- 1School of Dentistry, University of Queensland, Brisbane, QLD, Australia
- 2Department of Conservative and Prosthetic Dentistry, School of Dentistry, Complutense University, Madrid, Spain
Introduction: Cyclic fatigue resistance of rotary endodontic instruments has been extensively studied in the last two decades, yet with no standardization. While new low-cost instruments are now manufactured, a standard is lacking to guarantee a minimum quality. This study aimed to validate a new model for CF testing through a fixture proposed for ISO Specification 3630-1 and to establish a minimum quality standard based on testing conditions and material characteristics.
Materials and methods: Size 25/0.06 instruments of ProFile Vortex (PF) and Vortex Blue (VB) were run until failure in curved metallic fixtures (5 or 7.5 mm radius) built according to a proposal for an additional test for the ISO 3630-1 standard. High resolution videos were recorded, number of cycles to failure (NCF) registered and apical fragments measured with a digital caliper. Surface strain was determined from test dimensions and fragment lengths. Mean life, β and η parameters were calculated with Weibull analysis. NCF data were compared using student's t-tests and referenced to a minimum required cycles at fracture (Cmin) based on austenite finish temperatures, testing temperature and deformation.
Results: VB instruments were statistically more resistant than PF in both 7.5 mm radius curve (p = 0.001) and 5 mm radius curve (p = 0.002) simulated canals. Weibull probability plots showed higher mean life for VB than PV. NCF for both instruments were higher than Cmin.
Conclusions: The NCF results in this study support the findings of previous studies where VB and PF were compared. The novel test design appears a suitable addition to ISO 3630-1.
Introduction
Since Walia et al. (1) introduced nickel-titanium (NiTi) instruments for root canal therapy in 1988, their properties have improved, with newer rotary systems demonstrating increased flexibility (2), as well as efficiency and cutting abilities (3). Further, the availability of proprietary thermal treatments in gas atmospheres or salt baths (4) has resulted in an apparent improvement in the mechanical properties of shaping instruments (4–6).
Indeed, numerous studies have already detailed ex vivo performance of these instruments. Specifically, torsional or cyclic fatigue (CF) behaviors of instruments were discussed (4, 7). CF resistance of nickel-titanium rotary endodontic instruments have been analyzed as early as 1997 (8). Subsequently, CF resistance of rotary endodontic instruments has been rigorously studied for more than two decades now. A variety of approaches have been deployed in the assessment of fatigue resistance and these have been extensively reviewed (4, 9).
The basic requirements for the testing of endodontic instruments are defined in the revised ADA Specification No. 28 (10) and ISO Specification 3630-1:2008 (11). However, none of these norms describe instruments with tapers >0.02 and neither include a description of a fatigue test. In the absence of one applicable norm fatigue devices have been designed with very different characteristics.
In 2009, Plotino et al. (9) criticized that although many studies at that time (and to this day) used a roughly comparable design, no attention has been paid to the diameter of the tubes or to the fit of the instrument inside the tubes. Nevertheless, a considerable variance in details of study protocols is evident in the literature, making comparisons of results very difficult, if not impossible. Some form of standardization was deemed necessary to obtain comparable results and identify reliable clinical implications.
At the same time, new martensitic alloys have demonstrated superior fatigue behavior (6, 12). Most CF studies of NiTi files have been performed at room temperature, however recent literature confirmed that sodium hypochlorite placed in root canals at room temperature rapidly approached body temperature (13). For this reason, instruments are expected to work at body temperature during clinical use. Importantly, a decrease in CF of martensitic instruments has been reported for increased temperature (14–16).
Differential scanning calorimetry (DSC) analysis of a specific instrument identifies the crystallographic transformation temperatures. This phase transformation determines the temperature at which the instrument would be working in a martensitic or austenitic phase and hence allows to understand the properties of the instrument in clinical conditions. Therefore, any testing device specifically designed to investigate the cyclic fatigue resistance of rotary endodontic instruments should consider the transformation temperatures of the specific alloy included in the study. Moreover, low-cost instruments are now manufactured, and no comprehensive standard has been established to guarantee that instruments have a minimum quality before being marketed.
Therefore, the aim of this study was to validate a new model to evaluate cyclic fatigue resistance of nickel-titanium (NiTi) instruments through a test rig proposed for ISO Specification 3630-1 (11) and to establish a minimum quality standard based on testing conditions and material characteristics.
Materials and Methods
Instruments
A total of 80 Vortex rotary instruments (40 ProFile Vortex (PF) manufactured with M-wire and 40 Vortex Blue (VB) manufactured with the so-called blue wire) (Dentsply Sirona, York, PA, USA), tip size 25 and 0.06 taper were randomly selected from stock. All instruments were tested for CF resistance actioned statically at manufacturer recommended setting of 500 rpm, with a 3Ncm torque limit. Instruments from both groups were randomly distributed into 2 groups (n = 20 each) and run until failure in metallic artificial canals with either a small or a large radius of curvature (5 or 7.5 mm) and a fixed angle of curvature of 75°. A fixture with the artificial canals was specifically designed for the purpose (Figure 1).
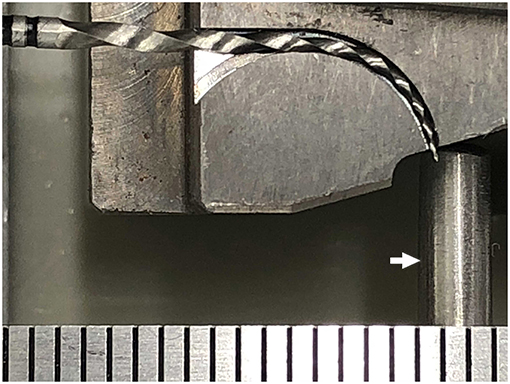
Figure 1. Photograph of a ProFile Vortex size 25, 0.06 taper instrument inserted into the fixture. The probe of the thermometer is indicated by the arrow. Gradation of ruler is in mm.
Fixture Design
The testing environment was constructed according to a proposal for an additional test to be added to the ISO 3630-1 standard (Supplementary Material). In brief, a set of curved fixtures consisted of a surface-hardened steel base with a lid that kept the tested instrument aligned in a milled tapered channel. A cut-out in the lid provided visual access to the instrument tip. The fixture was mounted, along with an electric motor, on a base plate so that the rotary could be placed into the artificial canal with the tip just short of the backstop built into the lid of the fixture.
The fixture was fitted with a digital thermometer and temperature was continuously recorded during each test run. Cooling was accomplished by compressed air flow directed toward the rotating instrument through an opening in the simulated canal. Air pressure was regulated so that temperatures did not increase more than 2° C during a run.
A high-resolution video clip recorded each run at 30 frames per second and the frame numbers of the beginning and end of instrument rotation were tabulated. Apical instrument fragments were collected, and length determined with a digital caliper with a 0.01-mm readout.
The surface strains at the locations of fracture were derived from the test dimensions and fragment lengths using Equation 1:
The test proposal calls for a strain rate between 2 and 8% for the test to be valid.
Data Evaluation
Numbers of rotations to failure were calculated and was used as dependent variable. Both the instrument alloy and the radius of curvature of the fixture design (large radius, low strain vs. small radius, high strain) were included as independent variables.
An initial analysis confirmed that number of cycles to failure (NCF) data was compatible with a normal distribution. Levene's test of equality of variances demonstrated similar variances for groups tested at 7.5-mm radius of curvature (p = 0.34), but unequal for 5-mm radius curvature (p = 0.016). NCF for PF and VB for each radius of curvature were then compared with Student's t-tests. Degrees of freedom were adjusted using the Welch-Satterthwaite method to compensate for the violation for Equality of Variances when cyclic fatigue was tested in a 5-mm radius curvature.
Secondly, a Weibull analysis (Weibull ++ 7, Reliasoft Corporation, Tucson AZ, USA) was used to compare groups and calculate the following parameters (and their 95% confidence intervals) for each group:
- Mean life (s) or the expected or average time to failure.
- Beta or shape parameter (dimensionless), the values of which are equal to the slopes of the regressed lines in the Weibull probability plot and are particularly significant because they provide a clue to the physics of the failure.
- Eta (s), characteristic life or scale parameter: expected time that 63.2% of the files will attain without breakage.
Lastly, a correction factor (Ct) based on the austenite finish temperature (Af) determined with Differential Scanning Calorimetry (PF: 50.23°C; VB: 37.09°C, Y. Gao, personal communication) and testing temperature (Tt) was used as stipulated in the proposal:
Ct accounted for the ambient temperature during the test was 3.0 for PF and 2.66±0.06 for VB instruments. As proposed, a minimum NCF (Cmin) was calculated based on the Equation 3:
The instrument passes the test if NCF ≥Cmin. NCF values for each instrument were compared to Cmin. As is stipulated in the proposal, if 8 or less samples pass out of 10, the product fails. If nine samples pass, 10 additional samples should be tested. All instruments should pass the test individually for the product to comply.
Results
Mean values and standard deviations for each tested variable and environmental factors at the two different radii and for both instruments are shown in Table 1. VB instruments were statistically more resistant to cyclic fatigue than PF when tested both in a 7.5-mm radius curve (p = 0.001) and 5-mm radius curve simulated root canal (p = 0.002). However, no significant differences were found when the same instrument was compared under the two different radii of curvature settings. Figure 2 shows the relation of surface strain to NCF, indicating the formation of 4 clusters, albeit with considerable variation within each group. Linear regression analysis did not reveal any strong associations between the two variables when viewed separately in the four clusters, neither was there an overall relationship.
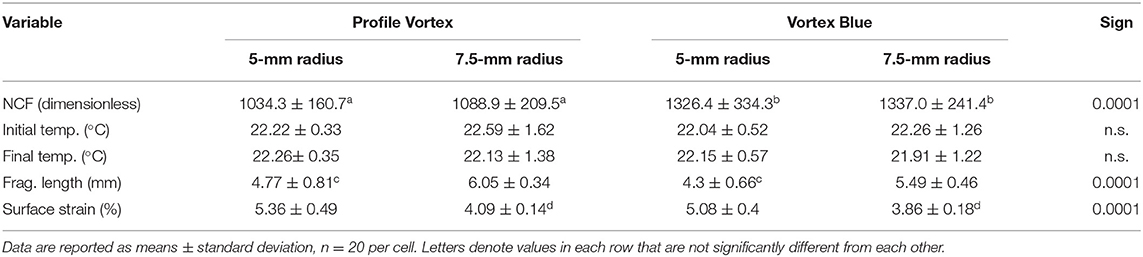
Table 1. Parametric statistics comparing experiments with the two rotaries and the two different fixtures.
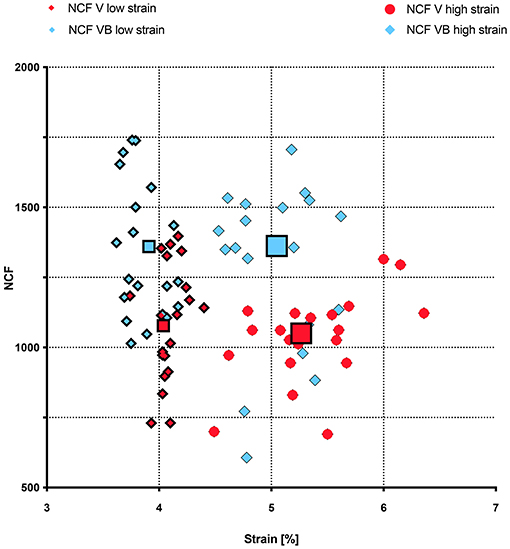
Figure 2. Numbers of rotations to failure (NCF) plotted against strain values. Vortex data is shown in red, VB in light blue. Symbol size related to low strain (7.5 mm radius) and large to high strain (5 mm radius). Global group means and standard deviations are indicated.
Weibull probability plots (reliability vs. time) per group for both tested radii of curvature are shown in Figure 3. Mean life, eta, and beta parameters and their 95% confidence intervals are shown in Table 2.
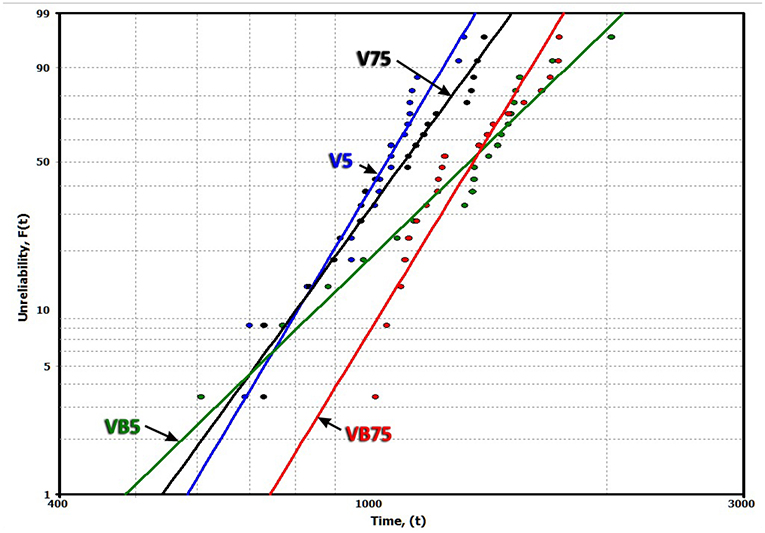
Figure 3. Weibull probability plots (reliability vs. time) per group for both tested radii of curvature.
Vortex Blue instruments significantly outlasted PF instruments for both tested radii of curvature with a probability of 79% when the instruments were tested in a setting with 7.5-mm radius of curvature and 77% when the radius of curvature was set at 5 mm. Neither PF nor VB instruments showed significant differences when tested at the two different radii of curvature settings. On the other hand, the highest beta parameter, indicating the highest predictability, was found for VB when tested at a 7.5 mm radius curvature and PF when tested in a 5 mm radius of curvature simulated root canal. The slope of the line in the figure also confirmed this assumption.
When using the correction factor, the range of rotations needed to pass the test ranged from 109 to 171, with a mean of 143 NCF. Using this criterion for a successful test, all rotaries tested passed the test for both geometrical conditions.
Discussion
This work describes an initial validation study for a new framework to potentially be added to the current ISO 3630-1 norm. This norm in its current form does not address contemporary rotary instruments that vary considerably from traditional so-called ISO norm hand instruments. Specifically, there is no test described to determine CF resistance of engine-driven rotary instruments, yet this remains a primary source of intracanal fracture. Understandably, no fatigue test model can perfectly recreate all the clinical parameters observed during an endodontic procedure. The implementation of a standardized fatigue testing device would be a valuable tool for manufacturers to quality control prototypes for future novel endodontic instruments, but not necessarily as a standard for comparison between different proprietary instruments, particularly where the geometries and dimensions of the instruments differ.
ProFile Vortex and Vortex Blue were compared in the present study. These instruments were specifically selected for the present study to avoid the influence of instrument design. Both instruments share the exact same design except for the alloy in which they were manufactured. Other authors have previously used PF and VB to isolate the effect of the alloy in CF resistance (5, 17–20). Table 3 presents selected data of those publications that also compared PF and VB instruments regarding their cyclic fatigue resistance.
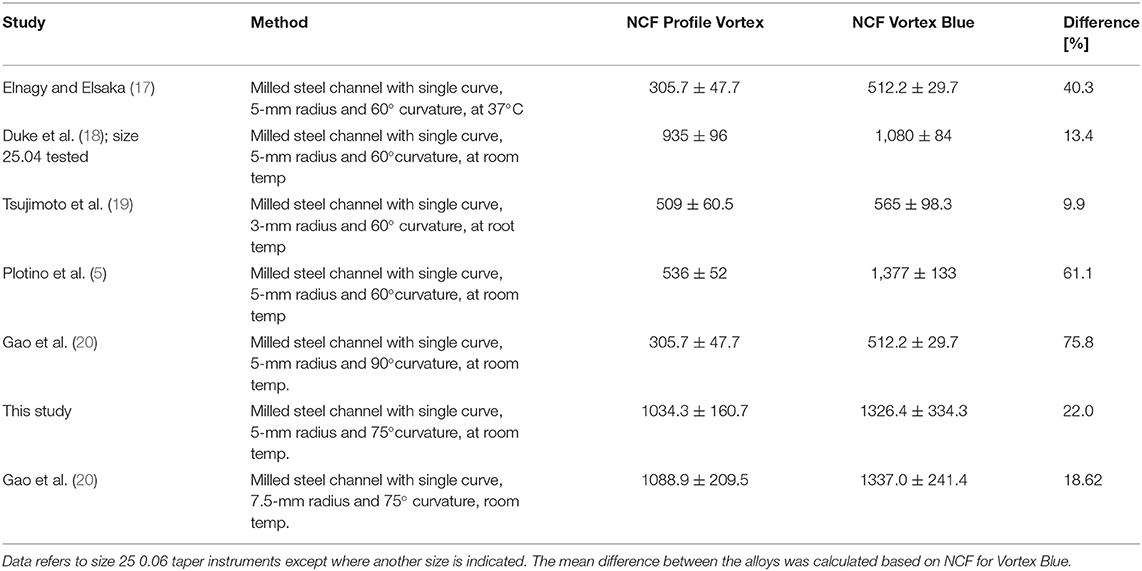
Table 3. Selected data of publications that directly compared Profile Vortex and Vortex Blue instruments regarding their cyclic fatigue resistance.
Expectedly, the results in this study demonstrate a higher cyclic fatigue resistance for VB compared to PF, which is in agreement with the studies detailed in Table 3. The mean NCF values of both the VB and PF instruments appear to have exceeded the minimum mean NCF by over 10-fold, with a pass rate of 100% for every sample tested in each of the 5 and 7.5 mm test rigs. The criterion for passing the benchmark appear to be considerably low when compared to the high NCF values that PF and VB are capable of. One may speculate that that there could potentially be a market for lower-costing NiTi instruments that would pass the minimum requirements. The expectation is that new instrument designs, particularly for novel metallurgical compositions, can also be tested against this benchmark before proceeding with full scale production.
The proposed cyclic fatigue test rig is also validated with regards to strain rates seen in both the 5 and 7.5 mm fixture, with all the test values shown to be between 2 and 8%, which appears to be similar to strain rates expected in clinical use, as judged from canal curvatures. Previous fatigue studies have not discussed whether their test conditions were reasonable and may have either subceeded or exceeded the clinically relevant strain rates.
On the other hand, there certain limitations with the proposed system; for example, the test fixture does not approximate clinical conditions (21). Rather it presents a standardized test for manufacturing standards. Moreover, the mathematical approach taken in Equations 2, 3 was based on empirical findings from currently marketed instruments. It appears that future research is needed to potentially amend and fine-tune the equations that are meant to provide a cut-off for quality assurance.
Recent studies have convincingly demonstrated a higher CF resistance of instruments with new alloys and designs; however, low-cost instruments are being incorporated to the market and a lower reliability has been reported to be expected when using such offerings (12, 22). We suggest that minimum criterion be implemented for manufacturers to demonstrate the reliable use of new instruments in clinical settings, before marketing a new product. In fact, as Hülsmann suggested in recent publications (21, 23), it should be the responsibility of the manufacturers to provide comprehensive data on CF resistance. Indeed, such relevant data should be based on accepted standards across all manufacturers to demonstrate required performance.
Such standards should also evolve continuously at the same rhythm as industry launches new developments. As an example, the proposal in the present study applied a correction factor based on the austenite finish temperature of each alloy included in the study, determined with differential scanning calorimetry, and the testing temperature. This stipulation was not included in any standard before, but literature has thoroughly demonstrated how CF resistance of martensitic alloys is considerably affected by temperature (14–16). Any further development that may affect CF resistance of endodontic rotary instruments would require a new implementation or factor correction in future proposals. In conclusion, the data presented in this study support the findings of previous studies where VB and PF were compared. This study affirms the new test model as a suitable addition to ISO 3630-1.
Data Availability Statement
The raw data supporting the conclusions of this article will be made available by the authors, without undue reservation.
Author Contributions
OP conceived and designed the study. KA collected data. OP, AA, and JM analyzed the data. PC, OP, and AA wrote the manuscript. OP and PC edited the final version of the text. All authors reviewed and approved the manuscript.
Conflict of Interest
OP is a consultant for Dentsply Sirona.
The remaining authors declare that the research was conducted in the absence of any commercial or financial relationships that could be construed as a potential conflict of interest.
Publisher's Note
All claims expressed in this article are solely those of the authors and do not necessarily represent those of their affiliated organizations, or those of the publisher, the editors and the reviewers. Any product that may be evaluated in this article, or claim that may be made by its manufacturer, is not guaranteed or endorsed by the publisher.
Acknowledgments
The authors thank Mr. Francois Aeby, Dentsply Sirona, Ballaigues, Switzerland, for donating the test fixture.
Supplementary Material
The Supplementary Material for this article can be found online at: https://www.frontiersin.org/articles/10.3389/fdmed.2021.744809/full#supplementary-material
References
1. Walia HM, Brantley WA, Gerstein H. An initial investigation of the bending and torsional properties of Nitinol root canal files. J Endod. (1988) 14:346–51. doi: 10.1016/S0099-2399(88)80196-1
2. Pereira ES, Viana AC, Buono VT, Peters OA, Bahia MG. Behavior of nickel-titanium instruments manufactured with different thermal treatments. J Endod. (2015) 41:67–71. doi: 10.1016/j.joen.2014.06.005
3. Peters OA, Morgental RD, Schulze KA, Paque F, Kopper PM, Vier-Pelisser FV. Determining cutting efficiency of nickel-titanium coronal flaring instruments used in lateral action. Int Endod J. (2014) 47:505–13. doi: 10.1111/iej.12177
4. Shen Y, Cheung GS. Methods and models to study cyclic fatigue. Endod Topics. (2013) 44:18–41. doi: 10.1111/etp.12046
5. Plotino G, Grande NM, Cotti E, Testarelli L, Gambarini G. Blue treatment enhances cyclic fatigue resistance of vortex nickel-titanium rotary files. J Endod. (2014) 40:1451–3. doi: 10.1016/j.joen.2014.02.020
6. De-Deus G, Silva EJ, Vieira VT, Belladonna FG, Elias CN, Plotino G, et al. Blue thermomechanical treatment optimizes fatigue resistance and flexibility of the reciproc files. J Endod. (2017) 43:462–6. doi: 10.1016/j.joen.2016.10.039
7. Wei X, Ling J, Jiang J, Huang X, Liu L. Modes of failure of ProTaper nickel-titanium rotary instruments after clinical use. J Endod. (2007) 33:276–9. doi: 10.1016/j.joen.2006.10.012
8. Pruett JP, Clement DJ, Carnes DL. Cyclic fatigue testing of nickel-titanium endodontic instruments. J Endod. (1997) 23:77–85. doi: 10.1016/S0099-2399(97)80250-6
9. Plotino G, Grande NM, Cordaro M, Testarelli L, Gambarini G. A review of cyclic fatigue testing of nickel-titanium rotary instruments. J Endod. (2009) 35:1469–76. doi: 10.1016/j.joen.2009.06.015
10. ANSI/ADA Standard No. 28 - Root Canal Files and Reamers, Type K: 2008 (Reaffirmed 2013). Dental Products: Standards, Technical Specifications and Technical Reports. American Dental Association (2013).
11. ISO 3630–1:2008 Dentistry — Root-Canal Instruments — Part 1: General Requirements and Test Methods. International Organization for Standardization 2012 (2012).
12. Scott R, Arias A, Macorra JC, Govindjee S, Peters OA. Resistance to cyclic fatigue of reciprocating instruments determined at body temperature and phase transformation analysis. Aust Endod J. (2019) 45:400–6. doi: 10.1111/aej.12374
13. de Hemptinne F, Slaus G, Vandendael M, Jacquet W, De Moor RJ, Bottenberg P. In vivo intracanal temperatureevolution during endodontic treatment after the injection of room temperature or preheated sodium hypochlorite. J Endod. (2015) 41:1112–5. doi: 10.1016/j.joen.2015.02.011
14. Jamleh A, Yahata Y, Ebihara A, Bakhsh T, Suda H. Performance of NiTi endodontic instruments under different temperatures. Odont. (2016) 104:324–8. doi: 10.1007/s10266-015-0214-5
15. de Vasconcelos RA, Murphy S, Curvalho CAT, Govindjee R, Govindjee S, Peters OA. Evidence of reduced fatigue resistance of contemporary rotary instruments exposed to body temperature. J Endod. (2016) 42:782–7. doi: 10.1016/j.joen.2016.01.025
16. Plotino G, Grande NM, Mercade Bellido M, Testarelli L, Gambarini G. Influence of temperature on cyclic fatigue resistance of ProTaper Gold and ProTaper Universal rotary files. J Endod. (2017) 43:200–2. doi: 10.1016/j.joen.2016.10.014
17. Elnaghy AM, Elsaka SE. Cyclic fatigue resistance of one curve, 2Shape, ProFile vortex, vortex blue, and RaCe nickel-titanium rotary instruments in single and double curvature canals. J Endod. (2018) 44:1725–30. doi: 10.1016/j.joen.2018.07.023
18. Duke F, Shen Y, Zhou H, Ruse ND, Wang ZJ, Hieawy A, et al. Cyclic fatigue of ProFile vortex and vortex blue nickel-titanium files in single and double curvatures. J Endod. (2015) 41:1686–90. doi: 10.1016/j.joen.2015.06.012
19. Tsujimoto M, Irifune Y, Tsujimoto Y, Yamada S, Watanabe I, Hayashi Y. Comparison of conventional and new-generation nickel-titanium files in regard to their physical properties. J Endod. (2014) 40:1824–9. doi: 10.1016/j.joen.2014.06.009
20. Gao Y, Gutmann JL, Wilkinson K, Maxwell R, Ammon D. Evaluation of the impact of raw materials on the fatigue and mechanical properties of ProFile Vortex rotary instruments. J Endod. (2012) 38:398–401. doi: 10.1016/j.joen.2011.11.004
21. Hülsmann M, Donnermeyer D, Schäfer E. A critical appraisal of studies on cylic fatigue resistance of engine-driven endodontic instruments. Int Endod J. (2019) 52:1427–45 doi: 10.1111/iej.13182
22. Arias A, Hejlawy S, Murphy S, de la Macorra JC, Govindjee S, Peters OA. Variable impact by ambient temperature on fatigue resistance of heat-treated nickel titanium instruments. Clin Oral Invest. (2019) 23:1101–8. doi: 10.1007/s00784-018-2543-6
Keywords: endodontics, nickel titanium, martensite, rotary, cyclic fatigue, norm, validation, Weibull analysis
Citation: Peters OA, Chien PY-H, Armitt K, Macorra JC and Arias A (2021) Testing Cyclic Fatigue Resistance of Nickel Titanium Rotary Endodontic Instruments: A Validation Study for a Minimum Quality Criterion in a Standardized Environment. Front. Dent. Med. 2:744809. doi: 10.3389/fdmed.2021.744809
Received: 21 July 2021; Accepted: 25 August 2021;
Published: 27 September 2021.
Edited by:
Ya Shen, University of British Columbia, CanadaReviewed by:
Sangwon Kwak, Pusan National University, South KoreaHe Liu, University of British Columbia, Canada
Copyright © 2021 Peters, Chien, Armitt, Macorra and Arias. This is an open-access article distributed under the terms of the Creative Commons Attribution License (CC BY). The use, distribution or reproduction in other forums is permitted, provided the original author(s) and the copyright owner(s) are credited and that the original publication in this journal is cited, in accordance with accepted academic practice. No use, distribution or reproduction is permitted which does not comply with these terms.
*Correspondence: Ove A. Peters, by5wZXRlcnMmI3gwMDA0MDt1cS5lZHUuYXU=