- 1School of Medicine, Dentistry and Biomedical Sciences, The Wellcome-Wolfson Institute for Experimental Medicine, Queen's University Belfast, Belfast, United Kingdom
- 2Department of Oral Sciences, Faculty of Dentistry, Sir John Walsh Research Institute, University of Otago, Dunedin, New Zealand
- 3Aix-Marseille University, CNRS, ISM, The Institute of Movement Science, Marseille, France
- 4Institute of Clinical Sciences, The University of Birmingham School of Dentistry, Birmingham, United Kingdom
- 5Division of Restorative Dentistry and Periodontology, Trinity College Dublin, Dublin Dental University Hospital, University of Dublin, Dublin, Ireland
Research over several decades has increased our understanding of the nature of reparative and regenerative processes in the dental pulp, at both the cellular and molecular level. However, advances in scientific knowledge have not translated into novel clinical treatment strategies for caries-induced pulpitis. This narrative review explores the evidence regarding the ability of inflamed pulp tissue to heal and how this knowledge may be used therapeutically. A literature search and evidence analysis covering basic, translational and clinical pulp biology research was performed. The review focuses on (1) the regenerative and defense capabilities of the pulp during caries-induced inflammation; (2) the potential of novel biomaterials to harness the reparative and regenerative functions of the inflamed pulp; and (3) future perspectives and opportunities for conservative management of the inflamed pulp. Current conservative management strategies for pulpitis are limited by a combination of unreliable diagnostic tools and an outdated understanding of pulpal pathophysiological responses. This approach leads to the often unnecessary removal of the entire pulp. Consequently, there is a need for better diagnostic approaches and a focus on minimally-invasive treatments utilizing biologically-based regenerative materials and technologies.
Introduction
Dental caries remains the most important threat to the dental pulp (1) with current treatments dependent on the extent and depth of the lesion, its rate of progression, disease activity, related signs and symptoms, as well as the views of the treating dentist (2). Research investigating caries progression in enamel and dentine has greatly enhanced our understanding of the nature of the carious process and improved our management strategies. Caries is no longer considered a progressive, destructive process, and opportunities exist to reverse its advancement by promoting an optimal healing environment which encourages remineralisation and repair of the lesion (3). Notably, however, conservative treatment approaches for caries-induced pulpal inflammation and its management are yet to be fully developed. Although, appropriate management of caries can predictably reverse a mild pulpitic response, the current management strategies for more advanced caries-induced pulpal inflammation are often invasive and technically-demanding procedures involving pulpectomy and root canal treatment.
The aim of the caries-induced pulpal inflammatory response is to defend the tissue from further insult and promote healing (4). The inflammatory process is therefore closely linked to regenerative events and the presence of pulpal inflammation should not be assumed to result exclusively in tissue degeneration. Traditionally, deep carious lesions (5) were associated with bacterial invasion of the dental pulpal tissue and if the resulting pulpal inflammation was irreversible, the removal of the entire pulp or the extraction of the tooth was indicated (6). However, this approach to diagnosis and treatment is somewhat empirical and does not reflect variations in the pulp status found beneath deep carious lesions. For instance, it has long been recognized that within deep carious lesions, large variations in activity is present (7). Therefore, it is reasonable to speculate that large variations in pulpal responses also exist within deep lesions and that pulpitis is not necessarily linearly progressive (8).
Maintaining the vitality of the dental pulp is essential as it possesses important sensory, defensive, nutritional and functional properties (9). Given our developing understanding of pulpal biology, the promotion of minimally invasive vital pulp treatments (VPTs) to retain its viability is now paramount. This review, therefore, aims to examine the scientific basis for such therapies by exploring at the cellular and molecular level the healing potential of the inflamed pulp and to discuss future perspectives for its' management.
The Process of Tissue Repair in Pulpitis
Repair is defined as the restoration of tissue architecture and function after an injury. It encompasses two separate processes: tissue regeneration and replacement (10). Regeneration refers to healing in which new growth restores portions of damaged tissue to their normal (pre-disease) state. Replacement is a form of healing in which severely damaged tissues, which cannot be regenerated, are repaired by the laying down of connective tissue. The nature of the repair process whether, regeneration, replacement or both, depends on the type and severity of tissue damage. Tissues with more proliferative capacities and rich in a stem cell population, favor regeneration, while tissues that contain terminally differentiated cells are likely to heal and repair using tissue replacement. Anatomically the dental pulp, although populated by terminally differentiated cells, including odontoblasts, also contains a resident population of adult stem as well as migratory progenitor cell populations (11) that can contribute to reparative processes, consequently a combination of replacement and regeneration could occur in the diseased and damaged pulp. The terms repair and regeneration have generally been used interchangeably when describing the nature of pulp wound healing process (12). In reality, however true regeneration and reconstitution of the native tissues is seldom achieved after damage to dentin-pulp complex using current clinically-approved treatment modalities, therefore the pulpal healing response is most appropriately described as a repair.
In general, any tissue repair process involves the three sequential phases of inflammation, proliferation and remodeling. These phases, which also occur in pulpitis, are shown in Figure 1 and are discussed below.
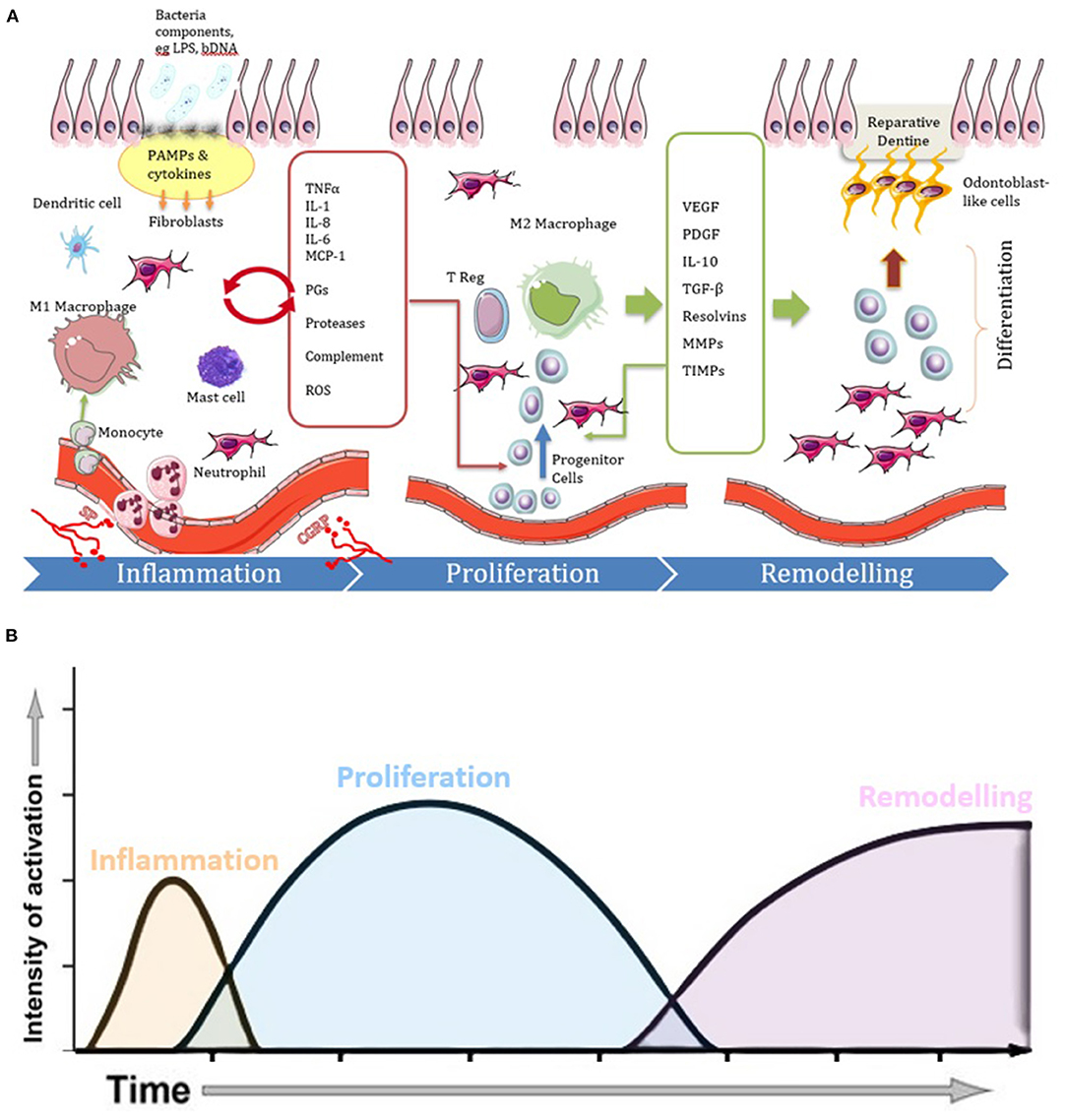
Figure 1. Diagrams illustrating the three phases of the repair process in pulpitis, showing (A) cell and molecular involvement and (B) temporal dynamics. Following carious infection and lesion progression the inflammatory phase becomes characterized by vasodilation and increased migration of immune cells (e.g., monocytes, macrophages, dendritic cells, mast cells and neutrophils) in response to cytokines and other chemotactic signals released by tissue resident cells in response to PAMPs. The cytokines and chemokines act via paracrine signaling (red arrow) to amplify the inflammation. Neuronal tissues also release neurotrophic factors, such as SP and CGRP, which also influence the course of the inflammatory and healing reactions. When the infection is under control, the inflammatory process begins to resolve and there is an increase in cells and molecules which act in an anti-inflammatory manner. Many of the cytokines which drove the inflammatory response along with newly expressed molecules promote proliferative responses as the disease context changes toward a healing response. M2 macrophages are also evident which promote inflammation resolution. Proliferation and migration of stem/progenitor cells continue to complete the healing process by the differentiation of new pulp tissue and odontoblast-like cells that produce reparative dentine during the remodeling phase.
The Inflammatory Phase
The dental pulp has unique cellular, neural and vascular elements that play important roles in pulpal homeostasis and pathophysiology in response to carious injury (13). In pulpitis, PAMPs trigger a protective inflammatory response characterized by the dynamic release of inflammatory mediators (14). This response has molecular and cellular components, the purpose of which are primarily to combat invading microbes, but critically it can also support the repair process (15).
A hallmark of this inflammatory process is the activation of the NFκB and MAPK signaling cascades, following PAMP binding by immune and non-immune cells. This process subsequently results in the release of a range of pro-inflammatory cytokines, including TNFα, IL-1, IL-8, IL-12, IL-6, and, IFN δ (Figure 1) (14). A range of dental pulp cells, including odontoblasts, fibroblasts, neurones, endothelial cells and stem cells express intracellular and extracellular receptors able to detect bacterial components. The best characterized receptor family within the pulp are the Toll-like receptors (TLRs), which can detect PAMPs ranging from bacterial DNA to cell wall derived lipopolysaccharides (16). The cytokines subsequently elicited are known to be abundantly expressed in inflamed pulpal tissue (17, 18). Once released they further exacerbate NFκB and other signaling pathways, including AP-1 and STAT to amplify the cytokine response in a paracrine manner. Notably, recent studies have indicated that the levels of these cytokines are highly expressed in teeth diagnosed with advanced, irreversible pulpitis and, therefore their assay has been suggested as a potential for use as diagnostic biomarkers for pulpitis (19).
The exact role these cytokines play in the repair process is not known, although evidence suggests their contribution is via several different mechanisms all of which are likely context-dependent. For example, IL-8 is produced by immune and pulp cells to attract neutrophils, but also has pro-angiogenic properties (20) and can act as a chemoattractant for DPSCs (21). Similarly, TNFα contributes to the repair process via multiple mechanisms including promoting DPSC functions, such as migration and proliferation (22, 23), and osteogenic and odontogenic differentiation (24, 25). However, these effects are likely to be more evident in the relatively early acute rather than the chronic inflammatory phase, where excessive chronic inflammation is generally understood to cause impaired healing (26, 27).
The rich and extensive innervation of the dental pulp results in pulpal nerves being located at sites able to respond to injury by mounting a so-called neurogenic inflammatory response, which is characterized by the release of neuropeptides (28, 29). Neuropeptides such as SP and CGRP have been shown to be upregulated in pulps associated with deep carious lesions as well as in symptomatic pulpitis (30). The neuronal sprouting associated with pulpal inflammation and the local release of neuropeptides into the dental pulp tissue, mediate not only an enhanced protective pain reflex but also a neurogenic inflammatory response targeted at combatting infection and facilitating healing and repair (31). Locally released neuropeptides play important roles in regulating pulpal blood flow (32) and in the production of angiogenic growth factors (GFs) which facilitate healing (28). In addition, neuropeptides display antimicrobial activities against oral pathogens including those associated with pulpal infection (33).
At the cellular level, pulp cells, such as fibroblasts, odontoblasts and DPSCs, also play critical roles in regulating the pulpal response to caries. While odontoblasts are primarily responsible for dentine formation however due to their anatomical location in the peripheral region of the pulp, it is not surprising that these cells display sensory (34) and immunological properties, which mediate early protective innate immune functions in response to cariogenic bacteria and external injurious stimuli (35). Fibroblasts are the most abundant cells within the pulp and have the ability to sense the external environment (36). These cells can produce numerous cytokines and GFs that facilitate healing and repair (28). Furthermore, pulp fibroblasts have been shown to synthesize functional complement components that have both antimicrobial activity and the ability to recruit stem cells in response to bacterial toxins (37, 38). DPSCs in addition to their key role in tissue regenerative also provide immunomodulatory functions that enable control of inflammation to achieve tissue homeostasis (39).
The Proliferative Phase
The proliferative phase often overlaps with the inflammatory phase. Here cellular activities predominate, including activation, proliferation, migration, and differentiation of tissue-resident progenitor cells. Activation and recruitment of these cellular processes begin to occur when pulp inflammation begins to subside (40). Indeed, the signals required to mobilize these cells from their niche are largely products of inflammation as described above and include cytokines, chemokines, GFs and complement components (37, 38).
Transition From Inflammation to Proliferation
Although initiated by inflammation, for the proliferative phase to progress to repair, the inflammatory processes have to be first controlled and then resolved. In general, if the inflammatory response is able to contain the microbial infection, then the overall response is shifted toward the generation of anti-inflammatory signals and the resolution of inflammation. The signals mediating timely control of the inflammatory response and the shift to resolution and repair are not entirely elucidated, however a role for macrophages has been suggested. In the early inflammatory phase macrophages are activated by the inflammatory microenvironment to polarize into pro-inflammatory phenotype (M1 macrophages), that perform vital innate immune functions such as phagocytosis of microbes and dead cells and production of pro-inflammatory cytokines. Later in the healing process and with the transition from inflammatory to proliferative phase, macrophages transit from a pro-inflammatory M1 to a reparative M2 phenotype, expressing anti-inflammatory mediators such as, IL-10 and TGF-B1, which results in matrix deposition and tissue remodeling (Figure 1). The M1–M2 transition is critical for the resolution of inflammation and tipping the balance to tissue repair (41). This, together with lipid-derived pro-resolving mediators, as well as activation of intracellular stop signals that inhibit the innate immune response (42), all orchestrate to control the inflammatory process.
IL-10 is a potent anti-inflammatory cytokine which acts by suppressing the synthesis of pro-inflammatory cytokines and chemokines in macrophages through activation of STAT3 signaling (43). Notably, high levels of IL-10 have been shown to be detectable in the dental pulps from teeth with deep caries lesions (44) and in cariously-exposed pulps (45). In comparison with IL-8, the levels of IL-10 were higher in asymptomatic carious exposure cases compared with cases clinically diagnosed with irreversible pulpitis (45), which suggests that there is a different degree of inflammation in asymptomatic cariously exposed pulp compared with symptomatic pulps. Although carious exposure is generally considered irreversible pulpitis (6), the outcomes for VPT are better in cariously exposed pulp with a diagnosis of reversible pulpitis (46), than for symptomatic irreversible pulpitis, when presumably the infection and inflammatory processes are more severe (47).
Several members of the TGF-β family have been implicated in negative regulation of the inflammatory reaction. Indeed, high-through-put gene expression analysis showed increased levels of TGF-β family proteins and their receptors (48) in carious compared with healthy pulps. Furthermore, TGF-β1 was relatively highly expressed in the odontoblastic and sub-odontoblastic layer of pulpal specimens with irreversible pulpitis indicating that it played a significant role in the dentinal repair processes after pulpal inflammation (49, 50).
Pro-resolving lipid mediators, such as lipoxins, resolvins, protectins and maresins, possess potent anti-inflammatory properties and may play an important role in the resolution of dysregulated persistent inflammation in pulpitis. Lipoxins prevent the influx of neutrophils and help clear dead cells and tissue debris to modulate inflammation. Resolvins also reduce inflammation and promote healing (51), and Resolvin E1 has been shown to reduce pulpal inflammation in vitro and in a rat model of pulpitis (52). However, the potential role of endogenous pro-resolving lipid mediators in the suppression and resolution of pulpitis remains to be fully investigated.
In addition to the above molecular processes, many intracellular stop pathways can be activated to control the dysregulated inflammatory response, including signaling involving IRAK, SOCS-1 and SOCS-3, and theTNFAIP3) or A20 (53, 54). Despite the evidence demonstrating a role for all these pathways in controlling inflammation, their contribution to the regulation of pulp inflammation has yet to be rigorously investigated.
The Remodeling Phase
The final phase of the repair process involves tissue formation and maturation. In the case of the mineralised dentine barrier repair in pulpitis, DPSCs and other progenitor cells activated in the proliferative phase can differentiate into odontoblast-like cells under the influence of bioactive molecules. These cells replace the original odontoblasts that were lost due to carious disease in a process termed reparative tertiary dentinogenesis. DPSCs are able to differentiate, given a conducive environment, under the influence of certain environmental cues. However, the signals required to switch on this differentiation process are complex and not yet fully elucidated. Furthermore, the exact phenotype of the odontoblast-like cells and their differentiation origin also requires further characterization (54–57).
Regardless of their origin and nature, these cells lay down a collagenous matrix that subsequently undergoes mineralisation to form reparative dentine. The structure of reparative dentine is generally different to that of primary and the reactionary dentine which can be generated by the original developmentally derived odontoblasts. In general reparative dentine is less organized and lacks the continuous tubular structure of primary, secondary and reactionary dentine; however, this lack of permeability may protect the pulp from further external insult (58). Several studies have shown that the pulp wound healing response often results in the early formation of fibrodentine with an osteotypic appearance (59), and this structure cannot provide an effective barrier to protect the pulp from exogenous irritants. The factors that influence the nature and the quality of the final hard tissue formed are not known, but they are likely to be related to the extent of the inflammatory process, level of infection, the pulp capping material used, as well as the technical skill of the clinical operator.
The Pulpitis Diagnosis Conundrum
Currently, the clinical diagnosis of pulpal status is based on subjective tests relying on patients' reported symptoms, which unfortunately do not accurately correlate with the histological status of the pulp (60). In healthy teeth the odontoblast layer is intact, with no evidence of inflammatory cells within the pulp tissue (Figure 2A). In teeth that caries does not expose the pulp, the inflammation is likely to be relatively mild or moderate (Figure 2B) and resolve with the removal of the causative agent following clinical intervention. Consequently, pulpitis is treatable, is considered reversible and therefore the pathology is defined clinically as reversible pulpitis (6). In this situation, the degree of inflammation is generally compatible with healing and native odontoblast produce reactionary dentine to protect the pulp from further injury.
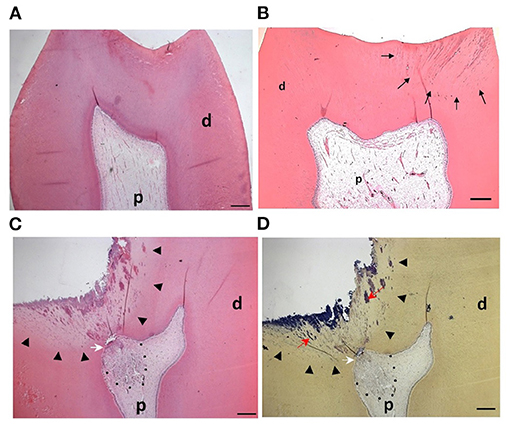
Figure 2. Pulp response to caries progression: (A) H&E staining of the dental pulp from a sound molar tooth showing healthy tissue with an intact odontoblast layer (B) In shallow caries bacteria and their components (black arrows) diffuse through dentinal tubules but have not reached the pulp. The odontoblast layer remains intact and the pulp tissue responds with formation of reactionary dentine. (C) In deep caries there is a more pronounced inflammatory response to the increased levels of infiltrating bacteria within the dentine (arrowheads). The dentine structure is irregular, partially destroyed and exhibits a non-tubular morphology. Reparative dentine (white arrow) is deposited beneath the carious lesion and there is an apparent and localized inflammatory cell infiltrate (dotted line). (D) Brown and Brenn staining of cariogenic bacteria (red arrows) in the same section as is shown in (C). P = pulp, d = dentine. Figure adapted from El Karim et al. (61), J Endod. Permission obtained from Elsevier.
In deep caries (lesion extend to the inner quarter of dentine, but with zone of hard or firm dentine between the caries and the pulp) and in the extremely deep caries lesions (lesion penetrates the entire thickness of the dentine) (5), bacteria may invade the pulp leading to more severe forms of pulpitis (Figures 2C,D). Here the pulpal diagnosis is generally considered irreversible. This means that the pulp is not amenable to treatment and should be removed by pulpectomy or extraction (6). However, this empirical approach to diagnosis and treatment is not compatible with the emerging minimal intervention approaches and does not translate our current knowledge on the importance of inflammation in the tissue repair process described above.
The dogma that claims severe pulp inflammation is irreversible, is based on the assumption that the pulp has limited ability to recover from the infectious and inflammatory challenge due to the anatomical constraint of its low compliance environment and lack of collateral circulation (62). However, the dental pulp possesses anatomical and physiological properties that may help compensate for these limitations. The pulpal blood flow is relatively high, compared with that of other oral tissues (63), and numerous shunt vessels exist (64), which provide direct communication between pulpal arterioles and venules. Notably, when intrapulpal pressure rises during inflammation, shunt vessels open to reduce this pressure, thereby allowing normal blood flow to be maintained (65).
Another compensatory mechanism for the low compliance environment may be provided by the dental pulp's extracellular matrix (ECM). It is accepted that the resilience of the ECM limits intra-pulpal pressure to the site of irritation (66). Indeed, significant pressure differences have been observed at sites only 1–2 mm apart in the pulp (67). Pressure from the increased tissue fluid collapses the thin-walled venules in the microenvironment of the affected pulp tissue, causing a localized vascular stasis and ischemia, which results in localized cellular death. It is only when the structural integrity of the pulp tissue is lost, as a result of overwhelming inflammation, that the increased tissue pressure spreads resulting in compression of the blood vessels at the apex of the tooth leading to total pulpal necrosis (68).
The pulpal ECM may also act as a barrier to the spread of microorganisms and toxic products, thereby limiting the infection and inflammation to a specific microenvironment or compartment within the inflamed pulp (Figures 3D–F). In general, the inflammatory process usually begins at the site of bacterial entry and spreads circumferentially from one compartment to another (70, 71). Consequently, the co-existence of different histopathological conditions in various parts of the same pulp has previously been reported (72), making a single diagnosis for the entire pulp tissue impossible in most cases. Indeed, a range of classical studies reported no clear association between clinical signs and symptoms and the histological state of the pulp (73, 74). As a result, it has been considered impossible to accurately classify the pulpal condition and in particular to clearly differentiate between pulps that are reversibly or irreversibly inflamed (73). Notably histological studies determined that some teeth clinically diagnosed with irreversible pulpitis had histological features in common with reversible pulpitis (69). Furthermore, complete absence of inflammation and bacteria in the radicular pulp tissue of teeth clinically diagnosed with irreversible pulpitis was reported (Figure 3C). This is clinically relevant as it supports the use of pulpotomy techniques and removal of only the inflamed pulp tissue in teeth with irreversible pulpitis. It should be noted that reparative dentine formation could be observed in teeth with cariously exposed pulps and bacterial infection (Figure 2C), further supporting the above argument and questioning the traditional approach of diagnosing pulpitis as reversible or irreversible.
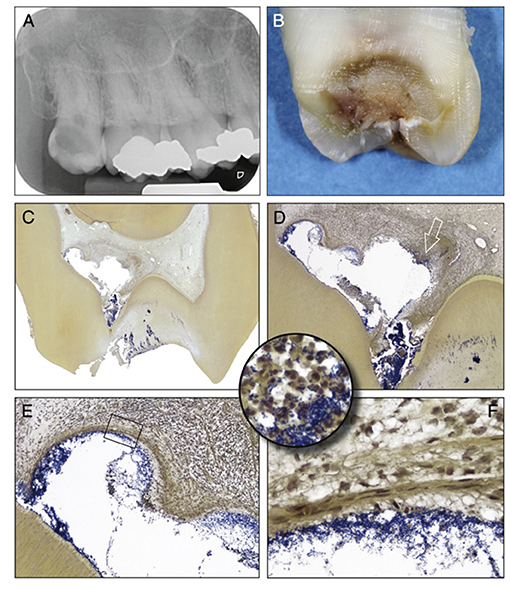
Figure 3. Clinical vs. histological diagnosis of pulpitis: tooth with deep caries showing different phases of destruction and inflammation reflecting different histological diagnosises. (A) Radiograph of a maxillary third molar from a patient with severe spontaneous pain and clinical diagnosis of irreversible pulpitis. (B) Buccolingual sectioning plane of the tooth showing the infected dentine. (C) Histological section showing the dentine and pulp chamber in. An abscess is present buccally (Taylor's modified Brown and Brenn, original magnification X8). (D) A detailed view of the abscess cavity with necrotic debris heavily colonized by bacteria. (E) Higher magnification view of the upper left portion of the abscess, the bacterial infiltration is limited by fibrous connective tissue. (F) High-power view of the rectangular area in (E) showing the fibrous connective tissue. (Circular inset) shows a high-power view of the area of the right portion of the abscess indicated by the arrow in (D). Increased accumulation of bacteria and PMNs are evident. [Figure adapted from Ricucci et al. (69), J Endod. Permission obtained from Elsevier].
Future Perspectives for Pulpitis Management
Persistent pulp inflammation is likely to delay and compromise the transition to the proliferative and remodeling phase to complete the repair process. Therefore, novel approaches to resolve chronic inflammation, based on creating an environment inductive of repair are discussed below.
Improve the Accuracy of Pulp Status Diagnosis
As discussed above the current description of pulpitis as reversible/irreversible is not “fit for purpose” as the development of minimally invasive approaches such as VPT have shown that maintenance of at least part of the pulp is possible in teeth displaying signs and symptoms of irreversible pulpitis (47). Clinical research is required to validate novel accurate diagnostic classifications of pulp disease status and related treatments (75). In addition, the current limitations of thermal and electric diagnostic tools for pulpitis should be addressed by developing more accurate and sophisticated molecular chairside tests. This is particularly relevant in cases of exposed pulps where blood or tissues obtained during routine treatment can be utilized on clinics in real-time to determine the level of appropriate biomarkers which subsequently enable accurate diagnosis and case selection. Biomarkers could also be collected and measured non-invasively in gingival crevicular fluid and dentinal fluid for pulpal diagnosis (19).
Deal With Bacterial Infection
Chronic pulp inflammation is sustained by the presence of a heavy bacterial burden. With the progression of caries, bacteria enter the pulp space and cause a localized infection resulting in a cariously-exposed pulp (76). Although sub-infection levels of bacteria have been shown to enhance the infiltration and function of neutrophils and monocytes/macrophages to accelerate healing, infection is likely to compromise the protective inflammatory phase, disrupt the normal inflammation-proliferation phase transition and consequently impair healing (77). It is therefore not surprising, that reactionary dentine formation reportedly occurs in the case of cavitated and non-cavitated lesion in the absence of bacterial invasion and infection of the dental pulp (78). Moreover, the progression of the carious lesion can result in different cellular responses within the pulp and different patterns of dentine formation (79), suggesting different bacterial biofilm growth conditions within the carious lesion (80).
In deep carious lesions with mild to moderate pulpal inflammation, removal of heavily infected dentine using stepwise excavation will reduce the bacterial load and following the placement of a restoration with a good seal, the maintenance of pulp vitality and dentine repair should be achieved (81). In cases of an exposed pulp, wound infection already exists and considerations should be given to cleaning the wound with an antibacterial irrigant, such as sodium hypochlorite and to ensure appropriate isolation to prevent further contamination with oral bacteria (82). VPTs such as pulp capping and pulpotomy procedures are indicated for managing cariously exposed pulps (5). Direct pulp capping is generally highly successful in non-infected traumatic exposure (83), but recent data showed high success rate for direct pulp capping of cariously exposed pulp using calcium silicate cements (84). These materials have a direct anti-bacterial action as well as tight sealing properties to prevent microleakage and subsequent infection. The latter property is considered critical for their superior histological and clinical performance compared with traditional calcium hydroxide preparations (85).
Harnessing Cellular Signaling Pathways in Pulpitis for Repair
The translation of extracellular signals surrounding the dental pulp to cellular responses is the result of a complex integration of numerous signaling pathways. These pathways regulate the inflammatory responses and therefore may directly influence the repair process in pulpitis. Among these, as has been previously highlighted, the NFκB and MAPK pathways are well-characterized in dental pulpal inflammatory and repair processes.
The NFκB pathway is one of the most important signaling cascades in the regulation of immune responses and considered a prototypical pro-inflammatory signaling pathway, largely based on its role in regulating the expression of numerous pro-inflammatory genes, including cytokines, chemokines and adhesion molecules (86). As many molecules with important functions in repair, including receptors for chemokines and GFs, mediate their effects through NF- kB pathway activation, its' signaling controls repair events through direct or indirect mechanisms. Indeed, many studies have already shown that this intracellular pathway mediates osteogeneic and odontogenic differentiation of DPSCs under a range of environmental contexts (87, 88). In an acute inflammatory response, NFkB signaling may mediate the production of cytokines or GFs that are known for their ability to induce repair. In situations, however, where the stimulus is likely to produce sustained and dysregulated activation of NFκB signaling, healing and repair are likely to be compromised (27). Therefore, reducing excessive and uncontrolled NFκB signaling is reportedly important for repair (89) and regulators of NFkB signaling such as TNFAIP3/A20 rather than complete inhibition should be considered for targeting for future pulp therapies.
MAPK pathway is another important signaling pathway in pulp inflammation. MAPKs comprise a large family of proline-directed, serine/threonine kinases with three subfamilies being described including; the ERK-1/2, JNK and p38 MAPK (90). ERKs are activated by mitogens and GFs, whereas JNK and p38 are activated by inflammatory cytokines, such as IL-1α/β and TNF-α (91). The pathway regulates translation of these extracellular stimuli into specific cellular responses, such as embryogenesis, cell proliferation, differentiation, and survival. Signal transduction via MAPK pathways regulate several pulpal reparative processes (88, 92, 93). Data from these studies and others have demonstrated that this signaling pathway can be activated in dental cells by inflammation-related molecules, including bacterial components (88), ROS (94), and cytokines (25). MAPK dependent signaling in these studies reportedly directly drives in vitro mineralization and differentiation of DPSCs. It is therefore not surprising that several biomaterials which are used successfully as pulp capping agents to induced dentine repair, such as hydraulic tricalcium silicate cements MTA (ProRoot MTA Dentsply, Tulsa, OK, USA) and Biodentine (Septodont, Saint-Maur-des-Fossés, France) mediate their effects via the MAPK signaling pathway (95, 96).
Next Generation Biomaterials and Pulp Regenerative Therapies
Treatment of deep carious lesions with indirect, direct pulp capping and pulpotomy procedures provides an opportunity for pulpal healing and repair and the formation of a reparative mineralised barrier. The placement of biomaterials such as MTA and other hydraulic tricalcium silicate cements potentially facilitates recruitment of DPSCs, the release of GFs and subsequent production of reparative dentine (97). Currently, the success of the traditional pulpotomy procedure is measured only by the absence of symptoms and the formation of a calcific barrier; however, the procedure lacks the potential for the regeneration of the removed coronal pulp tissue. Thus, the ideal treatment for teeth requiring pulpotomy will be a therapeutic option that allows for regeneration of the coronal pulp tissue previously damaged by infection and inflammation. Pulp regeneration can be defined as the formation of new pulp tissue using the tissue-engineering concepts which include stem cells, scaffolds and signaling molecules. Indeed, complete and partial pulp regeneration can potentially be achieved using these principles. The dental pulp is well-populated with mesenchymal stem cells, and partial pulp regeneration using existing pulp stem cells and GFs in the presence or absence of scaffolds has already been reported (98). The challenges however associated with partial regeneration of the cariously exposed and inflamed pulp include the control of inflammation and bacterial contamination, as well as the predictable recruitment of stem cells which can be activated to differentiate into the desired cell types. Importantly, however, properties of DPSCs derived from inflamed pulp were shown to be comparable to those derived from healthy tissue indicating the potential for these cells to be harnessed for regenerative purposes (99). The availability of functionalized scaffolds that can act as effective carriers for biological molecules also provides an opportunity for the control of inflammation and the delivery of GFs to modify the tissue microenvironment in favor of regeneration (100). Alternatively, scaffolds can be engineered to interact with the dentine matrix, to enable the release of local GFs and other bioactive molecules fossilized within the structure (101). The development of self-assembling peptides scaffolds with good biocompatibility, handling properties, and potential as carrier vehicles for anti-inflammatory, antimicrobial and other bioactive molecules provide a significant opportunity to overcome the challenges to enable the regeneration of the diseased pulp (102). Evidence supports the use of these peptide scaffolds for pulp regeneration in a murine xenograft model. However, further work is needed to explore the potential of these and similar approaches for controlling pulp inflammation and ultimately enabling regeneration of dental pulp tissue.
Conclusion
The ability of the inflamed pulp to heal is proven with the available clinical evidence, although preliminary that supports a front-line role for VPT in the treatment of symptomatic deep carious lesion in permanent teeth. There is clearly a need for better diagnostic approaches to accurately assess the level of pulpal inflammation as this can be used to indicate an appropriate treatment modality. However, more conservative treatment methodologies, focusing on, and utilizing recent development in biomaterials and regenerative techniques will provide the 5way forward for VPTs.
Author Contributions
IE and HD contributed to conception, design, and drafting the manuscript. FL, PC, PT, and IA contributed to writing, editing, and revising the manuscript. All authors agree to be accountable for the content of the work.
Conflict of Interest
The authors declare that the research was conducted in the absence of any commercial or financial relationships that could be construed as a potential conflict of interest.
Abbreviations
AP-1, Activator protein-1; CGRP, Calcitonin related peptide; DPSCs, Dental pulp stem cells; ERK, Extracellular signal-regulated kinase; IL-1α, Interleukin-1α; IL-2, Interleukin 2; IL-6, Interleukin 6; IL-8, Interleukin-8; IL-10, Interleukin-10; IL-12, Interleukin 12; IL-β, Interleukin- 1β; IRAK, Interleukin-1 receptor-associated kinase, JNK, The c-jun N-terminal kinase; MAPK, Mitogen activated protein kinase; MTA, Mineral trioxide aggregate, NFKB, Nuclear factor kappa b; NLRs, Nod like receptors; PAMPs, Pathogen associated molecular patterns; PRR, Pattern recognition receptor; ROS, Reactive oxygen species; SOCS, Suppressor of cytokine signaling; SP, Substance P; STAT, Signal transducer and activator of transcription; TGFβ, Transformer growth factor β; TLR, Toll like receptor; TNF-α, Tumor necrosis factor alpha; TNFAIP3, TNF-alpha induced protein 3; VPT, Vital pulp treatment.
References
1. Kassebaum NJ, Bernabé E, Dahiya M, Bhandari B, Murray CJL, and Marcenes W. Global burden of untreated caries: a systematic review and metaregression. J Dent Res. (2015) 94:650–8. doi: 10.1177/0022034515573272
2. Careddu R, Plotino G, Cotti E, and Duncan HF. The management of deep carious lesions and the exposed pulp amongst members of two European endodontic societies: a questionnaire-based study. Int Endod J. (2021) 54:366–76. doi: 10.1111/iej.13418
3. Kidd E. The implications of the new paradigm of dental caries. J Dent. (2011) 39(Suppl. 2):S3–8. doi: 10.1016/j.jdent.2011.11.004
4. Cooper PR, Chicca IJ, Holder MJ, and Milward MR. Inflammation and regeneration in the Dentin-pulp complex: net gain or net loss? J Endod. (2017) 43:S87–94. doi: 10.1016/j.joen.2017.06.011
5. Duncan HF, Galler KM, Tomson PL, Simon S, El-Karim I, Kundzina R, et al. European society of endodontology position statement: management of deep caries and the exposed pulp. Int Endod J. (2019) 52:923–34. doi: 10.1111/iej.13080
6. Levin LG, Law AS, Holland GR, Abbott PV, and Roda RS. Identify and define all diagnostic terms for pulpal health and disease states. J Endod. (2009) 35:1645–57. doi: 10.1016/j.joen.2009.09.032
7. Thylstrup A, Leach SA, and Qvist V. Optical properties of dentin. In: Thylstrup A, Leach SA, Qvist V, editors. Dentine and Dentine Reactions in the Oral Cavity. Oxford: Oxford University Press (1987). p. 34–40.
8. Ricucci D, Siqueira JF, Li Y, and Tay FR. Vital pulp therapy: histopathology and histobacteriology-based guidelines to treat teeth with deep caries and pulp exposure. J Dent. (2019) 86:41–52. doi: 10.1016/j.jdent.2019.05.022
9. Smith AJ, Duncan HF, Diogenes A, Simon S, and Cooper PR. Exploiting the bioactive properties of the dentin-pulp complex in regenerative endodontics. J Endod. (2016) 42:47–56. doi: 10.1016/j.joen.2015.10.019
10. Krafts KP. Tissue repair: the hidden drama. Organogenesis. (2010) 6:225–33. doi: 10.4161/org.6.4.12555
11. Yu T, Volponi AA, Babb R, An Z, and Sharpe PT. Stem cells in tooth development, growth, repair, and regeneration. Curr Topics Dev Biol. (2015) 115:187–212. doi: 10.1016/bs.ctdb.2015.07.010
12. Widbiller M, and Schmalz G. Endodontic regeneration: hard shell, soft core. Odontology. (2020). doi: 10.1007/s10266-020-00573-1. [Epub ahead of print].
13. Tjäderhane L. The mechanism of pulpal wound healing. Aust Endod J. (2002) 28:68–74. doi: 10.1111/j.1747-4477.2002.tb00386.x
14. Hahn C Lo, and Liewehr FR. Innate immune responses of the dental pulp to caries. J Endod. (2007) 33:643–51. doi: 10.1016/j.joen.2007.01.001
15. Goldberg M, and Smith AJ. Cells and extracellular matrices of dentin and pulp: a biological basis for repair and tissue engineering. Crit Rev Oral Biol Med. (2004) 15:13–27. doi: 10.2485/jhtb.13.55
16. Farges JC, Alliot-Licht B, Renard E, Ducret M, Gaudin A, Smith AJ, et al. Dental pulp defence and repair mechanisms in dental caries. Mediators Inflamm. (2015) 2015:230251. doi: 10.1155/2015/230251
17. Silva ACO, Faria MR, Fontes A, Campos MS, and Cavalcanti BN. Interleukin-1 beta and interleukin-8 in healthy and inflamed dental pulps. J Appl Oral Sci. (2009) 17:527–32. doi: 10.1590/S1678-77572009000500031
18. McLachlan JL, Smith AJ, Bujalska IJ, and Cooper PR. Gene expression profiling of pulpal tissue reveals the molecular complexity of dental caries. Biochim Biophys Acta Mol Basis Dis. (2005) 1741:271–81. doi: 10.1016/j.bbadis.2005.03.007
19. Rechenberg DK, Galicia JC, and Peters OA. Biological markers for pulpal inflammation: a systematic review. PLoS ONE. (2016) 11:e0167289. doi: 10.1371/journal.pone.0167289
20. Koch AE, Polverini PJ, Kunkel SL, Harlow LA, DiPietro LA, Elner VM, et al. Interleukin-8 as a macrophage-derived mediator of angiogenesis. Science. (1992) 258:1798–801. doi: 10.1126/science.1281554
21. Zhai S, Wang Y, Jiang W, Jia Q, Li J, Wang W, et al. Nemotic human dental pulp fibroblasts promote human dental pulp stem cells migration. Exp Cell Res. (2013) 319:1544–52. doi: 10.1016/j.yexcr.2013.03.018
22. Ueda M, Fujisawa T, Ono M, Hara ES, Pham HT, Nakajima R, et al. A short-term treatment with tumor necrosis factor-alpha enhances stem cell phenotype of human dental pulp cells. Stem Cell Res Ther. (2014) 5:31. doi: 10.1186/scrt420
23. Qin Z, Li Y, Li Y, and Liu G. Tumor necrosis factor alpha stimulates proliferation of dental pulp stem cells via akt/glycogen synthase kinase-3β/cyclin D1 signaling pathway. J Endod. (2015) 41:1066–72. doi: 10.1016/j.joen.2015.02.020
24. Yang X, Zhang S, Pang X, and Fan M. Pro-inflammatory cytokines induce odontogenic differentiation of dental pulp-derived stem cells. J Cell Biochem. (2012) 113:669–77. doi: 10.1002/jcb.23396
25. Paula-Silva FWG, Ghosh A, Silva LAB, and Kapila YL. TNF-α promotes an odontoblastic phenotype in dental pulp cells. J Dent Res. (2009) 88:339–44. doi: 10.1177/0022034509334070
26. Qin Z, Fang Z, Zhao L, Chen J, Li Y, and Liu G. High dose of TNF-α suppressed osteogenic differentiation of human dental pulp stem cells by activating the Wnt/β-catenin signaling. J Mol Histol. (2015) 46:409–20. doi: 10.1007/s10735-015-9630-7
27. Boyle M, Chun C, Strojny C, Narayanan R, Bartholomew A, Sundivakkam P, et al. Chronic inflammation and angiogenic signaling axis impairs differentiation of dental-pulp stem cells. PLoS ONE. (2014) 9:e0113419. doi: 10.1371/journal.pone.0113419
28. El karim IA, Linden GJ, Irwin CR, and Lundy FT. Neuropeptides regulate expression of angiogenic growth factors in human dental pulp fibroblasts. J Endod. (2009) 35:829–33. doi: 10.1016/j.joen.2009.03.005
29. El Karim IA, Lamey PJ, Linden GJ, Awawdeh LA, and Lundy FT. Caries-induced changes in the expression of pulpal neuropeptide Y. Eur J Oral Sci. (2006) 114:133–7. doi: 10.1111/j.1600-0722.2006.00343.x
30. Awawdeh L, Lundy FT, Shaw C, Lamey PJ, Linden GJ, and Kennedy JG. Quantitative analysis of substance P, neurokinin A and calcitonin gene-related peptide in pulp tissue from painful and healthy human teeth. Int Endod J. (2002) 35:30–6. doi: 10.1046/j.1365-2591.2002.00451.x
31. Byers MR, and Taylor PE. Effect of sensory denervation on the response of rat molar pulp to exposure injury. J Dent Res. (1993) 72:613–8. doi: 10.1177/00220345930720031001
32. Olgart L. Neural control of pulpal blood flow. Crit Rev Oral Biol Med. (1996) 7:159–71. doi: 10.1177/10454411960070020401
33. El Karim IA, Linden GJ, Orr DF, and Lundy FT. Antimicrobial activity of neuropeptides against a range of micro-organisms from skin, oral, respiratory and gastrointestinal tract sites. J Neuroimmunol. (2008) 200:11–6. doi: 10.1016/j.jneuroim.2008.05.014
34. El Karim IA, Linden GJ, Curtis TM, About I, McGahon MK, Irwin CR, et al. Human odontoblasts express functional thermo-sensitive TRP channels: implications for dentin sensitivity. Pain. (2011) 152:2211–23. doi: 10.1016/j.pain.2010.10.016
35. Farges JC, Keller JF, Carrouel F, Durand SH, Romeas A, Bleicher F, et al. Odontoblasts in the dental pulp immune response. J Exp Zool Part B Mol Dev Evol. (2009) 312:425–36. doi: 10.1002/jez.b.21259
36. El Karim IA, Linden GJ, Curtis TM, About I, McGahon MK, Irwin CR, et al. Human dental pulp fibroblasts express the “cold-sensing” transient receptor potential channels TRPA1 and TRPM8. J Endod. (2011) 37:473–8. doi: 10.1016/j.joen.2010.12.017
37. Chmilewsky F, Jeanneau C, Laurent P, and About I. Pulp fibroblasts synthesize functional complement proteins involved in initiating dentin-pulp regeneration. Am J Pathol. (2014) 184:1991–2000. doi: 10.1016/j.ajpath.2014.04.003
38. Rufas P, Jeanneau C, Rombouts C, Laurent P, and About I. Complement C3a mobilizes dental pulp stem cells and specifically guides pulp fibroblast recruitment. J Endod. (2016) 42:1377–84. doi: 10.1016/j.joen.2016.06.011
39. Li Z, Jiang CM, An S, Cheng Q, Huang YF, Wang YT, et al. Immunomodulatory properties of dental tissue-derived mesenchymal stem cells. Oral Dis. (2014) 20:25–34. doi: 10.1111/odi.12086
40. Téclès O, Laurent P, Zygouritsas S, Burger AS, Camps J, Dejou J, et al. Activation of human dental pulp progenitor/stem cells in response to odontoblast injury. Arch Oral Biol. (2005) 50:103–8. doi: 10.1016/j.archoralbio.2004.11.009
41. Wynn TA, and Vannella KM. Macrophages in tissue repair, regeneration, and fibrosis. Immunity. (2016) 44:450–62. doi: 10.1016/j.immuni.2016.02.015
42. Frangogiannis NG. Regulation of the inflammatory response in cardiac repair. Circ Res. (2012) 110:159–73. doi: 10.1161/CIRCRESAHA.111.243162
43. Matsukawa A, Kudo S, Maeda T, Numata K, Watanabe H, Takeda K, et al. Stat3 in resident macrophages as a repressor protein of inflammatory response. J Immunol. (2005) 175:3354–9. doi: 10.4049/jimmunol.175.5.3354
44. Hahn C Lo, Best AM, and Tew JG. Cytokine induction by Streptococcus mutans and pulpal pathogenesis. Infect Immun. (2000) 68:6785–9. doi: 10.1128/IAI.68.12.6785-6789.2000
45. Elsalhy M, Azizieh F, and Raghupathy R. Cytokines as diagnostic markers of pulpal inflammation. Int Endod J. (2013) 46:573–80. doi: 10.1111/iej.12030
46. Alqaderi H, Lee CT, Borzangy S, and Pagonis TC. Coronal pulpotomy for cariously exposed permanent posterior teeth with closed apices: a systematic review and meta-analysis. J Dent. (2016) 44:1–7. doi: 10.1016/j.jdent.2015.12.005
47. Cushley S, Duncan HF, Lappin MJ, Tomson PL, Lundy FT, Cooper P, et al. Pulpotomy for mature carious teeth with symptoms of irreversible pulpitis: a systematic review. J Dent. (2019) 88:103158. doi: 10.1016/j.jdent.2019.06.005
48. McLachlan JL, Smith AJ, Sloan AJ, and Cooper PR. Gene expression analysis in cells of the dentine-pulp complex in healthy and carious teeth. Arch Oral Biol. (2003) 48:273–83. doi: 10.1016/S0003-9969(03)00003-7
49. Sloan AJ, Perry H, Matthews JB, and Smith AJ. Transforming growth factor-β isoform expression in mature human healthy and carious molar teeth. Histochem J. (2000) 32:247–52. doi: 10.1023/A:1004007202404
50. Piattelli A, Rubini C, Fioroni M, Tripodi D, and Strocchi R. Transforming growth factor-beta 1 (TGF-beta 1) expression in normal healthy pulps and in those with irreversible pulpitis. Int Endod J. (2004) 37:114–9. doi: 10.1111/j.0143-2885.2004.00758.x
51. Colombo JS, Moore AN, Hartgerink JD, and D'Souza RN. Scaffolds to control inflammation and facilitate dental pulp regeneration. J Endod. (2014) 40(Suppl. 4):S6–12. doi: 10.1016/j.joen.2014.01.019
52. Xu H, Chen J, Ge J, Xia K, Tao S, Su Y, et al. Resolvin E1 ameliorates pulpitis by suppressing dental pulp fibroblast activation in a chemerin receptor 23-dependent manner. J Endod. (2019) 45:1126–34.e1. doi: 10.1016/j.joen.2019.05.005
53. Kobayashi K, Hernandez LD, Galán JE, Janeway CA, Medzhitov R, and Flavell RA. IRAK-M is a negative regulator of Toll-like receptor signaling. Cell. (2002) 110:191–202. doi: 10.1016/S0092-8674(02)00827-9
54. Coornaert B, Carpentier I, and Beyaert R. A20: Central gatekeeper in inflammation and immunity. J Biol Chem. (2009) 284:8217–21. doi: 10.1074/jbc.R800032200
55. Arthur A, Shi S, and Gronthos S. Dental pulp stemcells. In: Vishwakarma A, Sharpe P, Shi S, Ramalingam M, editors. Stem Cell Biology and Tissue Engineering in Dental Sciences. Amsterdam: Elsevier (2015). p. 279–89.
56. Yianni V, and Sharpe PT. Molecular programming of perivascular stem cell precursors. Stem Cells. (2018) 36:1890–904. doi: 10.1002/stem.2895
57. Ricucci D, Loghin S, Lin LM, Spångberg LSW, and Tay FR. Is hard tissue formation in the dental pulp after the death of the primary odontoblasts a regenerative or a reparative process? J Dent. (2014) 42:1156–70. doi: 10.1016/j.jdent.2014.06.012
58. Izumi T, Inoue H, Matsuura H, Mukae F, Osoegawa H, Hirano H, et al. Changes in the pattern of horseradish peroxidase diffusion into predentin and dentin after cavity preparation in rat molars. Oral Surg Oral Med Oral Pathol Oral Radiol Endod. (2001) 92:675–81. doi: 10.1067/moe.2001.117264
59. Higashi T, and Okamoto H. Characteristics and effects of calcified degenerative zones on the formation of hard tissue barriers in amputated canine dental pulp. J Endod. (1996) 22:168–72. doi: 10.1016/S0099-2399(96)80094-X
60. Mejàre IA, Axelsson S, Davidson T, Frisk F, Hakeberg M, Kvist T, et al. Diagnosis of the condition of the dental pulp: a systematic review. Int Endod J. (2012) 45:597–613. doi: 10.1111/j.1365-2591.2012.02016.x
61. El Karim IA, McCrudden MTC, McGahon MK, Curtis TM, Jeanneau C, Giraud T, et al. Biodentine reduces tumor necrosis factor alpha-induced TRPA1 expression in odontoblastlike cells. J Endod. (2016) 42:589–95. doi: 10.1016/j.joen.2015.12.017
62. Yu C, and Abbott P. An overview of the dental pulp: its functions and responses to injury. Aust Dent J. (2007) 52:S4–6. doi: 10.1111/j.1834-7819.2007.tb00525.x
63. Kim S. Microcirculation of the dental pulp in health and disease. J Endod. (1985) 11:465–71. doi: 10.1016/S0099-2399(85)80219-3
64. Kim S, Schuessler G, and Chien S. Measurement of blood flow in the dental pulp of dogs with the 133xenon washout method. Arch Oral Biol. (1983) 28:501–5. doi: 10.1016/0003-9969(83)90181-4
65. Kim S, Lipowsky HH, Usami S, and Chien S. Arteriovenous distribution of hemodynamic parameters in the rat dental pulp. Microvasc Res. (1984) 27:28–38. doi: 10.1016/0026-2862(84)90039-6
66. Van Hassel HJ. Physiology of the human dental pulp. Oral Surg Oral Med Oral Pathol. (1971) 32:126–34. doi: 10.1016/0030-4220(71)90258-1
67. Tønder KJH, and Kvinnsland I. Micropuncture measurements of interstitial fluid pressure in normal and inflamed dental pulp in cats. J Endod. (1983) 9:105–9. doi: 10.1016/S0099-2399(83)80106-X
68. Heyeraas KJ, and Berggreen E. Interstitial fluid pressure in normal and inflamed pulp. Crit Rev Oral Biol Med. (1999) 10:328–36. doi: 10.1177/10454411990100030501
69. Ricucci D, Loghin S, and Siqueira JF. Correlation between clinical and histologic pulp diagnoses. J Endod. (2014) 40:1932–9. doi: 10.1016/j.joen.2014.08.010
70. Langeland K. Tissue response to dental caries. Dent Traumatol. (1987) 3:149–71. doi: 10.1111/j.1600-9657.1987.tb00619.x
71. Ricucci D, and Siqueira JF. Fate of the tissue in lateral canals and apical ramifications in response to pathologic conditions and treatment procedures. J Endod. (2010) 36:1–15. doi: 10.1016/j.joen.2009.09.038
72. Baume LJ. Diagnosis of diseases of the pulp. Oral Surg Oral Med Oral Pathol. (1970) 29:102–16. doi: 10.1016/0030-4220(70)90416-0
73. Dummer PMH, Hicks R, and Huws D. Clinical signs and symptoms in pulp disease. Int Endod J. (1980) 13:27–35. doi: 10.1111/j.1365-2591.1980.tb00834.x
74. Seltzer S, Bender IB, and Ziontz M. The dynamics of pulp inflammation: correlations between diagnostic data and actual histologic findings in the pulp. Oral Surg Oral Med Oral Pathol. (1963) 16:969–77. doi: 10.1016/0030-4220(63)90201-9
75. Wolters WJ, Duncan HF, Tomson PL, Karim IE, McKenna G, Dorri M, et al. Minimally invasive endodontics: a new diagnostic system for assessing pulpitis and subsequent treatment needs. Int Endod J. (2017) 50:825–9. doi: 10.1111/iej.12793
76. Martin FE. Carious pulpitis: microbiological and histopathological considerations. Aust Endod J. (2003) 29:134–7. doi: 10.1111/j.1747-4477.2003.tb00538.x
77. Jones SG, Edwards R, and Thomas DW. Inflammation and wound healing: the role of bacteria in the immuno-regulation of wound healing. Int J Low Extrem Wounds. (2004) 3:201–8. doi: 10.1177/1534734604271810
78. Ricketts DNJ, Ekstrand KR, Kidd EAM, and Larsen T. Relating visual and radiographic ranked scoring systems for occlusal caries detection to histological and microbiological evidence. Oper Dent. (2002) 27:231–7.
79. Bjørndal L, and Darvann T. A light microscopic study of odontoblastic and non-odontoblastic cells involved in tertiary dentinogenesis in well-defined cavitated carious lesions. Caries Res. (1999) 33:50–60. doi: 10.1159/000016495
80. Lucchini M, Romeas A, Couble ML, Bleicher F, Magloire H, and Farges JC. TGFβ1 signaling and stimulation of osteoadherin in human odontoblasts in vitro. Connect Tissue Res. (2002) 43:345–53. doi: 10.1080/03008200290000790
81. Schwendicke F, Frencken JE, Bjørndal L, Maltz M, Manton DJ, Ricketts D, et al. Managing carious lesions: consensus recommendations on carious tissue removal. Adv Dental Res. (2016) 28:58–67. doi: 10.1177/0022034516639271
82. Ballal NV, Duncan HF, Rai N, Jalan P, and Zehnder M. Sodium hypochlorite reduces postoperative discomfort and painful early failure after carious exposure and direct pulp capping-initial findings of a randomized controlled trial. J Clin Med. (2020) 9:2408. doi: 10.3390/jcm9082408
83. Murray PE, Windsor LJ, Smyth TW, Hafez AA, and Cox CF. Analysis of pulpal reactions to restorative procedures, materials, pulp capping, and future therapies. Crit Rev Oral Biol Med. (2002) 13:509–20. doi: 10.1177/154411130201300607
84. Cushley S, Duncan H, Lappin M, Chua P, Elamin A, Clarke M, et al. Efficacy of direct pulp capping for management of cariously exposed pulps in permanent teeth: a systematic review and meta-analysis. Int Endod J. (2020) doi: 10.1111/iej.13449. [Epub ahead of print].
85. Nair PNR, Duncan HF, Pitt Ford TR, and Luder HU. Histological, ultrastructural and quantitative investigations on the response of healthy human pulps to experimental capping with mineral trioxide aggregate: a randomized controlled trial. Int Endod J. (2009) 42:422–44. doi: 10.1111/j.1365-2591.2009.01558.x
86. Bonizzi G, and Karin M. The two NF-κB activation pathways and their role in innate and adaptive immunity. Trends Immunol. (2004) 25:280–8. doi: 10.1016/j.it.2004.03.008
87. Feng X, Feng G, Xing J, Shen B, Li L, Tan W, et al. TNF-α triggers osteogenic differentiation of human dental pulp stem cells via the NF-κB signalling pathway. Cell Biol Int. (2013) 37:1267–75. doi: 10.1002/cbin.10141
88. He W, Wang Z, Luo Z, Yu Q, Jiang Y, Zhang Y, et al. LPS Promote the odontoblastic differentiation of human dental pulp stem cells via MAPK signaling pathway. J Cell Physiol. (2015) 230:554–61. doi: 10.1002/jcp.24732
89. Hozhabri NST, Benson MD, Vu MD, Patel RH, Martinez RM, Nakhaie FN, et al. Decreasing NF-κB expression enhances odontoblastic differentiation and collagen expression in dental pulp stem cells exposed to inflammatory cytokines. PLoS ONE. (2015) 10:e0113334. doi: 10.1371/journal.pone.0113334
90. Arthur JSC, and Ley SC. Mitogen-activated protein kinases in innate immunity. Nat Rev Immunol. (2013) 13:679–92. doi: 10.1038/nri3495
91. Kyriakis JM, and Avruch J. Sounding the alarm: protein kinase cascades activated by stress and inflammation. J Biol Chem. (1996) 271:24313–6. doi: 10.1074/jbc.271.40.24313
92. Simon S, Smith AJ, Berdal A, Lumley PJ, and Cooper PR. The MAP kinase pathway is involved in odontoblast stimulation via p38 phosphorylation. J Endod. (2010) 36:256–9. doi: 10.1016/j.joen.2009.09.019
93. Wang Y, Yan M, Fan Z, Ma L, Yu Y, and Yu J. Mineral trioxide aggregate enhances the odonto/osteogenic capacity of stem cells from inflammatory dental pulps via NF-κB pathway. Oral Dis. (2014) 20:650–8. doi: 10.1111/odi.12183
94. Lee DH, Lim BS, Lee YK, and Yang HC. Effects of hydrogen peroxide (H2O2) on alkaline phosphatase activity and matrix mineralization of odontoblast and osteoblast cell lines. Cell Biol Toxicol. (2006) 22:39–46. doi: 10.1007/s10565-006-0018-z
95. Zhao X, He W, Song Z, Tong Z, Li S, and Ni L. Mineral trioxide aggregate promotes odontoblastic differentiation via mitogen-activated protein kinase pathway in human dental pulp stem cells. Mol Biol Rep. (2012) 39:215–20. doi: 10.1007/s11033-011-0728-z
96. Jung JY, Woo SM, Lee BN, Koh JT, Nör JE, and Hwang YC. Effect of biodentine and bioaggregate on odontoblastic differentiation via mitogen-activated protein kinase pathway in human dental pulp cells. Int Endod J. (2015) 48:177–84. doi: 10.1111/iej.12298
97. Tomson PL, Lumley PJ, Smith AJ, and Cooper PR. Growth factor release from dentine matrix by pulp-capping agents promotes pulp tissue repair-associated events. Int Endod J. (2017) 50:281–92. doi: 10.1111/iej.12624
98. Nakashima M, and Akamine A. The application of tissue engineering to regeneration of pulp and dentin in endodontics. J Endod. (2005) 31:711–8. doi: 10.1097/01.don.0000164138.49923.e5
99. Pereira LO, Rubini MR, Silva JR, Oliveira DM, Silva ICR, Poças-Fonseca MJ, et al. Comparison of stem cell properties of cells isolated from normal and inflamed dental pulps. Int Endod J. (2012) 45:1080–90. doi: 10.1111/j.1365-2591.2012.02068.x
100. Piva E, Silva AF, and Nör JE. Functionalized scaffolds to control dental pulp stem cell fate. J Endod. (2014) 40(Suppl. 4):S33–40. doi: 10.1016/j.joen.2014.01.013
101. Duncan HF, Kobayashi Y, and Shimizu E. Growth factors and cell homing in dental tissue regeneration. Curr Oral Heal Rep. (2018) 5:276–85. doi: 10.1007/s40496-018-0194-y
Keywords: pulpitis, vital pulp treatment, deep caries, reparative dentine, pulp wound healing, biomaterials
Citation: El karim IA, Cooper PR, About I, Tomson PL, Lundy FT and Duncan HF (2021) Deciphering Reparative Processes in the Inflamed Dental Pulp. Front. Dent. Med. 2:651219. doi: 10.3389/fdmed.2021.651219
Received: 08 January 2021; Accepted: 19 February 2021;
Published: 31 March 2021.
Edited by:
Bruno Cavalcanti, University of Michigan, United StatesReviewed by:
Matthias Widbiller, University of Regensburg, GermanyNaoto Ohkura, Niigata University, Japan
Copyright © 2021 El karim, Cooper, About, Tomson, Lundy and Duncan. This is an open-access article distributed under the terms of the Creative Commons Attribution License (CC BY). The use, distribution or reproduction in other forums is permitted, provided the original author(s) and the copyright owner(s) are credited and that the original publication in this journal is cited, in accordance with accepted academic practice. No use, distribution or reproduction is permitted which does not comply with these terms.
*Correspondence: Ikhlas A. El karim, aS5lbGthcmltJiN4MDAwNDA7cXViLmFjLnVr