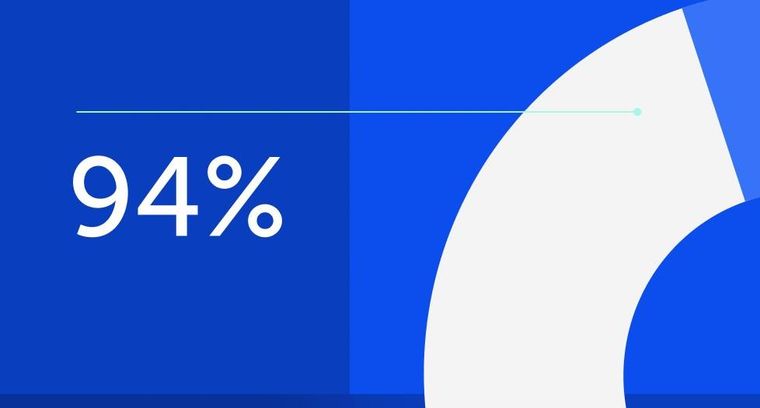
94% of researchers rate our articles as excellent or good
Learn more about the work of our research integrity team to safeguard the quality of each article we publish.
Find out more
ORIGINAL RESEARCH article
Front. Conserv. Sci., 21 March 2025
Sec. Plant Conservation
Volume 6 - 2025 | https://doi.org/10.3389/fcosc.2025.1552578
The Mediterranean Basin is known as a global biodiversity hotspot, featuring an impressive variety of plant species, with the Maltese Archipelago playing a significant role. This study assesses plant diversity within Malta’s rocky cliffs, focusing on changes over five decades (1973 and 2024). Using Hill numbers alongside coverage-based and size-based rarefaction and extrapolation methods, the research evaluates species richness, Simpson diversity, and Shannon diversity. Results indicate overall stability in species richness but reveal declining evenness and increasing dominance by a few species, suggesting significant ecological shifts. Statistical analyses, including NMDS, ANOSIM, and PERMANOVA, validate temporal changes in community composition. Notably, some endemic species, such as Salsola melitensis, show slight increases, whereas others, like Chiliadenus bocconei, exhibit severe declines. These findings underscore the importance of rocky habitats as reservoirs of endemic and specialized flora, highlighting the urgent need for conservation efforts to mitigate anthropogenic pressures and adapt to changing environmental conditions. This study demonstrates the effectiveness of Hill numbers and advanced diversity metrics in monitoring biodiversity dynamics and informing conservation strategies.
Research on plant diversity plays a fundamental role in understanding biodiversity, especially in the Mediterranean region. This area is internationally acknowledged as a biodiversity hotspot and an essential center of plant species richness and endemism (Medail and Quezel, 1997). The Mediterranean Basin is recognized as one of the 25 global biodiversity hotspots due to its remarkable richness in plant species, featuring approximately 25,000 native species, more than half of which are endemic (Myers et al., 2000). This exceptional diversity results from a unique combination of geography, history, and climate, which has allowed plants to evolve and adapt to the region’s various environments continually. However, this valuable biodiversity faces serious threats. The region is home to 570 million people who experience significant economic disparities (Kiss et al. 2020). As economic development grows, it places increasing pressure on the environment, creating a complex challenge for conservation efforts. The main threats to the Mediterranean flora include human disturbances, mass tourism development, and ongoing climate change (Fischer and Schär, 2010; Gouveia et al., 2017). Mediterranean islands represent a small portion of the land but are home to a remarkable diversity of biological life subject to several changes over time (Sciandrello et al., 2021). Within the Mediterranean hotspot, islands significantly contribute to plant diversity, providing an exceptional research opportunity for botanists (Brullo et al., 2023; Cambria et al., 2023; Minissale et al., 2023).
The Maltese Archipelago, situated in the central Mediterranean, is notable for its distinctive plant life (Lanfranco, 1995). The main islands, Malta, Gozo, and Comino, are home to an assemblage of c. 1000 native plant species, including several endemics such as Cheirolophus crassifolius (Bertol.) Susanna, Atriplex lanfrancoi (Brullo & Pavone) G. Kadereit & Sukhor, and Helichrysum melitense (Pignatti) Brullo, Lanfranco, Pavone & Ronsisvalle (Brullo et al., 2020). The flora of the Maltese Islands represents a fascinating blend of Mediterranean, North African, and Middle Eastern elements, shaped by the archipelago’s strategic location and unique climatic conditions. In the Maltese Islands, chasmophilous phytocoenoses thrive in the cracks and crevices of limestone rock faces, especially on the sheer coastal sea-facing cliffs and within the valleys known as ‘widien’, analogous to the North African ‘wadis’. These habitats host numerous endemic species, many with ancient evolutionary origins, and are taxon-guide of chasmophytic vegetation, adapted to these extreme and often inaccessible environments. Much of the intact biodiversity has been relegated to marginal places, such as cliffs (Brullo et al., 2020). Therefore, it is critical to inventory and understand them.
Currently, there are gaps in knowledge regarding the flora in the Maltese Islands and its conservation status (Tavilla et al., 2023). The first official National Red Data Book, which details the flora of the region, was published by Schembri and Sultana (1989). Since then, there have been no substantial updates, only narrow and unique contributions (Mifsud, 2013; Camilleri et al., 2024a), representing a significant knowledge gap.
This study aims to carry out a first plant diversity assessment using statistical analyses. Assessing biodiversity is crucial for ecological study and conservation, but frequently, the instruments and indices used to measure diversity have drawbacks, particularly when contrasting communities with widely differing species richness and composition. Despite their intuitiveness, traditional metrics like species richness and Shannon entropy have severe disadvantages in real-world applications. Species richness, for instance, is highly sensitive to sample size and is limited in its ability to account for the distribution of species abundances within a community. This sensitivity often results in biased comparisons, especially when assemblages of different ecological complexities, such as temperate and tropical forests, are compared using similar sampling efforts (Colwell et al., 2012; Chao et al., 2014). To address these limitations, Hill numbers have been increasingly promoted as an integrated approach for quantifying species diversity (Chao et al., 2014). By considering species richness and relative abundances and adjusting the sensitivity to common versus uncommon species, Hill numbers, also known as the effective number of species, provide a consistent framework for assessing biodiversity. Strong comparisons across various sample sizes are made possible by the mathematical characteristics of Hill numbers, which enable smooth rarefaction (interpolation) and extrapolation (prediction) of diversity data. For ecological surveys, which frequently encounter logistical challenges in data collecting, these characteristics lessen the inherent biases connected to under-sampling or unequal sample attempts (Colwell et al., 2012; Chao and Jost, 2012). Hill numbers also have the benefit of being able to measure sample completeness using coverage-based methods as opposed to only sample size (Chao and Jost, 2012). Fairer comparisons result from coverage-based rarefaction, which makes sure that samples of various assemblages are normalized by their completeness. This is particularly useful for communities with varying distributions of species abundance, where an equal sampling effort may not capture the full diversity of species. Coverage-based rarefaction also minimizes the risk of discarding data, which is common in traditional rarefaction methods where larger samples are often artificially reduced to match smaller samples (Chao and Jost, 2012). Furthermore, Hill numbers adhere to the replication principle, meaning that diversity effectively doubles when two identical communities are combined. This principle makes Hill numbers intuitive and mathematically consistent, unlike many other indices that fail to capture this property (Chao et al., 2013, 2014, 2020). In addition, Hill numbers allow for the construction of diversity profiles that plot diversity as a continuous function of parameter q, representing different diversity orders (Chao and Jost, 2015). Such profiles enable a comprehensive visual and statistical comparison of the compositional complexities of multiple assemblages, providing insights into both species’ richness and diversity. This capability to produce unified diversity profiles makes Hill numbers a versatile tool for ecological studies, especially for assessing changes in species variety of ecological communities over time or comparing habitats with varying levels of environmental stability (Hsieh et al., 2016; Maturo and Di Battista, 2018). Integrating rarefaction and extrapolation through Hill numbers, supported by advanced tools such as the iNEXT R package, provides an effective means to accurately and meaningfully assess biodiversity (Hsieh et al., 2016). It ensures that the assemblage differences are identified by accounting for sampling effort and species detectability. This approach enhances the robustness and interpretability of ecological studies, supporting conservation management and deepening our understanding of the processes that drive species diversity in different habitats.
Hence, the primary aim of this study is to compare changes over time and, for the first time, assess the floristic diversity of Malta’s cliffs. The goal of this study is to increase our understanding of plant diversity in cliff vegetation, especially considering conservation efforts and environmental change. Our objective is to offer a more precise evaluation of floristic diversity and pinpoint the changes and impacts of this habitat type by utilizing Hill numbers and coverage-based and size-based techniques.
Malta, located in the central Mediterranean Sea at approximately 35°53′N latitude and 14°30′E longitude, is the largest island in the Maltese archipelago comprising three main islands: Malta, Gozo, and Comino (Figure 1). The islands are predominantly composed of sedimentary limestone formations resulting from marine deposition during the Oligocene and Miocene epochs. This geological composition has created a rugged topography characterized by cliffs, karst landscapes, and limited soil depth (Schembri, 2019). The landscape of the island of Malta, shaped by the tectonics of the central Mediterranean, particularly the Pantelleria Rift System, is tilted from southwest to northeast. Cliffs of Coralline Limestone characterize the southwestern coast, while the northeastern coast features gently sloping terrain. Malta’s coastal cliffs comprise various geomorphological features, ranging from Upper Coralline Limestone plateau to Blue Clay slopes overlaying older layers of Globigerina Limestone and Lower Coralline Limestone, as seen at Dingli Cliffs. The erosion of Blue Clay leads to the formation of boulder screes, locally known as “rdum,” when blocks of the overlying Upper Coralline Limestone collapse and fragment. The cliffs also incorporate the mouths of dry valleys (widien) that open into the sea and are flanked by garrigue communities and agricultural areas. Some regions feature perennial or near-perennial springs due to seepage from a perched aquifer at the Blue Clay and Upper Coralline Limestone junction, as observed in Baħrija and Għajn it-Tajba (ERA-Environment and Resources Authority, 2019). The climate of Malta is classified as Mediterranean, featuring hot, dry summers and mild, wet winters. Average annual temperatures range from 10°C to 37°C, with precipitation predominantly occurring between October and March (Galdies, 2011). With 519,562 inhabitants in 2021, the Maltese Islands have one of the highest population densities in the EU, with around 1,715 people per square kilometer (National Statistics Office, 2023). Malta’s coastal cliffs and rocky outcrops host unique rupicolous habitats, supporting specialized flora and fauna adapted to these environments. Several of these habitats are protected under the European Union’s Natura 2000 network, which aims to ensure the long-term survival of Europe’s most valuable and threatened species and habitats (Directive, 1992). Notably, over 13.8% of Malta’s terrestrial area, encompassing approximately 43.6 square kilometers, is designated as Natura 2000 sites (ERA - Environment and Resources Authority, 2024). The surveyed sites fall within the Special Area of Conservation named “Rdumijiet ta’ Malta: Ir-Ramla ta·-Ċirkewwa sal-Ponta ta’ Bengħisa” located in the south southeastern and southern side of Malta (code: MT0000024).
The field data collected by Brullo et al. (2020: Table 6.1) and the unpublished data gathered in our study, were obtained in both cases using a phytosociological approach. This method, also known as the Braun–Blanquet approach (Braun-Blanquet, 1964), takes into account the relationships between plant communities and their environment, as well as the interactions within communities. Phytosociological relevés include vegetation plots, vegetation cover, and species occurrence. Following the Braun–Blanquet scale, each species was assigned a cover value ranging from 1 (1–5% coverage) to 5 (75–100% coverage), while sporadic species (<1% coverage) were marked with the “+” symbol. The phytosociological relevés collected in 1973 were carried out in late June, whereas those conducted in 2024 took place in late May. These periods are comparable, as the flowering range of the physiognomic species of these environments falls within this seasonal window. The median plot size used for vegetation surveys by Brullo et al. (2020) was 50 square meters, whereas in this study, unpublished surveys were conducted using 20 square meter plots. This smaller plot size was chosen as it is more suitable for investigating this specific type of vegetation (Chytrý and Otýpková, 2003).
The biological forms follow the classification of Raunkiaer (1934) and Pignatti et al. (2017-2019), the latter being used for species shared between the Italian and Maltese flora. The nomenclature of taxa is in accordance with the Portal to the Flora of Italy (2024) and POWO (2024).
The dataset (Supplementary Table S1) comprised presence-absence data from Brullo et al. (2020), collected during 1973, and unpublished field data collected by the authors in 2024. Subsequently, both phytosociological tables were converted into presence-absence values (0 and 1). To assess species diversity, we utilized the iNEXT online tool (Chao et al., 2016), which mirrors the functionalities of the R package iNEXT (Hsieh et al., 2016). The iNEXT tool offers a strong foundation for assessing species diversity across different sample sizes and coverage levels by making it easier to compute rarefaction and extrapolation curves based on Hill numbers. Based on sampling-unit-based incidence data, we produced rarefaction and extrapolation curves for species diversity, both sample-size-based and coverage-based. Interpolation and extrapolation sampling curves of Hill numbers were calculated for the three most widely used orders: species richness (q=0), Shannon diversity (q=1), and Simpson diversity (q=2). Rarefaction was utilized to standardize sample sizes by systematically reducing the number of individuals, allowing for equitable comparisons of species richness across samples of varying sizes. Extrapolation extended the data beyond the original sample size to estimate potential species diversity with increased sampling effort. We applied 200 bootstrap replicates and set the confidence interval (CI) at 95% for these analyses. It was set as a default endpoint and number of knots, doubling the sample size for each reference. The methods for sample-size-based rarefaction and extrapolation for species richness were developed by Colwell et al. (2012), while the corresponding coverage-based approaches were formulated by Chao and Jost (2012). Chao et al. (2014) extended these methods to encompass Hill numbers of varying orders. To evaluate species diversity in more “natural” conditions, i.e., without disturbing conditions, the analyses were conducted again using a refined dataset labeled “clean”. This dataset included only the typical species of Maltese rocky environments, selected in accordance with Biondi et al. (2024) and based on the expert knowledge of the authors regarding characteristic species of these habitats. This method made it possible to compare species diversity in 1973 and 2024 with a specific emphasis on highlighting changes in local flora. Non-metric Multidimensional Scaling (NMDS) ordination was performed using the Jaccard distance metric to visualize differences in species composition between the two time periods. The NMDS provides a two-dimensional representation of the similarity or dissimilarity among samples based on species presence-absence data. To statistically test for differences in species composition between the 1973 and 2024 groups, we performed an Analysis of Similarities (ANOSIM) and a Permutational Multivariate Analysis of Variance (PERMANOVA) using the “vegan” package in R (Oksanen et al., 2020). ANOSIM is a non-parametric test that assesses whether differences between groups are statistically significant based on a dissimilarity matrix. PERMANOVA is a permutation-based multivariate analysis of variance that does not assume normal distributions and is suitable for complex ecological data. Both tests employed 999 permutations to assess the significance of group differences, providing robust statistical support for observed patterns. Multivariate analyses were conducted using R software v. 4.3.1 (R Core Team, 2023).
Our results provide an analysis of the plant diversity of Maltese rocky cliffs (Figure 2), both currently and historically, by comparing present data with that from 1973, highlighting changes in species richness, Shannon and Simpson diversity, and community structure over time. Among the species with the highest frequency of occurrence were Cheirolophus crassifolius (15 in 1973 and 18 in 2024), Salsola melitensis (13 in 1973 and 17 in 2024), and Hypericum aegypticum subsp. webbii (18 in 1973 and 15 in 2024). Comparative analysis between 1973 and 2024 revealed minimal discrepancies; notably, Salsola melitensis exhibits a slight increase in 2024, while Hypericum aegypticum subsp. webbii shows a modest decline. A significant deviation is observed in Chiliadenus bocconei, which shows more than 50% reduction in occurrences in our 2024 surveys.
Figure 2. Maltese rocky cliffs landscape: (A) Dingli cliffs; (B) Għar Ħasan; (C) Miġra l-Ferħa; (D) Ta’ Ħammud. Photographs by Gianmarco Tavilla.
Analyzing the chart of life forms (Figure 3), it is evident that in 1973 the flora of Maltese rocky cliffs was predominantly represented by chamaephytes (44.74%), hemicryptophytes (26.32%), and nanophanerophytes (18.42%). Although the differences were not particularly striking, a shift in the percentages of these life forms can be observed in 2024. Overall, there is a reduction in some life forms, accompanied by a sometimes-substantial increase in others. This is particularly evident in the case of therophytes, which now account for 17.95%, while for phanerophytes and geophytes there was an approximate doubling in relative representation.
Figure 3. Life form spectra of taxa recorded: (CH) chamaephytes; (H) hemicryptophytes; (NP) nanophanerophytes; (T) therophytes; (P) phanerophytes; (G) geophytes.
Regarding diversity order q=0 (species richness), in 1973, estimated sample coverage (SC) starts at 0.494 with minimal sampling effort (t = 1) and gradually increases to 0.999 as the sample size reaches t = 36 (Figure 4). The estimated species richness (qD) rises from 11.67 to 38.19, indicating that a significant portion of species diversity was captured even at low sampling levels, with further sampling leading to incremental increases in species discovery. In 2024, the initial sample coverage is slightly lower at 0.453 (t = 1), reaching 0.991 by t = 36. The estimated species richness increases from 8.39 to 39.98. Notably, at lower coverage levels, species richness in 2024 is less than in 1973, suggesting fewer species are initially detected in 2024 despite similar sampling efforts. However, at higher coverage levels (SC ≈ 0.94), the estimated richness in 2024 (35.46) surpasses that of 1973 (33.66). Achieving this level of coverage requires a larger sample size in 2024 (t = 17) compared to 1973 (t = 10), indicating a higher prevalence of rare species or a more uneven distribution in 2024. In 2024, sample coverage was lower (0.946) with an estimated richness of 36.00, but in 1973, sample coverage was 0.984 with an estimated richness of 37.00. These contrasts are further highlighted by the observed data at t = 18.
Figure 4. Diversity results: (A) Sample completeness curve; (B) Coverage-based rarefaction and extrapolation sampling curve for q=0; (C) Coverage-based rarefaction and extrapolation sampling curve for q=1; (D) Coverage-based rarefaction and extrapolation sampling curve for q=2; (E) Sample-size-based rarefaction and extrapolation sampling curve for q=0; (F) Sample-size-based rarefaction and extrapolation sampling curve for q=1; (G) Sample-size-based rarefaction and extrapolation sampling curve for q=2.
For diversity order q=1 (Shannon diversity), in 1973, at SC = 0.494 with minimal sampling effort (t = 1), the estimated diversity is 11.67 species, indicating a relatively high diversity even at low coverage. In 2024, at a similar coverage level of 0.453 (t = 1), the estimated diversity was significantly lower at 8.39 species, suggesting that the 1973 plant community was more diverse and species were more evenly distributed. As sampling effort and coverage increase, diversity estimates in both years rise, but 1973 consistently shows higher diversity. For instance, at SC ≈ 0.95 (t = 11 in 1973), estimated diversity reaches 26.45, while in 2024, at a comparable SC of 0.95 (t = 19), it is lower at 24.38. Examining observed data at t = 18, the estimated diversity in 1973 is 27.81 species with high sample coverage (0.984), whereas in 2024, at the same sampling effort, diversity is 24.21 with a lower sample coverage of 0.946.
For diversity order q=2 (Simpson diversity), in 1973, the estimated diversity at low sample coverage (SC = 0.494, t = 1) is 11.67 species, reflecting a community with no overwhelming species dominance. In contrast, in 2024, diversity at a similar coverage level is lower at 8.39 species, indicating that dominant species in 2024 are more abundant relative to others, leading to lower overall diversity. As sampling effort and coverage increase, both assemblages show rising diversity, but consistent gaps between the two years reveal important differences. In 1973, at SC ≈ 0.95, the estimated diversity was 21.62 species; in 2024, it decreased to 17.37 species. The analysis of observed data at t = 18 indicates that species diversity in 1973 was at 22.36 species (SC = 0.984), whereas, in 2024, it declined to 17.37 species (SC = 0.946).
In 1973, for diversity order q=0, at the smallest sample size (t = 1), the estimated species richness (qD) was 11.67 species (SC = 0.494). As sampling extends to t = 10, nearly all species are detected (Figure 4), with estimated richness reaching 33.66 (SC = 0.94). By t = 18, the richness reaches 37.00 (SC = 0.984), suggesting that most of the total diversity has been detected with relatively modest effort. In 2024, initial richness is lower at 8.39 species (SC = 0.453, t = 1). At comparable sample sizes (t = 10), estimated richness is also lower (30.13, SC = 0.876), and at t = 18, it reaches 36.00 but with lower coverage (0.946), indicating that fewer species are detected within similar sampling limits compared to 1973. When sampling extends to t = 36, the estimated richness in 2024 surpasses that of 1973, reaching 39.98 species (SC = 0.991). However, confidence intervals are more comprehensive, suggesting more significant uncertainty due to a higher prevalence of rare species.
For diversity order q=1, in 1973, with minimal sampling (t = 1), the estimated diversity was 11.67 species (SC = 0.494). At t = 10, diversity rises to 26.11 species (SC = 0.94), indicating a well-distributed diversity among species. In 2024, diversity starts lower at 8.39 species (SC = 0.453, t = 1) and reaches 21.87 species (SC = 0.876) by t = 10, suggesting a less even species distribution. At t = 18, the diversity estimate in 1973 is 27.81 species (SC = 0.984), while in 2024 it is 24.21 species (SC = 0.946).
For diversity order q=2, in 1973, starting with t = 1, the estimated diversity was 11.67 species (SC = 0.494). By t = 10, diversity among dominant species is 21.44 (SC = 0.94), showing a balanced distribution. In 2024, diversity estimates are consistently lower, indicating an increase in dominance and a decline in the evenness of common species over time. At t = 18, the estimated diversity in 1973 is 22.36 species (SC = 0.984), compared to 17.37 species (SC = 0.946) in 2024, reinforcing the observation that species dominance has increased, reducing diversity.
To assess the naturalness of rocky cliffs in Malta, we compared the comprehensive datasets from 1973 and 2024 with simplified versions that exclude species not specific to these environments. The following results present this comparative analysis (Figure 5). Concerning the cover-based approach for order q=0, the whole dataset shows a uniform species distribution throughout the rocky environment in 1973, with a greater starting species richness and a progressive rise with sample coverage. The full data set for 2024 shows a delayed increase in species detection and a lower initial richness of species, indicating fewer species overall and a greater prevalence of rarer ones. In the simplified dataset, this trend is exacerbated by a quicker plateau and decline in species richness, suggesting fewer typical species in 1973. The dataset from 1973 shows a high Shannon diversity index for diversity order q=1, reflecting both a balanced distribution of species and a wide range of them. Substantial diversity is still present in the more recent condensed dataset, indicating that the distinctive flora continues to play a vital role in maintaining the evenness of the community. In both datasets, Shannon diversity declined by 2024, with the simpler version emphasizing the dominance of fewer species. For diversity order q=2, in 1973, the Simpson diversity values suggest a stable community with multiple co-dominant species in the complete dataset, which is similarly reflected in the simplified dataset. In contrast, the 2024 complete dataset and simplified version show a marked decrease in Simpson diversity, indicating that a few species have become increasingly dominant.
Figure 5. Diversity results with clean dataset: (A) Sample completeness curve; (B) Coverage-based rarefaction and extrapolation sampling curve for q=0; (C) Coverage-based rarefaction and extrapolation sampling curve for q=1; (D) Coverage-based rarefaction and extrapolation sampling curve for q=2; (E) Sample-size-based rarefaction and extrapolation sampling curve for q=0; (F) Sample-size-based rarefaction and extrapolation sampling curve for q=1; (G) Sample-size-based rarefaction and extrapolation sampling curve for q=2.
Regarding the size-based method for diversity order q=0, the size-based analysis of the complete dataset shows a steady increase in species richness with sampling in 1973, indicating that more species would be detected with increased sampling effort. The simplified dataset, focusing on characteristic species, reaches an earlier plateau, showing the core flora is well-sampled with fewer efforts. In contrast, the 2024 simplified dataset shows a rapid plateau at lower richness values, suggesting limited species diversity in characteristic species and indicating a more homogenized and potentially stressed ecosystem. For diversity order q=1, the 1973 data show balanced Shannon diversity across the community, with the simplified dataset maintaining diversity among typical species. In 2024, however, both datasets exhibit reduced diversity, with the simplified version showing significant declines in the diversity of the core species. For diversity order q=2, Simpson diversity values in the complete and simplified datasets indicate a well-balanced dominance structure among species in 1973, showing a healthy ecosystem with multiple co-dominant species. The 2024 results, however, show that Simpson diversity in the simplified dataset is markedly lower, indicating that only a few characteristic species dominate the community.
Analyzing empirical and estimated diversity and entropy profiles for 1973 and 2024 offers insights into the shifts in floristic composition over five decades (Figure 6). These profiles allow us to assess species richness, evenness, and dominance changes by examining diversity across different orders (q), with empirical values reflecting observed data and estimated values based on modeled predictions. In 1973, empirical species richness (q=0), which does not account for species abundances, was 37.00 species (s.e. = 1.50; CI: 34.40–39.42). For 2024, the estimated species richness at q=0 is slightly lower, at 36.00 species (s.e. = 2.02; CI: 32.11–39.11), indicating relative stability in total species counts. Despite overlapping confidence intervals, the minimal difference hints at a stable number of species. As q increases, giving greater weight to species abundances, differences between years become more apparent. For example, at q=0.25, diversity in 1973 drops to 34.29 species (s.e. = 1.34; CI: 31.51–36.80), while in 2024, it declines more steeply to 32.65 species (s.e. = 1.80; CI: 29.41–35.87). By q=1 (Shannon entropy), which balances richness and evenness, 1973 has 27.81 species (s.e. = 1.18; CI: 25.46–30.18), versus 24.21 species in 2024 (s.e. = 1.43; CI: 21.64–27.13), suggesting lower evenness in the latter year. The divergence intensifies at higher orders like q=2 (Simpson’s diversity index), which emphasizes common species. In 1973, diversity at q=2 is 22.36 species (s.e. = 1.10; CI: 20.23–24.61), significantly higher than 2024’s 17.37 species (s.e. = 1.18; CI: 14.94–19.78). By q=3, these values shift to 19.29 species (1973) and 14.10 species (2024), indicating increased dominance by a few species in 2024. Entropy values reinforce these findings. Higher entropy denotes greater evenness and diversity. In 1973, entropy at q=1 is 3.33 (s.e. = 1.18; CI: 2.15–4.50), slightly exceeding the 2024 value of 3.19 (s.e. = 1.43; CI: 1.76–4.61). While the difference is minor, it becomes more evident at higher q values. In summary, while overall species richness remains similar across decades, the decreased diversity at higher q values suggests a reduction in evenness and an increase in species dominance by 2024.
Figure 6. Asymptotic analysis – Diversity profiles: (A) Empirical with full dataset; (B) Estimated with full dataset; (D) Empirical with clean dataset; (C) Estimated with clean dataset.
The NMDS analysis, conducted on presence-absence data from two floristic datasets (1973 and 2024), revealed distinct clustering patterns between the two time periods (Figure 7). The NMDS plot shows two main clusters, with Group 1 representing the 1973 dataset and Group 2 representing the 2024 dataset. The distinct separation between these clusters suggests notable changes in species composition over time. We performed ANOSIM test to evaluate these differences statistically. The ANOSIM results (Supplementary Table S2) yielded an R statistic of 0.371 with a significance level of 0.001, indicating a significant distinction in community composition between the two groups. The distribution of dissimilarity ranks shows that the median rank for “between-group” dissimilarities is substantially higher than those within each group, confirming the temporal divergence in species assemblages. Furthermore, a PERMANOVA analysis was conducted to validate the observed compositional differences between the two datasets (Supplementary Table S3). The PERMANOVA results (F = 5.744, p = 0.001) confirmed a significant variation in species composition across the two periods. The R-squared (R²) values further indicate that approximately 66.67% of the variation in species composition can be attributed to temporal changes.
Figure 7. Non-Metric Multidimensional Scaling (NMDS): Group 1, 1973 dataset; Group 2, 2024 dataset. Stress = 0.2057.
Our approach has effectively assessed the current plant diversity of Maltese coastal cliffs by comparing it with data collected in 1973, 51 years prior. The NMDS suggest a significant temporal shift in community structure between 1973 and 2024, potentially reflecting ecological changes or anthropogenic impacts over the intervening decades. Utilizing Hill numbers, we evaluated plant diversity in 2024 and compared it to 1973. Based on the coverage- and size-based method results, the 1973 community exhibited higher species richness and a more balanced distribution of abundances. In contrast, the analysis under the naturalness hypothesis indicates a decline in the variety of distinct species over time, likely driven by environmental stress or habitat degradation altering the natural composition of the community. By 2024, Shannon diversity had decreased in both datasets, with the simplified dataset emphasizing the growing dominance of fewer species. This trend suggests a shift towards a less evenly distributed community, potentially due to the expansion of invasive species or habitat modifications that disrupt the equilibrium of rocky vegetation. Evidence of reduced dominance diversity among characteristic species is particularly apparent in the simplified dataset, indicating a decline in community resilience as the natural species balance deteriorates. Moreover, the marked reduction in Simpson diversity within the 2024 simplified dataset suggests that only a few dominant species now prevail, further reinforcing the impact of environmental disturbances, such as urbanization, habitat fragmentation, and tourism. These factors appear to have altered the habitat structure, favoring dominant species while reducing the characteristic diversity of natural rocky environments. Additionally, high diversity does not always correspond to a favorable conservation status; for instance, the presence of invasive or non-native species can increase diversity but may indicate habitat degradation.
To achieve a more accurate assessment of conservation status, we also focused on evaluating the presence of key or indicator species specific to Maltese rocky cliffs, aiming to assess conditions that reflect greater naturalness (Morabito et al., 2024). The results show a strong representation of both common and unusual species, and this pattern points to a diversified environment. Conversely, the simplified dataset, which excludes non-typical species, presents a somewhat reduced species richness yet still captures a large portion of the characteristic flora. This reduced dataset plateaus earlier, indicating that typical species are well-detected with moderate sampling effort, reflecting a stabilized natural community. To better understand these findings, it is essential to consider the ecological characteristics of rocky habitats, which play a crucial role in shaping species diversity and distribution.
Rocky habitats, often perceived as inhospitable, surprisingly harbor specialized flora. In fact, rocky outcrops are stressful habitats, in which local processes constrain diversity by the action of strong physical local filters, competition for scarce resources (e.g., soil, nutrients, water), and frequent disturbances (Scrosati et al., 2011; Chappuis et al., 2014; Keddy and Laughlin, 2022). However, substrate availability can also negatively impact species richness, likely due to competitive exclusion in areas where resources are more abundant and microenvironmental conditions are more homogeneous. On the other hand, vegetation cover enhances plant diversity likely due to the presence of more individuals. This increase can occur through the gradual colonization of species from the regional pool or through facilitation mechanisms among different species (Callaway, 1997; Storch et al., 2018; Sánchez-Martín et al., 2023). In this study, we observed relatively high species richness, which also demonstrated considerable temporal stability. The rocky substrate enhances the structural complexity of the landscape, providing refuge from extreme environmental conditions and encouraging species specialization (Lambrinos et al., 2006; Peters et al., 2008). This could represent the case of Atriplex lanfrancoi, a rare and endemic species unique to Malta. It is thought to represent an ancient lineage that likely endured the Ice Age exclusively within the Maltese Islands, serving as a potential ancestor to the more recently evolved sea-purslanes. Hence, these cliffs host numerous Maltese endemic species, which have had sufficient time to undergo all the necessary speciation processes. Additionally, Camilleri et al. (2024b) found topographic complexity was a consistent and significant predictor for both the number of taxa and the proportion of endemic taxa, whereas isolation was significant only for the proportion of endemic species. This highlights that complex topography, which creates diverse microhabitats and isolation within an island, supports speciation by providing varied ecological niches and limiting gene flow. The complex topography of rocky areas creates a variety of microhabitats, each characterized by different exposures to sunlight, temperature fluctuations, soil moisture levels, and nutrient availability (Crous et al., 2013; García et al., 2020). This heterogeneity facilitates the presence of a broader range of plant species, each specifically adapted to its unique environmental conditions. Moreover, the crevices and cliffs can mitigate extreme temperature variations, providing refuge for more sensitive species (García et al., 2020). Recent studies have shown that topography produces temperature mosaics with variations at small spatial scales that are comparable to those found over long elevational gradients (Scherrer and Köerner, 2010, 2011; Suggitt et al., 2018). Hence, we can understand the landscape not just as a random assortment of species, but as a means of recognizing the role of specific functional groups and their abiotic correlates in shaping patterns of plant biodiversity (Purvis and Hector, 2000).
To survive in rocky environments, plants develop unique adaptations to address challenges related to water and nutrients. These adaptations may include deep root systems that reach water, succulent leaves for water storage, or prostrate growth forms that resist wind. All morphological features are also found in the dominant species of the Maltese cliffs. The variety of these adaptation strategies contributes to the diversity of plant species. However, while rocky environments create unique habitats, the availability of substrate for plant growth can limit species richness due to competitive exclusion (Mai et al., 2024). Light acts as an environmental filter that influences which species can survive and thrive under specific conditions. In areas with high light exposure, such as open rock outcrops, plant communities are often dominated by species that are adapted to high solar irradiance. These species typically possess traits such as thick, leathery leaves to minimize water loss, or specialized photosynthetic mechanisms that optimize efficiency in intense light conditions (Rafiee et al., 2022; Mai et al., 2024). Among the most common species, we find two Amaranthaceae: Atriplex lanfrancoi and Salsola melitensis. All exhibit clear adaptations that enable them to thrive in these environments, such as the succulent leaves present in the latter two species.
Rupicolous plant communities, while seemingly resilient, face several significant threats. Coastal urbanization, habitat fragmentation, and the invasion of non-native species pose considerable challenges to conserving these unique ecosystems. Human activities and climate change cause habitat loss, threatening the survival of many species, particularly endemic ones (Laface et al., 2023). Changes in micro-relief, the introduction of alien species, and alterations to natural conditions due to the marine stress gradient can undermine the factors that support species coexistence and biodiversity (Gadino and Taveira, 2020). Several Maltese areas that are popular with climbers, may see a substantial decline in plant abundance, coverage, and diversity due to climbing activities, regardless of their intensity (Baldacchino and Caruana, 2024). Recent studies have highlighted this issue, showing that the dominant plant species, along with some rare species, have been particularly affected (March-Salas et al., 2023; Morales-Armijo et al., 2024). Therefore, effective conservation and management strategies are essential to mitigate the harmful effects of human activities on these biodiversity-rich habitats.
Based on both the calculated diversity indices and the analysis of biological forms, this habitat appears to be in relatively good condition, as the overall decline has been minimal. However, the most severe threat currently affecting these habitats seems to be climate change and rising temperatures. Increased temperatures and shifts in precipitation patterns can also significantly affect rocky plant communities (Kazakis et al., 2021). A significant warning sign is the notable increase in therophytes. These annual plants, which survive unfavorable conditions as seeds, are particularly favored by hot, seasonal, and dry climates. They thrive in habitats with low water and soil nitrogen availability (Taylor et al., 2023; Prochazka et al., 2024). The impact of climate change on natural habitats in Malta appears to be continually increasing, with noticeable shifts in species composition already evident over relatively short timeframes (Tavilla et al., 2024b).
To conclude, our findings provide a valuable basis for sustainable environmental management. They also highlight the significance of utilizing Hill numbers to assess plant diversity when evaluating the conservation status of rocky cliffs. This study represents a preliminary assessment of Maltese plant diversity in this environment. Such research is essential, as this habitat type falls under 8210 “Calcareous rocky slopes with chasmophytic vegetation”, a habitat listed in Annex I of the Habitats Directive. The significance of this work lies not only in its findings but also in the methodology applied, marking the first study of its kind in Malta. In contrast to previous investigations by Brullo et al. (2020), which focused solely on phytosociological analysis, this study integrates a broader ecological perspective to better understand the underlying dynamics of these plant communities. To deepen our understanding of the ecological processes governing these environments, future research should incorporate a long-term monitoring strategy, addressing both biotic and abiotic factors influencing vegetation dynamics. A key step in this direction would be the establishment of permanent plots, systematically monitored using high-resolution drone imagery and remote sensing techniques. This approach would allow for the detection of subtle vegetation monitoring especially in protected areas (Tavilla et al., 2024a). This would be particularly valuable given the increasing pressures from rising temperatures, demographic expansion, and tourism, which are likely to be key drivers of vegetation shifts in the Maltese Islands. From a conservation perspective, limiting human disturbance in the most vulnerable areas should be a priority. The implementation of visitor regulations and awareness campaigns aimed at promoting responsible tourism would contribute to the sustainable management of these fragile ecosystems. Additionally, fostering collaborations with local authorities and conservation organizations could facilitate the development of adaptive management strategies tailored to the specific needs of these environments. By establishing a robust, interdisciplinary monitoring framework, this research aims to provide a foundation for long-term conservation efforts, ensuring that the exceptional plant biodiversity of Maltese rocky habitats is preserved for future generations.
The raw data supporting the conclusions of this article will be made available by the authors, without undue reservation.
GT: Conceptualization, Data curation, Formal Analysis, Investigation, Methodology, Supervision, Validation, Visualization, Writing – original draft, Writing – review & editing. SL: Investigation, Supervision, Visualization, Writing – review & editing.
The author(s) declare that no financial support was received for the research and/or publication of this article.
We thank the reviewers for their helpful suggestions that improved the manuscript.
The authors declare that the research was conducted in the absence of any commercial or financial relationships that could be construed as a potential conflict of interest.
The author(s) declare that no Generative AI was used in the creation of this manuscript.
All claims expressed in this article are solely those of the authors and do not necessarily represent those of their affiliated organizations, or those of the publisher, the editors and the reviewers. Any product that may be evaluated in this article, or claim that may be made by its manufacturer, is not guaranteed or endorsed by the publisher.
The Supplementary Material for this article can be found online at: https://www.frontiersin.org/articles/10.3389/fcosc.2025.1552578/full#supplementary-material
Baldacchino G., Caruana S. (2024). Sport Tourism in Malta: Getting off the Beach, in Sport Tourism, Island Territories and Sustainable Development. Sports Economics, Management and Policy Vol. 24. Eds. Rheenen D.V., Naria O., Melo R., Sobry C. (Cham, Switzerland: Springer Nature), 111–122. doi: 10.1007/978-3-031-51705-1_7
Biondi E., Blasi C., Burrascano S., Casavecchia S., Copiz R., Del Vico E., et al. (2024). Manuale Italiano di Interpretazione Degli Habitat Della Direttiva 92/43/CEE. (Società Botanica Italiana. Ministero dell’Ambiente e Della Tutela del Territorio e del Mare, D.P.N). Available online at: http://vnr.unipg.it/habitat (Accessed November 14, 2024).
Braun-Blanquet J. (1964). Pflanzensoziologie: Grundzuege der Vegetationskunde; Dritte, Neubearbeitete und Wesentlich Vermehrte Auflage (Vienna: Springer).
Brullo S., Brullo C., Cambria S., Giusso del Galdo G. (2020). The Vegetation of the Maltese Islands (Cham: Springer Nature).
Brullo S., Brullo C., Cambria S., Minissale P., Sciandrello S., Giuseppe S., et al. (2023). Taxonomical remarks on Solenopsis laurentia (Campanulaceae) in Italy. Phytotaxa 58 4, 59–88. doi: 10.11646/phytotaxa.584.2.1
Callaway R. M. (1997). Positive interactions in plant communities and the individualistic-continuum concept. Oecologia 112, 143–149. doi: 10.1007/s004420050293
Cambria S., Azzaro D., Caldarella O., Aleo M., Bazan G., Guarino R., et al. (2023). New data on native and alien vascular flora of sicily (Italy): new findings and updates. Plants 12, 1743. doi: 10.3390/plants12091743
Camilleri L., Debono K., Grech F., Bellia A. F., Pace G., Lanfranco S. (2024b). Topographic complexity is a principal driver of plant endemism in mediterranean islands. Plants 13, 546. doi: 10.3390/plants13040546
Camilleri L., Ghose Roy R., Buhagiar J., Lanfranco S. (2024a). Quantification of the size and distribution of the only known population of Crepis pusilla (Sommier) Merxm. (Asteraceae Cichorieae) Malta. Webbia 79, 183–189. doi: 10.36253/jopt-15219
Chao A., Gotelli N. J., Hsieh T. C., Sander E. L., Ma K. H., Colwell R. K., et al. (2014). Rarefaction and extrapolation with Hill numbers: a framework for sampling and estimation in species diversity studies. Ecol. Monogr. 84, 45–67. doi: 10.1890/13-0133.1
Chao A., Hsieh T. C., Ma K. H. (2016). User's guide for iNEXT Online: software for interpolation and extrapolation of species diversity. Available online at: https://www.researchgate.net/publication/307598336 (Accessed November 18, 2024).
Chao A., Jost L. (2012). Coverage-based rarefaction and extrapolation: standardizing samples by completeness rather than size. Ecology 93, 2533–2547. doi: 10.1890/11-1952.1
Chao A., Jost L. (2015). Estimating diversity and entropy profiles via discovery rates of new species. Methods Ecol. Evol. 6, 873–882. doi: 10.1111/2041-210X.12349
Chao A., Kubota Y., Zelený D., Chiu C. H., Li C. F., Kusumoto B., et al. (2020). Quantifying sample completeness and comparing diversities among assemblages. Ecol. Res. 35, 292–314. doi: 10.1111/1440-1703.12102
Chao A., Wang Y. T., Jost L. (2013). Entropy and the species accumulation curve: a novel entropy estimator via discovery rates of new species. Methods Ecol. Evol. 4, 1091–1100. doi: 10.1111/2041-210X.12108
Chappuis E., Terradas M., Cefalì M. E., Mariani S., Ballesteros E. (2014). Vertical zonation is the main distribution pattern of littoral assemblages on rocky shores at a regional scale. Estuar. Coast. Shelf Sci. 147, 113–122. doi: 10.1016/j.ecss.2014.05.031
Chytrý M., Otýpková Z. (2003). Plot sizes used for phytosociological samplingof European vegetation. J. Veg. Sci. 14, 563–570. doi: 10.1111/j.1654-1103.2003.tb02183.xopen_in_new
Colwell R. K., Chao A., Gotelli N. J., Lin S. Y., Mao C. X., Chazdon R. L., et al. (2012). Models and estimators linking individual-based and sample-based rarefaction, extrapolation, and comparison of assemblages. J. Plant Ecol. 5, 3–21. doi: 10.1093/jpe/rtr044
Crous C. J., Samways M. J., Pryke J. S. (2013). Associations between plant growth forms and surface rockiness explain plant diversity patterns across an Afro-montane grassland landscape. S. Afr. J. Bot. 88, 90–95. doi: 10.1016/j.sajb.2013.05.006
Directive H. (1992). Council Directive 92/43/EEC of 21 May 1992 on the conservation of natural habitats and of wild fauna and flora. Off. J. Eur. Union 206, 50.
ERA-Environment and Resources Authority (2019). NATURA 2000 - STANDARD DATA FORM, MT0000024. Available online at: https://era.org.mt/wp-content/uploads/2019/05/20180601_MT0000024-Rdum-Malta-SAC.pdf (Accessed November 14, 2024).
ERA - Environment and Resources Authority (2024). Natura 2000 in Malta. Available online at: https://era.org.mt/topic/natura-2000-in-malta/ (Accessed November 14, 2024).
Fischer E. M., Schär C. (2010). Consistent geographical patterns of changes in high-impact European heatwaves. Nat. Geosci 3, 398–403. doi: 10.1038/ngeo866
Gadino I., Taveira G. (2020). Ordenamiento y gestión del territorio en zonas costeras con turismo residencial. El caso de Región Este, Uruguay. Rev. Geogr. Norte Gd. 77, 233–251. doi: 10.4067/S0718-34022020000300233
Galdies C. (2011). The Climate of Malta: statistics, trends and analysis 1951-2010 (Valletta: National Statistics Office).
García M. B., Domingo D., Pizarro M., Font X., Gómez D., Ehrlén J. (2020). Rocky habitats as microclimatic refuges for biodiversity. A close-up thermal approach. Environ. Exp. Bot. 170, 103886. doi: 10.1016/j.envexpbot.2019.103886
Gouveia C. M., Trigo R. M., Beguería S., Vicente-Serrano S. M. (2017). Drought impacts on vegetation activity in the Mediterranean region: An assessment using remote sensing data and multi-scale drought indicators. Glob. Planet. Change 151, 15–27. doi: 10.1016/j.gloplacha.2016.06.011
Hsieh T. C., Ma K. H., Chao A. (2016). iNEXT: an R package for rarefaction and extrapolation of species diversity (Hill numbers). Methods Ecol. Evol. 7, 1451–1456. doi: 10.1111/2041-210X.12613
Kazakis G., Ghosn D., Remoundou I., Nyktas P., Talias M. A., Vogiatzakis I. N. (2021). Altitudinal vascular plant richness and climate change in the alpine zone of the Lefka Ori, Crete. Diversity 13, 22. doi: 10.3390/d13010022
Keddy P. A., Laughlin D. C. (2022). A framework for community ecology: species pools, filters and traits (Cambridge: Cambridge University Press).
Kiss E., Fazekas I., Zichar M., Balla D. (2020). “A comprehensive investigation of climate change strategies in the Mediterranean region,” in Proceedings of the 4th Edition of International Conference on Geo-IT and Water Resources, (New York, USA: Association for Computing Machinery), 2020, 1–5.
Laface V. L. A., Musarella C. M., Tavilla G., Sorgonà A., Cano-Ortiz A., Quinto Canas R., et al. (2023). Current and potential future distribution of endemic salvia ceratophylloides ard. (Lamiaceae). Land 12, 247. doi: 10.3390/land12010247
Lambrinos J. G., Kleier C. C., Rundel P. W. (2006). Plant community variation across a puna landscape in the Chilean Andes. Rev. Chil. Hist. Nat. 79, 233–343. doi: 10.4067/S0716-078X2006000200009
Lanfranco E. (1995). The Maltese flora and conservation. Ecol. Medit 21, 165–168. doi: 10.3406/ecmed.1995.1763
Mai P., Marchesi E., Pollero A., Zabaleta M., Cappuccio L., Fernández S., et al. (2024). Unraveling local and regional determinants of high plant diversity at marine rocky outcrops in Uruguay. J. Veg. Sci. 35, e13284. doi: 10.1111/jvs.13284
March-Salas M., Morales-Armijo F., Hernández-Agüero J. A., Estrada-Castillón E., Sobrevilla-Covarrubias A., Arévalo J. R., et al. (2023). Rock climbing affects cliff-plant communities by reducing species diversity and altering species coexistence patterns. Biodivers. Cons. 32, 1617–1638. doi: 10.1007/s10531-023-02567-1
Maturo F., Di Battista T. (2018). A functional approach to Hill's numbers for assessing changes in species variety of ecological communities over time. Ecol. Indic. 84, 70–81. doi: 10.1016/j.ecolind.2017.08.016
Medail F., Quezel P. (1997). Hot-spots analysis for conservation of plant biodiversity in the Mediterranean Basin. Ann. Missouri Bot. Gard 84, 112–127. doi: 10.2307/2399957
Mifsud S. (2013). Distribution of some rare or endemic chasmophytic and rupestral species growing along the coastal cliffs of the Maltese Islands. Webbia 68, 35–50. doi: 10.1080/00837792.2013.807451
Minissale P., Cambria S., Montoleone E., Tavilla G., Giusso del Galdo G., Sciandrello S., et al. (2023). The alien vascular flora of the Pantelleria Island National Park (Sicily Channel, Italy): new insights into the distribution of some potentially invasive species. BioInvasions Rec. 12, 861–885. doi: 10.3391/bir.2023.12.4.01
Morabito A., Musarella C. M., Caruso G., Spampinato G. (2024). Biodiversity as a tool in the assessment of the conservation status of coastal habitats: A case study from Calabria (Southern Italy). Diversity 16, 535. doi: 10.3390/d16090535
Morales-Armijo F., Sobrevilla-Covarrubias A., Estrada-Castillón E., Escudero A., Scheepens J. F., Lorite J., et al. (2024). Climbing route development affects cliff vascular plants more than subsequent climbing: A guide to evidence-based conservation management to regulate climbing. J. Appl. Ecol. 61, 2679–2689. doi: 10.1111/1365-2664.14785
Myers N., Mittermeier R. A., Mittermeier C. G., Da Fonseca G. A., Kent J. (2000). Biodiversity hotspots for conservation priorities. Nature 403, 853–858. doi: 10.1038/35002501
National Statistics Office (2023). Malta in Figures 2023. Available online at: https://nso.gov.mt/wp-content/uploads/Regional-Statistics-Malta-2023-Edition.pdf (Accessed November 14, 2024).
Oksanen J., Blanchet F. G., Friendly M., Kindt R., Legendre P., McGlinn D., et al. (2020). vegan: Community Ecology Package. R package version 2.5-7 Available online at: https://CRAN.R-project.org/package=vegan (Accessed November 14, 2024).
Peters E. M., Martorell C., Ezcurra E. (2008). Nurse rocks are more important than nurse plants in determining the distribution and establishment of globose cacti (Mammillaria) in the Tehuacán Valley, Mexico. J. Arid Env. 72, 593–601. doi: 10.1016/j.jaridenv.2007.10.004
POWO (2024). Plants of the World Online. Facilitated by the Royal Botanic Gardens, Kew. Available online at: https://powo.science.kew.org/ (Accessed November 14, 2024).
Prochazka L. S., Alcantara S., Rando J. G., Vasconcelos T., Pizzardo R. C., Nogueira A. (2024). Resource availability and disturbance frequency shape evolution of plant life forms in Neotropical habitats. New Phytol. 242, 760–773. doi: 10.1111/nph.19601
Purvis A., Hector A. (2000). Getting the measure of biodiversity. Nature 405, 212–219. doi: 10.1038/35012221
Rafiee F., Ejtehadi H., Farzam M., Zare H., Bashirzadeh M. (2022). Changes in plant biodiversity facets of rocky outcrops and their surrounding rangelands across precipitation and soil gradients. Sci. Rep. 12, 9022. doi: 10.1038/s41598-022-13123-2
Raunkiaer C. (1934). The Life Forms of Plants and Statistical Plant Geography (Oxford: Oxford University Press).
R Core Team (2023). R: A Language and Environment for Statistical Computing (Vienna, Austria: R Foundation for Statistical Computing). Available online at: https://www.R-project.org/ (Accessed November 14, 2024).
Sánchez-Martín R., Verdú M., Montesinos-Navarro A. (2023). Interspecific facilitation favors rare species establishment and reduces performance disparities among adults. J. Veg. Sci. 34, 1–11. doi: 10.1111/jvs.13185
Schembri J. A. (2019). “The Geographical Context of the Maltese Islands,” in Landscapes and Landforms of the Maltese Islands. World Geomorphological Landscapes. Eds. Gauci R., Schembri J. A. (Springer Nature, Cham), 9–17.
Schembri P. J., Sultana J. (1989). Red data book for the Maltese Islands (Malta: Department of Information).
Scherrer D., Köerner C. (2010). Infra-red thermometry of alpine landscapes challenges climatic warming projections. Glob. Change Biol. 16, 2602–2613. doi: 10.1111/j.1365-2486.2009.02122.x
Scherrer D., Körner C. (2011). Topographically controlled thermal-habitat differentiation buffers alpine plant diversity against climate warming. J. Biogeogr. 38, 406–416. doi: 10.1111/j.1365-2699.2010.02407.x
Sciandrello S., Cambria S., Giusso del Galdo G., Guarino R., Minissale P., Pasta S., et al. (2021). Floristic and vegetation changes on a small mediterranean island over the last century. Plants 10, 680. doi: 10.3390/plants10040680
Scrosati R. A., van Genne B., Heaven C. S., Watt C. A. (2011). Species richness and diversity in different functional groups across environmental stress gradients: a model for marine rocky shores. Ecography 34, 151–161. doi: 10.1111/j.1600-0587.2010.06119.X
Storch D., Bohdalkova E., Okie J. (2018). The more individuals hypothesis revisited: the role of community abundance in species richness regulation and the productivity diversity relationship. Ecol. Lett. 21, 920–937. doi: 10.1111/ele.12941
Suggitt A. J., Wilson R. J., Isaac N. J. B., Beale C. M., Auffret A. G., August T., et al. (2018). Extinction risk from climate change is reduced by microclimatic buffering. Nat. Clim. Change 8, 713–717. doi: 10.1038/s41558-018-0231-9
Tavilla G., Crisafulli A., Minissale P., Tomaselli V., Adamo M. (2024a). The use of drones for cost-effective surveys in natura 2000 protected areas: A case study on monitoring plant diversity in sicily (Italy). Land 13, 804. doi: 10.3390/land13060804
Tavilla G., Lamoliere A., Gabarretta J., Attard V., Henwood J., Stevens D. T., et al. (2024b). Climate change and wetland ecosystems: the effects on halophilous vegetation diversity in il-Ballut ta’ Marsaxlokk natura 2000 site (Malta). Land 12, 1679. doi: 10.3390/land12091679
Tavilla G., Minissale P., Camilleri L., Lanfranco S. (2023). Addition of two species to the Maltese flora: Lythrum tribracteatum Salzm. ex Spreng. (Lythraceae) and Poa maroccana Nannf. (Poaceae). Check List 19, 743–751. doi: 10.15560/19.5.743
Keywords: incidence data, Malta flora, Mediterranean basin, vascular flora, Shannon diversity, Simpson diversity, species richness
Citation: Tavilla G and Lanfranco S (2025) Assessing plant species diversity in Maltese rocky cliffs using Hill numbers and its implications for conservation. Front. Conserv. Sci. 6:1552578. doi: 10.3389/fcosc.2025.1552578
Received: 28 December 2024; Accepted: 13 March 2025;
Published: 21 March 2025.
Edited by:
Brenda Molano-Flores, Illinois Natural History Survey (INHS), United StatesReviewed by:
Smrithy Vijayan, Agharkar Research Institute, IndiaCopyright © 2025 Tavilla and Lanfranco. This is an open-access article distributed under the terms of the Creative Commons Attribution License (CC BY). The use, distribution or reproduction in other forums is permitted, provided the original author(s) and the copyright owner(s) are credited and that the original publication in this journal is cited, in accordance with accepted academic practice. No use, distribution or reproduction is permitted which does not comply with these terms.
*Correspondence: Gianmarco Tavilla, Z3RhdmlsbGFAb3V0bG9vay5jb20=
Disclaimer: All claims expressed in this article are solely those of the authors and do not necessarily represent those of their affiliated organizations, or those of the publisher, the editors and the reviewers. Any product that may be evaluated in this article or claim that may be made by its manufacturer is not guaranteed or endorsed by the publisher.
Research integrity at Frontiers
Learn more about the work of our research integrity team to safeguard the quality of each article we publish.