- Centre for Craniofacial and Regenerative Biology, Faculty of Oral and Craniofacial Sciences, Guy's Hospital, King's College London, London, United Kingdom
Teeth are complex structures where a soft dental pulp tissue is enriched with nerves, vasculature and connective tissue and encased by the cushioning effect of dentin and the protection of a hard enamel in the crown and cementum in the root. Injuries such as trauma or caries can jeopardise these layers of protection and result in pulp exposure, inflammation and infection. Provision of most suitable materials for tooth repair upon injury has been the motivation of dentistry for many decades. Wnt signalling, an evolutionarily conserved pathway, plays key roles during pre- and post-natal development of many organs including the tooth. Mutations in the components of this pathway gives rise to various types of developmental tooth anomalies. Wnt signalling is also fundamental in the response of odontoblasts to injury and repair processes. The complexity of tooth structure has resulted in diverse studies looking at specific compartments or cell types of this organ. This review looks at the current advances in the field of tooth development and regeneration. The objective of the present review is to provide an updated vision on dental biomaterials research, focusing on their biological properties and interactions to act as evidence for their potential use in vital pulp treatment procedures. We discuss the outstanding questions and future directions to make this knowledge more translatable to the clinics.
WNT Signalling Pathway
Wnt signalling pathway is critical during various stages of embryogenesis, tissue homeostasis and wound repair where it controls regulation of cell proliferation, differentiation, polarisation, and apoptosis. Here, a brief introduction of Wnt signalling pathway is followed by its role during tooth and periodontal tissue development, homeostasis, and regeneration. Particularly, significance of Wnt signalling is reviewed in dental pulp stem cells and odontoblasts. We also look at factors affecting odontoblast's function and regulation of epigenetics in dental pulp cells by Wnt signalling pathway. We finally look at how new advances in biomedicine and technology utilises the new knowledge in tissue regeneration and how that can be applied in dentistry.
Wnt signalling pathway consists of 19 cysteine rich protein ligands and the receptor complex which comprises of 10 seven-pass transmembrane receptors called Frizzled (Fzd), and LDL receptor-related proteins 5 and 6 (LRP5 and LRP6) which mediate the signalling. Upon binding of the Wnt ligand to the extracellular cysteine rich domain of Fzd, signal is transduced to a cytoplasmic phosphoprotein called Dishevelled (Dsh/Dvl). Wnt signalling pathway can be canonical or non-canonical (1). In canonical Wnt signalling, binding of ligands to receptors and coreceptors results in the formation of Wnt-Fz-LRP complex and recruitment of Dsh/Dvl. This results in recruitment of the Axin complex to the receptor, inhibition of Axin-mediated β-catenin phosphorylation and subsequently stabilisation of β-catenin. This results in translocation of β-catenin into nuclei to form the TCF/LEF complex and activate Wnt target genes. In the absence of Wnt, cytoplasmic β-catenin is degraded by a β-catenin destruction complex. This complex includes Axin, adenomatosis polyposis coli (APC), protein phosphatase 2A (PP2A), glycogen synthase kinase 3 (GSK3) and casein kinase 1α (CK1α) (2).
Non-canonical Wnt signalling consists of Wnt-PCP and Wnt-Ca2+ pathway (3). This pathway signals through Fzds, ROR2, RYK or through Fzds with ROR, or RYK as co-receptor and results in activation of downstream effectors such as calcium/Calmodulin dependent protein kinase II, mobilisation of Ca2+, heterotrimeric G proteins and multiple small GTPases (4). Non Canonical Wnt signalling is involved in the maintenance of stem cells, regulating cell polarity, directional cell movement, promoting invasion, and inhibiting the canonical Wnt//β-catenin signalling cascade (5) (Figure 1).
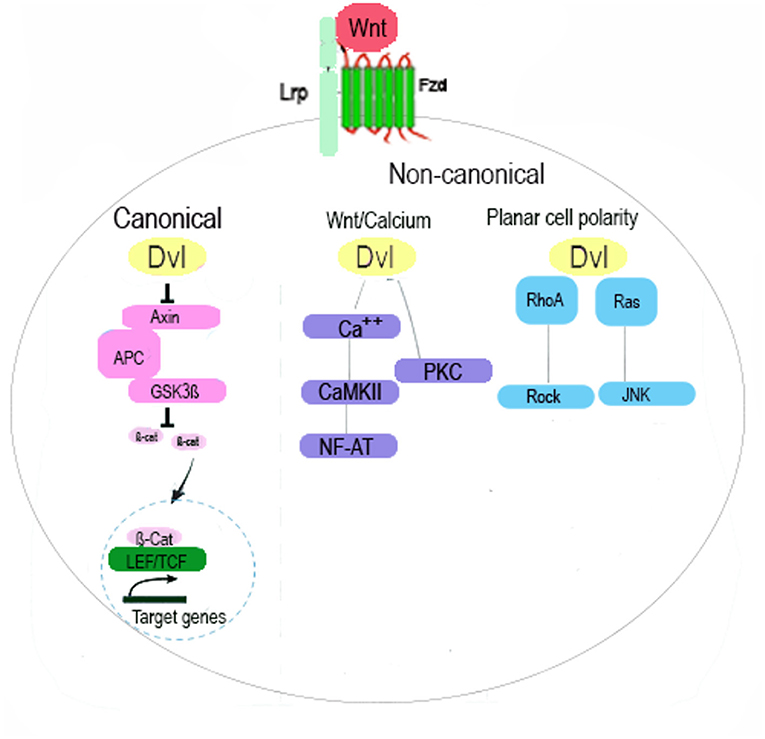
Figure 1. Schematic Illustration of Wnt Signalling Pathway. The canonical Wnt pathway, acts through Fzd and LRP5/6 receptors is mediated by β-catenin and its translocation to nucleus, activation of TCF/LEF transcription factor and regulation of gene expression. Non-canonical Wnt pathway signals through Fzd and/or ROR1/ROR2/RYK receptors and is transduced by the WNT/PCP (planar cell polarity), WNT/RTK (receptor tyrosine kinase) and WNT/Ca2+ signalling cascades. In the Wnt/Ca2+ pathway, binding of Wnt to Fzd activates DVL which then activates calcium-binding proteins including protein kinase C (PKC) and calmodulin-dependent kinase II (CamKII) and subsequently transcription factor NFAT. In the Wnt/PCP pathway, signal is mediated by GTPases RhoA and Ras and activates RhoA-Rho-associated kinase (ROCK) axis or JNK and subsequently regulation the cytoskeleton.
WNT Signalling During Tooth Development
Teeth are epithelial appendages and develop through reciprocal interaction between surface epithelium and the underlying neural crest derived mesenchyme in the developing maxillary and mandibular arches. Critical roles of various signalling pathways such as Wnt, Shh, FGF and Eda in this process has been well-established. The very specific location of tooth initiation is determined by these signalling pathways (6). Wnt/β-catenin signalling is expressed at early stages of tooth development and in the epithelial signalling centres that regulate budding and crown morphogenesis (6–9). Active β-catenin signalling is also expressed in the underlying mesenchyme and is required for epithelial morphogenesis and the induction of odontogenic fate (6, 8, 10). Similarly, late stages of tooth development and formation of the crown and roots requires a well-orchestrated network of different signalling pathways which also includes Wnt (11). It has recently been shown that Wnt inhibitor, NOTUM, is required for normal development of molar root. Ablation of Runx2, which is expressed in subpopulation of root progenitors results in down regulation of NOTUM upregulation of Wnt signalling and subsequently disruption of odontoblastic differentiation and altered root morphology (12).
Non canonical Wnt signalling pathway has also been shown to regulate root development through Receptor tyrosine kinase (RTK)-like orphan receptor 2 (Ror2) which is of the non-canonical Wnt receptors. Ror2 is expressed in dental mesenchyme and its loss results in disruption of proliferation and differentiation of mesenchymal cells and subsequently alteration in molar root size. Cdc42 required for cell cycle progression has been identified as a potential downstream mediator of Ror2 signalling in root formation (13). These findings partially explain how disruption in Wnt signalling during different stages results in various forms of developmental defects ranging from tooth agenesis to odontomas (14–17).
WNT Signalling in Tooth Homeostasis
With the many significant roles that Wnt pathway plays during different stages of tooth development, it is no surprise for it to be implicated in tooth homeostasis. In fact, odontoblasts are responsive to endogenous Wnt signals and maintain their Wnt responsiveness throughout their lifetime. The regenerative capacity of multiple mammalian tissues depends on Wnt/β-catenin signalling pathway and its activation. This has been extensively shown in murine teeth where shallow tooth damage usually results in activation of odontoblasts and formation of reactionary dentin that protects the pulp. Severe tooth damage, however, leads to odontoblast death and subsequent activation of resident dental pulp stem cells, their proliferation and differentiation into new odontoblast-like cells. These cells are then recruited to the site of damage to form reparative dentin (18, 19). This repair process is accompanied by increased Axin2 expression which results in differentiation of Axin2 expressing cells into odontoblasts-like cells. These cells are produced in the event of trauma or injury and secrete reparative dentin, a process finely tuned by the autocrine Wnt signals produced by Axin2 expressing cells (20). In addition, proliferation and apoptosis in dental pulp stem cells is regulated by Wnt signalling. Treatment of human dental pulp stem cells with liposome-reconstituted form of Wnt3A (L-WNT3A) results in increased Wnt response, enhanced mitotic activity and reduced apoptosis. Similarly, treatment of injured teeth with L-WNT3A preserves pulp vitality after acute exposure and results in elevated Wnt response and subsequently dentin regeneration (21). Various studies have demonstrated the significance of Wnt signalling pathway in tooth regeneration. Similarly, Lithium ions and Lithium Chloride (LiCl) that activate the Wnt pathway can induce tubular dentin formation. In vivo application of LiCl in rat molars results in higher β-catenin and a complete tertiary dentin (22). A number of small molecules that inhibit glycogen synthase kinase 3 (GSK3), a key enzyme in Wnt signalling pathway, have been shown to promote activation of Wnt pathway in vitro and dentin repair in mice and rats with experimental pulp exposures (23–26). Treatment of dental pulp exposures with Semaphorin 3A, promotes formation of an odontoblastic layer, dentin tubules, and predentin (27). Presence of Wnt/β-catenin signalling in dental pulp cells does not necessarily translate into promotion of odontoblast differentiation and dentin regeneration. For example, overexpression of Wnt10a significantly increases the proliferation of DPSCs, but decreases the expression of odontoblast differentiation-related genes, such as Dentin Sialophosphoprotein (DSPP), Dentin Matrix Acidic Phosphoprotein 1 (DMP1), Alkaline Phosphatase (ALP), and Collagen type 1 alpha 1 chain (COL1A1), suggesting that overexpression of Wnt10a may negatively regulate the differentiation of DPSCs into odontoblast (28, 29). Another factor that can decrease dentin regeneration is the sympathetic nervous system. Beta-2 adrenergic receptors are located in the odontoblastic layer of dental pulp in rat molars and treatment of experimental cavities with the sympatholytic beta antagonist, propranolol, results in higher tertiary dentin formation than control groups (30). Other signalling pathways such as TGF-β and BMP are also involved in formation of reparative dentin by regulation. For example, BMP-2 regulates differentiation of pulp cells to odontoblasts whilst TGF-β stimulates odontoblast differentiation and mineralisation (31–33).
Dental Pulp Stem Cells
The close anatomical and functional relationship between dentin and pulp results in formation of a dentin-pulp complex. Pulp cells contribute to the turnover of extracellular matrix and play a crucial role in the recovery of tooth damage (34, 35). Dental pulp stem cells (DPSC) demonstrate great proliferation and have great potential for a range of applications in stem cell research and regenerative medicine thanks to their ability to differentiate into various cell lineages in vitro. As an example, when subject to loading, they express tendon makers such as Scleraxis, Tenascin-C, and Collagens (36). DPSCs have recently been shown to have higher odontogenic potential than other sources of stem cells in the tooth [stem cells from the apical papilla < DPSC, periodontal stem cells (PDLSC)]. This capacity can be enhanced by supplementing cultures with 17ß-estradiol (37). When cultured on different surfaces such as plastic, hydroxyapatite and β-tricalcium phosphate, DPSCs show higher proliferation capacity and greatest osteogenic potential when compared to cells isolated from adipose tissue, and bone marrow (38).
There is an increasing interest in better understanding the capacity of DPSC differentiation into odontoblasts to explore avenues that enhance this process. Induced pluripotent stem cells have been shown to induce pulp-like tissue with the presence of tubular dentin in vivo (39). A group of unique multipotent stem cells have been identified from mouse dental papilla through three-dimensional spheroid culture. These cells ae called multipotent dental pulp regenerative stem cells and demonstrate osteogenic/odontogenic differentiation capabilities and are able to form dentin and neurovascular-like structure (40). Exogenous factors such as nitric oxide are shown to directly induce odontogenic capacity of DPSCs in rats (41). Platelet-rich plasma can promote proliferation and odontogenic differentiation of Neural Crest Stem cells derived from human dental apical papilla when used at the correct concentration (42). Further in-depth analysis of the mechanism of these processes and key singling pathways involved would be valuable. A recent study has shown that retinoid acid receptor-related orphan receptor α (RORα), is expressed in dental papilla cells and is upregulated during odontoblastic differentiation (43). RORα is also a receptor for melatonin and mediates the pro-odontogenic effect of melatonin suggesting a potential of their use in dentin regeneration.
Impact of Inflammation and Senescence on Odontoblasts
One of the factors affecting odontoblast function is senescence. Aged odontoblasts demonstrate decreased autophagic activity, accumulation of intracellular lipids, and loss of functionality (44). Aged DPSCs also demonstrate lower levels of cytoplasmic DMP1 in odontogenic differentiation, reduced contribution to mineralisation process, altered secretion of matrix metalloproteinases, and lower neurogenic differentiation potential (45–50). These changes may have an impact on formation of tertiary dentin at later stages of life. It is also worth exploring signalling pathways upstream and downstream of senescence in the tooth. Wnt signalling pathway can mediate senescence in bone marrow MSCs and its chronic activation can induce senescence in lung epithelial cells (51, 52). Regulation of senescence in odontoblasts can pave the way for new therapeutics in geriatric dentistry. Diet and metabolism have an impact on tooth homeostasis. Mice with Low Density Lipoprotein (LDL) receptor deficiency (Ldlr−/−) and on high fat diet demonstrate narrower pulp, less elongated incisor, and disappearance of predentin in incisors (53). Treating experimental pulp exposures with leptin results in angiogenesis, odontogenic differentiation and mineralisation in rat (54). Interestingly, A drug commonly used for the treatment of hyperlipidaemia, Simvastatin, has been shown to promote odontogenic differentiation and formation of new dentin (55).
Odontoblasts are immunocompetent cells and respond to bacterial components at early stages. They produce a range of antibacterial substances such as defensins, nitric oxides, chemokines, and cytokines and contribute to a staged pulpal inflammatory response (56). This response is governed by the intensity of the inflammatory reaction. Detection of microbial pathogen in odontoblast is mediated by pattern recognition receptors (PRRs) such as Toll-like receptor and the nucleotide-binding oligomerisation domain (NOD) (57). Toll-like receptors (TLR) mediate signals from components of bacterial cell wall during inflammatory reactions (58). Interestingly, Toll-like receptors have a role during development of mouse tooth germs. Activation of TLR4 inhibits mineralisation of enamel and dentin suggesting that TLR4 may decrease the mineralisation of hard tissues and trigger the maturation of ameloblasts (59).
Resident inflammatory cells in a healthy pulp also detect their environment via immune-surveillance and challenge pathogenic bacteria. In fact, macrophage populations in dental pulp are critical for dental pulp stem cell activation and formation of reparative dentin (60). Wnt signalling may play an important role in regulation of tooth inflammation. An inflamed pulp tissue exhibits increased levels of some MMPs, such as MMP2 and 3 (50, 61, 62). Wnt signalling have been shown to induce MMP expression and subsequently affect transmigration of T cell (63). GSK3, is also a key mediator of pro-inflammatory cytokine production during bacterial infections through the TLR pathway and is a potential regulator of periodontal inflammation in vitro (64). These studies suggest potential regulation of inflammation in tooth by targeting Wnt signalling.
Treatment of murine teeth with Lipopolysaccharide which is an inflammatory stimulus and Simvastatin results in angiogenesis, repressed inflammatory mediators, and increased dentin regeneration. Here Simvastatin, acts by minimising inflammatory effects and increasing regenerative potential (55). Resolvin E1 (RvE1) is a dietary omega-3 polyunsaturated fatty-acid metabolite and effective in resolving inflammation and wound healing. Treatment of experimentally induced pulp injury in rats with RvE1, demonstrated pro-healing properties, reduced necrosis of damaged pulp, and promoted formation of reparative dentin (65).
One of the key requirements during tissue regeneration is angiogenesis vascular endothelial growth factor (VEGF) family are expressed in human dental pulp and exhibit autocrine and paracrine roles in local blood vessels and immune cells. VEGF is also the most potent angiogenic and vasculogenic factor in tertiary dentin formation with positive effect on proliferation, differentiation, mineralisation, neovascularizing, and formation of reparative dentin both in vitro and in vivo (66). Lineage tracing studies have shown that during reparative dentinogenesis, odontoblasts arise from perivascular cells expressing alpha Smooth Muscle Actin (aSMA) (33).
In a carious exposure, the unique effect of pathogens is added to cellular changes secondary to inflammation. A factor that needs to be considered in tooth regeneration. Interestingly, it has been shown that expression of VEGF is higher in teeth with caries (67). This finding can be utilised in development of natural avenues for treatment of carious teeth. Comparison of ultrastructural and chemical changes that take place in arrested carious lesions demonstrate extensive remineralisation with deposition of Mg containing Hydroxyapatite crystals (Mg-Hap) in tubules of caries-arrested dentin (68). This suggests natural process of remineralisation can contribute to caries treatment a potential that can be harvested in therapeutic approaches.
Epigenetics
Epigenetic modification serves an important role in cell differentiation. Epigenetics can operate at various levels, such as interference with transcriptional and translational information through non-coding RNAs, modification of posttranslational histone cores and methylation of cytosine residues in DNA structure. Histone methylation is one of the most robust epigenetic marks and is essential for the regulation of multiple cellular processes. A recent study has shown that a short-term activation of Wnt signalling by WNT-3A induces a genomic DNA demethylation and increases histone acetylation and methylation in DPSCs, highlighting the regulation of the epigenetic barrier by Wnt signalling in DPSCs (69). Histone methyltransferases (HMTs) and histone demethylases (HDMs) are crucial for the osteogenic differentiation of human bone marrow and tooth. And histone demethylation may play an important role in reparative dentinogenesis. HDM KDM5A is an enzyme with significantly enhanced expression during cytodifferentiation in hDPCs undergoing odontogenic induction. Knocking down KDM5A in hDPCs results in greater alkaline phosphatase activity, mineral deposition and increased expression of odontogenic markers DMP1, DSPP, Zinc finger protein Osterix (OSX), and Osteocalcin (OCN) (70). DNA methylation regulates the inflammatory response of human odontoblasts in carious pulp. knocking down the DNA Methyltransferase 1 gene results in Lipoteichoic acid-induced inflammatory cytokines in human odontoblast-like cells (71). Acetylation governs differentiation and de-differentiation potential of DPSCs. A range of Histone deacetylases (HDACs) are expressed in dentin-pulp complex that regulate odontoblasts differentiation (72, 73). As an example, inhibition of HDAC4 and HDAC5, increases odontoblastic gene expression and promote the odontoblast induction (74). HDAC6 regulates the fusion of autophagosomes and lysosome during odontoblast differentiation. Decreased autophagy results in downregulation of odontoblastic differentiation capacity (75).
WNT Signalling in Periodontal Homeostasis and Disease
Periodontitis is a chronic inflammatory condition of tooth supporting tissues that results in loss of tissue attachment. This condition has also been linked to many systemic conditions such as diabetes, rheumatoid arthritis and cognitive impairment (76, 77).
GSK3 has been shown as a potential regulator of periodontal inflammation in vitro (64). Systemic administration of GSK3β inhibitors in vivo result in abrogation of bacterial-induced bone loss. A recent murine study has demonstrated that differentiation of cementoblasts producing the mineralised tissue in the root, contributes to maintenance of periodontal tissue attachment as well as its restoration in the event of periodontitis. Different populations of stem cells contribute to cementoblasts differentiation at various stages of life. Perivascular-derived cells expressing CD90 and perivascular-associated cells that express Axin2 contribute to cementoblasts during post-natal development. Whereas, during adult homeostasis, cementoblast are formed from responsive Axin2+ cells. Contribution of CD90 expressing cells to cementoblast differentiation occurs only upon induction of periodontitis (78). These findings have great clinical implication and emphasise the crucial role of Wnt signalling in homeostasis of tooth and its periodontium. These roles of Wnt signalling are summarised in table and schematic in the Figure 2.
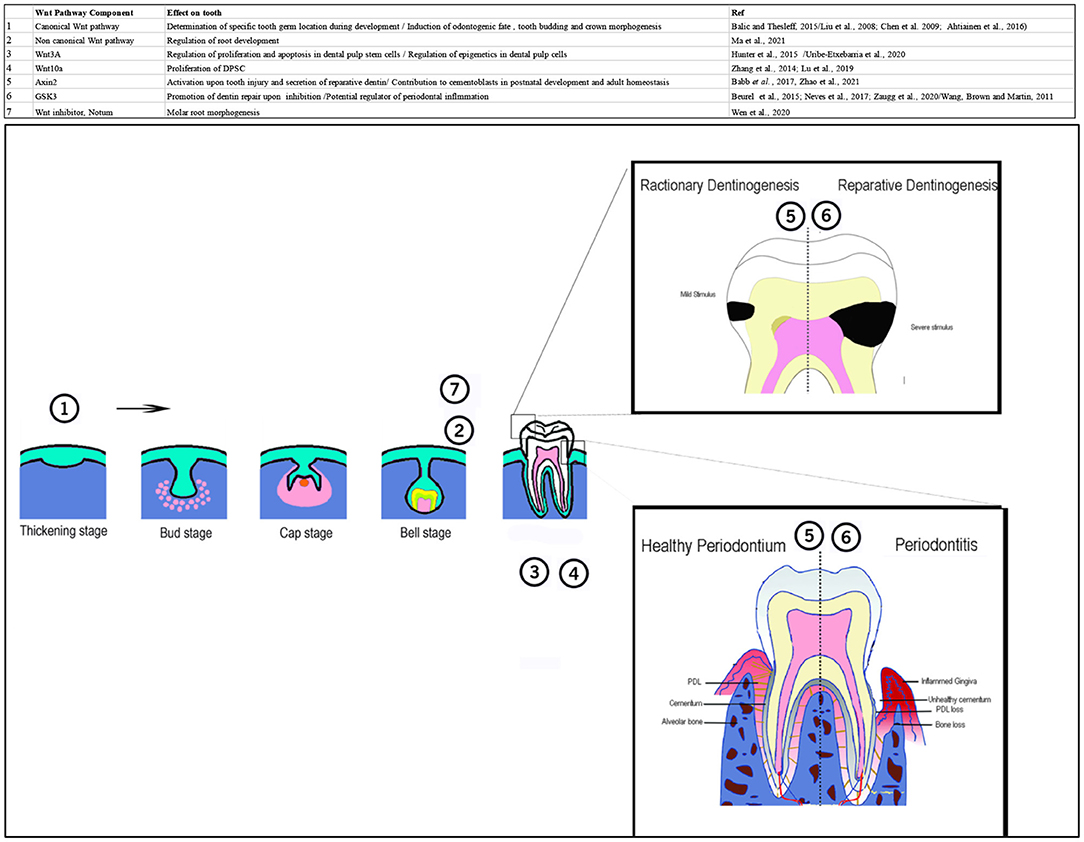
Figure 2. Table and schematic, summarising different roles of Wnt pathway during development, homeostasis, and regeneration of tooth and periodontium. Numbers in the schematic corresponds to the number of pathway component in the table. 1: canonical Wnt pathway is involved in determination of specific tooth germ location during development and induction of odontogenic fate, tooth budding and crown morphogenesis. 2: Noncanonical Wnt pathway regulates root development. 3: Wnt3A regulates proliferation and apoptosis in dental pulp stem cells. It is also involved in the epigenetic regulation of DPSCs. 4: Wnt10 regulates proliferation of DPSCs. 5: Axin2+ cells are activated upon tooth injury and induce secretion of reparative dentin. In the periodontium these cells contribute to cementoblasts formation during postnatal development and adult homeostasis. 6; Inhibition of GSK3 promotes dentin repair. It is a potential regulator of periodontal inflammation. 7: Notum, Wnt inhibitor is involved in molar root morphogenesis. A part of this schematic was adapted from Xu et al. (79).
The development of a new generation of dental therapies on biological-based approaches is now a major goal in regenerative dentistry. Our understanding of dental tissue regeneration is advancing but needs to be combined with a compatible and practical delivery system to be translated into clinical application. Advanced biomaterials and technology can be used to modulate tissue microenvironment and enhance the efficiency of regeneration process. This has been shown in the use of low level laser and magnetic fields to enhance DPCs differentiation as well as novel imaging techniques to visualise dental pulp using tissue clearing method (80–84). However, these advancements need to be tested for their accessibility and delivery. Development of suitable scaffolds that can stimulate and guide stem cell differentiation in dental tissues is equally important and can serve as delivery vehicle.
ReDent (Regeneration of Dentine) allows delivery of small concentration of a novel GSK3 small molecule inhibitor drug via hydrogel into tooth cavity and results in rapid stimulation of resident DPSCs to proliferate and differentiate into odontoblast-like cells that produce reparative dentine (85). LithGlass is a novel glass ionomer formulation specifically designed to rapidly release lithium ions to stimulate odontoblast activity in non-exposed pulp lesions. The reactionary dentin produced restores original dentin thickness (86). Both ReDent and LithGlass represent simple, affordable solutions to improve dental care and hopefully form part of the new vanguard driving regenerative dentistry.
Author Contributions
All authors listed have made a substantial, direct and intellectual contribution to the work, and approved it for publication.
Funding
Original research from the Sharpe lab was funded by the Medical Research Council (UKRI).
Conflict of Interest
The authors declare that the research was conducted in the absence of any commercial or financial relationships that could be construed as a potential conflict of interest.
Publisher's Note
All claims expressed in this article are solely those of the authors and do not necessarily represent those of their affiliated organizations, or those of the publisher, the editors and the reviewers. Any product that may be evaluated in this article, or claim that may be made by its manufacturer, is not guaranteed or endorsed by the publisher.
References
1. Komiya Y, Habas R. Wnt signal transduction pathways. Organogenesis. (2008) 4:68–75. doi: 10.4161/org.4.2.5851
2. Gordon MD, Nusse R. Wnt signaling: multiple pathways, multiple receptors, and multiple transcription factors. J Biol Chem. (2006) 281:22429–33. doi: 10.1074/jbc.R600015200
3. Fuerer C, Nusse R, ten Berge D. Wnt signalling in development and disease: max delbrück center for molecular medicine meeting on Wnt signaling in development and disease. EMBO Rep. (2008) 9:134–8. doi: 10.1038/sj.embor.7401159
4. Anastas JN, Moon RT. WNT signalling pathways as therapeutic targets in cancer. Nat Rev Cancer. (2013) 13:11–26. doi: 10.1038/nrc3419
5. Katoh M. Canonical and non-canonical WNT signaling in cancer stem cells and their niches: cellular heterogeneity, omics reprogramming, targeted therapy and tumor plasticity (Review). Int J Oncol. (2017) 51:1357–69. doi: 10.3892/ijo.2017.4129
6. Balic A, Thesleff I. Tissue interactions regulating tooth development and renewal. Curr Topics Dev Biol. (2015) 115:157–86. doi: 10.1016/bs.ctdb.2015.07.006
7. Sarkar L, Sharpe PT. Expression of Wnt signalling pathway genes during tooth development. Mech Dev. (1999) 85:197–200. doi: 10.1016/S0925-4773(99)00095-7
8. Liu F, Chu EY, Watt B, Zhang Y, Gallant NM, Andl T, et al. Wnt/beta-catenin signaling directs multiple stages of tooth morphogenesis. Dev Biol. (2008) 313:210–24. doi: 10.1016/j.ydbio.2007.10.016
9. Ahtiainen L, Uski I, Thesleff I, Mikkola ML. Early epithelial signaling center governs tooth budding morphogenesis. J Cell Biol. (2016) 214:753–67. doi: 10.1083/jcb.201512074
10. Chen J, Lan Y, Baek J-A, Gao Y, Jiang R. Wnt/beta-catenin signaling plays an essential role in activation of odontogenic mesenchyme during early tooth development. Dev Biol. (2009) 334:174–85. doi: 10.1016/j.ydbio.2009.07.015
11. Thesleff I, Sharpe P. Signalling networks regulating dental development. Mech Dev. (1997) 67:111–23. doi: 10.1016/S0925-4773(97)00115-9
12. Wen Q, Jing J, Han X, Feng J, Yuan Y, Ma Y, et al. Runx2 regulates mouse tooth root development via activation of Wnt inhibitor NOTUM. J Bone Miner Res. (2020) 35:2252–64. doi: 10.1002/jbmr.4120
13. Ma Y, Jing J, Feng J, Yuan Y, Wen Q, Han X, et al. Ror2-mediated non-canonical Wnt signaling regulates Cdc42 and cell proliferation during tooth root development. Development. (2021) 148:1–12. doi: 10.1242/dev.196360
14. Fujii S, Nagata K, Matsumoto S, Kohashi K ichi, Kikuchi A, Oda Y, et al. Wnt/β-catenin signaling, which is activated in odontomas, reduces Sema3A expression to regulate odontogenic epithelial cell proliferation and tooth germ development. Sci Rep. (2019) 9:4257. doi: 10.1038/s41598-019-39686-1
15. Abid MF, Simpson MA, Barbosa IA, Seppala M, Irving M, Sharpe PT, et al. WNT10A mutation results in severe tooth agenesis in a family of three sisters. Orthod Craniofacial Res. (2018) 21:153–9. doi: 10.1111/ocr.12231
16. Kantaputra PN, Hutsadaloi A, Kaewgahya M, Intachai W, German R, Koparal M, et al. WNT10B mutations associated with isolated dental anomalies. Clin Genet. (2018) 93:992–9. doi: 10.1111/cge.13218
17. Järvinen E, Shimomura-Kuroki J, Balic A, Jussila M, Thesleff I. Mesenchymal Wnt/β-catenin signaling limits tooth number. Development. (2018) 145:dev158048. doi: 10.1242/dev.158048
18. Couve E, Osorio R, Schmachtenberg O. Reactionary dentinogenesis and neuroimmune response in dental caries. J Dent Res. (2014) 93:788–93. doi: 10.1177/0022034514539507
19. Smith AJ, Cassidy N, Perry H, Bègue-Kirn C, Ruch J V, Lesot H. Reactionary dentinogenesis. Int J Dev Biol. (1995) 39:273–80.
20. Babb R, Chandrasekaran D, Carvalho Moreno Neves V, Sharpe PT. Axin2-expressing cells differentiate into reparative odontoblasts via autocrine Wnt/β-catenin signaling in response to tooth damage. Sci Rep. (2017) 7:3102. doi: 10.1038/s41598-017-03145-6
21. Hunter DJ, Bardet C, Mouraret S, Liu B, Singh G, Sadoine J, et al. Wnt acts as a prosurvival signal to enhance dentin regeneration. J Bone Miner Res. (2015) 30:1150–9. doi: 10.1002/jbmr.2444
22. Ishimoto K, Hayano S, Yanagita T, Kurosaka H, Kawanabe N, Itoh S, et al. Topical application of lithium chloride on the pulp induces dentin regeneration. PLoS ONE. (2015) 10:e0121938. doi: 10.1371/journal.pone.0121938
23. Beurel E, Grieco SF, Jope RS. Glycogen synthase kinase-3 (GSK3): regulation, actions, and diseases. Pharmacol Ther. (2015) 148:114–31. doi: 10.1016/j.pharmthera.2014.11.016
24. Birjandi AA, Suzano FR, Sharpe PT. Drug repurposing in dentistry; towards application of small molecules in dentin repair. Int J Mol Sci. (2020) 21:6394. doi: 10.3390/ijms21176394
25. Neves VCM, Babb R, Chandrasekaran D, Sharpe PT. Promotion of natural tooth repair by small molecule GSK3 antagonists. Sci Rep. (2017) 7:39654. doi: 10.1038/srep39654
26. Zaugg LK, Banu A, Walther AR, Chandrasekaran D, Babb RC, Salzlechner C, et al. Translation approach for dentine regeneration using GSK-3 antagonists. J Dent Res. (2020) 99:544–51. doi: 10.1177/0022034520908593
27. Yoshida S, Wada N, Hasegawa D, Miyaji H, Mitarai H, Tomokiyo A, et al. Semaphorin 3A induces odontoblastic phenotype in dental pulp stem cells. J Dent Res. (2016) 95:1282–90. doi: 10.1177/0022034516653085
28. Lu X, Yang J, Zhao S, Liu S. Advances of Wnt signalling pathway in dental development and potential clinical application. Organogenesis. (2019) 15:101–10. doi: 10.1080/15476278.2019.1656996
29. Zhang Z, Guo Q, Tian H, Lv P, Zhou C, Gao X. Effects of WNT10A on proliferation and differentiation of human dental pulp cells. J Endod. (2014) 40:1593–9. doi: 10.1016/j.joen.2014.07.009
30. Gu J, Ikeda H, Suda H. Sympathetic regulation of tertiary dentinogenesis via beta-2 adrenergic receptor on rat odontoblasts. J Endod. (2015) 41:1056–60. doi: 10.1016/j.joen.2015.01.010
31. Niwa T, Yamakoshi Y, Yamazaki H, Karakida T, Chiba R, Hu JCC, et al. The dynamics of TGF-β in dental pulp, odontoblasts and dentin. Sci Rep. (2018) 8:4450. doi: 10.1038/s41598-018-22823-7
32. Tóth F, Gáll JM, Tozsér J, Hegedus C. Effect of inducible bone morphogenetic protein 2 expression on the osteogenic differentiation of dental pulp stem cells in vitro. Bone. (2020) 132:115214. doi: 10.1016/j.bone.2019.115214
33. Ahmed GM, Abouauf EA, Abubakr N, Dörfer CE, El-Sayed KF. Tissue engineering approaches for enamel, dentin, and pulp regeneration: an update. Stem Cells Int. (2020) 2020:5734539. doi: 10.1155/2020/5734539
34. Pagella P, Neto E, Lamghari M, Mitsiadis T. Investigation of orofacial stem cell niches and their innervation through microfluidic devices. Eur Cells Mater. (2015) 29:213–23. doi: 10.22203/eCM.v029a16
35. Shimabukuro Y, Ueda M, Ozasa M, Anzai J, Takedachi M, Yanagita M, et al. Fibroblast growth factor−2 regulates the cell function of human dental pulp cells. J Endod. (2009) 35:1529–35. doi: 10.1016/j.joen.2009.08.010
36. Chen Y-Y, He S-T, Yan F-H, Zhou P-F, Luo K, Zhang Y-D, et al. Dental pulp stem cells express tendon markers under mechanical loading and are a potential cell source for tissue engineering of tendon-like tissue. Int J Oral Sci. (2016) 8:213–22. doi: 10.1038/ijos.2016.33
37. Son YB, Kang YH, Lee HJ, Jang SJ, Bharti D, Lee SL, et al. Evaluation of odonto/osteogenic differentiation potential from different regions derived dental tissue stem cells and effect of 17β-estradiol on efficiency. BMC Oral Health. (2021) 21:15. doi: 10.1186/s12903-020-01366-2
38. Mucientes A, Herranz E, Moro E, Gonz A. Influence of mesenchymal stem cell sources on their regenerative capacities on different surfaces. Cells. (2021) 10:481. doi: 10.3390/cells10020481
39. Xie H, Dubey N, Shim W, Ramachandra CJA, Min KS, Cao T, et al. Functional odontoblastic-like cells derived from human iPSCs. J Dent Res. (2018) 97:77–83. doi: 10.1177/0022034517730026
40. Chen H, Fu H, Wu X, Duan Y, Zhang S, Hu H, et al. Regeneration of pulpo-dentinal-like complex by a group of unique multipotent CD24a+ stem cells. Sci Adv. (2020) 6:eaay1514. doi: 10.1126/sciadv.aay1514
41. Sonoda S, Mei YF, Atsuta I, Danjo A, Yamaza H, Hama S, et al. Exogenous nitric oxide stimulates the odontogenic differentiation of rat dental pulp stem cells. Sci Rep. (2018) 8:3419. doi: 10.1038/s41598-018-21183-6
42. Li J, Xiang L, Guan C, Yang X, Hu X, Zhang X, et al. Effects of platelet-rich plasma on proliferation, viability, and odontogenic differentiation of neural crest stem-like cells derived from human dental apical papilla. Biomed Res Int. (2020) 2020:4671989. doi: 10.1155/2020/4671989
43. Kang J, Chen H, Zhang F, Yan T, Fan W, Jiang L, et al. RORα regulates odontoblastic differentiation and mediates the pro-odontogenic effect of melatonin on dental papilla cells. Molecules. (2021) 26:1098. doi: 10.3390/molecules26041098
44. Couve E, Schmachtenberg O. Autophagic activity and aging in human odontoblasts. J Dent Res. (2011) 90:523–8. doi: 10.1177/0022034510393347
45. Feng X, Xing J, Feng G, Huang D, Lu X, Liu S, et al. p16INK4A mediates age-related changes in mesenchymal stem cells derived from human dental pulp through the DNA damage and stress response. Mech Ageing Dev. (2014) 141–2:46–55. doi: 10.1016/j.mad.2014.09.004
46. Yi Q, Liu O, Yan F, Lin X, Diao S, Wang L, et al. Analysis of senescence-related differentiation potentials and gene expression profiles in human dental pulp stem cells. Cells Tissues Organs. (2017) 203:1–11. doi: 10.1159/000448026
47. Iezzi I, Cerqueni G, Licini C, Lucarini G, Mattioli Belmonte M. Dental pulp stem cells senescence and regenerative potential relationship. J Cell Physiol. (2019) 234:7186–97. doi: 10.1002/jcp.27472
48. Coppé J-P, Desprez P-Y, Krtolica A, Campisi J. The senescence-associated secretory phenotype: the dark side of tumor suppression. Annu Rev Pathol Mech Dis. (2010) 5:99–118. doi: 10.1146/annurev-pathol-121808-102144
49. Feng X, Xing J, Feng G, Sang A, Shen B, Xu Y, et al. Age-Dependent impaired neurogenic differentiation capacity of dental stem cell is associated with Wnt/β-catenin signaling. Cell Mol Neurobiol. (2013) 33:1023–31. doi: 10.1007/s10571-013-9965-0
50. Iezzi I, Pagella P, Mattioli-Belmonte M, Mitsiadis TA. The effects of ageing on dental pulp stem cells, the tooth longevity elixir. Euro Cells Mater. (2019) 37:175–85. doi: 10.22203/eCM.v037a11
51. Gu Z, Tan W, Feng G. Wnt/b -catenin signaling mediates the senescence of bone marrow-mesenchymal stem cells from systemic lupus erythematosus patients through the p53/p21 pathway. Mol Cell Biochem. (2014) 387:27–37. doi: 10.1007/s11010-013-1866-5
52. Lehmann M, Hu Q, Hu Y, Hafner K, Costa R, Berg A Van Den, et al. Chronic WNT / β -catenin signaling induces cellular senescence in lung epithelial cells. Euro Res J. (2020) 54:PA4122. doi: 10.1183/13993003.congress-2019.PA4122
53. Kurotaki Y, Sakai N, Miyazaki T, Hosonuma M, Sato Y, Karakawa A, et al. Effects of lipid metabolism on mouse incisor dentinogenesis. Sci Rep. (2020) 10:5102. doi: 10.1038/s41598-020-61978-0
54. Choi SH, Jang JH, Koh JT, Chang HS, Hwang YC, Hwang IN, et al. Effect of leptin on odontoblastic differentiation and angiogenesis: an in vivo study. J Endod. (2019) 45:1332–41. doi: 10.1016/j.joen.2019.08.003
55. Soares DG, Zhang Z, Mohamed F, Eyster TW, de Souza Costa CA, Ma PX. Simvastatin and nanofibrous poly(L-lactic acid) scaffolds to promote the odontogenic potential of dental pulp cells in an inflammatory environment. Acta Biomater. (2018) 68:190–203. doi: 10.1016/j.actbio.2017.12.037
56. Fawzy El-Sayed KM, Elsalawy R, Ibrahim N, Gadalla M, Albargasy H, Zahra N, et al. The dental pulp stem/progenitor cells-mediated inflammatory-regenerative axis. Tissue Eng Part B Rev. (2019) 25:445–60. doi: 10.1089/ten.teb.2019.0106
57. Yumoto H, Hirao K, Hosokawa Y, Kuramoto H, Takegawa D, Nakanishi T, et al. The roles of odontoblasts in dental pulp innate immunity. Jpn Dent Sci Rev. (2018) 54:105–17. doi: 10.1016/j.jdsr.2018.03.001
58. Hosokawa Y, Hirao K, Yumoto H, Washio A, Nakanishi T, Takegawa D, et al. Functional roles of NOD1 in odontoblasts on dental pulp innate immunity. Biomed Res Int. (2016) 2016:9325436. doi: 10.1155/2016/9325436
59. Papp T, Hollo K, Meszar-Katona E, Nagy Z, Polyak A, Miko E, et al. TLR signalling can modify the mineralisation of tooth germ. Acta Odontol Scand. (2016) 74:307–14. doi: 10.3109/00016357.2015.1130853
60. Neves VCM, Yianni V, Sharpe PT. Macrophage modulation of dental pulp stem cell activity during tertiary dentinogenesis. Sci Rep. (2020) 10:20216. doi: 10.1038/s41598-020-77161-4
61. Shin SJ, JI L, SH B, Lim S. Tissue levels of matrix metalloproteinases in pulps and periapical lesions. J Endod. (2002) 28:313–5. doi: 10.1097/00004770-200204000-00013
62. Goda S, Kato Y, Domae E, Hayashi H, Tani-Ishii N, Iida T. Effects of JNK1/2 on the inflammation cytokine TNF-α-enhanced production of MMP-3 in human dental pulp fibroblast-like cells. Int Endod J. (2014) 48:1122–8. doi: 10.1111/iej.12411
63. Wu B, Crampton SP, Hughes CCW. Article Wnt signaling induces matrix metalloproteinase expression and regulates T cell transmigration. Immunity. (2007) 26:227–39. doi: 10.1016/j.immuni.2006.12.007
64. Wang H, Brown J, Martin M. Glycogen synthase kinase 3: a point of convergence for the host inflammatory response. Cytokine. (2011) 53:130–40. doi: 10.1016/j.cyto.2010.10.009
65. Chen J, Xu H, Xia K, Cheng S, Zhang Q. Resolvin E1 accelerates pulp repair by regulating inflammation and stimulating dentin regeneration in dental pulp stem cells. Stem Cell Res Ther. (2021) 12:75. doi: 10.1186/s13287-021-02141-y
66. Zhang J, Liu X, Yu W, Zhang Y, Shi C, Ni S, et al. Effects of human vascular endothelial growth factor on reparative dentin formation. Mol Med Rep. (2016) 13:705–12. doi: 10.3892/mmr.2015.4608
67. Chen Y, Li X, Wu J, Lu W, Xu W, Wu B. Dental pulp stem cells from human teeth with deep caries displayed an enhanced angiogenesis potential in vitro. J Dent Sci. (2020) 16:318–26. doi: 10.1016/j.jds.2020.03.007
68. Yoshihara K, Nagaoka N, Nakamura A, Hara T, Hayakawa S, Yoshida Y, et al. Three-dimensional observation and analysis of remineralisation in dentinal caries lesions. Sci Rep. (2020) 10:4387. doi: 10.1038/s41598-020-61111-1
69. Uribe-Etxebarria V, García-Gallastegui P, Pérez-Garrastachu M, Casado-Andrés M, Irastorza I, Unda F, et al. Wnt-3a induces epigenetic remodeling in human dental pulp stem cells. Cells. (2020) 9:652. doi: 10.3390/cells9030652
70. Li Q, Li J, Feng Z, Lin H, Xu Q. Effect of histone demethylase KDM5A on the odontogenic differentiation of human dental pulp cells. Bioengineered. (2020) 11:449–62. doi: 10.1080/21655979.2020.1743536
71. Meng R, Li D, Feng Z, Xu Q. MyD88 hypermethylation mediated by DNMT1 is associated with LTA-induced inflammatory response in human odontoblast-like cells. (2019). 413–23. doi: 10.1007/s00441-019-02993-0
72. Yamauchi Y, Cooper PR, Shimizu E, Kobayashi Y, Smith AJ, Duncan HF. Histone acetylation as a regenerative target in the dentine-pulp complex. Front Genet. (2020) 11:1. doi: 10.3389/fgene.2020.00001
73. Tao H, Li Q, Lin Y, Zuo H, Cui Y, Chen S, et al. Coordinated expression of p300 and HDAC3 upregulates histone acetylation during dentinogenesis Huangheng. J Cell Biochem. (2020). 121:2478–88. doi: 10.1002/jcb.29470
74. Liu Z, Chen T, Han Q, Chen M, You JIE, Fang F, et al. HDAC inhibitor LMK - 235 promotes the odontoblast differentiation of dental pulp cells. Mol Med Rep. (2018). 1445–52. doi: 10.3892/mmr.2017.8055
75. Zhan Y, Wang H, Zhang L, Pei F, Chen Z. HDAC6 regulates the fusion of autophagosome and lysosome to involve in odontoblast differentiation. Front Cell Dev Biol. (2020) 8:605609. doi: 10.3389/fcell.2020.605609
76. Vitkov L, Hannig M, Minnich B, Herrmann M. Periodontal sources of citrullinated antigens and TLR agonists related to RA. Autoimmunity. (2018) 51:304–9. doi: 10.1080/08916934.2018.1527907
77. Xue L, Zou X, Yang XQ, Peng F, Yu DK, Du JR. Chronic periodontitis induces microbiota-gut-brain axis disorders and cognitive impairment in mice. Exp Neurol. (2020) 326:113176. doi: 10.1016/j.expneurol.2020.113176
78. Zhao J, Faure L, Adameyko I, Sharpe PT. Stem cell contributions to cementoblast differentiation in healthy periodontal ligament and periodontitis. Stem Cells. (2021) 39:92–102. doi: 10.1002/stem.3288
79. Xu X, Li X, Wang J, He X, Sun H, Chen F. Concise review: periodontal tissue regeneration using stem cells: strategies and translational considerations. Stem Cells Transl Med. (2019) 8:392–403. doi: 10.1002/sctm.18-0181
80. Kim HB, Baik KY, Seonwoo H, Jang KJ, Lee MC, Choung PH, et al. Effects of pulsing of light on the dentinogenesis of dental pulp stem cells in vitro. Sci Rep. (2018) 8:2057. doi: 10.1038/s41598-018-19395-x
81. El Nawam H, El Backly R, Zaky A, Abdallah A. Low-level laser therapy affects dentinogenesis and angiogenesis of in vitro 3D cultures of dentin-pulp complex. Lasers Med Sci. (2019) 34:1689–89. doi: 10.1007/s10103-019-02804-6
82. Lew WZ, Feng SW, Lin CT, Huang HM. Use of 0.4-Tesla static magnetic field to promote reparative dentine formation of dental pulp stem cells through activation of p38 MAPK signalling pathway. Int Endod J. (2019) 52:28–43. doi: 10.1111/iej.12962
83. Chang K, Chen RS, Chang FH, Chen MH. Promoting dentinogenesis of DPSCs through inhibiting microRNA-218 by using magnetic nanocarrier delivery. J Formos Med Assoc. (2019) 118:1005–13. doi: 10.1016/j.jfma.2018.10.018
84. França CM, Riggers R, Muschler JL, Widbiller M, Lococo PM, Diogenes A, et al. 3D-imaging of whole neuronal and vascular networks of the human dental pulp via CLARITY and light sheet microscopy. Sci Rep. (2019) 9:5899. doi: 10.1038/s41598-019-47221-5
85. Alaohali A, Salzlechner C, Zaugg LK, Suzano F, Martinez A, Gentleman E, et al. GSK3 inhibitor-induced dentinogenesis using a hydrogel. J Dent Res. (2021) 002203452110206. doi: 10.1177/00220345211020652. [Epub ahead of print].
Keywords: tooth development, regeneration, adult stem cells, reparative dentinogenesis, reactionary dentin
Citation: Birjandi AA and Sharpe P (2021) Wnt Signalling in Regenerative Dentistry. Front. Dent. Med. 2:725468. doi: 10.3389/fdmed.2021.725468
Received: 15 June 2021; Accepted: 30 July 2021;
Published: 30 August 2021.
Edited by:
Catherine Chaussain, Université de Paris, FranceReviewed by:
Brigitte Alliot-licht, Université de Nantes, FranceMarco Bottino, University of Michigan, United States
Copyright © 2021 Birjandi and Sharpe. This is an open-access article distributed under the terms of the Creative Commons Attribution License (CC BY). The use, distribution or reproduction in other forums is permitted, provided the original author(s) and the copyright owner(s) are credited and that the original publication in this journal is cited, in accordance with accepted academic practice. No use, distribution or reproduction is permitted which does not comply with these terms.
*Correspondence: Paul Sharpe, cGF1bC5zaGFycGUmI3gwMDA0MDtrY2wuYWMudWs=