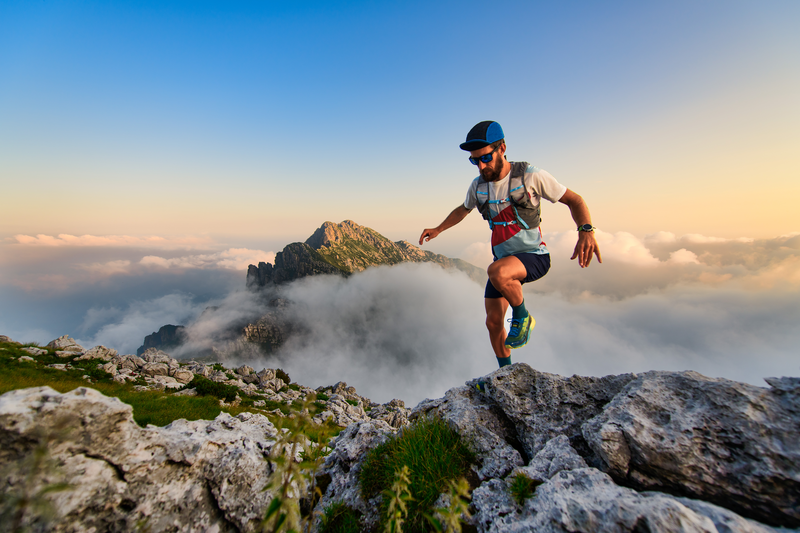
94% of researchers rate our articles as excellent or good
Learn more about the work of our research integrity team to safeguard the quality of each article we publish.
Find out more
MINI REVIEW article
Front. Dent. Med , 13 August 2021
Sec. Dental Materials
Volume 2 - 2021 | https://doi.org/10.3389/fdmed.2021.687983
This article is part of the Research Topic Biofunctional Applications of Zirconia in Dental Devices View all 5 articles
Titanium is currently the most commonly used material for manufacturing dental implants. However, its potential toxic effects and the gray color have resulted in increasing requests for metal-free treatment options. Zirconia is a type of ceramic materials that has been extensively used in medicine field, such as implant abutments and various joint replacement appliances. Amounts of clinical evaluations have indicated good biocompatibility for zirconia products. Besides, its toothlike color, low affinity for plaque and outstanding mechanical and chemical properties have made it an ideal candidate for dental implants. The aim of this study is to review the laboratory and clinical papers about several kinds of zirconia materials and zirconia surface modification techniques. Although there are plenty of literatures on these topics, most of the researches focused on the mechanical properties of the materials or based on cell and animal experiments. Randomized clinical trials on zirconia materials are still urgently needed to validate their application as dental implants.
Over the past few decades, dental implant procedures were applied to support single crowns as well as fixed or removable partial or full dentures. Early osseointegration is a critical factor for the clinical success of oral implants. Titanium showed a high biocompatibility, resistance to corrosion and favorable mechanical properties, these characteristics were particularly relevant in the early osseointegration of the healing period. Nevertheless, failures of titanium implants were hard to avoid. To improve titanium surfaces, several methods have been used, one of these methods was to deposit a coating on the surface of titanium.
Zirconia was first introduced to implant dentistry in the form of coatings to improve osseointegration of the titanium implants (1). As people moved toward further exploration, the unique characteristics of zirconia such as superior toughness, strength, fatigue resistance and corrosion resistance made it an ideal implant material (2). Besides, clinicians and patients started to reconsider about the safety of titanium implants after the accumulation of titanium particles was detected in tissues close to the implants and local lymph nodes (3). Some reported about the allergy caused by the application of titanium implants (4). As of now, there is no report about the toxicity of zirconia implants. Another advantage of zirconia is its tooth-like color. To meet with esthetic needs from patients, the ivory color offers a possibility of staining zirconia with tooth or gingival colors, which elevates the esthetic scores and leads to patient satisfactions (5).
The successful use of zirconia as a material for dental implants is mainly contributed by its excellent osseointegration behavior. According to the in vivo studies, zirconia has shown predictable osseointegration, cell metabolism and positive tissue response, some results were taken out under loaded conditions (6, 7). Furthermore, the inflammatory response and bone loss induced by zirconia implants are much less than those induced by titanium implants, suggesting the good biocompatibility of zirconia material (8–11). In the quest to achieve more predictable osseointegration, especially in difficult clinical sites, researchers have attempted to modify zirconia surface to make it desirable for a better cell attachment, growth, proliferation and differentiation. The methods on improving zirconia surfaces were reported as preclinical results, most of the surface modification procedures promoted a superior cell response. The purpose of this study is to perform a literature review on different kinds of zirconia for dental implants and surface modification techniques of zirconia implants, analyze their behavior via preclinical evaluations and clinical trials.
When people started to explore whether ceramic implants are alternative to titanium implants, alumina implants were shortly used as an implant material. But clinical investigations using different alumina oral implants showed poor survival rates for different indications (12). Thereafter, biomedical grade zirconia was introduced to solve the problem caused by the fracture or potential failure of alumina implants (13). One of the advantages of zirconia is that the phase transformation inside the material increases its crack propagation resistance. However, the metastability character of zirconia also causes its aging in the presence of water (14). The consequences of aging did not cause manufacturers' attention until the year 2001, 400 femoral heads made of zirconia failed in a very short period (15). After that, researchers expended considerable efforts seeking to resolve this problem. One of the approaches was to add oxides to stabilize the crystal structure transformation during firing at an elevated temperature and improve the physical properties of zirconia.
As mentioned above, zirconia can undergo a phase transformation at different temperatures. Upon heating, the monoclinic phase of zirconia starts transforming to the tetragonal phase. On cooling, the transformation is from the tetragonal to the monoclinic phase. A disadvantage of the phase transformation is that during the transformation, there is a 4% volume change, which results in the formation of ceramic cracks. It is known that the phase transformation can be induced by stress, heat and high energy heavy ion irradiation, but the mechanism is not clear yet (16–22). Oxides such as ceria, yttria, alumina, magnesia and calcia have been used to stabilize the structure of zirconia when calcination temperatures changes. The mechanism of the stabilization is to stop tetragonal phases from transforming into monoclinic phase under normal cooling conditions, and consequently, crack formation, due to volume changes, is avoided (2). In this review, Y-TZP (Yttria-stabilized Tetragonal Zirconia Polycrystal ceramics) and NANOZR (ceria-stabilized zirconia/alumina nanocomposite) are included for their wide application as dental implant materials.
Currently the material most often chosen for ceramic dental implants is Y-TZP. Y-TZP has a clear benefit to the aging resistance, as well as preserving good toughness and strength. Y-TZP retains some promising physical and mechanical properties, including low thermal conductivity, high flexural strength (900–1,200 MPa), favorable fracture resistance, as well as wear and corrosion resistance. Besides, an advantage of the phase transformation is that due to the unit cell of monoclinic configuration occupies about 4% more volume than the tetragonal configuration, the volume increase essentially squeezes the crack to close and thus increases its toughness. The surface toughening further improves the properties of Y-TZP (23). As a kind of implant material, clinicians always pay close attentions on its resistance to fracture. In Silva's research (24), titanium implant with titanium abutment, titanium implant with zirconia abutment and one-piece zirconia implant were tested to determine their fracture resistance to impact load. The result of their experiment was that the fracture energy for all three test groups was not statistically significant, ranging from 3.2 to 5 J. The results are nearly three times higher than the energy needed to fracture the most resistant natural teeth (maxillary cuspids), which was reported ~1.26 J. Therefore, we can assume that a patient receiving a traumatic injury would result in fracture of a natural tooth, but not fracture of a zirconia implant or abutment.
However, a negative property of zirconia, which is described as low-temperature degradation (LTD) or aging, results in large decreases in strength, toughness and density. The LTD can be summarized as a slow transformation from the tetragonal phase into the monoclinic phase. The phase transformation is greatly enhanced by the presence of water or water vapor and accompanied by micro- and macrocracking, which leads to progressive deterioration of the material (25). Nowadays, several studies have been performed on the LTD phenomenon, but these are still in need of further investigations.
To avoid the low-temperature degradation, a ceria-stabilized zirconia/alumina nanocomposite (NANOZR) was introduced to dentistry. NANOZR is highly resistant to low-temperature degradation and therefore suitable for load-bearing applications such as dental implants. It also exhibits greater flexural strength and fracture toughness when comperes with Y-TZP (26). According to some studies, NANOZR is a good candidate implant material because of its good osseointegration capacity (27, 28).
Polishing is to use silicon carbide polishing paper and diamond suspension with a polishing machine. The procedure usually results in a very smooth surface even observed using a microscope. It is known that epithelial cells are more likely to attach to a smooth polished surface than to a rough surface, and a smooth surface is a favorable circumstance for epithelial cell proliferation (29). Oral keratinocyte is a kind of highly specialized epithelial cell. According to Kimura's study, when detecting the responses of keratinocytes to an implant material, a polishing surface of zirconia made it comparable to titanium (30).
Sandblasting and acid etching are most common procedures using for implant surface modification. One of the advantages is that this treatment process can increase the surface area of implants for osseointegration (31). Another is that rougher surfaces have been proved to enhance osteoblast adhesion and proliferation (32). For zirconia implants, according to related reports, the RTQ (removal torque) value was significantly more for sandblasted zirconia than machined zirconia (33). Besides, the corporation of fluoride at zirconia surface could enhance osteoblastic differentiation and interfacial bone formation. It was reported that BIC of HF etched zirconia is approximately 81% (34).
There are also some disadvantages of sandblasting. Studies showed that the sandblasting process can cause deep micro-cracks, which may result in the reduction of strength (31). Therefore, a proper protocol is needed when treating zirconia surface with sandblasting to avoid the decrease of the strength.
UV treatment is an effective physicochemical method for surface modification of zirconia implants. UV light can induce electron excitation, increase zirconia surface energy, and result in a super-hydrophilic surface (35). Hydrophilicity is one of the key factors for enhancing the attachment, growth, proliferation, and differentiation of osteoblast (36). One in vivo study showed that the super hydrophilic surface of zirconia increased osteoblasts attachment and spreading, and then induced faster healing and higher BIC percentage (37). Besides, in Att's study, the atomic percentage of hydrocarbon changed after UV treatment, which resulted in a better protein adsorption and cell adhesion (38). In conclusion, UV surface treatment is a promising strategy to promote bone morphogenesis around zirconia implants.
Many studies have reported using laser treatment to modify the surface properties of zirconia. The main mechanism for laser to improve the surface is that the treatment increases the surface energy and consequent wettability (39), which plays a key role in protein adsorption and cell adhesion. Another advantage of laser treatment compared to other surface treatment methods is that it can produce microscale patterns with regular geometry instead of random surface patterns. The laser-induced periodic surface structures have been proved to improve osseointegration, reduce biofilm formation, and enhance soft tissue attachment (40). Besides, significantly higher BIC and RTQ is related to a laser-treated zirconia implant (41, 42). However, the laser treatment induces thermal cracking, which can reduce mechanical strength. The laser also induces phase transformation of zirconia, which may impair the long-term stability of dental implants. Therefore, the choice of laser treatment process and the consequential microcrack should be taken into consideration (40).
Self-assembly is defined as the autonomous process by which components organize into patterns or structures without external intervention (43). In the year 2008, Rauscher found a pseudo periodic-array of nano islands on the surface of YZP after thermal treatment (44). This nanostructure was further investigated, and reported to have satisfying cellular responses such as better cell spreading and cytoskeletal protein distribution (45).
Different coatings on zirconia surfaces have frequently been applied to improve surface properties. Among the many kinds of coatings, Hydroxyapatite (HA) is an important one that explored deeply by many researches. HA has a similar mineral composition to that of bone, and thus shows bioactive properties that enhances osseointegration (46). Calcium phosphate (CP) is another bioactive coating with a chemical composition close to that of the mineral phase of bone. CP-based material allows zirconia implant to form a rigid bond with surrounding tissue, and results in good performance in early-osseointegration (47). However, both HA and CP coatings were reported with poor stability and weak bonding strength. To overcome these drawbacks, some filler materials, i.e., tricalcium phosphate and zirconia powder, were added into the coatings to enhance the coatings' strength (48, 49). Not only to convert the inert zirconia into a bioactive material, some coatings also act as drug delivery systems (50). Porous zirconia scaffolds coated with HA have been used as a drug delivery system to enhance bone response and assure proper osseointegration (51). In summary, bioactive coatings can enhance the biocompatibility and osteogenic response of zirconia, but most of the reports are preclinical studies, clinical trails are still needed.
BIC is commonly measured by histomorphometric analyses at different time points and used to assess the quality of osseointegration in a quantitative manner. According to current studies, tibia of rabbits (6), tibia of minipigs (52), jaws and femur of sheep (53), mandible of dogs (54), and maxilla of monkeys (55) have been used to investigate the BIC of zirconia. Both functionally loaded or not loaded implants were included. Titanium implants were most used as a control group to compare with zirconia implants. These studies proved that both titanium and zirconia surfaces were osteoconductive, and most of the studies reported that the two materials did not have any significant different effect on the BIC values (56–58), while some reported that zirconia was better (59, 60). Yet BIC values depend a lot on the individual animal model, the difference was significant (56). Compared to unloaded and immediately loaded implants, conventionally loaded implants showed significantly increased BIC values (61, 62). For different study durations, people founded that a longer investigation and loading period could result in a significant increase in BIC values (63, 64). In summary, all of the studies demonstrated excellent BIC values around zirconia implants, although the values may vary depending on the study animals and the loading status.
RTQ is a quantitative way for assessing osseointegration via calculating the torsional strengths needed to remove an inserted implant. The effect of different implant materials on RTQ is controversial. According to current studies, some reported no significant differences in RTQ between zirconia and titanium (58, 63, 65), while some reported that RTQ was significantly lower for zirconia implants than for titanium implants (66–69). However, RTQ is depending a lot on different surface modifications. Some authors reported that zirconia implants with decreased surface roughness could reach equivalent RTQ values compared to control titanium implants with increased surface roughness (70). In one study, researchers compared the biomechanical properties of six types implant surfaces and found that the RTQ values were highest for the SLA titanium, followed by SLA and Calcium phosphate (CaP)-coated titanium, SLA and bisphosphonate-coated titanium, SLA and collagen-coated titanium, SLA zirconia and SLA and anodic plasma chemical surface-treated titanium (66). However, when modified titanium implants were compared with modified zirconia implants, the RTQ values were similar (71). These results can be summarized that RTQ depends more on different surfaces than different materials. Some other factors also may have an impact on the RTQ values. According to some reports, a longer loading period is always related to a significantly increase in RTQ (60, 72). Besides, conventionally loaded implants showed increased RTQ compared to unloaded implants (56). A meta-analysis upon preclinical evaluations using different animal models revealed that the individual animal model also significantly influenced the evaluated RTQ outcomes (56).
Push-in test in combination with histomorphometry was first established by Ogawa, using as a valid model for research of the osseointegration process in an established rat-animal model (73). The main impact factors on PI values include surface topography, individual animal model and PI testing protocols. Kohal compared two kinds of materials, titanium and zirconia, with different surfaces. It turned out that there was no significant difference between the modified titanium surface and the modified zirconia surface, while the values were significantly higher compared with the machined implant surfaces. Moreover, the study also reported that significantly decreased PI values for zirconia compared to titanium were associated with an increased surface roughness value (63). These can be summarized that PI differences between titanium and zirconia are related to surface topography characteristics and not to material properties.
In recent years, zirconia implants have been recommended for excellent esthetic efforts, especially in the anterior maxillary zone. But up to date, there are only a few primary studies about clinical outcomes of zirconia implants, not to mention long-term follow-ups. In spite of the disadvantages of titanium implants such as potential local and systematic toxicity and gray color, the long-term clinical survival rate has been proved sufficiently. Data from recent systematic reviews suggest that the survival rate of titanium implants that support single crowns is ~97.2 and 95.2% over a period of 5 and 10 years, respectively (74). These results are relatively ideal for the clinical application of an implant material. However, some clinicians still consider that zirconia implants may not offer a sufficient validation of their performance as equivalent to or better than titanium implants.
According to Elnayef's meta-analysis (75), the survival rate of 1,948 zirconia implants is 91.5%, significantly lower than that of titanium implants. Thus, he concluded that for certain conditions, such as a thin gingiva or in the maxillary anterior area, zirconia implants may offer some benefit. In another study, Pieralli reported a 1-year implant survival rate of 95.6%. They also mentioned that most of the implant failures occurred in the early healing period, followed by a survival rate decrease of 0.05% per year (76). Other studies reported the survival rate after 12 months ranged between 85% (77) and 100% (78, 79). It can be inferred that the differences among the outcomes of clinical trials may be related to the insufficient duration for investigations and the shortage of long-term studies.
Several prospective clinical trials evaluating MBL as a secondary outcome have been performed since 2010 (76). As the same as the survival rate, there are great differences among the reports. As a quantitative index, MBL was reported ranging from 0.7 mm (56) to 0.98 mm (80). In some studies, zirconia implants were reported as presenting similar MBL outcomes to titanium implants (76, 81). However, according to Elnayef's research, titanium implants performed better than zirconia implants upon the comparison with MBL (75). While in Vohra's research, the results were opposite (82). In conclusion, the short-term clinical outcomes of zirconia implants are acceptable, but evaluation of long-term outcomes are still needed to compare the clinical performances between zirconia implants and titanium implants.
The present review analyzed the zirconia implant materials currently used and the techniques reported for zirconia surface modifications. In summary, zirconia is a good candidate for dental implant because of its good mechanical, esthetic and biocompatible performance. Zirconia dental implants have deeply been studied and promising results in clinical trials have been shown. Considering outcomes of preclinical studies and clinical trials are sometimes controversial when compared with titanium, whether zirconia material will be used as an alternative to titanium is not clear due to the lack of long-term follow-ups. Surface modifications of zirconia implants were investigated widely, most of the modification methods are proved beneficial to zirconia implants in several aspects, including enhancing cell response and improving their osseointegration. But the investigations are not as sufficient as those of titanium implants. For most of the techniques, clinical trials are still needed.
YQ was in charge of manuscript preparation. LL contributed to literature research and manuscript revision. All authors contributed to the article and approved the submitted version.
The authors declare that the research was conducted in the absence of any commercial or financial relationships that could be construed as a potential conflict of interest.
All claims expressed in this article are solely those of the authors and do not necessarily represent those of their affiliated organizations, or those of the publisher, the editors and the reviewers. Any product that may be evaluated in this article, or claim that may be made by its manufacturer, is not guaranteed or endorsed by the publisher.
1. Lacefield WR. Materials characteristics of uncoated/ceramic-coated implant materials. Adv Dental Res. (1999) 13:21–6. doi: 10.1177/08959374990130011001
2. Bona AD, Pecho OE, Alessandretti R. Zirconia as a dental biomaterial. Materials. (2015) 8:4978–91. doi: 10.3390/ma8084978
3. Weingart D, Steinemann S, Schilli W, Strub JR, Hellerich U, Assenmacher J, et al. Titanium deposition in regional lymph nodes after insertion of titanium screw implants in maxillofacial region. Int J Oral Maxillofac Surg. (1994) 23:450–2. doi: 10.1016/S0901-5027(05)80045-1
4. Sicilia A, Cuesta S, Coma G, Arregui I, Guisasola C, Ruiz E, et al. Titanium allergy in dental implant patients: a clinical study on 1500 consecutive patients. Clin Oral Implants Res. (2008) 19:823–35. doi: 10.1111/j.1600-0501.2008.01544.x
5. Kniha K, Kniha H, Grunert I, Edelhoff D, Hölzle F, Modabber A. Esthetic evaluation of maxillary single-tooth zirconia implants in the esthetic zone. Int J Periodont Restorat Dentist. (2019) 39:e195–201. doi: 10.11607/prd.3282
6. Scarano A, Piattelli M, Vrespa G, Caputi S, Piattelli A. Bacterial adhesion on titanium nitride-coated and uncoated implants: an in vivo human study. J Oral Implantol. (2003) 29:80–5. doi: 10.1563/1548-1336(2003)029<0080:BAOTNA>2.3.CO;2
7. Akagawa Y, Hosokawa R, Sato Y, Kamayama K. Comparison between freestanding and tooth-connected partially stabilized zirconia implants after two years' function in monkeys: a clinical and histologic study. J Prosthet Dent. (1998) 80:551–8. doi: 10.1016/S0022-3913(98)70031-9
8. Ichikawa Y, Akagawa Y, Nikai H, Tsuru H. Tissue compatibility and stability of a new zirconia ceramic in vivo. J Prosthet Dent. (1992) 68:322–6. doi: 10.1016/0022-3913(92)90338-B
9. Ozkurt Z, Kazazoglu E. Zirconia dental implants: a literature review. J Oral Implantol. (2011) 37:367–76. doi: 10.1563/AAID-JOI-D-09-00079
10. Siddiqi A, Khan AS, Zafar S. Thirty years of translational research in zirconia dental implants: a systematic review of the literature. J Oral Implantol. (2017) 43:314–25. doi: 10.1563/aaid-joi-D-17-00016
11. Cionca N, Hashim D, Mombelli A. Zirconia dental implants: where are we now, and where are we heading? Periodontology 2000. (2017) 73:241–58. doi: 10.1111/prd.12180
12. Andreiotelli M, Wenz HJ, Kohal RJ. Are ceramic implants a viable alternative to titanium implants? A systematic literature review. Clin Oral Implants Res. (2009) 20(Suppl. 4):32–47. doi: 10.1111/j.1600-0501.2009.01785.x
13. Christel P, Meunier A, Dorlot JM, Crolet JM, Witvoet J, Sedel L, et al. Biomechanical compatibility and design of ceramic implants for orthopedic surgery. Ann N Y Acad Sci. (1988) 523:234–56. doi: 10.1111/j.1749-6632.1988.tb38516.x
14. Lawson S. Environmental degradation of zirconia ceramics. J Eur Ceram Soc. (1995) 15:485–502. doi: 10.1016/0955-2219(95)00035-S
15. Chevalier J. What future for zirconia as a biomaterial? Biomaterials. (2006) 27:535–43. doi: 10.1016/j.biomaterials.2005.07.034
16. Torres-Rodríguez J, Kalmár J, Menelaou M, Celko L, Dvorak K, Cihlár J, et al. Heat treatment induced phase transformations in zirconia and yttria-stabilized zirconia monolithic aerogels. J Supercrit Fluids. (2019) 149:54–63. doi: 10.1016/j.supflu.2019.02.011
17. Fabris S, Paxton AT, Finnis MW. A stabilization mechanism of zirconia based on oxygen vacancies only. Acta Mater. (2002) 50:5171–8. doi: 10.1016/S1359-6454(02)00385-3
18. Benyagoub A, Couvreur F, Bouffard S, Levesque F, Dufour C, Paumier E. Phase transformation induced in pure zirconia by high energy heavy ion irradiation. Nucl Instrument Methods Phys Res. (2001) 175–7:417–21. doi: 10.1016/S0168-583X(00)00609-1
19. Pan LS, Horibe S. An in-situ investigation on the critical phase transformation stress of tetragonal zirconia polycrystalline ceramics. J Mater Sci. (1996) 31:6523–7. doi: 10.1007/BF00356258
20. Muddle BC. Displacive phase transformations in zirconia-based ceramics. MRS Proc. (1986) 78:3. doi: 10.1557/PROC-78-3
21. McMeeking RM, Evans AG. Mechanics of transformation-toughening in brittle materials. J Am Ceram Soc. (1982) 65:242–6. doi: 10.1111/j.1151-2916.1982.tb10426.x
22. Heuer AH, Claussen N, Kriven WM, Ruhle M. Stability of tetragonal ZrO2 particles in ceramic matrices. J Am Ceram Soc. (1982) 65:642–50. doi: 10.1111/j.1151-2916.1982.tb09946.x
23. Sanon C, Chevalier J, Douillard T, Cattani-Lorente M, Scherrer SS, Gremillard L. A new testing protocol for zirconia dental implants. Dent Mater. (2015) 31:15–25. doi: 10.1016/j.dental.2014.09.002
24. Silva NR, Nourian P, Coelho PG, Rekow ED, Thompson VP. Impact fracture resistance of two titanium-abutment systems versus a single-piece ceramic implant. Clin Implant Dent Relat Res. (2011) 13:168–73. doi: 10.1111/j.1708-8208.2009.00187.x
25. Swab JJ. Low temperature degradation of Y-TZP materials. J Mater Sci. (1991) 26:6706–14. doi: 10.1007/BF02402664
26. Nishizaki M, Komasa S, Taguchi Y, Nishizaki H, Okazaki J. Bioactivity of NANOZR induced by alkali treatment. Int J Mol Sci. (2017) 18:780. doi: 10.3390/ijms18040780
27. Nawa M, Bamba N, Sekino T, Niihara K. The effect of TiO2 addition on strengthening and toughening in intragranular type of 12Ce-TZP/Al2O3 nanocomposites. J Eur Ceram Soc. (1998) 18:209–19. doi: 10.1016/S0955-2219(97)00116-7
28. Han JM, Hong G, Lin H, Shimizu Y, Wu Y, Zheng G, et al. Biomechanical and histological evaluation of the osseointegration capacity of two types of zirconia implant. Int J Nanomed. (2016) 11:6507–16. doi: 10.2147/IJN.S119519
29. Linkevicius T, Apse P. Influence of abutment material on stability of peri-implant tissues: a systematic review. Int J Oral Maxillofac Implants. (2008) 23:449–56. doi: 10.1016/j.prosdent.2020.01.025
30. Kimura Y, Matsuzaka K, Yoshinari M, Inoue T. Initial attachment of human oral keratinocytes cultured on zirconia or titanium. Dent Mater J. (2012) 31:346–53. doi: 10.4012/dmj.2011-189
31. Fischer J, Schott A, Martin S. Surface micro-structuring of zirconia dental implants. Clin Oral Implants Res. (2016) 27:162–6. doi: 10.1111/clr.12553
32. Brett PM, Harle J, Salih V, Mihoc R, Olsen I, Jones FH, et al. Roughness response genes in osteoblasts. Bone. (2004) 35:124–33. doi: 10.1016/j.bone.2004.03.009
33. Sivaraman K, Chopra A, Narayan AI, Balakrishnan D. Is zirconia a viable alternative to titanium for oral implant? A critical review. J Prosthodont Res. (2018) 62:121–33. doi: 10.1016/j.jpor.2017.07.003
34. Oliva J, Oliva X, Oliva JD. Five-year success rate of 831 consecutively placed Zirconia dental implants in humans: a comparison of three different rough surfaces. Int J Oral Maxillofac Implants. (2010) 25:336−44.
35. Ogawa T. Ultraviolet photofunctionalization of titanium implants. Int J Oral Maxillofac Implants. (2014) 29:e95–102. doi: 10.11607/jomi.te47
36. Henningsen A, Smeets R, Heuberger R, Jung OT, Hanken H, Heiland M, et al. Changes in surface characteristics of titanium and zirconia after surface treatment with ultraviolet light or non-thermal plasma. Eur J Oral Sci. (2018) 126:126–34. doi: 10.1111/eos.12400
37. Tuna T, Wein M, Altmann B, Steinberg T, Fischer J, Att W. Effect of ultraviolet photofunctionalisation on the cell attractiveness of zirconia implant materials. Eur Cell Mater. (2015) 29:82–94; discussion 5–6. doi: 10.22203/eCM.v029a07
38. Aita H, Hori N, Takeuchi M, Suzuki T, Yamada M, Anpo M, et al. The effect of ultraviolet functionalization of titanium on integration with bone. Biomaterials. (2009) 30:1015–25. doi: 10.1016/j.biomaterials.2008.11.004
39. Hao L, Lawrence J, Chian KS. Osteoblast cell adhesion on a laser modified zirconia based bioceramic. J Mater Sci Mater Med. (2005) 16:719–26. doi: 10.1007/s10856-005-2608-3
40. Han J, Zhang F, Van Meerbeek B, Vleugels J, Braem A, Castagne S. Laser surface texturing of zirconia-based ceramics for dental applications: a review. Mater Sci Eng C. (2021) 123:112034. doi: 10.1016/j.msec.2021.112034
41. Yasuno K, Kakura K, Taniguchi Y, Yamaguchi Y, Kido H. Zirconia implants with laser surface treatment: peri-implant bone response and enhancement of osseointegration. J Hard Tissue Biol. (2014) 23:93–100. doi: 10.2485/jhtb.23.93
42. Hao L, Lawrence J. Effects of CO2 laser irradiation on the wettability and human skin fibroblast cell response of magnesia partially stabilised zirconia. Mater Sci Eng C. (2003) 23:627–39. doi: 10.1016/S0928-4931(03)00056-0
43. Soon G, Pingguan-Murphy B, Lai KW, Akbar SA. Review of zirconia-based bioceramic: surface modification and cellular response. Ceram Int. (2016) 42:12543–55. doi: 10.1016/j.ceramint.2016.05.077
44. Rauscher MD, Boyne A, Dregia SA, Akbar SA. Self-assembly of pseudoperiodic arrays of nanoislands on YSZ-(001). Adv Mater. (2008) 20:1699–705. doi: 10.1002/adma.200701383
45. Parikh KS, Rao SS, Ansari HM, Zimmerman LB, Lee LJ, Akbar SA, et al. Ceramic nanopatterned surfaces to explore the effects of nanotopography on cell attachment. Mater Sci Eng C. (2012) 32:2469–75. doi: 10.1016/j.msec.2012.07.028
46. Schünemann FH, Galárraga-Vinueza ME, Magini R, Fredel M, Silva F, Souza JCM, et al. Zirconia surface modifications for implant dentistry. Mater Sci Eng C. (2019) 98:1294–305. doi: 10.1016/j.msec.2019.01.062
47. Karthigeyan S, Ravindran AJ, Bhat RTR, Nageshwarao MN, Murugesan SV, Angamuthu V. Surface modification techniques for zirconia-based bioceramics: a review. J Pharm Bioallied Sci. (2019) 11:S131–4. doi: 10.4103/JPBS.JPBS_45_19
48. Yang J-Z, Sultana R, Ichim P, Hu X-Z, Huang Z-H, Yi W, et al. Micro-porous calcium phosphate coatings on load-bearing zirconia substrate: processing, property and application. Ceram Int. (2013) 39:6533–42. doi: 10.1016/j.ceramint.2013.01.086
49. Pardun K, Treccani L, Volkmann E, Li Destri G, Marletta G, Streckbein P, et al. Characterization of wet powder-sprayed zirconia/calcium phosphate coating for dental implants. Clin Implant Dentist Relat Res. (2015) 17:186–98. doi: 10.1111/cid.12071
50. Qu Y, Hong G, Liu L, Sasaki K, Chen X. Evaluation of silk fibroin electrogel coating for zirconia material surface. Dental Mater J. (2019) 38:813–20. doi: 10.4012/dmj.2018-228
51. Cho Y, Hong J, Ryoo H, Kim D, Park J, Han J. Osteogenic responses to zirconia with hydroxyapatite coating by aerosol deposition. J Dental Res. (2015) 94:491–9. doi: 10.1177/0022034514566432
52. Depprich R, Zipprich H, Ommerborn M, Naujoks C, Wiesmann HP, Kiattavorncharoen S, et al. Osseointegration of zirconia implants compared with titanium: an in vivo study. Head Face Med. (2008) 4:30. doi: 10.1186/1746-160X-4-30
53. Siddiqi A, Duncan WJ, De Silva RK, Zafar S. One-piece zirconia ceramic versus titanium implants in the jaw and femur of a sheep model: a pilot study. Biomed Res Int. (2016) 2016:6792972. doi: 10.1155/2016/6792972
54. Dubruille JH, Viguier E, Le Naour G, Dubruille MT, Auriol M, Le Charpentier Y. Evaluation of combinations of titanium, zirconia, and alumina implants with 2 bone fillers in the dog. Int J Oral Maxillofac Implants. (1999) 14:271–7.
55. Kohal RJ, Weng D, Bachle M, Strub JR. Loaded custom-made zirconia and titanium implants show similar osseointegration: an animal experiment. J Periodontol. (2004) 75:1262–8. doi: 10.1902/jop.2004.75.9.1262
56. Roehling S, Schlegel KA, Woelfler H, Gahlert M. Zirconia compared to titanium dental implants in preclinical studies—A systematic review and meta-analysis. Clin Oral Implants Res. (2019) 30:365–95. doi: 10.1111/clr.13425
57. Schultze-Mosgau S, Schliephake H, Radespiel-Troger M, Neukam FW. Osseointegration of endodontic endosseous cones: zirconium oxide vs titanium. Oral Surg Oral Med Oral Pathol Oral Radiol Endod. (2000) 89:91–8. doi: 10.1016/S1079-2104(00)80022-0
58. Gahlert M, Rohling S, Wieland M, Sprecher CM, Kniha H, Milz S. Osseointegration of zirconia and titanium dental implants: a histological and histomorphometrical study in the maxilla of pigs. Clin Oral Implants Res. (2009) 20:1247–53. doi: 10.1111/j.1600-0501.2009.01734.x
59. Langhoff JD, Voelter K, Scharnweber D, Schnabelrauch M, Schlottig F, Hefti T, et al. Comparison of chemically and pharmaceutically modified titanium and zirconia implant surfaces in dentistry: a study in sheep. Int J Oral Maxillofac Surg. (2008) 37:1125–32. doi: 10.1016/j.ijom.2008.09.008
60. Aboushelib MN, Salem NA, Taleb AL, El Moniem NM. Influence of surface nano-roughness on osseointegration of zirconia implants in rabbit femur heads using selective infiltration etching technique. J Oral Implantol. (2013) 39:583–90. doi: 10.1563/AAID-JOI-D-11-00075
61. Janner SFM, Gahlert M, Bosshardt DD, Roehling S, Milz S, Higginbottom F, et al. Bone response to functionally loaded, two-piece zirconia implants: a preclinical histometric study. Clin Oral Implants Res. (2018) 29:277–89. doi: 10.1111/clr.13112
62. Thoma DS, Benic GI, Munoz F, Kohal R, Sanz Martin I, Cantalapiedra AG, et al. Histological analysis of loaded zirconia and titanium dental implants: an experimental study in the dog mandible. J Clin Periodontol. (2015) 42:967–75. doi: 10.1111/jcpe.12453
63. Kohal RJ, Wolkewitz M, Hinze M, Han JS, Bachle M, Butz F. Biomechanical and histological behavior of zirconia implants: an experiment in the rat. Clin Oral Implants Res. (2009) 20:333–9. doi: 10.1111/j.1600-0501.2008.01656.x
64. Hoffmann O, Angelov N, Gallez F, Jung RE, Weber FE. The zirconia implant-bone interface: a preliminary histologic evaluation in rabbits. Int J Oral Maxillofac Implants. (2008) 23:691–5.
65. Shin D, Blanchard SB, Ito M, Chu T-MG. Peripheral quantitative computer tomographic, histomorphometric, and removal torque analyses of two different non-coated implants in a rabbit model. Clin Oral Implants Res. (2011) 22:242–50. doi: 10.1111/j.1600-0501.2010.01980.x
66. Ferguson SJ, Langhoff JD, Voelter K, von Rechenberg B, Scharnweber D, Bierbaum S, et al. Biomechanical comparison of different surface modifications for dental implants. Int J Oral Maxillofac Implants. (2008) 23:1037–46.
67. Gahlert M, Gudehus T, Eichhorn S, Steinhauser E, Kniha H, Erhardt W. Biomechanical and histomorphometric comparison between zirconia implants with varying surface textures and a titanium implant in the maxilla of miniature pigs. Clin Oral Implants Res. (2007) 18:662–8. doi: 10.1111/j.1600-0501.2007.01401.x
68. Hoffmann O, Angelov N, Zafiropoulos GG, Andreana S. Osseointegration of zirconia implants with different surface characteristics: an evaluation in rabbits. Int J Oral Maxillofac Implants. (2012) 27:352–8.
69. Schliephake H, Hefti T, Schlottig F, Gedet P, Staedt H. Mechanical anchorage and peri-implant bone formation of surface-modified zirconia in minipigs. J Clin Periodontol. (2010) 37:818–28. doi: 10.1111/j.1600-051X.2010.01549.x
70. Bormann K-H, Gellrich N-C, Kniha H, Dard M, Wieland M, Gahlert M. Biomechanical evaluation of a microstructured zirconia implant by a removal torque comparison with a standard Ti-SLA implant. Clin Oral Implants Res. (2012) 23:1210–6. doi: 10.1111/j.1600-0501.2011.02291.x
71. Manzano G, Herrero LR, Montero J. Comparison of clinical performance of zirconia implants and titanium implants in animal models: a systematic review. Int J Oral Maxillofac Implants. (2014) 29:311–20. doi: 10.11607/jomi.2817
72. Gahlert M, Rohling S, Wieland M, Eichhorn S, Kuchenhoff H, Kniha H. A comparison study of the osseointegration of zirconia and titanium dental implants. A biomechanical evaluation in the maxilla of pigs. Clin Implant Dent Relat Res. (2010) 12:297–305. doi: 10.1111/j.1708-8208.2009.00168.x
73. Ogawa T, Ozawa S, Shih JH, Ryu KH, Sukotjo C, Yang JM, et al. Biomechanical evaluation of osseous implants having different surface topographies in rats. J Dental Res. (2000) 79:1857–63. doi: 10.1177/00220345000790110701
74. Jung RE, Zembic A, Pjetursson BE, Zwahlen M, Thoma DS. Systematic review of the survival rate and the incidence of biological, technical, and aesthetic complications of single crowns on implants reported in longitudinal studies with a mean follow-up of 5 years. Clin Oral Implants Res. (2012) 23(Suppl. 6):2–21. doi: 10.1111/j.1600-0501.2012.02547.x
75. Elnayef B, Lázaro A, Suárez-López Del Amo F, Galindo-Moreno P, Wang HL, Gargallo-Albiol J, et al. Zirconia implants as an alternative to titanium: a systematic review and meta-analysis. Int J Oral Maxillofac Implants. (2017) 32:e125–34. doi: 10.11607/jomi.5223
76. Pieralli S, Kohal RJ, Jung RE, Vach K, Spies BC. Clinical outcomes of zirconia dental implants: a systematic review. J Dent Res. (2017) 96:38–46. doi: 10.1177/0022034516664043
77. Cannizzaro G, Torchio C, Felice P, Leone M, Esposito M. Immediate occlusal versus non-occlusal loading of single zirconia implants. A multicentre pragmatic randomised clinical trial. Eur J Oral Implantol. (2010) 3:111–20. doi: 10.1111/clr.303_13509
78. Jung RE, Grohmann P, Sailer I, Steinhart YN, Fehér A, Hämmerle C, et al. Evaluation of a one-piece ceramic implant used for single-tooth replacement and three-unit fixed partial dentures: a prospective cohort clinical trial. Clin Oral Implants Res. (2016) 27:751–61. doi: 10.1111/clr.12670
79. Spies BC, Balmer M, Patzelt SB, Vach K, Kohal RJ. Clinical and patient-reported outcomes of a zirconia oral implant: three-year results of a prospective cohort investigation. J Dent Res. (2015) 94:1385–91. doi: 10.1177/0022034515598962
80. Haro Adanez M, Nishihara H, Att W. A systematic review and meta-analysis on the clinical outcome of zirconia implant-restoration complex. J Prosthodont Res. (2018) 62:397–406. doi: 10.1016/j.jpor.2018.04.007
81. Roehling S, Schlegel KA, Woelfler H, Gahlert M. Performance and outcome of zirconia dental implants in clinical studies: a meta-analysis. Clin Oral Implants Res. (2018) 29(Suppl. 16):135–53. doi: 10.1111/clr.13352
Keywords: zirconia, implants, surface modification, biocompatibility, mechanical properties
Citation: Qu Y and Liu L (2021) Zirconia Materials for Dental Implants: A Literature Review. Front. Dent. Med. 2:687983. doi: 10.3389/fdmed.2021.687983
Received: 30 March 2021; Accepted: 19 July 2021;
Published: 13 August 2021.
Edited by:
James K. H. Tsoi, The University of Hong Kong, Hong KongReviewed by:
Walter Y. H. Lam, The University of Hong Kong, Hong KongCopyright © 2021 Qu and Liu. This is an open-access article distributed under the terms of the Creative Commons Attribution License (CC BY). The use, distribution or reproduction in other forums is permitted, provided the original author(s) and the copyright owner(s) are credited and that the original publication in this journal is cited, in accordance with accepted academic practice. No use, distribution or reproduction is permitted which does not comply with these terms.
*Correspondence: Yinying Qu, cXVfeXlAZm94bWFpbC5jb20=
Disclaimer: All claims expressed in this article are solely those of the authors and do not necessarily represent those of their affiliated organizations, or those of the publisher, the editors and the reviewers. Any product that may be evaluated in this article or claim that may be made by its manufacturer is not guaranteed or endorsed by the publisher.
Research integrity at Frontiers
Learn more about the work of our research integrity team to safeguard the quality of each article we publish.