner force delivery relies on the shape-molding effect (5), the geometrical fit and material properties are among the major factors influencing the efficacy of the appliance. These in turn depend on the choice of the material and the method of manufacturing.
2. Methods of clear aligner manufacturing
Orthodontic clear aligners can be manufactured by (i) thermoforming on the 3D printed models, or (ii) direct 3D printing of aligners. The conventional thermoforming technique is indirect in nature (6). After treatment planning, sequential dental models representing the patient's treatment progress will be printed, and thermally manipulated transparent plastic sheets will be molded against each dental model by a pressure- or vacuum-forming machine for aligner production. The retrieved aligners will be trimmed and finished for patient delivery and insertion (7) (Figure 1). On the other hand, the direct 3D printing technique designs and fabricates the aligner directly using biocompatible clear resins without requiring a dental model (8) (Figure 2).
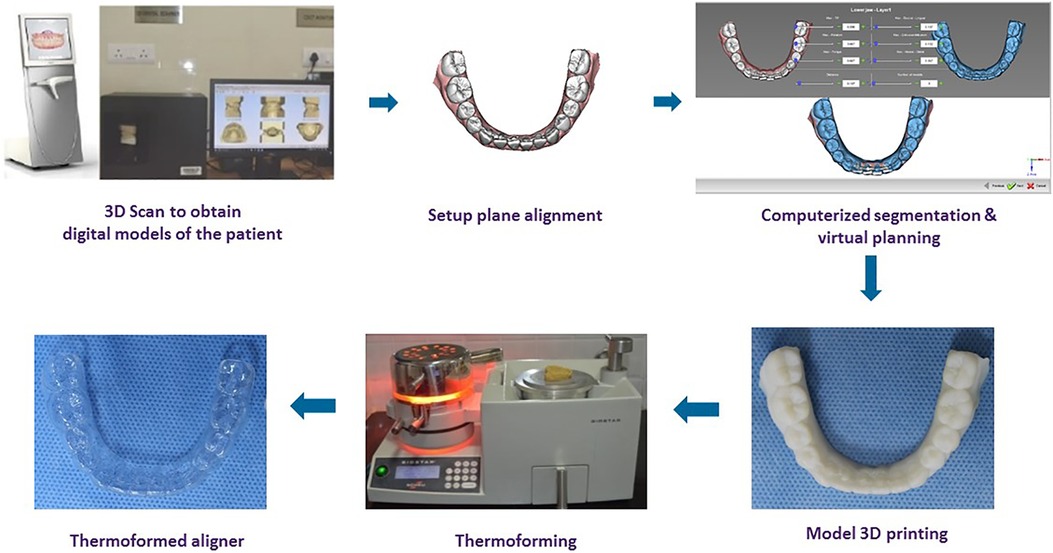
Figure 1. The key stages of laboratory procedure in the manufacturing of thermoformed clear aligner.
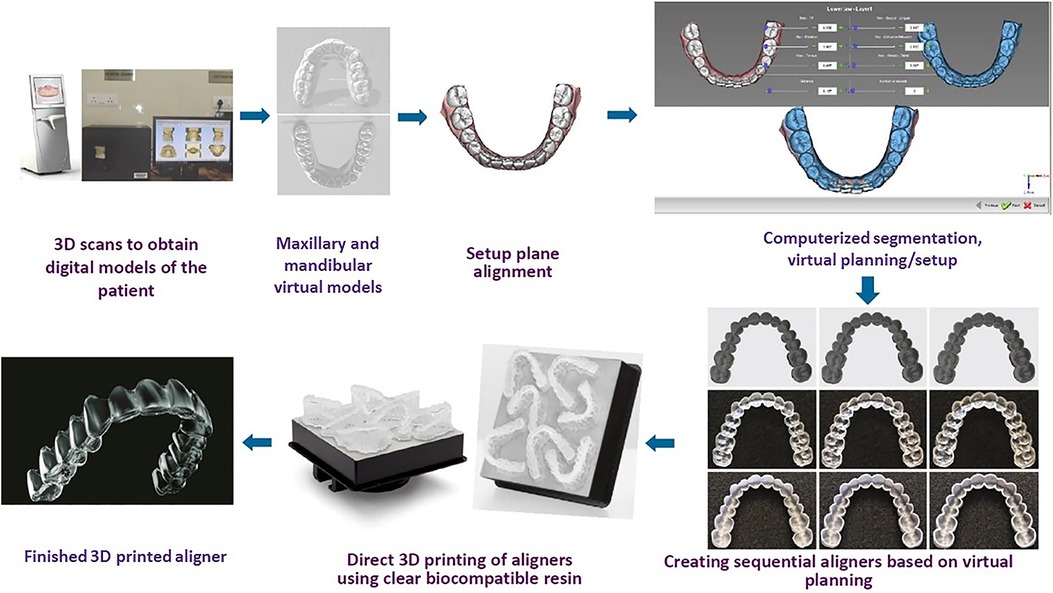
Figure 2. The key stages of laboratory procedure in the manufacturing of direct 3D-printed clear aligner.
The shortcomings related to the thermoforming technique of clear aligner manufacturing have been well documented. The process of thermoforming affects the thickness and the properties of the material. As noted from the observations of a clinical trial by Bucci et al., thermoplastic sheets of 0.75mm before processing were measured to vary between 0.38-0.69mm at different regions after processing (9). This has been further substantiated by Hahn et al., who indicated unintended local thickness variations with thermoformed aligners (10). A 10% reduction of aligner material thickness could reduce exerted forces by up to 30% theoretically (11). Thermoforming is an important way to alter material properties like transparency, surface hardness, water solubility, water absorption ability, flexural modulus, and elastic modulus of various aligner material brands and thicknesses (12–14).
Furthermore, literature evidence (11, 15) indicates that the dental model's height and thickness can affect the dimensional accuracy of the thermoformed appliance. Ihessen et al. (11) detected that aligners made from the high (i.e., 5 mm) base height of 3D-printed models were significantly thinner and more homogenous compared to those made from narrow ones (i.e., without a base). In an attempt to reduce the resin material quantity consumed, hollow models were investigated as an alternative. However, hollow models without adequate thickness (i.e., <2 mm) underwent deformation during thermoforming, thus compromising the clinical utility of the appliance (15).
Though improvements have been incorporated with newer materials and auxiliary designs in aligner orthodontics, the vast majority of manufacturing has remained to be the thermoforming technique so far (8). Leading global providers like Invisalign (Align Technology, San Jose, CA, USA), K-line (K Line Europe GmbH, Düsseldorf, Germany), and ClearCorrect (Straumann, Texas, USA) digitally plan tooth movement and print staged 3D models to make aligners using the thermoforming technique.
With the evolving digital technology and material science, the utilization of integrated systems manufacturing is widening the horizons of clear aligner manufacturing by directly printing the aligners rather than being limited to working model preparation. This article aims to concisely sum up the digital orthodontic workflow of the direct 3D-printed clear aligner manufacturing technique with literature evidence.
3. The digital orthodontic workflow of 3D-printed aligners
The sequential steps encompassing the process of direct 3D-printed clear aligner manufacturing are (i) data acquisition using scanning, (ii) virtual planning and computer-aided designing of clear aligners, (iii) 3D printing using the appropriate resin-printer combination, and (iv) post-processing (Figure 2). The description of each step is detailed as follows.
3.1. Scanning
The acquisition of patient data i.e., teeth, gingiva, and bite/occlusion relationship is employed using digital scanners based on a direct or an indirect technique. The direct technique makes use of intra-oral scanners to capture the data for developing 3D models; the indirect technique uses scanning impressions/plaster models with a desktop scanner or CT-based scanner (16).
Direct intra-oral scanners are Class-I medical electrical devices developed in compliance with ANSI/IEC 60601-1 standard (17). This obviates the need for an alginate/elastomeric impression and has positive attributes for patient comfort compared to the conventional approach (18). A wide variety of intraoral scanners with different combinations of light sources and working principles are available for the clinician's choice. The resulting file format can be proprietary or open or a combination of both. Well-known representations are (17): Trios (3Shape, Copenhagen, Denmark), iTero (Align Technology, Inc. San Jose, Calif., USA), and CS 3500 (Carestream Dental, Atlanta, GA, USA) based on parallel confocal microscopy; true definition (3M ESPE, Seefeld, Germany) based on 3D in-motion video; and Lythos (Ormco Corporation, Orange, Calif., USA) based on accordion fringe interferometry. Integrative near-infrared imaging in the scanning system widens its functionality by detecting the internal structure of the tooth.
The desktop scanner function is based on the principle of active triangulation, wherein the illumination source, the illuminated part of the intended object, and the detector constitute the resultant triangle and process the plaster models (19). The examples include R-series desktop scanners (3Shape, Copenhagen, Denmark), Maestro 3D (AGE Solutions, Pisa, Italy), and Ortho Insight 3D (Motion View Software, LLC, Chattanooga TN, USA) (20).
Digital scanners are capable of recording, storing, and transferring information, as well as carrying out occlusal evaluations, basic measurements, and model analysis. The resultant scanned data formatted as an STL (Standard Tessellation Language) file is exported into the treatment planning software.
3.2. Virtual treatment planning and designing of 3D-printed clear aligner
The software intended for computer-aided designing of clear aligners allows the segmentation of individual teeth to reset them in the desired configuration in an incremental manner. The updated versions have the feature to stitch the DICOM (Digital Imaging and Communications in Medicine) data from cone-beam/conventional computed tomographic scans to the dental model, thereby enabling better simulation and visualization of teeth roots. The virtual setup indicates the end-treatment outcome along with projected stages of tooth movement. After creating this treatment blueprint (i.e., staging), designing will ensue with the undercut block out used to facilitate an unrestricted insertion/removal path and to configure the aligner with the desired thickness, extension, attachments, and labeling.
Researchers have employed Maestro Studio CAD/CAM software (AGE solutions, Pisa, Italy) (21), dental CAD software (exocad GmbH, Germany) (22), and Deltaface CAD software (Coruo, Limoges, France) (6). Grey literature findings enlist 3Shape Clear Aligner Studio (3Shape, Copenhagen, Denmark), Blue Sky Plan (Blue Sky Bio, Libertyville, Illinois, USA), ArchFrom (Sunnyvale, Calif., USA), uDesign 7 (uLab Systems Inc., Memphis, USA), and CS Model+ (Carestream Dental, Atlanta, USA). With MeshMixer (Autodesk Inc, San Rafael, Calif., USA), the design can be prepared for a single selected model (23, 24). The output data can be transferred to the slicing software to proceed with the 3D printing of clear aligners.
3.3. 3D printing
The technique involving the rapid fabrication of physical prototypes using computer-aided manufacturing has been referred to as “Rapid prototyping” (RP). RP technologies can be divided into additive and subtractive methods. While the additive method of manufacturing builds the object layer by layer (3D printing), the subtractive method involves material removal to generate the same (25).
3D-printed aligner is a digital orthodontic innovation by the additive manufacturing technique of rapid prototyping. The computer-aided manufacturing makes use of suitable photosensitive resin and a 3D printer with its interlinked slicing software.
3.3.1. Print preparation
The process considers slicing software to accomplish nesting (i.e., packing/grouping to effectively utilize the printing space), support designing (i.e., structures that hold the printing object and preclude islands), and slicing (i.e., the act of converting STL file data into a set of instructions like G-code—a programming language to guide and direct the print head movement). Rayware (Sprintray, Los Angeles, Calif., USA), PreForm (Form Labs, Somerville, Mass., USA), and UNIZ Dental (Uniz, San Diego, Calif., USA) belong to this category. The sliced file can be exported to the 3D printer with a USB drive or wireless communication as appropriate.
3.3.2. 3D printers
3D printers used for additive manufacturing operate in all three axes including two planar dimensions (X: right-left; Y: front-back) and the Z dimension (up-down). The type of printing technology, the thickness of each print layer (Z-resolution), the printing orientation (0°, 25°, 45°, 90° to the print bed), and the overall height of the designed entity will determine the time taken for printing. Amongst the processing technologies, vat polymerization has received the most evidence-based attention so far for clear aligner fabrication. Vat polymerization is a method in 3D printing that utilizes a vat of liquid polymer resin by exposing it to ultraviolet light to solidify. The important printing technologies based on this method are stereolithography (SLA), digital light processing (DLP), and liquid crystal display (LCD) (26).
While the DLP printer uses a projector that directs light on specific areas of the resin layer by employing thousands of tiny mirrors known as digital micromirror devices, the LCD printer contains LCD panels to block out the places that are not to be solidified on each layer. Hence LCD technology has been referred to as “masked stereolithography” (mSLA). Both are fast-paced technologies that cure the entire layer of resin at once (6). The stereolithography principle uses concentrated ultra-violet laser light that cures a small specific area at one time (25). With the same and post-processing techniques, varied mechanical properties of the resultant aligner were observed with different printing technologies (6).
The literature has documented the use of DLP printers such as SprintRay Pro (Sprintray, Los Angeles, Calif., USA) (6, 23, 27), MiiCraft 125 (Young Optics Inc., Hsinchu City, Taiwan) (6, 22), Uniz 4K (Uniz, San Diego, Calif., USA) (28), and Moonray S100 (Sprintray, Los Angeles, Calif., USA) (29); LCD printers (6) such as Ka;rv LP 550 (Shinwon Dental, Seoul, Republic of Korea), Dazz 3D L120 (Nanshan, Shenzhen, China), and Slash 2 Uniz (Uniz, San Diego, Calif., USA); and a stereolithography printer, Form 2 (Form Labs, Somerville, Mass., USA) (21, 24, 30–34) in clear aligner manufacturing. All of the abovementioned devices use 365–405 nm wavelength of ultraviolet radiation to cure the photopolymerizable resin.
3.3.2. Resins for 3D printing of clear aligners
The printing resin material should possess adequate physical, mechanical, optical, and biological properties for aligner material fabrication. Currently, the Tera Harz TC-85 DAC developed by Graphy Inc., Seoul, Republic of Korea is a known CE (Conformité Européenne) Class-IIa material with Korean and United States FDA (Food and Drug Administration) approval for clear aligner direct 3D printing. It is an aliphatic vinyl ester–urethane polymer, with methacrylate functionalization (29) that exhibits shape memory (28). However, Willi et al. (35) have detected the release of UDMA (urethane di-methacrylate) from in-vitro samples that assessed the biocompatibility of its clinical usage.
A Japanese team developed an acrylic-epoxy hybrid photopolymerizable resin known as 3D:1M, Okamoto Chemicals, and undertook aligner fabrication with thicknesses of 0.7 and 1.5 mm (22). However, the aligners were fragile in the middle portion thereby necessitating further improvements in strength and flexibility, as well as requiring Pharmaceutical Affairs Act approval.
Though research models have been undertaken to print and test the clear aligner using Dental Clear LT resin (Formlabs, Somerville, Mass., USA) (21, 24, 30–34), this resin has been indicated for manufacturing direct 3D-printed hard splints, bite guards and other orthodontic appliances (36). In the gray literature, E-ortholign (EnvisionTEC, Rockhill, South Carolina, USA) and 3Dresyn OD-Clear TF LTP - Rigid and LV versions (3Dresyns, Barcelona, Spain) have been mentioned but are yet to be explored for clinical usage.
3.4. Post-processing
The post-preparation of the printed aligner material encompasses procedures like cleaning the uncured residual resin, support removal, and post-production curing. For the Tera Harz TC-85 aligners, recommendations mention the process of cleaning by centrifugation for around 3–4 min (6, 27) or removal with a soft scraper (28). This is followed by support removal and post-production curing with Cure M (Graphy, Seoul, Republic of Korea). The nitrogen generation curing unit has been utilized (28) and recommended to enhance the physical properties of the resin material by providing an inert environment, thereby preventing the formation of oxygen inhibition layer (37). THC 2 UV Curing system (Graphy Inc., Seoul, Republic of Korea) is a known representation of this category.
For the Dental LT-clear resin aligners, washing by ultrasonication with isopropyl alcohol (96/99%) and post-production curing with the Form Cure unit (Formlabs, Somerville, Mass., USA) (24, 34, 38) are undertaken.
4. Literature summary
Studies involving the development and evaluation of physical, mechanical, and biological properties of direct 3D-printed aligners have been represented in Table 1.
5. Strengths
With direct 3D-printing technology for clear aligner manufacturing, the errors related to the intermediate steps of making thermoformed aligners, such as model formation, molding, removal, and finishing, can be bypassed. Thus, it can be anticipated that aligner production will impart better precision between the initial set-up and the expected outcome. Establishing the offset for various printer and resin combinations will offer a greater advantage to control the desired and resultant thicknesses. Dimensionally controlled differential thickness can be programmed and processed according to the individual requirements of the case, resulting in better force application. The production can be with shorter supply chains, significantly shorter lead times, and lower costs. Since the thermoforming workflow generates a significant amount of non-biodegradable waste such as petroleum-based polymers used in model fabrication, the direct 3D-printing technique bestows a more environmentally friendly choice in the employment of digital technology.
6. Potential developments
The exploration of the components of system integration of direct 3D-printed aligners, such as digital scanners, virtual treatment planning modules, appliance designing software, and printing technologies, have yielded a wide range of clinically acceptable elements of an efficient standard. Currently, there is a need for more research and development in the field of material science. To come up with a cost-effective biocompatible material for intraoral contact of >20 h per day for 7–14 days (according to the protocol) that is capable of exerting optimal force levels on activation, withstand masticatory forces with adequate thickness, is esthetically nearly indistinguishable alongside teeth, and is also suitable for disinfection without any loss of material properties mandates collaborative work-up, development and testing.
7. Conclusion
The current level of research must progress with tested hypotheses to validate the evidence for the clinical application of direct 3D aligner-printing in-house technology. Further improvements and innovations will allow the delivery of easily accessible, cost-effective, digitally-driven orthodontic care in the modern era.
Author contributions
Author PKC conceived the idea and reviewed the paper; author AR prepared the manuscript. All authors contributed to the article and approved the submitted version.
Conflict of interest
The authors declare that the research was conducted in the absence of any commercial or financial relationships that could be construed as a potential conflict of interest.
Publisher's note
All claims expressed in this article are solely those of the authors and do not necessarily represent those of their affiliated organizations, or those of the publisher, the editors and the reviewers. Any product that may be evaluated in this article, or claim that may be made by its manufacturer, is not guaranteed or endorsed by the publisher.
References
1. Proffit WR, Fields HW, Larson BE, Sarver DM. Contemporary orthodontics. Philadelphia: Elsevier (2019). 16 p.
2. Yassir YA, Nabbat SA, McIntyre GT, Bearn DR. Clinical effectiveness of clear aligner treatment. Clin Oral Investig. (2022) 26:2353–70. doi: 10.1007/s00784-021-04361-1
3. Patterson BD, Foley PF, Ueno H, Mason SA, Schneider PP, Kim KB. Class II malocclusion correction with invisalign: is it possible? Am J Orthod Dentofacial Orthop. (2021) 159:e41–8. doi: 10.1016/j.ajodo.2020.08.016
4. Sycińska-Dziarnowska M, Szyszka-Sommerfeld L, Woźniak K, Lindauer SJ, Spagnuolo G. Predicting interest in orthodontic aligners: a google trends data analysis. Int J Environ Res Public Health. (2022) 19:3105. doi: 10.3390/ijerph19053105
5. Upadhyay M, Arqub SA. Biomechanics of clear aligners: hidden truths & first principles. J World Fed Orthod. (2022) 11:12–21. doi: 10.1016/j.ejwf.2021.11.002
6. Zinelis S, Panayi N, Polychronis G, Papageorgiou SN, Eliades T. Comparative analysis of mechanical properties of orthodontic aligners produced by different contemporary 3D printers. Orthod Craniofac Res. (2022) 25:336–41. doi: 10.1111/ocr.12537
7. Boyd RL, Miller RJ, Vlaskalic V. The invisalign system in adult orthodontics: mild crowding and space closure cases. J Clin Orthod. (2000) 34:203–12.
8. Tartaglia GM, Mapelli A, Maspero C, Santaniello T, Serafin M, Farronato M, et al. Direct 3D printing of clear orthodontic aligners: current state and future possibilities. Materials (Basel). (2021) 5(14):1799. doi: 10.3390/ma14071799
9. Bucci R, Rongo R, Levatè C, Michelotti A, Barone S, Razionale AV, et al. Thickness of orthodontic clear aligners after thermoforming and after 10 days of intraoral exposure: a prospective clinical study. Prog Orthod. (2019) 20:1–8. doi: 10.1186/s40510-019-0289-6
10. Hahn W, Dathe H, Fialka-Fricke J, Fricke-Zech S, Zapf A, Kubein-Meesenburg D, et al. Influence of thermoplastic appliance thickness on the magnitude of force delivered to a maxillary central incisor during tipping. Am J Orthod Dentofacial Orthop. (2009) 136:12.e1–7. doi: 10.1016/j.ajodo.2008.12.015
11. Ihssen BA, Kerberger R, Rauch N, Drescher D, Becker K. Impact of dental model height on thermoformed PET-G aligner thickness—an in vitro micro-CT study. Appl Sci. (2021) 21(11):6674. doi: 10.3390/app11156674
12. Ryu JH, Kwon JS, Jiang HB, Cha JY, Kim KM. Effects of thermoforming on the physical and mechanical properties of thermoplastic materials for transparent orthodontic aligners. Korean J Orthod. (2018) 48:316. doi: 10.4041/kjod.2018.48.5.316
13. Dalaie K, Fatemi SM, Ghaffari S. Dynamic mechanical and thermal properties of clear aligners after thermoforming and aging. Prog Orthod. (2021) 22:15. doi: 10.1186/s40510-021-00362-8
14. Golkhani B, Weber A, Keilig L, Reimann S, Bourauel C. Variation of the modulus of elasticity of aligner foil sheet materials due to thermoforming. J Orofac Orthop Fortschritte Kieferorthopädie. (2022) 83:233–43. doi: 10.1007/s00056-021-00327-w
15. Kenning KB, Risinger DC, English JD, Cozad BE, Harris LM, Ontiveros JC, et al. Evaluation of the dimensional accuracy of thermoformed appliances taken from 3D printed models with varied shell thicknesses: an in vitro study. Int Orthod. (2021) 19:137–46. doi: 10.1016/j.ortho.2021.01.005
16. Wiranto MG, Engelbrecht WP, Tutein Nolthenius HE, van der Meer WJ, Ren Y. Validity, reliability, and reproducibility of linear measurements on digital models obtained from intraoral and cone-beam computed tomography scans of alginate impressions. Am J Orthod Dentofacial Orthop. (2013) 143:140–7. doi: 10.1016/j.ajodo.2012.06.018
17. Kravitz ND, Groth C, Jones PE, Graham JW, Redmond WR. Intraoral digital scanners. J Clin Orthod. (2014) 48:337–47. PMID: 2508375425083754
18. Christopoulou I, Kaklamanos EG, Makrygiannakis MA, Bitsanis I, Tsolakis AI. Patient-reported experiences and preferences with intraoral scanners: a systematic review. Eur J Orthod. (2022) 44:56–65. doi: 10.1093/ejo/cjab027
19. Park JM, Shim JS. Optical impression in restorative dentistry. In: Machoy ME, editor. Computer vision in dentistry. London: InTech Open (2019). p. 3–4. doi: 10.5772/intechopen.84605
20. Taneva E, Kusnoto B, Evans CA. 3D Scanning, imaging, and printing in orthodontics. In: Bourzgui F, editor. Issues in contemporary orthodontics. London: InTech (2015). p. 162–4. doi: 10.5772/60010
21. Jindal P, Juneja M, Bajaj D, Siena FL, Breedon P. Effects of post-curing conditions on mechanical properties of 3D printed clear dental aligners. Rapid Prototyp J. (2020) 2:1337–44. doi: 10.1108/RPJ-04-2019-0118
22. Nakano H, Kato R, Kakami C, Okamoto H, Mamada K, Maki K. Development of biocompatible resins for 3D printing of direct aligners. J Photopolym Sci Technol. (2019) 32:209–16. doi: 10.2494/photopolymer.32.209
23. Koenig N, Choi JY, McCray J, Hayes A, Schneider P, Kim KB. Comparison of dimensional accuracy between direct-printed and thermoformed aligners. Korean J Orthod. (2022) 52:249–57. doi: 10.4041/kjod21.269
24. McCarty MC, Chen SJ, English JD, Kasper F. Effect of print orientation and duration of ultraviolet curing on the dimensional accuracy of a 3-dimensionally printed orthodontic clear aligner design. Am J Orthod Dentofacial Orthop. (2020) 158:889–97. doi: 10.1016/j.ajodo.2020.03.023
25. Groth C, Kravitz ND, Jones PE, Graham JW, Redmond WR. Three-Dimensional printing technology. J Clin Orthod. (2014) 48:475–85. PMID: 2522604025226040
26. Piedra-Cascón W, Krishnamurthy VR, Att W, Revilla-León M. 3D Printing parameters, supporting structures, slicing, and post-processing procedures of vat-polymerization additive manufacturing technologies: a narrative review. J Dent. (2021) 109:103630. doi: 10.1016/j.jdent.2021.103630
27. Pratsinis H, Papageorgiou SN, Panayi N, Iliadi A, Eliades T, Kletsas D. Cytotoxicity and estrogenicity of a novel 3-dimensional printed orthodontic aligner. Am J Orthod Dentofacial Orthop. (2022) 162:e116–22. doi: 10.1016/j.ajodo.2022.06.014
28. Lee SY, Kim H, Kim HJ, Chung CJ, Choi YJ, Kim SJ, et al. Thermo-mechanical properties of 3D printed photocurable shape memory resin for clear aligners. Sci Rep. (2022) 12:6246. doi: 10.1038/s41598-022-09831-4
29. Can E, Panayi N, Polychronis G, Papageorgiou SN, Zinelis S, Eliades G, et al. In-house 3D-printed aligners: effect of in vivo ageing on mechanical properties. Eur J Orthod. (2022) 44:51–5. doi: 10.1093/ejo/cjab022
30. Boyer RA, Kasper FK, English JD, Jacob HB. Effect of print orientation on the dimensional accuracy of orthodontic aligners printed 3-dimensionally. Am J Orthod Dentofacial Orthop. (2021) 160:732–42.e1. doi: 10.1016/j.ajodo.2021.01.018
31. Milovanović A, Sedmak A, Golubović Z, Mihajlović KZ, Žurkić A, Trajković I, et al. The effect of time on mechanical properties of biocompatible photopolymer resins used for fabrication of clear dental aligners. J Mech Behav Biomed Mater. (2021) 119:104494. doi: 10.1016/j.jmbbm.2021.104494
32. Jindal P, Juneja M, Siena FL, Bajaj D, Breedon P. Mechanical and geometric properties of thermoformed and 3D printed clear dental aligners. Am J Orthod Dentofacial Orthop. (2019) 156:694–701. doi: 10.1016/j.ajodo.2019.05.012
33. Jindal P, Worcester F, Siena FL, Forbes C, Juneja M, Breedon P. Mechanical behaviour of 3D printed vs thermoformed clear dental aligner materials under non-linear compressive loading using FEM. J Mech Behav Biomed Mater. (2020) 112:104045. doi: 10.1016/j.jmbbm.2020.104045
34. Ahamed SF, Kumar SM, Kanna ASA, Dharshini KI. Cytotoxic evaluation of directly 3D printed aligners and invisalign. Clin Med. (2020) 7:12.
35. Willi A, Patcas R, Zervou SK, Panayi N, Schätzle M, Eliades G, et al. Leaching from a 3D-printed aligner resin. Eur J Orthod. (2022) cjac056. doi: 10.1093/ejo/cjac056
36. Panayi NC. Consider material properties when selecting a resin for 3-dimensionally printed aligners. Am J Orthod Dentofacial Orthop. (2021) 159(6):706. doi: 10.1016/j.ajodo.2021.02.012
37. Wada J, Wada K, Gibreel M, Wakabayashi N, Iwamoto T, Vallittu PK, et al. Effect of nitrogen gas post-curing and printer type on the mechanical properties of 3D-printed hard occlusal splint material. Polymers (Basel). (2022) 14:3971. doi: 10.3390/polym14193971
Keywords: clear aligner appliance, 3D printed aligners, clear dental brace, removable orthodontic appliance, digital orthodontics, digital dentistry, additive manufacturing, 3D printing in orthodontics
Citation: Rajasekaran A and Chaudhari PK (2023) Integrated manufacturing of direct 3D-printed clear aligners. Front. Dent. Med 3:1089627. doi: 10.3389/fdmed.2022.1089627
Received: 4 November 2022; Accepted: 30 November 2022;
Published: 6 January 2023.
Edited by:
Francisco Nociti, Universidade Estadual de Campinas, BrazilReviewed by:
Ana Millás, 3D Biotechnology Solutions - 3DBS - Brazil, BrazilCopyright: © 2023 Rajasekaran and Chaudhari. This is an open-access article distributed under the terms of the Creative Commons Attribution License (CC BY). The use, distribution or reproduction in other forums is permitted, provided the original author(s) and the copyright owner(s) are credited and that the original publication in this journal is cited, in accordance with accepted academic practice. No use, distribution or reproduction is permitted which does not comply with these terms.
*Correspondence: Prabhat Kumar Chaudhari, ZHIucHJhYmhhdGtjQGdtYWlsLmNvbQ==
Specialty Section: This article was submitted to Systems Integration, a section of the journal Frontiers in Dental Medicine