- 1Jean and Paul Amos Parkinson's Disease and Movement Disorder Program, Department of Neurology, Emory University, Atlanta, GA, United States
- 2Department of Neurology, University of California, San Diego, La Jolla, CA, United States
- 3Center for Advanced Neuroimaging, University of California, Riverside, Riverside, CA, United States
- 4Department of Biomedical Informatics, Emory University, Atlanta, GA, United States
- 5Department of Bioengineering, University of California, Riverside, Riverside, CA, United States
Substantia nigra pars compacta (SNc) and locus coeruleus (LC) are neuromelanin-rich nuclei implicated in diverse cognitive and motor processes in normal brain function and disease. However, their roles in aging and neurodegenerative disease mechanisms have remained unclear due to a lack of tools to study them in vivo. Preclinical and post-mortem human investigations indicate that the relationship between tissue neuromelanin content and neurodegeneration is complex. Neuromelanin exhibits both neuroprotective and cytotoxic characteristics, and tissue neuromelanin content varies across the lifespan, exhibiting an inverted U-shaped relationship with age. Neuromelanin-sensitive MRI (NM-MRI) is an emerging modality that allows measurement of neuromelanin-associated contrast in SNc and LC in humans. NM-MRI robustly detects disease effects in these structures in neurodegenerative conditions, including Parkinson's disease (PD). Previous NM-MRI studies of PD have largely focused on detecting disease group effects, but few studies have reported NM-MRI correlations with phenotype. Because neuromelanin dynamics are complex, we hypothesize that they are best interpreted in the context of both disease stage and aging, with neuromelanin loss correlating with symptoms most clearly in advanced stages where neuromelanin loss and neurodegeneration are coupled. We tested this hypothesis using NM-MRI to measure SNc and LC volumes in healthy older adult control individuals and in PD patients with and without freezing of gait (FOG), a severe and disabling PD symptom. We assessed for group differences and correlations between NM-MRI measures and aging, cognition and motor deficits. SNc volume was significantly decreased in PD with FOG compared to controls. SNc volume correlated significantly with motor symptoms and cognitive measures in PD with FOG, but not in PD without FOG. SNc volume correlated significantly with aging in PD. When PD patients were stratified by disease duration, SNc volume correlated with aging, cognition, and motor deficits only in PD with disease duration >5 years. We conclude that in severe or advanced PD, identified by either FOG or disease duration >5 years, the observed correlations between SNc volume and aging, cognition, and motor function may reflect the coupling of neuromelanin loss with neurodegeneration and the associated emergence of a linear relationship between NM-MRI measures and phenotype.
Introduction
The neuromelanin-rich catecholamine nuclei, substantia nigra pars compacta (SNc) and locus coeruleus (LC), are implicated in diverse cognitive and motor processes in normal physiology and disease states. However, in vivo study of their roles in human brain function has remained difficult due to a lack of adequate tools. It has also been challenging to clarify the mechanistic role of neuromelanin in Parkinson's disease (PD) because its roles in normal cellular functioning and in disease states are complex. Neuromelanin exhibits both neuroprotective and cytotoxic characteristics in SNc and LC neurons, and is impacted differently by the aging process and neurodegenerative disease (Zecca et al., 2003). In humans neuromelanin pigment first appears at about age three and it accumulates with age (Mann and Yates, 1974; Bogerts, 1981; Zecca et al., 2001, 2002, 2008; Sulzer et al., 2008). Neuromelanin formation is itself a neuroprotective process, which involves removal of neurotoxic substances from the cytosol (Zecca et al., 2006; Zucca et al., 2017). Its synthesis is driven by excess cytosolic dopamine in catecholamine neurons, and it sequesters iron, toxic dopamine metabolites (e.g., dopamine-o-quinone), and a mixture of aggregated proteins, including α-synuclein (Sulzer et al., 2000; Zucca et al., 2017). By sequestering these substances neuromelanin synthesis prevents neurodegeneration (Zecca et al., 2003).
The accumulation of neuromelanin with age does not continue indefinitely, however, and neuromelanin content in SNc and LC peaks in late middle age, between about age 50 and 60, and then declines (Fearnley and Lees, 1991; Manaye et al., 1995; Shibata et al., 2006; Xing et al., 2018). Consequently, over the human lifespan SNc and LC neuromelanin content follows an inverted U-shaped curve (Manaye et al., 1995; Xing et al., 2018). In PD and related forms of neurodegenerative parkinsonism selective loss of melanized neurons occurs in these structures, but the mechanistic role of neuromelanin in these conditions has not been clarified (Fearnley and Lees, 1991; Damier et al., 1999; Zarow et al., 2003; Benarroch, 2023). The early accumulation of neuromelanin during periods of normal brain function, which is then followed by a decline that occurs in parallel with neurodegeneration implies a possible role for neuromelanin in PD pathogenesis. Exploration of this hypothesis in animal models has been limited, though, because rodents do not produce neuromelanin, and therefore rodent models of PD have not included study of its biology. This has changed, recently, with the development of a new rodent model of PD that produces neuromelanin in SNc (Carballo-Carbajal et al., 2019). Work using this model demonstrates that after a threshold concentration is reached, gradually increasing levels of intracellular neuromelanin are associated with cell sickness, with an initial loss of protein expression followed by nigrostriatal neurodegeneration (Vila, 2019; Vila et al., 2019). The model develops Lewy body-like inclusions, and a hypokinetic parkinsonian phenotype. Furthermore, the phenotype can be rescued with an over-expression of vesicular monoamine transporter 2 (VMAT2), which causes increased sequestration of cytosolic dopamine, thereby decreasing neuromelanin-synthesis (Gonzalez-Sepulveda et al., 2023). Other research has shown that neurodegeneration-associated release of neuromelanin granules into the extracellular space activates microglia and drives neuroinflammation (Orr et al., 2005; Zecca et al., 2006; Viceconte et al., 2015). Clearance of neuromelanin from SNc in advanced PD is a long-established finding on gross pathology, and post-mortem studies suggest that activated microglia may clear extracellular neuromelanin, which accumulates secondary to neuronal death (Orr et al., 2005; Beach et al., 2007; Huddleston et al., 2018). The complex dynamics of neuromelanin in the biology of aging and neurodegenerative disease are depicted schematically in Figure 1. In combination, these insights suggest that in vivo monitoring of tissue neuromelanin content may yield insights into the biology of parkinsonian neurodegeneration, and modulation of neuromelanin biology may be a viable therapeutic strategy.
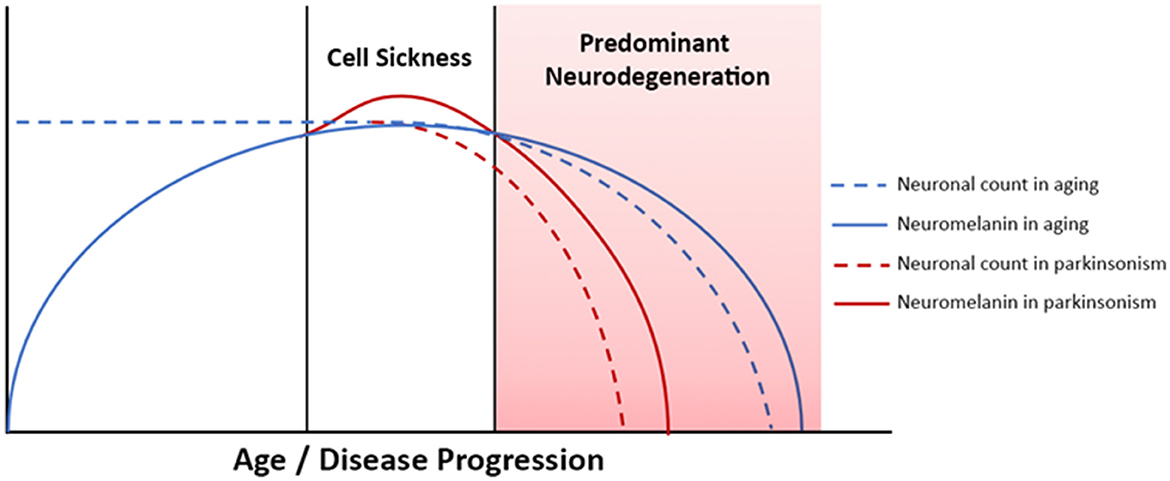
Figure 1. Neuromelanin dynamics in aging and neurodegenerative parkinsonism. Advanced PD is associated with neurodegeneration and neuromelanin loss, whereas in early PD tissue neuromelanin content and neurodegeneration are not yet coupled. Correlation between phenotypic progression and neuromelanin loss are therefore most easily observed in more severe/advanced disease.
Neuromelanin-sensitive MRI (NM-MRI) measurement of SNc and LC volumes has emerged as a highly reproducible modality to measure tissue neuromelanin in vivo and to detect disease effects (Langley et al., 2017; van der Pluijm et al., 2021). NM-MRI contrast in these structures is a result of magnetization transfer (MT) effects, and both explicit and incidental MT contrast approaches have been used (Huddleston et al., 2018). In this study an MT prepared gradient echo (MT-GRE) NM-MRI pulse sequence was used because this approach has previously been shown to exhibit improved contrast-to-noise ratio (CNR) in SNc imaging as compared to incidental MT-based NM-MRI sequences (Chen et al., 2014). Both the MT-GRE sequence and the automated image processing method for threshold-based volume measurement of LC and SNc applied here have been shown to have superior scan-rescan reproducibility compared to incidental MT-based NM-MRI and manual segmentation approaches for SNc and LC measurement (Langley et al., 2017; Tona et al., 2017; van der Pluijm et al., 2021). These methods were chosen because highly reproducible NM-MRI methods with favorable CNR are necessary to advance our understanding of neuromelanin biology and to identify candidate biomarkers for translational applications.
In this study we applied these tools to study PD with and without freezing of gait (FOG). FOG is a common and disabling feature of PD. FOG is a leading cause of falls and immobility, and it leads to social isolation and reduced quality of life (Moore et al., 2007; Rahman et al., 2008; Latt et al., 2009; Kerr et al., 2010; Hatcher-Martin et al., 2021; Kwon et al., 2023). Its prevalence increases with disease progression; at 10 years of disease duration ~60–70% of PD patients exhibit FOG (Giladi et al., 2001b; Ge et al., 2020). Thus, FOG may be considered a marker of advanced motor dysfunction in PD. Both dopaminergic and noradrenergic dysfunction are implicated in FOG (McKay et al., 2019, 2023). However, so far no studies have reported NM-MRI assessment of SNc and LC volumes in PD patients whose FOG status is characterized, nor have any studies assessed NM-MRI correlation with cognitive and motor phenotype in early vs. advanced PD stages.
Here we report our investigation of LC and SNc volumes in healthy older adults, PD without FOG (PD-NoFOG) and PD with FOG (PD-FOG) using NM-MRI. We assess for correlations between NM-MRI measurements of LC and SNc volumes and cognitive and motor aspects of PD. Specifically, we investigate relations between these widely projecting nuclei and global cognition, cognitive reserve, parkinsonian motor dysfunction, FOG, and aging in PD. The objectives of the study were to test in living humans the hypotheses implied in Figure 1: In advanced PD (shaded pink), a) a linear decline in neuromelanin is associated with neurodegeneration and the motor and cognitive phenotypes associated with neurodegeneration, and b) increasing age exacerbates neurodegeneration in LC and SNc. To address these questions in a cross-sectional study design, in one set of analyses the PD groups were stratified based on the presence vs. absence of FOG, a symptom of severe motor dysfunction. This is useful because rates of disease progression can vary widely between patients with PD, and FOG can identify clinically advanced PD independent of disease duration. Correlations were then assessed between NM-MRI derived SNc volumes and measures of motor and cognitive function in early vs. advanced PD, defined by the presence or absence of FOG. However, because disease duration may capture aspects of PD biological progression differently than FOG status, a second set of analyses were performed in early vs. advanced PD, defined by shorter (<5 years) vs. longer (≥5 years) disease duration. Finally, we assessed for correlations between age and LC volume or SNc volume in PD-FOG, PD-NoFOG, PD with <5 years duration, PD with ≥5 years duration, and older adult control individuals.
We hypothesized that linear relationships between LC and SNc integrity and motor and cognitive disease features will be most readily observed in more advanced PD, defined by the presence of the more severe motor phenotype, FOG, or based on longer disease duration, i.e., >5 years. This was expected because neurodegenerative effects on tissue neuromelanin content and NM-MRI contrast will predominate in advancing disease. This, in turn, is expected to cause the emergence of linear associations between NM-MRI and neurodegeneration-associated motor and cognitive phenotypes. Conversely, NM-MRI contrast is expected to plateau earlier in the disease course due to the competing effects of cell sickness (neuromelanin accumulation) and neurodegeneration (neuromelanin loss). As a result of these complex effects influencing NM-MRI contrast, we do not expect to observe linear relationships between NM-MRI and neurodegeneration-associated phenotypes in early disease. This hypothesis is based on the understanding of neuromelanin dynamics in aging and neurodegenerative disease reviewed above and displayed schematically in Figure 1.
Methods
Research participants
Forty-nine individuals with PD meeting the UK Brain Bank Criteria as assessed by a fellowship-trained movement disorders neurologist were recruited from the Emory Movement Disorders Clinic (Hughes et al., 1992). Nineteen older adult control participants were recruited from the Emory Alzheimer's Disease Research Center. FOG status was determined by either (1) a combination of clinical assessment by a movement disorder neurologist and Freezing of Gait Questionnaire (FOGQ) item 3 score ≥ 2, or (2) a structured clinical examination in the practically-defined OFF state and after a levodopa challenge as previously reported (Giladi et al., 2009; McKay et al., 2019). Mean dose of levodopa challenge was ~140% of the patient's typical dose. Participants in the Emory Freezing of Gait Study were assessed by a movement disorders neurologist for the presence or absence of FOG in three conditions: (1) 360 degree turns to left (twice) and right (twice), (2) timed up-and-go testing consisting of walking 10 feet, turning and returning to the starting position, and (3) the same timed up-and-go task done while performing serial calculations. Of the 49 PD participants, 19 were found to have no FOG and 30 were found to have FOG.
All study participants were clinically assessed with the UPDRS-III or the MDS-UPDRS-III as well as the Montreal Cognitive Assessment (MoCA) (Fahn et al., 1987; Goetz et al., 2008; Kasten et al., 2009). UPDRS-III scores were converted to MDS-UPDRS-III scores using the conversion approach published by Hentz et al. (2015), and MDS-UPDRS-III scores were used for analyses.
Image acquisition and processing
Each research participant underwent MRI scanning using one of two Siemens Trio 3 T scanners using a 12-channel receive only head coil at the Emory Center for Systems Imaging. NM-MRI data was acquired using a 2D GRE pulse sequence with a reduced flip angle MT preparation pulse with additional parameters as previously published (Huddleston et al., 2017). Image processing steps to determine SNc volume and LC volume were carried out using an automated, published approach, using FMRIB Software Library (FSL) and custom scripts developed in MATLAB (The Mathworks, Natick, MA) (Langley et al., 2017). Due to rare errors in field of view placement at the time of acquisition, SNc volume could not be calculated for one participant who had PD-FOG, and LC volume could not be calculated on a different individual with PD-FOG.
Statistical analysis
Differences in central tendency between study groups were assessed with ANOVA or Chi square tests as appropriate. Analyses of covariance (ANCOVA) were used to compare SNc volumes and LC volumes between groups while controlling for age, sex, years of education and scanner type. Pairwise comparisons following ANCOVA were performed using Fisher's least significant difference. Statistical analyses were performed in SPSS 26.0 (IBM) and plots were generated using GraphPad Prism 9.0.0.
Stratified analyses assessed relationships between SNc volume and motor and cognitive outcomes separately for PD patients with and without FOG as well as for all PD patients in the sample. One PD-FOG patient did not have an available SNc volume and another PD-FOG patient did not have an available LC volume value. Pearson correlation coefficients were calculated between SNc volume and MoCA, education, MDS-UPDRS-III, and FOGQ score. Considering the potential for ceiling effects with the MoCA and floor effects with the FOGQ score that could cause departures from normality, we used Spearman correlation as appropriate when the data were not normally distributed. Identified correlation coefficients were compared with partial correlation coefficients controlling for disease duration to determine whether imbalances in PD duration could impact significant findings. Additional correlation analyses were performed stratified on disease duration ≤ 5 or >5 years. One-tailed correlations were used in all correlational analyses because the direction of the predicted effect is clearly specified (lower volume in SNc or LC, consistent with neurodegeneration, is expected to correlate with worsened symptoms, i.e., higher MDS-UPDRS-III, lower MoCA, etc.).
Results
Clinical and demographic characteristics of the study groups are shown in Table 1. Healthy controls were significantly older (71 vs. 62 and 67 years) and had additional years of education (18 vs. 16 years) compared to the two PD groups (Table 1). Compared to PD-NoFOG, PD-FOG patients had significantly longer disease duration (9.6 vs. 5.8 years), higher levodopa equivalents (1,333 vs. 695 mg), and higher FOGQ scores (17.3 vs. 3.7). There were no significant differences in MDS-UPDRS-III scores between PD with and without FOG (p = 0.172).
Representative NM-MRI images of SNc of controls and PD patients with and without FOG are shown in Figure 2. Mean SNc volume varied significantly across groups (p = 0.019, ANCOVA) after controlling for age, gender, years of education, and scanner type. Mean SNc volumes are shown in Figure 3 (mean ± SE: control = 448.9 ± 30.7, PD-NoFOG = 373.5 ± 25.2, PD-FOG = 330.1 ± 21.0). Pairwise comparisons with Fisher's least significant difference found a significant difference in SNc volume between the healthy controls and PD-FOG group (p = 0.006), but not between controls and the PD-NoFOG group (p = 0.087), nor between PD-NoFOG and PD-FOG (p = 0.177). No significant differences in mean LC volume across groups were identified using similar ANCOVA models controlling for age, gender, education, and scanner type (p = 0.073; mean ± SE: control = 7.34 ± 0.92, PD-NoFOG = 5.69 ± 0.76, PD-FOG = 4.50 ± 0.63).
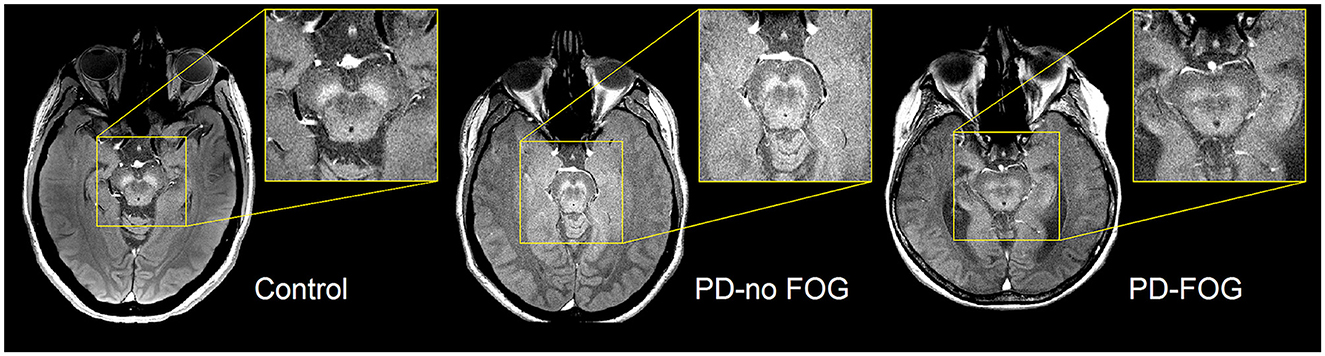
Figure 2. Representative images of NM-MRI contrast in SNc of healthy controls and PD patients with or without FOG. Hyperintense contrast in SNc of a healthy control individual acquired by NM-sensitive MRI. SNc NM-MRI signal intensity is decreased in PD-NoFOG compared to controls, and an even more profound loss of hyperintensity is seen in PD-FOG.
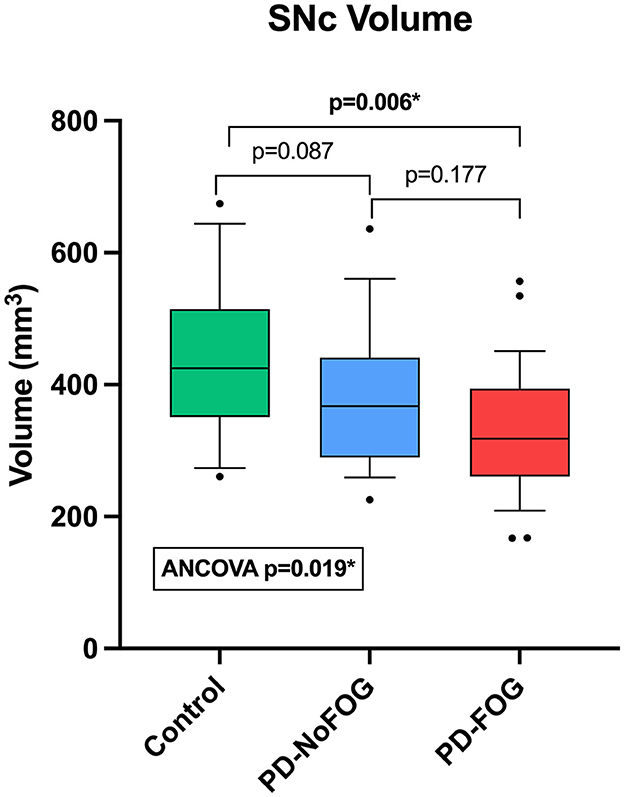
Figure 3. Comparison of SNc volumes between healthy controls, PD-NoFOG, and PD-FOG. ANCOVA including age, gender, years of education, and scanner type as covariates. Control N = 19, PD-NoFOG N = 19, PD-FOG N = 29. Boxes represent 25th−75th percentiles, whiskers show 10th−90th percentiles. Line is median. *p < 0.05.
SNc correlations with cognitive and motor measures in PD, stratifying by FOG status
In PD-FOG, there were significant positive correlations between SNc volume and MoCA score (Pearson: r = 0.506, p = 0.003; spearman: rho = 0.498, p = 0.003) and between SNc volume and education (r = 0.355, p = 0.029), as shown in Figure 4. No significant correlations between these measures were observed in PD-NoFOG. Furthermore, similar relationships between SNc volume and MoCA or education were observed when the data were analyzed using models of partial correlation accounting for potential confounding effects of age and scanner type (SNc vs. MoCA: r = 0.394, p = 0.021; SNc vs. education: r = 0.345, p = 0.039).
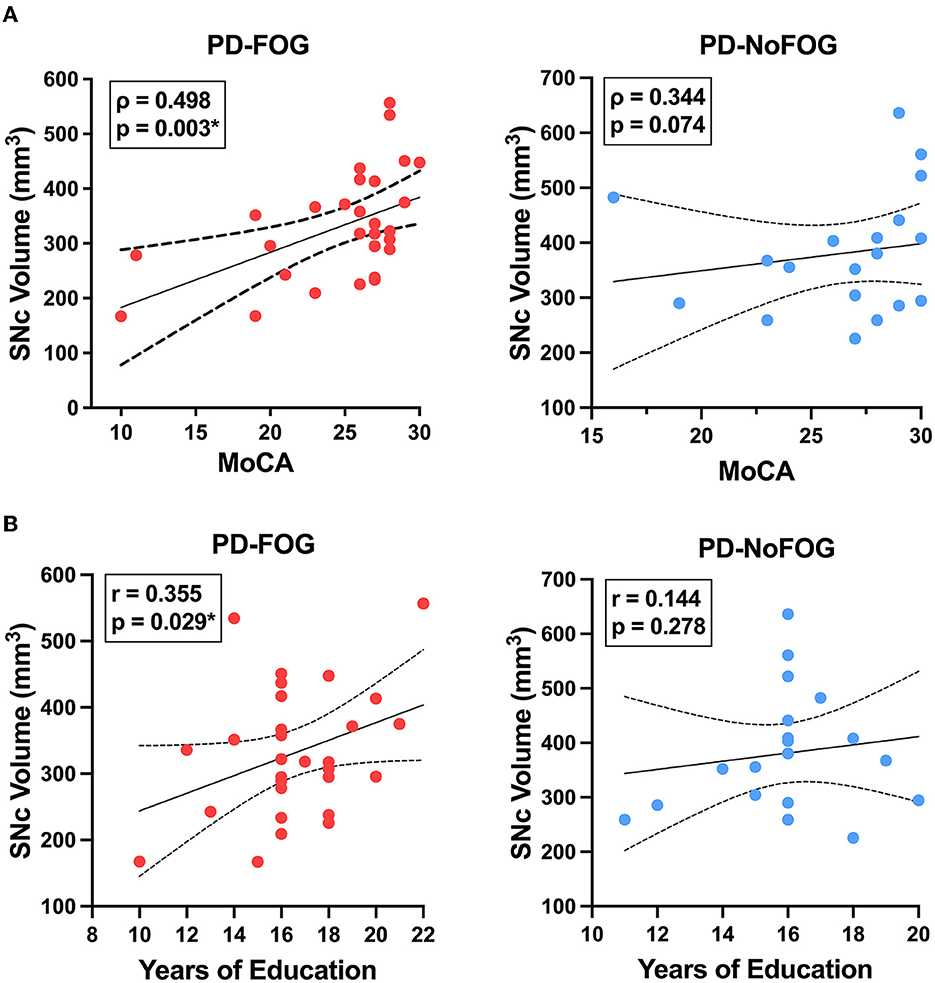
Figure 4. SNc volume vs. cognition and years of education in PD with and without FOG. SNc volume is positively correlated with MoCA (A, left) and years of education (B, left) in PD-FOG (N = 29), but no significant correlation between these measures is seen in PD-NoFOG (A, B, right; N = 19). Dotted lines represent 95% confidence intervals. *p < 0.05.
SNc volume showed significant negative correlations with ON medications MDS-UPDRS-III (r = −0.405, p = 0.015) and FOGQ scores (r = −0.318, p = 0.046) in the PD-FOG group, as shown in Figure 5. These relationships were also assessed using partial correlation controlling for age and scanner type, and the correlation between SNc volume and MDS-UPDRS-III remained significant (r = −0.328, p = 0.047), as did the correlation between SNc volume and FOGQ score (r = −0.401, p = 0.019). No significant correlations between SNc volume and ON medications MDS-UPDRS-III or FOGQ score were observed in the PD-NoFOG group. The relationship between SNc volume and FOGQ score in the PD-NoFOG group was also analyzed using Spearman's correlation and remained non-significant (ρ = −0.177, p = 0.234). Furthermore, this relationship remained non-significant following the omission from the analysis of the two apparent outliers with relatively high FOGQ scores (Figure 5B, right; ρ = −0.299, p = 0.124).
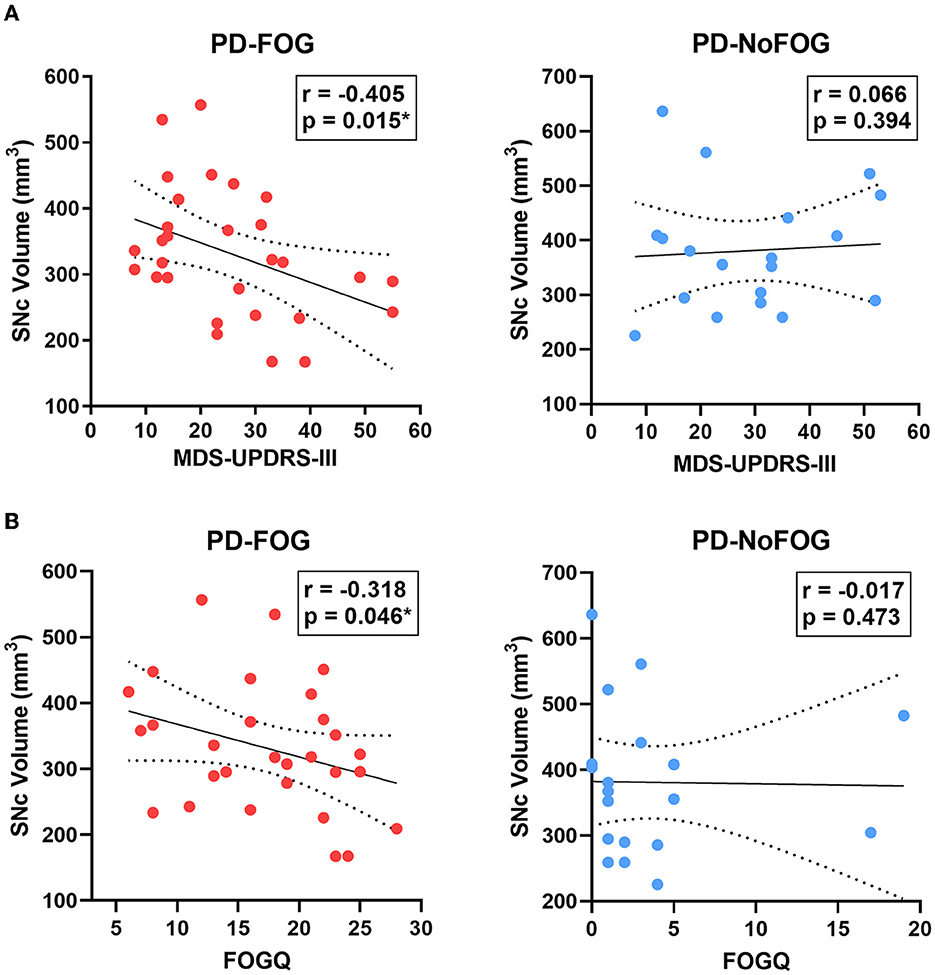
Figure 5. SNc volume vs. MDS-UPDRS-III motor score and FOGQ score in PD with and without FOG. SNc volume is negatively correlated with MDS-UPDRS-III (A, left) and FOGQ (B, left) in PD-FOG (N = 29), but no significant correlation between these measures is observed in PD-NoFOG (A, B, right; N = 19). Dotted lines represent 95% confidence intervals. *p < 0.05.
SNc correlations with cognitive and motor measures in PD, stratifying by disease duration
Among PD patients with > 5 years disease duration (N = 28), significant correlations were found between SNc volume and FOGQ score (r = −0.405, p = 0.016) and MoCA total score (r = 0.457, p = 0.007). Correlations between SNc volume and FOGQ score or MoCA remained significant when they were analyzed using partial correlation controlling for age and scanner type (p = 0.017, r = −0.410, SNc vs. FOGQ; p = 0.007, r = 0.457, SNc vs. MoCA). Among PD with ≤ 5 years disease duration (N = 19), no significant correlations were found between SNc volume and MDS-UPDRS-III score, FOGQ score, MoCA total score, years of education, or age.
SNc and LC volume correlations with age in PD
SNc volume was strongly negatively correlated with age in the PD group (r = −0.462, p = 0.0005), while no correlation was observed between SNc volume and age in the older adult control group, as shown in Figure 6A. The correlation between SNc volume and age in the PD group remained significant (r = −0.477, p < 0.001) when controlling for scanner type in partial correlation analysis. Both LC and SNc volumes were significantly negatively associated with age in PD with disease duration > 5 years as shown in Figure 6B (LC: r = −0.436, p = 0.010; SNc: r = −0.633, p = 0.0002) and these correlations remained significant when controlling for scanner type in partial correlation analysis (LC: r = −0.442, p = 0.011; SNc: r = −0.629, p < 0.001). There were no significant correlations between LC volume and age or between SNc volume and age in PD with ≤ 5 years disease duration.
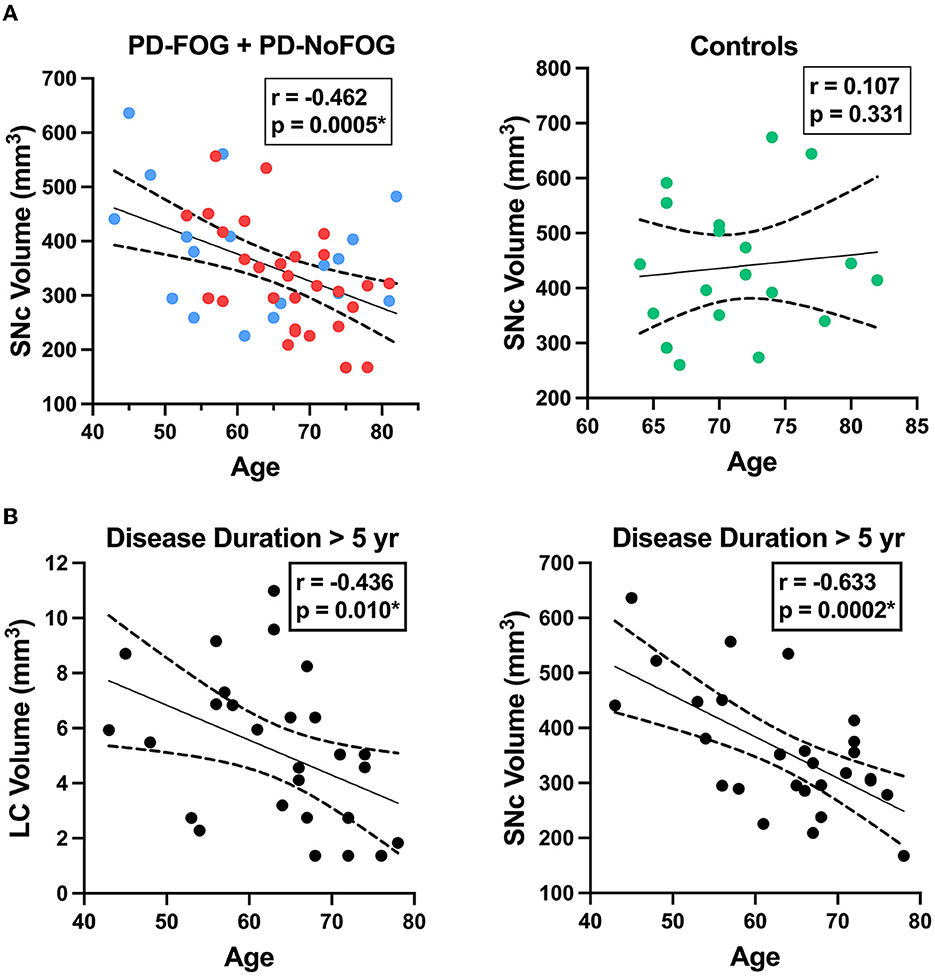
Figure 6. LC and SNc volumes vs. age in PD. (A, left) SNc volume exhibits a significant negative correlation with age in PD (N = 48, combined group with FOG shown in red (N = 29) and without FOG shown in blue (N = 19). (A, right) SNc volume is not correlated with age in older adult control participants (N = 19). (B, left) LC volume is negatively correlated with age in PD with disease duration > 5 years (N = 28). (B, right) SNc volume exhibits strong negative correlation with age in PD with disease duration > 5 years (N = 28). Dotted lines represent 95% confidence intervals. *p < 0.05.
Discussion
The baseline characteristics of the PD-NoFOG and PD-FOG groups in our study (Table 1) are consistent with other previous reports, as PD-FOG individuals were observed to have significantly higher levodopa equivalent daily dose (LEDD) than PD-NoFOG (McKay et al., 2019; Zhou et al., 2019). This was a strikingly large difference, as PD-FOG individuals were taking nearly double the LEDD as PD-NoFOG, despite the two groups having very similar MDS-UPDRS-III motor scores. This likely relates specifically to the limited levodopa response of FOG. The lack of difference in MDS-UPDRS-III score between the groups is important because this prevents overall motor severity from confounding our stratification of these groups based on FOG status. The longer disease duration observed in the PD-FOG group as compared to PD-NoFOG was also expected, because FOG is increasingly prevalent with longer disease duration in PD (Giladi et al., 2001b; Ge et al., 2020). The trend toward worse global cognition found in PD-FOG as compared to PD-NoFOG and controls was also consistent with prior observations that FOG is associated with cognitive impairment (Giladi and Hausdorff, 2006; Amboni et al., 2008; Nutt et al., 2011; Factor et al., 2014).
Comparison of NM-MRI measurements of SNc volume between groups, shown in Figure 3, revealed that PD-FOG had significantly lower SNc volume than older adult controls. Numerically, PD-NoFOG had SNc volume that was intermediate between the control group and PD-FOG, although the differences between PD-NoFOG and the other groups were not statistically significant. SNc degeneration is widely regarded as the substrate for parkinsonian motor findings generally, whereas FOG does not usually correlate with the cardinal features of parkinsonism, i.e., tremor, rigidity, and bradykinesia (Giladi et al., 2001a; Bartels et al., 2003; Dauer and Przedborski, 2003). Therefore, it is interesting and perhaps surprising that SNc volume is significantly reduced in PD-FOG when stratifying by FOG status. Reduced SNc volume in PD-FOG supports a major contribution from nigral dopaminergic system degeneration in the mechanism of FOG, and we may speculate that this relates to nigral projections other than the nigrostriatal tract alone, to account for its associations with cognitive impairment (e.g., the nigral-prefrontal projection) (Menke et al., 2010).
To interpret NM-MRI results in neurodegenerative disease states, the impacts and interactions between aging and the disease must be considered. As shown in Figure 6A, we found that in the combined PD group there is a significant negative correlation between SNc volume and age, whereas no significant correlation exists between these features in older adult controls. The presence of this relationship between age and SNc volume in the PD group, but not the control group suggests that there is an interaction between aging and PD that is strongly associated with SNc neurodegeneration. This finding remained significant when controlling for disease duration, indicating that this was not simply due to older PD patients with longer disease durations. This is a convergent finding in that it is already known that PD has increasing incidence with aging, and cellular mechanisms for this relation between PD and aging have been identified in model systems, such as increasing mitochondrial dysfunction that occurs with aging (Van Den Eeden et al., 2003; Diederich et al., 2019). However, to our knowledge, this is the first report of in vivo observation of a significant relationship between aging and SNc degeneration in PD patients. Importantly, it appears that this relationship is particularly strong in PD patients with > 5 years disease duration (Figure 6B, right). This is again consistent with the clinical observation that patients with older age of onset tend to have a more rapid decline than patients with younger onset PD (Kempster et al., 2010). This finding is also consistent with our proposed schema for neuromelanin accumulation and loss in aging and disease, shown in Figure 1. As the disease progresses, the period of predominant cell sickness (and complex neuromelanin dynamics) ends, and the period of predominant neurodegeneration ensues with progressive loss of neuromelanin. Also, in keeping with this hypothesis, we found that in PD patients with disease duration >5 years, LC volume is significantly negatively correlated with age (Figure 6B, left). This is the first in vivo report of a significant correlation between LC volume and age in PD to our knowledge, as well.
We observed that in PD-FOG there is a significant negative correlation between SNc volume and ON-medications MDS-UPDRS-III and FOG-Q score. However, in PD-NoFOG these correlations are not seen. These findings are shown in Figure 5. FOG is an advanced PD symptom that is associated with falls and cognitive impairment, which are also features of severe disease. The conflicting results in prior studies that did not find any significant correlation between SNc NM-MRI measures and MDS-UPDRS-III may have been due to the inclusion of entirely or almost entirely early to moderate PD and few, if any, PD patients with more severe motor symptoms (e.g., FOG or ON-medications MDS-UPDRS-III > 45). This is again consistent with the hypothesized model in Figure 1 in that NM-MRI contrast in early or less severe disease is expected to reflect competing effects on neuromelanin due to both cell sickness (neuromelanin increase) and neurodegeneration (neuromelanin loss) and a linear association between motor symptoms and NM-MRI contrast measures in SNc will not be observed. However, when neurodegeneration predominates in more severe disease (i.e., PD-FOG) net neuromelanin loss will also predominate, and a linear correlation between the neurodegenerative phenotype (increasing MDS-UPDRS-III score, increasing FOGQ score) and SNc volume loss can be observed. The results observed in this analysis are consistent with this interpretation. In the analysis stratifying participants based on disease duration, in the PD group with duration > 5 years, a similar association was observed between SNc volume and FOGQ score, but no significant association was seen between SNc volume and MDS-UPDRS-III in this group. This may be because disease duration alone is not a robust indicator of PD severity, given that PD progression is highly heterogeneous. Some patients will retain good levodopa response and stable clinical syndrome for much longer than others (Kempster et al., 2010; Greenland et al., 2019).
We found significant positive correlations between SNc volume and global cognition (MoCA) and cognitive reserve (years of education) in the PD-FOG group. No significant correlations between these measures were seen in the PD-NoFOG group. Although SNc neurodegeneration has long been implicated in the motor symptoms of PD, the notion that cognitive impairment in PD is due to rostral spread of synuclein pathology to cortical structures has become popular in the context of the Braak hypothesis (Braak et al., 2003). However, the role of SNc in cognition could not be well assessed prior to the advent of NM-MRI due to lack of an imaging contrast to define the ROI. The results shown in Figure 4 indicate that SNc volume is implicated in cognition in PD, and they suggest a novel association between cognitive reserve and SNc volume. Again, the identification of these correlations in the PD-FOG group and not in the PD-NoFOG group indicates that linear relationships between NM-MRI measurements of SNc volume and disease phenotype are most readily observed in the more advanced disease stages. A further implication of these results is that traditional localization of PD cognitive impairments, such as “fronto-striatal cognitive impairment” are likely incomplete and do not reflect the network organization of the nigral dopamine system, with wide-ranging projections in many brain regions that are impacted by the health of neurons with cell bodies in SNc. These results warrant further investigation and replication in a larger cohort.
Limitations and future directions
The PD-FOG and PD-NoFOG groups did not all have a complete battery of neuropsychological testing, and only MoCA was available from all participants for assessment of cognition. A larger study is needed with detailed neurocognitive testing on all subjects to confirm the association between cognition and SNc volume assessed with NM-MRI. We are currently recruiting PD-FOG and PD-NoFOG participants and acquiring a detailed neurocognitive battery for this purpose. In this ongoing study we will also perform analyses to further explore the relationship between cognition and motor dysfunction in PD-FOG vs. PD-NoFOG. We also plan recruitment of an age-matched control group for comparisons.
Assessment of the contribution of cerebrovascular disease to cognitive and motor impairment would be useful to add context for the findings shown here. Simultaneous study with both NM-MRI and pulse sequences sensitive to cerebrovascular disease burden would allow this, and we are currently collecting datasets for this purpose in a future study. No MRI dataset suitable for assessment of cerebrovascular disease burden is available for the current study, and this is a limitation. It would also be useful to investigate the associations examined in this work with further stratification of the PD-FOG group based on levodopa response. We did not have enough individuals with FOG characterized based on levodopa response to power such analyses in this study. Finally, although these results support our mechanistic hypothesis, we cannot rigorously test mechanistic hypotheses that involve cell and molecular biology concepts in human studies alone. Additional studies in model systems and radiologic-pathologic correlation studies are needed to further investigate the mechanistic role of neuromelanin in PD.
Conclusion
NM-MRI is a rapidly emerging neuroimaging modality with established capacity to detect neurodegenerative disease effects, but it remains underutilized to study PD pathogenesis and the neural substrates of PD phenomenology. The cognitive and motor correlations identified in this study enhance our understanding of the role of LC and SNc in PD, but also demonstrate the potential utility of NM-MRI in investigations of their biology in health and aging. The correlations identified between aging and SNc and LC volumes in advancing PD provide the first in vivo evidence of the interaction between aging and PD to drive neurodegeneration in melanized catecholamine nuclei in humans.
Data availability statement
The raw data supporting the conclusions of this article will be made available by the authors, without undue reservation.
Ethics statement
The studies involving human participants were reviewed and approved by Emory University Institutional Review Board. The patients/participants provided their written informed consent to participate in this study.
Author contributions
Conception: DH and SF. Study design: DH, SF, JL, and XH. Data collection and management: DH, SF, JM, KT, and RT. Data processing and analysis: DH, XC, JL, JM, and KH. Manuscript writing: DH. Manuscript editing and critique: DH, XC, KH, JL, RT, KT, JM, XH, and SF. All authors listed have made a substantial, direct, and intellectual contribution to the work and approved it for publication.
Funding
DH and XH receive funding from the Michael J. Fox Foundation for Parkinson's Research (MJFF 10854 and MJFF 010556). DH and JM were supported by the American Parkinson's Disease Association (APDA) Center for Advanced Research at Emory. JM was also supported by the McCamish Parkinson's Disease Innovation Program at Georgia Tech. SF and DH were supported by the Curtis Family and the Sartain Lanier Family Foundation. SF was also supported by NIH grant funding (U10 NS077366). DH was also supported by NIH grant funding (NIH-NINDS 1K23NS105944-01A1 and NIH-NINDS 1U19AG071754), the Emory Lewy Body Dementia Association (LBDA) Research Center of Excellence, and the McMahon Family. The Emory MRI facility used in this study was supported in part by funding from a Shared Instrumentation Grant (S10) Grant 1S10OD016413-01 to the Emory University Center for Systems Imaging Core. Recruitment of control individuals for this research was facilitated by the Emory Alzheimer's Disease Research Center (NIH-NINDS P50-AG025688).
Conflict of interest
DH is inventor on an issued patent (U.S. patent # 9600881, 3/21/17) which covers aspects of the neuromelanin-sensitive MRI methods discussed here, as well as a patent application (US-2021/0007603-A1) which covers aspects of the analysis method presented here. SF has the following disclosures: Honoraria: Lundbeck, Sunovion, Biogen, Acorda, Alterity, Takeda. Grants: Medtronics, Boston Scientific, Sun Pharmaceuticals Advanced Research Company, Biohaven, Impax, Sunovion Therapeutics, Neurocrine, Vaccinex, Voyager, Addex Pharma S.A., Prilenia Therapeutics CHDI Foundation, Michael J. Fox Foundation, Parkinson Foundation. Royalties: Demos, Blackwell Futura, Springer for textbooks, Uptodate Other Signant Health (Bracket Global LLC). JM performs technical consulting for Biocircuit Technologies. RT has following disclosures: Honoraria: UpToDate.
The remaining authors declare that the research was conducted in the absence of any commercial or financial relationships that could be construed as a potential conflict of interest.
Publisher's note
All claims expressed in this article are solely those of the authors and do not necessarily represent those of their affiliated organizations, or those of the publisher, the editors and the reviewers. Any product that may be evaluated in this article, or claim that may be made by its manufacturer, is not guaranteed or endorsed by the publisher.
References
Amboni, M., Cozzolino, A., Longo, K., Picillo, M., and Barone, P. (2008). Freezing of gait and executive functions in patients with Parkinson's disease. Mov. Disord. 23, 395–400. doi: 10.1002/mds.21850
Bartels, A. L., Balash, Y., Gurevich, T., Schaafsma, J. D., Hausdorff, J. M., Giladi, N., et al. (2003). Relationship between freezing of gait (FOG) and other features of Parkinson's: FOG is not correlated with bradykinesia. J. Clin. Neurosci. 10, 584–588. doi: 10.1016/S0967-5868(03)00192-9
Beach, T. G., Sue, L. I., Walker, D. G., Lue, L. F., Connor, D. J., Caviness, J. N., et al. (2007). Marked microglial reaction in normal aging human substantia nigra: correlation with extraneuronal neuromelanin pigment deposits. Acta Neuropathol. 114, 419–424. doi: 10.1007/s00401-007-0250-5
Benarroch, E. (2023). What are current concepts on the functional organization of the locus coeruleus and its role in cognition and neurodegeneration? Neurology 100, 132–137. doi: 10.1212/WNL.0000000000206736
Bogerts, B. (1981). A brainstem atlas of catecholaminergic neurons in man, using melanin as a natural marker. J. Comp. Neurol. 197, 63–80. doi: 10.1002/cne.901970106
Braak, H., Tredici, K. D., Rüb, U., de Vos, R. A. I., Jansen Steur, E. N. H., Braak, E., et al. (2003). Staging of brain pathology related to sporadic Parkinson's disease. Neurobiol. Aging 24, 197–211. doi: 10.1016/S0197-4580(02)00065-9
Carballo-Carbajal, I., Laguna, A., Romero-Giménez, J., Cuadros, T., Bové, J., Martinez-Vicente, M., et al. (2019). Brain tyrosinase overexpression implicates age-dependent neuromelanin production in Parkinson's disease pathogenesis. Nat. Commun. 10, 973. doi: 10.1038/s41467-019-08858-y
Chen, X., Huddleston, D. E., Langley, J., Ahn, S., Barnum, C. J., Factor, S. A., et al. (2014). Simultaneous imaging of locus coeruleus and substantia nigra with a quantitative neuromelanin MRI approach. Mag. Reson. Imag. 32, 1301–1306. doi: 10.1016/j.mri.2014.07.003
Damier, P., Hirsch, E. C., Agid, Y., and Graybiel, A. M. (1999). The substantia nigra of the human brain. II. Patterns of loss of dopamine-containing neurons in Parkinson's disease. Brain 122, 1437–1448. doi: 10.1093/brain/122.8.1437
Dauer, W., and Przedborski, S. (2003). Parkinson's disease: mechanisms and models. Neuron 39, 889–909. doi: 10.1016/S0896-6273(03)00568-3
Diederich, N. J., James Surmeier, D., Uchihara, T., Grillner, S., and Goetz, C. G. (2019). Parkinson's disease: is it a consequence of human brain evolution? Mov. Disord. 34, 453–459. doi: 10.1002/mds.27628
Factor, S. A., Scullin, M. K., Sollinger, A. B., Land, J. O., Wood-Siverio, C., Zanders, L., et al. (2014). Freezing of gait subtypes have different cognitive correlates in Parkinson's disease. Parkinson. Relat. Disord. 20, 1359–1364. doi: 10.1016/j.parkreldis.2014.09.023
Fahn, S., Elton, R., and Members, U. P. (1987). “Unified Parkinson's disease rating scale,” in Recent Developments in Parkinson's Disease, eds S. Fahn, C. Marsden, M. Goldstein, D. Calne, eds. (Florham Park, NJ: Macmillan Healthcare Information), 153–163.
Fearnley, J. M., and Lees, A. J. (1991). Ageing and Parkinson's disease: substantia nigra regional selectivity. Brain 114, 2283–2301. doi: 10.1093/brain/114.5.2283
Ge, H.-L., Chen, X.-Y., Lin, Y.-X., Ge, T.-J., Yu, L.-H., Lin, Z.-Y., et al. (2020). The prevalence of freezing of gait in Parkinson's disease and in patients with different disease durations and severities. Chin. Neurosurg. J. 6, 17. doi: 10.1186/s41016-020-00197-y
Giladi, N., and Hausdorff, J. M. (2006). The role of mental function in the pathogenesis of freezing of gait in Parkinson's disease. J. Neurol. Sci. 248, 173–176. doi: 10.1016/j.jns.2006.05.015
Giladi, N., McDermott, M. P., Fahn, S., Przedborski, S., Jankovic, J., Stern, M., et al. (2001a). Freezing of gait in PD: prospective assessment in the DATATOP cohort. Neurology 56, 1712–1721. doi: 10.1212/WNL.56.12.1712
Giladi, N., Tal, J., Azulay, T., Rascol, O., Brooks, D. J., Melamed, E., et al. (2009). Validation of the freezing of gait questionnaire in patients with Parkinson's disease. Movem. Disord. 24, 655–661. doi: 10.1002/mds.21745
Giladi, N., Treves, T. A., Simon, E. S., Shabtai, H., Orlov, Y., Kandinov, B., et al. (2001b). Freezing of gait in patients with advanced Parkinson's disease. J. Neural. Transm. 108, 53–61. doi: 10.1007/s007020170096
Goetz, C. G., Tilley, B. C., Shaftman, S. R., Stebbins, G. T., Fahn, S., Martinez-Martin, P., et al. (2008). Movement disorder society-sponsored revision of the unified Parkinson's disease rating scale (MDS-UPDRS): scale presentation and clinimetric testing results. Mov. Disord. 23, 2129–2170. doi: 10.1002/mds.22340
Gonzalez-Sepulveda, M., Compte, J., Cuadros, T., Nicolau, A., Guillard-Sirieix, C., Peñuelas, N., et al. (2023). In vivo reduction of age-dependent neuromelanin accumulation mitigates features of Parkinson's disease. Brain 146, 1040–1052. doi: 10.1093/brain/awac445
Greenland, J. C., Williams-Gray, C. H., and Barker, R. A. (2019). The clinical heterogeneity of Parkinson's disease and its therapeutic implications. Eur. J. Neurosci. 49, 328–338. doi: 10.1111/ejn.14094
Hatcher-Martin, J. M., McKay, J. L., Pybus, A. F., Sommerfeld, B., Howell, J. C., Goldstein, F. C., et al. (2021). Cerebrospinal fluid biomarkers in Parkinson's disease with freezing of gait: an exploratory analysis. NPJ Parkinsons. Dis. 7, 105. doi: 10.1038/s41531-021-00247-x
Hentz, J. G., Mehta, S. H., Shill, H. A., Driver-Dunckley, E., Beach, T. G., Adler, C. H., et al. (2015). Simplified conversion method for unified Parkinson's disease rating scale motor examinations. Mov. Disord. 30, 1967–1970. doi: 10.1002/mds.26435
Huddleston, D., Langley, J., Dusek, P., He, N., Faraco, C. C., Crosson, B., et al. (2018). Imaging Parkinsonian pathology in substantia Nigra with MRI. Curr. Radiol. Rep. 6, 1–13. doi: 10.1007/s40134-018-0272-x
Huddleston, D. E., Langley, J., Sedlacik, J., Boelmans, K., Factor, S. A., Hu, X. P., et al. (2017). In vivo detection of lateral-ventral tier nigral degeneration in Parkinson's disease. Hum. Brain Mapp. 38, 2627–2634. doi: 10.1002/hbm.23547
Hughes, A., Daniel, S., Kilford, L., and Lees, A. (1992). Accuracy of clinical diagnosis of idiopathic Parkinson's disease: a clinico-pathological study of 100 cases. J. Neurol. Neurosurg. Psychiatry 55, 181–184. doi: 10.1136/jnnp.55.3.181
Kasten, M., Bruggemann, N., Schmidt, A., and Klein, C. (2009). Validity of the MoCA and MMSE in the detection of MCI and dementia in Parkinson disease. Neurology 73, 1738–1745. doi: 10.1212/WNL.0b013e3181c34b47
Kempster, P. A., O'Sullivan, S. S., Holton, J. L., Revesz, T., and Lees, A. J. (2010). Relationships between age and late progression of Parkinson's disease: a clinico-pathological study. Brain 133, 1755–1762. doi: 10.1093/brain/awq059
Kerr, G. K., Worringham, C. J., Cole, M. H., Lacherez, P. F., Wood, J. M., Silburn, P. A., et al. (2010). Predictors of future falls in Parkinson disease. Neurology 75, 116–124. doi: 10.1212/WNL.0b013e3181e7b688
Kwon, H., Clifford, G. D., Genias, I., Bernhard, D., Esper, C. D., Factor, S. A., et al. (2023). An explainable spatial-temporal graphical convolutional network to score freezing of gait in Parkinsonian patients. Sensors 23, 1766. doi: 10.3390/s23041766
Langley, J., Huddleston, D. E., Liu, C. J., and Hu, X. (2017). Reproducibility of locus coeruleus and substantia nigra imaging with neuromelanin sensitive MRI. MAGMA 30, 121–125. doi: 10.1007/s10334-016-0590-z
Latt, M. D., Lord, S. R., Morris, J. G., and Fung, V. S. (2009). Clinical and physiological assessments for elucidating falls risk in Parkinson's disease. Mov. Disord. 24, 1280–1289. doi: 10.1002/mds.22561
Manaye, K. F., McIntire, D. D., Mann, D. M., and German, D. C. (1995). Locus coeruleus cell loss in the aging human brain: a non-random process. J. Comp. Neurol. 358, 79–87. doi: 10.1002/cne.903580105
Mann, D. M., and Yates, P. O. (1974). Lipoprotein pigments–their relationship to ageing in the human nervous system. II. The melanin content of pigmented nerve cells. Brain 97, 489–498. doi: 10.1093/brain/97.1.489
McKay, J. L., Goldstein, F. C., Sommerfeld, B., Bernhard, D., Perez Parra, S., Factor, S. A., et al. (2019). Freezing of Gait can persist after an acute levodopa challenge in Parkinson's disease. NPJ Parkinsons Dis. 5, 25. doi: 10.1038/s41531-019-0099-z
McKay, J. L., Nye, J., Goldstein, F. C., Sommerfeld, B., Smith, Y., Weinshenker, D., et al. (2023). Levodopa responsive freezing of gait is associated with reduced norepinephrine transporter binding in Parkinson's disease. Neurobiol. Dis. 179, 106048. doi: 10.1016/j.nbd.2023.106048
Menke, R. A., Jbabdi, S., Miller, K. L., Matthews, P. M., and Zarei, M. (2010). Connectivity-based segmentation of the substantia nigra in human and its implications in Parkinson's disease. Neuroimage 52, 1175–1180. doi: 10.1016/j.neuroimage.2010.05.086
Moore, O., Peretz, C., and Giladi, N. (2007). Freezing of gait affects quality of life of peoples with Parkinson's disease beyond its relationships with mobility and gait. Mov. Disord. 22, 2192–2195. doi: 10.1002/mds.21659
Nutt, J. G., Bloem, B. R., Giladi, N., Hallett, M., Horak, F. B., Nieuwboer, A., et al. (2011). Freezing of gait: moving forward on a mysterious clinical phenomenon. Lancet Neurol. 10, 734–744. doi: 10.1016/S1474-4422(11)70143-0
Orr, C. F., Rowe, D. B., Mizuno, Y., Mori, H., and Halliday, G. M. A. (2005). possible role for humoral immunity in the pathogenesis of Parkinson's disease. Brain 128, 2665–2674. doi: 10.1093/brain/awh625
Rahman, S., Griffin, H. J., Quinn, N. P., and Jahanshahi, M. (2008). Quality of life in Parkinson's disease: the relative importance of the symptoms. Mov. Disord. 23, 1428–1434. doi: 10.1002/mds.21667
Shibata, E., Sasaki, M., Tohyama, K., Kanbara, Y., Otsuka, K., Ehara, S., et al. (2006). Age-related changes in locus ceruleus on neuromelanin magnetic resonance imaging at 3 Tesla. Magn. Reson. Med. Sci. 5, 197–200. doi: 10.2463/mrms.5.197
Sulzer, D., Bogulavsky, J., Larsen, K. E., Behr, G., Karatekin, E., Kleinman, M. H., et al. (2000). Neuromelanin biosynthesis is driven by excess cytosolic catecholamines not accumulated by synaptic vesicles. Proc. Natl. Acad. Sci. U. S. A. 97, 11869–11874. doi: 10.1073/pnas.97.22.11869
Sulzer, D., Mosharov, E., Talloczy, Z., Zucca, F. A., Simon, J. D., Zecca, L., et al. (2008). Neuronal pigmented autophagic vacuoles: lipofuscin, neuromelanin, and ceroid as macroautophagic responses during aging and disease. J. Neurochem. 106, 24–36. doi: 10.1111/j.1471-4159.2008.05385.x
Tona, K.-D., Keuken, M. C., de Rover, M., Lakke, E., Forstmann, B. U., Nieuwenhuis, S., et al. (2017). In vivo visualization of the locus coeruleus in humans: quantifying the test-retest reliability. Brain Struct. Funct. 222, 4203–4217. doi: 10.1007/s00429-017-1464-5
Van Den Eeden, S. K., Tanner, C. M., Bernstein, A. L., Fross, R. D., Leimpeter, A., Bloch, D. A., et al. (2003). Incidence of Parkinson's disease: variation by age, gender, and race/ethnicity. Am. J. Epidemiol. 157, 1015–1022. doi: 10.1093/aje/kwg068
van der Pluijm, M., Cassidy, C., Zandstra, M., Wallert, E., de Bruin, K., Booij, J., et al. (2021). Reliability and reproducibility of neuromelanin-sensitive imaging of the substantia nigra: a comparison of three different sequences. J. Magn. Reson. Imag. 53, 712–721. doi: 10.1002/jmri.27384
Viceconte, N., Burguillos, M. A., Herrera, A. J., De Pablos, R. M., Joseph, B., Venero, J. L., et al. (2015). Neuromelanin activates proinflammatory microglia through a caspase-8-dependent mechanism. J. Neuroinflamm. 12, 5. doi: 10.1186/s12974-014-0228-x
Vila, M. (2019). Neuromelanin, aging, and neuronal vulnerability in Parkinson's disease. Mov. Disord. 34, 1440–1451. doi: 10.1002/mds.27776
Vila, M., Laguna, A., and Carballo-Carbajal, I. (2019). Intracellular crowding by age-dependent neuromelanin accumulation disrupts neuronal proteostasis and triggers Parkinson disease pathology. Autophagy 15, 2028–2030. doi: 10.1080/15548627.2019.1659621
Xing, Y., Sapuan, A., Dineen, R. A., and Auer, D. P. (2018). Life span pigmentation changes of the substantia nigra detected by neuromelanin-sensitive MRI. Mov. Disord. 33, 1792–1799. doi: 10.1002/mds.27502
Zarow, C., Lyness, S. A., Mortimer, J. A., and Chui, H. C. (2003). Neuronal loss is greater in the locus coeruleus than nucleus basalis and substantia Nigra in Alzheimer and Parkinson diseases. Arch. Neurol. 60, 337–341. doi: 10.1001/archneur.60.3.337
Zecca, L., Bellei, C., Costi, P., Albertini, A., Monzani, E., Casella, L., et al. (2008). New melanic pigments in the human brain that accumulate in aging and block environmental toxic metals. Proc. Natl. Acad. Sci. U. S. A. 105, 17567–17572. doi: 10.1073/pnas.0808768105
Zecca, L., Fariello, R., Riederer, P., Sulzer, D., Gatti, A., Tampellini, D., et al. (2002). The absolute concentration of nigral neuromelanin, assayed by a new sensitive method, increases throughout the life and is dramatically decreased in Parkinson's disease. FEBS Lett. 510, 216–220. doi: 10.1016/S0014-5793(01)03269-0
Zecca, L., Tampellini, D., Gerlach, M., Riederer, P., Fariello, R. G., Sulzer, D., et al. (2001). Substantia nigra neuromelanin: structure, synthesis, and molecular behaviour. Clin. Mol. Pathol. 54, 414–418.
Zecca, L., Zucca, F. A., Albertini, A., Rizzio, E., and Fariello, R. G. (2006). A proposed dual role of neuromelanin in the pathogenesis of Parkinson's disease. Neurology 67, S8–11. doi: 10.1212/WNL.67.7_suppl_2.S8
Zecca, L., Zucca, F. A., Wilms, H., and Sulzer, D. (2003). Neuromelanin of the substantia nigra: a neuronal black hole with protective and toxic characteristics. Trends Neurosci. 26, 578–580. doi: 10.1016/j.tins.2003.08.009
Zhou, Y., Zhao, J., Hou, Y., Su, Y., Chan, P., Wang, Y., et al. (2019). Dopaminergic pathway and primary visual cortex are involved in the freezing of gait in Parkinson's disease: a PET-CT study. Neuropsychiatr. Dis. Treat. 15, 1905–1914. doi: 10.2147/NDT.S197879
Keywords: neuromelanin (NM), substantia nigra (SN), freezing of gait (FOG), Parkinson's disease (PD), cognition, cognitive reserve, aging, neuromelanin-sensitive MRI (NM-MRI)
Citation: Huddleston DE, Chen X, Hwang K, Langley J, Tripathi R, Tucker K, McKay JL, Hu X and Factor SA (2023) Neuromelanin-sensitive MRI correlates of cognitive and motor function in Parkinson's disease with freezing of gait. Front. Dement. 2:1215505. doi: 10.3389/frdem.2023.1215505
Received: 02 May 2023; Accepted: 13 July 2023;
Published: 11 August 2023.
Edited by:
Charbel Moussa, Georgetown University, United StatesReviewed by:
Stanislaw Szlufik, Medical University of Warsaw, PolandKarin Mente, Case Western Reserve University, United States
Copyright © 2023 Huddleston, Chen, Hwang, Langley, Tripathi, Tucker, McKay, Hu and Factor. This is an open-access article distributed under the terms of the Creative Commons Attribution License (CC BY). The use, distribution or reproduction in other forums is permitted, provided the original author(s) and the copyright owner(s) are credited and that the original publication in this journal is cited, in accordance with accepted academic practice. No use, distribution or reproduction is permitted which does not comply with these terms.
*Correspondence: Daniel E. Huddleston, ZGFuaWVsLmh1ZGRsZXN0b24mI3gwMDA0MDtlbW9yeS5lZHU=
†ORCID: Daniel E. Huddleston orcid.org/0000-0003-0503-3736