- 1Marine and Environmental Biology Section, Department of Biological Sciences, University of Southern California, Los Angeles, CA, United States
- 2McGuire Center for Lepidoptera and Biodiversity, Department of Natural History, Florida Museum of Natural History University of Florida, Gainesville, FL, United States
- 3Department of Biology, Carleton University, Ottawa, ON, Canada
- 4Environmental Science and Forestry, State University of New York, Syracuse, NY, United States
- 5Center for Biodiversity and Conservation, American Museum of Natural History, New York, NY, United States
Introduction: The United States Endangered Species Act celebrated its 50th anniversary in 2023. As a hallmark piece of environmental legislation, the Act has successfully prevented the extinction of hundreds of species. During these last 50 years, we have observed the decline of many species of invertebrates, resulting in the listing of 356 species.
Methods: Here, we summarize the state of endangered invertebrates using text mining to review all listing documents, including listing decisions, species status assessments, critical habitat designations, and status reviews. In our review, we evaluate the most prevalent threats for aquatic and terrestrial invertebrates.
Results: We found that invertebrates have been assessed and listed consistently in the past 50 years, and the last eight years have seen an uptick in status reviews. Further, we find that pollution, natural system modifications (such as dams), and intrinsic factors (such as small population sizes or number of populations) are the major contributing threats to aquatic invertebrates. On the other hand, problematic biotic factors (such as invasive species), climate change, residential and commercial development, and pollution are the major threats to terrestrial invertebrates.
Discussion: Overall, our study reviews the current threats to invertebrates and provides a baseline for the next 50 years in the face of a shifting threat and conservation arena.
1 Introduction
Invertebrate conservation biology is an evolving discipline influenced by rapidly shifting scientific consensus, increased knowledge of threats faced by invertebrates, and economic and societal factors (New, 2012). As the most biodiverse group of animals on the planet, invertebrate conservation is further challenged due to a lack of structured monitoring data, lack of baseline knowledge of distributions, natural histories, and responses to global change, as well as a generally unfavorable view of so-called “creepy-crawlies” by the public (Cardoso et al., 2011; Didham et al., 2020; Mammola et al., 2020; Salvador et al., 2021). For many invertebrates, we lack even basic knowledge about their biology and ecological interactions, making it challenging to understand their specific conservation needs (Strayer, 2006; Cardoso et al., 2011). Even for more charismatic groups like dragon- and damselflies (Odonata), deficits in structured monitoring data and species-specific responses to climate change are apparent (Oliveira-Junior et al., 2022; Bried et al., 2020). Given the variety of threats faced by invertebrates and current records of their potential decline (Graves et al., 2020; Forister et al., 2021), evaluating the effectiveness of the tools we have available to protect species is of utmost importance, particularly as compared to the protection of more charismatic vertebrates. In the United States, the Endangered Species Act (ESA) is one tool that may facilitate invertebrate conservation.
The ESA has been a very successful piece of legislation, partly due to the strong protections it provides species and the constant review of species status. Ratified in 1973, the ESA garnered strong bipartisan support for protecting at-risk species in the United States (Williams, 1973). Now just over 50-years old, more than 1,300 species have been formally listed as threatened or endangered, and these listings have potentially protected the vast majority of species from the threat of extinction, according to a recent overview of the Act (Greenwald et al., 2019). Part of the success of preventing extinctions may come from the fact that the Act provides notable protections to species, including prohibitions on “take,” which is broadly defined as causing harm to listed organisms. Additionally, under the ESA, species recovery plans are produced, which outline the knowledge and actions required to conserve a species. These recovery plans detail population targets or goals as well as anticipated costs (Tear et al., 1993). The reality of these recovery plans has been the subject of much conversation in the literature, particularly surrounding the number of species that meet the median abundances set by these plans (Neel et al., 2012); how monitoring, restoration, and adaptive management are integrated into the recovery process (Bried et al., 2014); and overall allocation of budgetary resources to enacting recovery plans (Gerber, 2016). The express desire to enact post-delisting plans for continued management under the recovery framework has also been proposed (Scott et al., 2005).
In addition to recovery plans, reviews of species’ status are prescribed to occur every five years after initial listing in so-called five-year reviews; and additionally, introduced in 2016, species status assessments (SSAs) summarize all available knowledge regarding the focal species. These assessments aim to “deliver foundational science for informing all ESA decisions” (U.S. Fish & Wildlife Service, 2016; Smith et al., 2018). Previously, many assessments focused on the magnitude and immediacy of threats to a given species. However, they lacked numerical thresholds and criteria, did not explicitly analyze or predict a species’ response to those threats, and, as a result, had low transparency (Andelman et al., 2004). The current SSA is species-focused rather than threat-focused and uses all fundamental ecological information gathered about a species to make informed predictions about its current and future response to identified threats (Smith et al., 2018).
Invertebrates are the most diverse animal group, with approximately over 200,000 recorded species estimated to occur in North America (Global Biodiversity Information Facility, 2024b). However, it is unclear to what extent the Endangered Species Act has effectively conserved invertebrates compared to their more charismatic counterparts, such as vertebrates. The first invertebrate species listed in the Act’s early years included 21 species of clams and seven butterflies. Four of these initially listed clams have gone extinct, whereas all the initially listed butterflies are still considered endangered. Many of these species have seen their conservation outlooks improve, even if statuses have remained unchanged. For example, one of the main threats to the Karner Blue butterfly (Lycaeides melissa samuelis) at the time of its listing was habitat loss due wildfire and grazing suppression; without regular disturbance, succession inevitably causes the open habitats that its host plant (wild blue lupine, Lupinus perennis) requires to disappear (Haack, 1993). As a result of detailed statewide Habitat Conservation Plans (Hess and Hess, 2015) and local conservation efforts, including habitat restoration, replanting lupine and captive breeding, populations in some states such as Wisconsin and New Hampshire, have stabilized or are increasing (Swengel and Swengel, 2018; Lyons, 2022; Wheatley, 2022; New Hampshire Fish and Game Department, 2024). In other states, however, populations have continued to decline (Monfils et al., 2021), and climate change looms as an increasingly important threat. Already, this species has been potentially extirpated in Indiana after an unusually warm and dry summer (Patterson et al., 2020).
In total, 98 species of insects, 98 species of clams, 54 species of snails, 25 species of corals, 32 species of crustaceans, and 16 species of arachnids have been listed under the ESA, with many additional species currently petitioned or reviewed for potential listing (United States Fish and Wildlife Service, 2024a). Further, given the large corpus of documentation produced by the United States Fish and Wildlife Service (USFWS) as well as advances in text processing, we can now summarize these documents to better understand the threats to invertebrates listed under the ESA. In this review, we provide much-needed context for invertebrate conservation in the United States and beyond to address taxonomic and geographic areas of particular conservation concern.
On the 50th anniversary of the U.S. Endangered Species Act, we aimed to profile how the Act has worked for invertebrates and the most frequently identified threats to invertebrate populations across the country. We used text-mining approaches to summarize all available USFWS documentation. Specifically, we aimed to synthesize trends in documentation and identify threats to invertebrates over the last half-century using ChatGPT to “distill” ESA-relevant documentation. In doing so, we provide a first-ever comprehensive summary of USFWS documentation and threat indicators for aquatic and terrestrial invertebrates protected by the Act.
2 Methodology
To rapidly and accurately collect information on invertebrates listed under the ESA, we developed a web scraping pipeline to mine the USFWS Environmental Conservation Online System (ECOS) website and associated PDF files. We wrote a custom web scraper using the Python (v3.11.6) libraries selenium (Muthukadan, 2024) and BeautifulSoup (Richardson, 2007) to extract tables of species’ listing data, documentation, and relevant dates of document publication. Following this initial scraping, we used the Integrated Taxonomic Information System (ITIS; https://www.itis.gov) through the R package taxizedb (Chamberlain et al., 2023) to obtain higher taxonomic classifications through the kingdom level for all documents obtained through our scraping process (n = 9,379). We manually resolved higher taxonomy for cases where we received no results from our ITIS query and focused only on animals. Next, we split our dataset using this higher taxonomy into documents about species actively listed under the ESA for vertebrate (n = 1,340) and invertebrate (n = 915) groups. For each listed species, we classified the documents based on the document type, such as status assessments or other official documentation.
We scraped the URLs to all available documentation concerning each species. We completed this task using the above Python libraries and pdfplumber (Singer-Vine, 2024). Using a custom script that interfaced with the ChatGPT4-Turbo API (OpenAI, 2024), we provided the text from the URL link to the PDF files to obtain data on threats. We specifically issued the following prompt to ChatGPT4-Turbo for all PDF files in our corpus: “Given the text below, what are the identified threats to the species [Scientific Name]?”. We specified the scientific name for each document, as many documents simultaneously provided information for many species.
ChatGPT provided a paragraph from this prompt listing the threats identified in the document. To standardize these threats, we used the International Union for the Conservation of Nature’s (IUCN) Unified Threats Classification Scheme (Salafsky et al., 2008). The IUCN Unified Threats Classification Scheme categorizes threats into 11 discrete categories and an “other” option. We manually extracted and atomized threats for 100 randomly selected PDFs using the IUCN threat classification. Our initial approach attempted to atomize the paragraph provided by ChatGPT to the discrete categories using ChatGPT4-Turbo using the following prompt: “Using the IUCN’s Unified Threats Classification Scheme, atomize the following responses into a true/false CSV for each IUCN threat category; do not provide any additional text outside the table.” However, these secondary threat classification prompts to ChatGPT produced low-scoring results compared to human annotations (Supplementary Figure S1) and were prone to false threat identification. As a result, we shifted our approach to using a multinomial naive Bayes model for each threat category using our manually annotated data for training and validation. In short, the multinomial naive Bayes model took a frequency matrix of words and used that matrix to predict whether or not a specific threat was present for the species using Bayes Theorem (Duda and Hart, 1973). We trained our models using our human-annotated dataset, reserving 20% of our annotations for validation testing. We used the Python library scikitlearn to perform this task (Pedregosa et al., 2011). Because validation performance can be sensitive to training data, especially for small datasets, we trained multiple naive Bayes models by randomly shuffling our annotations up to 1,000 permutations. We ensured that at least one true and false identification appeared across all training datasets. We used naive Bayes instead of more complicated machine learning approaches since it performs well with small sample sizes and is computationally inexpensive to implement (Howedi and Mohd, 2014).
We ensembled our suite of naive Bayes models by calculating the prevalence of true threat identification values for each threat category across all models that obtained an F1-score of greater than 0.8. F1-scores measure model accuracy, accounting for both precision (positive predictive value) and recall (true positives) scores of each model (Taha and Hanbury, 2015). This procedure effectively ranks our confidence in identifying threat values in a given category. Thus, as a result of this procedure, all responses received a score between zero (no probability) and one (high probability) for each threat category, representing the prevalence of that threat being indicated from a particular paragraph extracted from ChatGPT.
Following data validation, we calculated simple summary statistics across the corpus. For each species, we downloaded all currently available data in the Global Biodiversity Information Facility (GBIF) (Global Biodiversity Information Facility, 2024a) via the R package ‘rgbif’ (Chamberlain and Boettiger, 2017), and binned it into 2-degree hex cells. We then summarized for each hex to calculate the total number of listed species in each hex. We also calculated the total number of cumulative threats across all species for each hex. We did this separately for both aquatic and terrestrial domains. Species that inhabit both aquatic and terrestrial domains (e.g., dragonflies) were included in the domain in which they begin their lives and are first exposed to potential threats. For example, in the case of dragonflies, this would be the aquatic domain. While we recognize this approach does not fully account for all potential threats to the species, it allows us to better visualize the threats.
All scraped, secondary data, and associated scripts are available via GitHub at https://github.com/EcolDataSciUSC/ESAInvertebratesReview; and on a static Zenodo archive at 10.5281/zenodo.13882703. Please note that a valid, paid key is required to issue queries via the ChatGPT API in the provided Python scripts.
3 Results
We scraped information from 687 documents on invertebrate conservation activity under the ESA. This reflects 75% of all available documentation for invertebrates. Missed documents included those with no searchable text or invalid URLs. Our ChatGPT extracted atomization and rankings did not perform well compared to human annotations on a subsample of 100 documents (73% mean agreement, but with high variation in performance across categories +/- 29% as one standard error)(Supplementary Table S1). A confusion matrix from the ChatGPT output for each threat is provided in Supplementary Figure S1. In contrast, our naive Bayes model performed well across 10 of the 11 IUCN threat categories and one additional category we defined called “Intrinsic Factors,” which included small population sizes/habitat fragmentation (Supplementary Figure S2). We excluded Transportation and Service Corridors, Geologic Events, and another custom category, Disease, from the results and discussion, based on poor Bayes model performance, likely because these threats did not occur frequently enough in the training data. The majority of listed species occur in the aquatic domain (n = 215; with the majority of those belonging to freshwater ecosystems, n = 206). The remaining species fall into the terrestrial (n = 99) and marine (n = 9) domains respectively.
Since introducing species status assessments (SSAs) in 2016, the agency’s priorities have shifted from new listings to conducting SSAs, recovery plans, and reviews (Figures 1a, c). Concurrently, productivity has significantly increased, with many of these documents completed within the last decade. These trends are evident for both invertebrates and vertebrates, and an equal amount of absolute effort is paid to both groups in producing documentation (Figure 1a). Despite this, given the substantially larger taxonomic diversity of invertebrates, there is likely a shortfall in assessing, listing, and reviewing invertebrates. When normalized by the estimated number of vertebrate (n = 7,378) and invertebrate (n = 30,284) species with over 100 reported occurrences in North America (Global Biodiversity Information Facility, 2024b, c), an apparent discrepancy emerges in the volume of documentation produced for invertebrates and vertebrate species (Figure 1b).
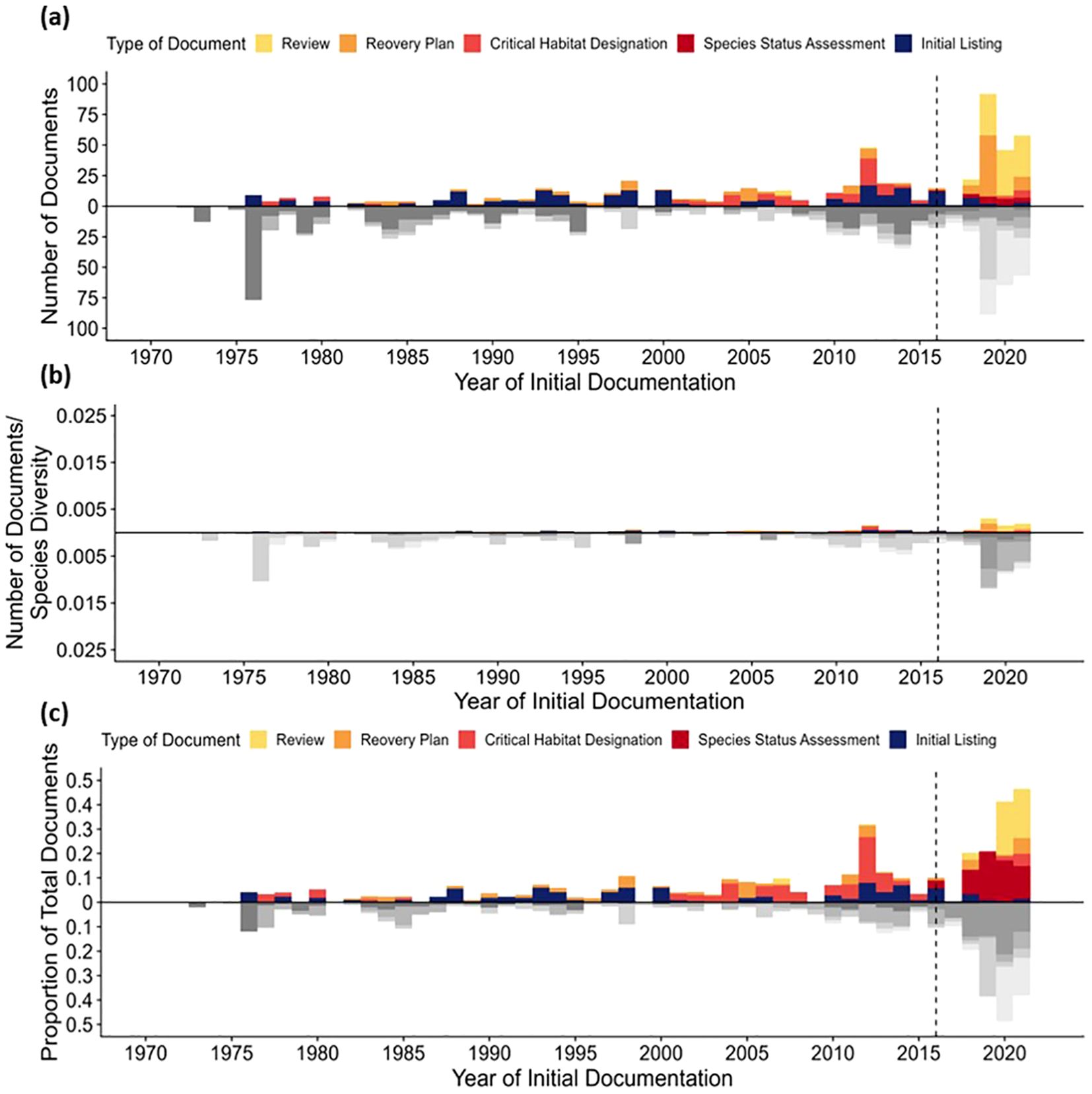
Figure 1. (a) A high-level summary of ESA-related documentation on vertebrates (greyscale) and invertebrates (color). The y-axis reflects the raw number of initial documentation completed for that taxon in that year. Panel (b) relates the raw number of documents produced to estimates of invertebrate and vertebrate species-level diversity across North America. Finally, panel (c) indicates the proportional rate of initial documents produced as a function of total documents produced for invertebrate and vertebrate taxa (e.g., roughly 5% of invertebrate initial listings were produced in 1976). A clear shift in documentation effort was observed when species status assessments were introduced in 2016 (dotted line)—shifting from listings and critical habitat designations to recovery plans, reviews, and species status assessments. This same period also saw a significant uptick in productivity from USFWS staff concerning the volume of documents produced.
Concerning downlisting or delisting information for invertebrates, five species have been downlisted, whereas one species has been uplisted from threatened to endangered status. The majority of species that have been delisted have gone extinct (n = 9). Notably, all species that went extinct were clams. One species, Inflectarius magazinensis, a snail endemic to Arkansas, has recovered since its initial listing in 1989 (United States Fish and Wildlife Service, 1989). Finally, five additional species have been delisted since subsequent information or taxonomic revisions have brought the validity of the taxon into question.
The prevalence of threats varied by aquatic and terrestrial domains (Figures 2a, b). For aquatic invertebrates, the most commonly indicated threats included pollution, modification to natural systems (e.g., modified hydrology, particularly in the form of dams), and intrinsic factors (e.g., small population size) (Figure 2a). Biological resource use and direct human intrusion were less commonly reported for aquatic (encompassing freshwater and marine) invertebrates. In comparison, terrestrial invertebrates are more threatened by problematic biotic factors (e.g., invasive species), climate change, and residential and commercial development projects (Figure 2b). Terrestrial invertebrates are less likely to be threatened by energy production/mining, transportation corridors, and biological resource use. Across aquatic and terrestrial domains, several threat prevalences are correlated. For example, Residential/commercial development was strongly correlated with climate change (r = 1) (Figure 2c). Other strong, positive correlations included human intrusion with biological resource use (r = 0.52) and problematic biotic factors with residential/commercial development (r = 0.39). Negatively correlated threats included natural systems modification and human intrusion (r = -0.58) and natural systems modification with biological resource use (r = -0.5) (Figure 2c).
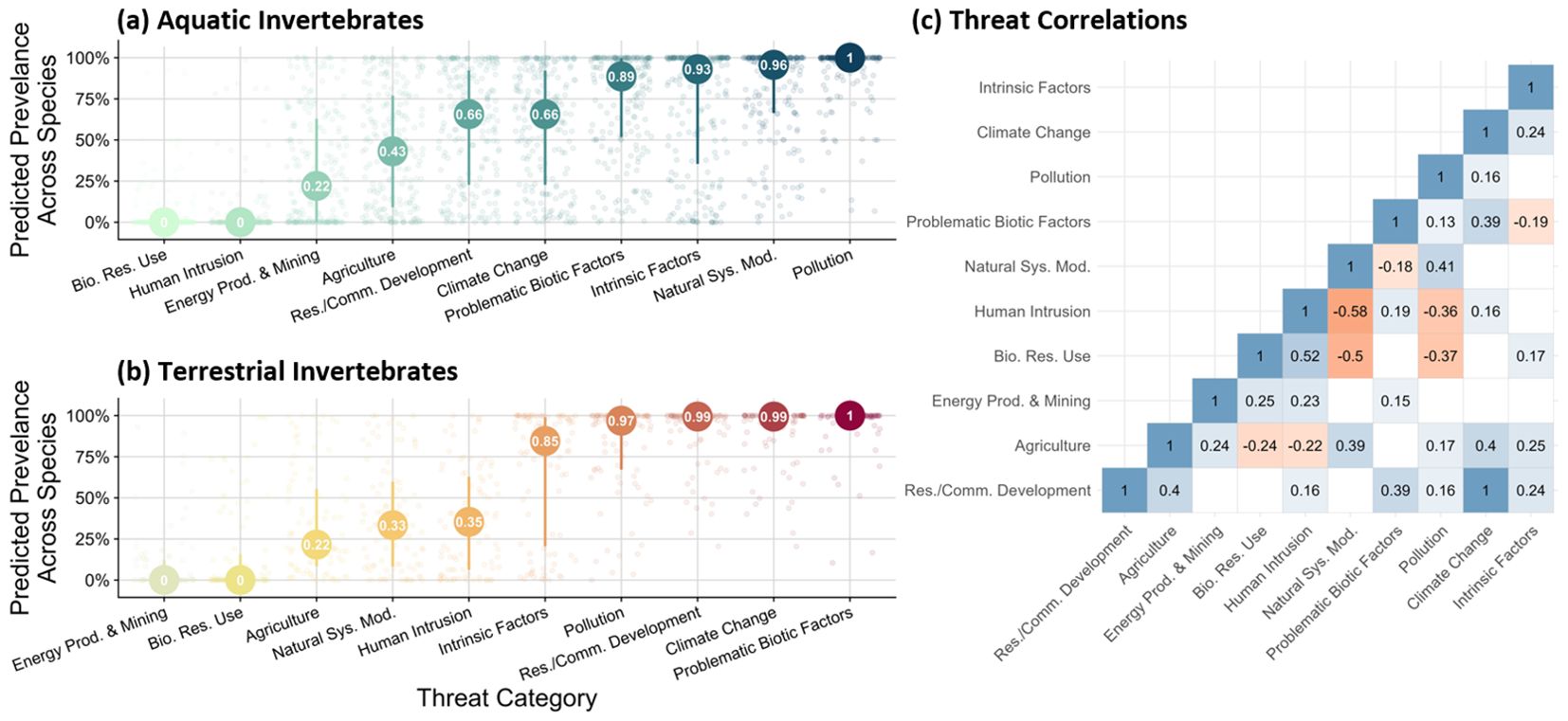
Figure 2. Summarized rankings of threats to conserving (a) aquatic and (b) terrestrial invertebrates across North America. Results were derived from manual extraction and automated processing of USFWS documentation using ChatGPT4-Turbo and tailored naive Bayes classifier models. Panel (c) indicates correlations between threats across aquatic and terrestrial domains. Only statistically significant correlations are visualized. Categories are non-exclusive.
Mapping these identified threats also revealed several regions of notable concern, either because of multiple overlapping endangered and threatened species or because of a greater diversity of potential threats to invertebrate biodiversity. Across aquatic invertebrates, the highest diversity of at-risk species and threat categories was prominently located in the southeastern part of the continental United States (Figure 3a). Additional hotspots of either at-risk species or threat types include California, Hawai‘i, and the Midwest. The geographic distribution of individual threats to endangered aquatic invertebrates appears to be mainly concentrated in the southeast, with mining and energy production slightly more dispersed into Appalachia (Supplementary Figure S4). Similarly, the geographic distribution of threats to endangered terrestrial invertebrates appears to be mainly focused in California, with additional prominence in the Midwest, Great Plains, and Hawai‘i (Figure 3b). Individual threats for terrestrial invertebrates are more geographically diverse, with large concentrations in the Midwest and specific threats such as energy production, mining, and biological resource use concentrated in the Great Plains states (Supplementary Figure S5). Overall, distributional knowledge for invertebrates listed under the ESA remains a challenge as the majority of species had fewer than 1,000 public occurrence records on GBIF (Supplementary Figure S6).
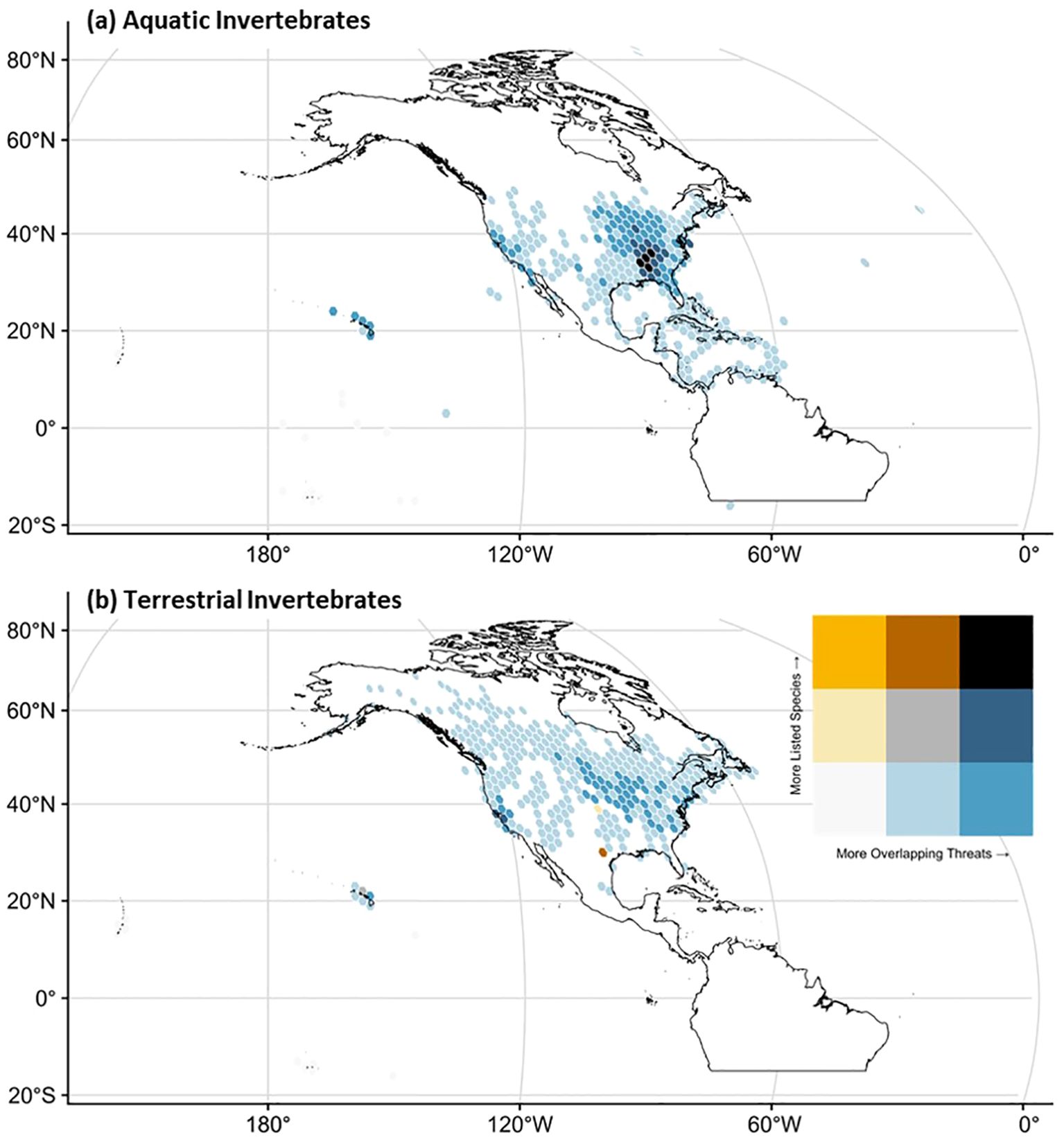
Figure 3. The geographic distribution of listed species and their identified threats to invertebrates split by (a) aquatic and (b) terrestrial domains. These maps represent an aggregation of threats for which GBIF occurrence data are also available. They, therefore, reflect a subset of listed invertebrates (n = 296) and may contain errors of omission (i.e., undersampled regions or missed detections). The spatial resolution is 2-degree hex cells with a Robinson map projection.
4 Discussion
4.1 Summary of ESA documentation and listing histories
The United States Endangered Species Act was a novel legislative action signed into law in 1973. Unlike previous iterations of biodiversity law in the United States, it explicitly protected invertebrates in its inception. The first invertebrates were listed under the Act only a short time after becoming law. While new listings have slowed in recent years, the focus of labor under the ESA has shifted towards conducting species status assessments and five-year reviews (Figure 1). This distinct shift in 2016 is noted for both vertebrates and invertebrates and may reflect changing attitudes around endangered species policy and the need to develop and adhere to the Act’s mandate to utilize “best available science” when making listing decisions (Choi et al., 2024). Additionally, the rigor of SSAs may contribute to fewer species meeting the qualifications of listing, reducing the number of listed species in recent years. Of notable recency are the listings of the Rusty Patched Bumble Bee (Bombus affinis) in 2017 (United States Fish and Wildlife Service, 2017) and Elkhorn corals (Acropora palmata and other Acropora spp.) in 2006, with additional congenerics listed in 2014.
4.2 Threats and status of aquatic invertebrates
The most prevalent threats for aquatic invertebrates were pollution, natural system modifications, intrinsic factors, and problematic biotic factors (Figure 2a). Pollution was most commonly cited and referenced. Both chemical and physical pollution in the form of chemical run-off and sedimentation were cited as problematic for many aquatic invertebrates. Still, other documents cited small populations or restricted connectivity between populations as limiting or intrinsic factors that increased the risk of extinction. Natural systems modifications most often refer to altered hydrological regimes across the extent of the designated critical habitat for a given species. Finally, problematic biotic factors, including invasive species, were also commonly mentioned. Examples include many freshwater bivalves that compete with introduced species such as zebra mussels (Dreissena polymorpha) (Strayer, 2009). The rusty crayfish (Orconectes rusticus) was also commonly listed as a problematic biotic factor, especially for endemic crayfish species (Wilson et al., 2004; Olden et al., 2006).
Both chemical and physical pollution (i.e., sedimentation) were commonly indicated as a top threat for aquatic invertebrates. In freshwater ecosystems, downstream impacts of run-off from mining, farming, and street treatments were frequently mentioned. For example, the big sandy crayfish (Cambarus callainus) and Guyandotta river crayfish (C. veteranus) are currently threatened by mining, construction, and non-point source pollution issues in central Appalachia (United States Fish and Wildlife Service, 2020a).
Additional modifications to their natural environment and intrinsic factors, most often involving changes to stream flow or other hydrological characteristics of the aquatic ecosystem, also threaten aquatic invertebrates. These threats can be especially prevalent for sessile organisms like most bivalves, including exemplars such as the western and Ouachita fanshell (Cyprogenia aberti and C. cf. aberti). For these species, altered streamflow characteristics, which have since led to the fragmentation of populations, were indicated as a primary threat to continued survival (United States Fish and Wildlife Service, 2020c).
Species that inhabit wetlands may be at increased risk of extinction (Gibbs, 1993; Kingsford et al., 2016). For example, the Hine’s Emerald dragonfly (Somatochlora hineana) spends its immature nymphal stage in wetlands, emerging as an adult after several years (Pulfer et al., 2013). In its larval habitat, changes to the groundwater hydrology of the wetlands is indicated as a primary threat (United States Fish and Wildlife Service, 2001). These hydrological modifications are felt through multiple potential channels including development for agriculture or commercial property (United States Fish and Wildlife Service, 2001). Further threats to the species may be additive as, once it emerges as an adult insect, the Hine’s Emerald may also be susceptible to threats indicated for terrestrial invertebrates such as pesticide use (United States Department of Agricultu…). The estimated cost of recovery of this particular wetland species is $13,163,000 USD, likely due to the sensitivity of restoring wetland conditions given the pace of development and requirements to establish viable habitat (United States Fish and Wildlife Service, 2001; Gibbs, 2000).
Finally, while most aquatic invertebrates listed under the ESA are found in freshwater ecosystems (about 95.8%), fewer species have been listed under the ESA in marine and brackish ecosystems. As the managing agency responsible for the stewardship of marine habitat, the National Ocean and Atmospheric Administration (NOAA) oversees the review and listing process for marine species rather than USFWS. Recent additions include several coral species and species endemic to anchialine pools in Hawai‘i, such as anchialine pool shrimp (Procaris hawaiana). NOAA continues to actively petition species for listing, and in 2024, recommended that six new species of marine giant clams be considered for listing under the ESA (National Ocean and Atmospheric Admini…).
Given these threats to aquatic invertebrates, the continued monitoring of threatened and non-threatened aquatic invertebrates is likely to occur nationwide. The United States government sponsors several national monitoring programs for aquatic invertebrates, including those coordinated through the United States Geological Survey (USGS), such as the National Water-Quality Assessment Program (NAWQA) and Nonindigenous Aquatic Species Program (NAS). In recent years, monitoring aquatic macroinvertebrate communities through environmental DNA (eDNA) has also increased (Dougherty et al., 2016). These less field-intensive approaches may increase the geographic reach of federally sponsored monitoring programs; however, there are still practical limitations to rolling these methods out as a replacement for traditional surveys (Rishan et al., 2023; Beng and Corlett, 2020). For example, eDNA can require substantial effort to develop appropriate reference libraries (Beng and Corlett, 2020). Phenology may impact the ability to robustly detect target species in aquatic habitat using eDNA approaches if aquatic ecosystems are not sampled throughout the year (Reinholdt Jensen et al., 2021; Sander et al., 2024) In the marine realm, research into the continued persistence of invertebrate species is ongoing, especially in coral resilience and husbandry (Hancock et al., 2021; Matsuda et al., 2022). This research and emerging approaches, combined with ongoing stream restoration projects for freshwater invertebrates (Kroll et al., 2019; Bogan et al., 2024), can provide a pathway for supporting healthy aquatic ecosystems and biodiversity well into the future.
4.3 Threats and status of terrestrial invertebrates
Many of the most prevalent threats to terrestrial invertebrates overlapped with those of aquatic invertebrates (pollution, problematic biotic factors, intrinsic factors), though residential and commercial development, as well as climate change, were considered much greater threats to terrestrial species than aquatic species. These largely reflect expert opinion on the top threats to insects (Miličić et al., 2021), though interestingly, the relative threat of agriculture was much lower in terrestrial invertebrate species-at-risk compared to the perception of its importance in the general decline of insects by experts. This could be because most federally listed insects are relatively range-restricted (Bossart and Carlton, 2002); these narrow and often highly specific habitat associations may limit the impact of agricultural expansion on listed species compared to the average invertebrate species. Both expert opinion and our study supported pollution as a primary threat, mainly via pesticide and herbicide application, which either had direct adverse effects on the species or indirect impacts through host plants. Pesticide application has previously been linked to widespread invertebrate declines (Ewald et al., 2015) and especially pollinators (Woodcock et al., 2016; Guzman et al., 2024; Van Deynze et al., 2024). Even if species-at-risk do not occupy or forage in agricultural landscapes, they may still be exposed: many listing documents discussed concerns over pesticide drift from agricultural run-off and aerial spraying on the species of conservation concern.
One notable exception to the relatively range-restricted species mentioned above, is the Rusty Patched Bumble Bee (Bombus affinis). Rusty Patched was federally listed as endangered in 2017 (United States Fish and Wildlife Service, 2017) due to its dramatic decline of relative abundance of 95% across its range (Hatfield et al., 2014; Colla et al., 2012; Cameron et al., 2011), and distributional declines of up to 87%. Historically, Rusty Patched Bumble Bee was commonly found across eastern United States, the midwest, and southern Quebec and Ontario, in Canada. The Rusty Patched Bumble Bee is listed as endangered in Canada as well (COSEWIC, 2010). Across both Canada and the US, the main threats for this species include pathogens or disease, primarily from Nosema bombi (Cameron et al., 2011), as well as exposure to pesticides, including both insecticides and fungicides. At the same time that Rusty Patched started declining (1990s) was the introduction of new classes of insecticides, neonicotinoids, which are commonly used in agriculture, and have been heavily implicated as a contributing cause of the Rusty Patched decline (Goulson et al., 2015).
Similarly to aquatic invertebrates, invasive species were identified as a top problematic biotic factor. Consistent with previous work, non-native social insects such as the red imported fire ant (Solenopsis invicta) and the Argentine ant (Linepithema humile) were identified as potent threats to species-at-risk because of their tendency to directly compete with and/or prey upon other arthropods (Wagner and Van Driesche, 2010). Two additional categories of problematic species were identified. First, invasive plants that degrade habitat outcompete native plants and reduce host plant and nectar plant availability in groups such as pollinators. These included grass species like smooth brome (Bromus inermis) and Kentucky bluegrass (Poa pratensis), and noxious weeds like knapweed (Centaurea spp.), perennial pepperweed (Lepidium latifolium) and iceplant (Carpobrotus chilensis) which can form dense infestations that directly reduce habitat or alter foraging behaviors in ground arthropods. Second, forest insect infestations (such as from the spongy moth, Lymantria dispar) can defoliate trees, and documents have noted the corresponding reduction in habitat for species such as land snails. Large-scale herbivore infestations can also result in the spraying of aerial pesticides, negatively affecting Lepidoptera species of conservation concern (Wagner and Van Driesche, 2010).
In comparison to better documented declines in insects, non-marine mollusks have dominated animal species extinctions, accounting for over 40 percent of all animal extinctions since the 1500’s (Régnier et al., 2009; Lydeard et al., 2004), and represent roughly 50% of listed invertebrate species. Kāhuli, or native Hawaiian land snails, are a uniquely diverse group containing over 700 known species. However, up to 90 percent of this number has already gone extinct (Régnier et al., 2015), and many remaining species exist only in captivity. Currently, 41 species, including an entire endemic genus Achatinella, are listed under the ESA. Despite these dire circumstances, the kāhuli are a bright spot in invertebrate conservation. The state’s Division of Forestry and Wildlife has dedicated a staffed and funded program to preventing their extinction through captive rearing, multiple releases into the wild, and habitat conservation—the Snail Extinction Prevention Program. The governor of Hawai‘i, declared 2023 as “The Year of the Kāhuli,” and there have been efforts across conservation partners to promote their cultural and biological importance to the Hawaiian Islands (Hawaii Department of Land and Natural…; Galka, 2022).
4.4 Evaluation and future
Evaluation of the ESA is contingent on subjective metrics of success versus failure and complicated by an absence of counterfactuals. Very few listed invertebrate species have gone extinct; similarly, few have been downlisted or removed from listing altogether. To the Act’s promise to prevent extinctions, most listed species have not gone extinct. The length of time since a species was listed was found to be correlated with improving population trends, suggesting that the benefits of the ESA accumulate through time (Taylor et al., 2005), and species with identified critical habitat and recovery plans were significantly more likely to be improving than those without. Additionally, by comparing the expected extinction rates of ESA-listed species to observed rates, it is estimated that the ESA has prevented the extinction of almost 300 species since its passing (Greenwald et al., 2019). In this regard, the Act has been successful. However, concerns abound: species wait an average of 12.1 years to receive that much-needed protection (with invertebrates experiencing longer wait times than average) (Puckett et al., 2016); by the time they do receive that protection, they have reached dangerously low population sizes, which increases their risk of extinction (Eberhard et al., 2022). Almost a quarter of listed species lack final recovery plans, and the majority of recovery plans that do exist are over 20 years old (Malcom and Li, 2018). While the level of government funding towards species recovery does positively affect recovery outcomes, funding levels have not kept pace with the number of species listed, meaning that USFWS is now allocating less on a per-species basis than in the past (Eberhard et al., 2022).
Recently, several proposed pieces of legislation from the United States Congress (Pfluger, 2023; Westerman, 2024) and Supreme Court decisions (Loper Bright Enterprises et al. v. Raimondo, Secretary of Commerce, et al., 2024) have drawn into question the ESA’s future reach and implementation. In addition to these challenges/proposed changes to the Act, several controversial invertebrate species have recently been petitioned for listing, including the monarch butterfly (Danaus plexippus). The monarch was identified as a candidate for listing in 2020 but was ultimately superseded by other priority species (United States Fish and Wildlife Service, 2020b; Shirey and Ries, 2023). Because of its wide distribution and obligate host plant (Asclepias spp.), considered an agricultural weed that reduces yield (Pleasants and Oberhauser, 2013), listing the Monarch and designating critical habitat could necessitate sweeping changes to herbicide and pesticide use in American agricultural practices. As of writing, the Monarch has officially been petitioned for threatened status. Whether the Act is equipped to pursue these changes and deal with the potential fall-out is unclear; however, USFWS already provides a breadth of resources related to this specific case (United States Fish and Wildlife Service, 2024b).
It is worth noting, that, in light of these potential changes or challenges to the ESA, state and local biodiversity regulation has also proven successful as a tool for conserving invertebrate biodiversity. As explored previously, state-level managements plans for the Karner blue butterfly, particularly in Wisconsin, have helped recover population levels (Hess and Hess, 2015). Good news also rings true for the American burying beetle (Nicrophorus americanus), which, thanks to coordinating private restoration efforts, has seen increasing population sizes at the ecoregion-scale (Roberts et al., 2025). Even if not designated under the ESA, state wildlife action plans can specify species of greatest conservation need (SGCNs). For example, in Pennsylvania, several freshwater mussels are designated as SGCNs without co-listing under the ESA (Pennsylvania Game Commission, Pennsylvania Fish & Boat Commission, 2015). These outcomes and additional avenues for invertebrate conservation further evidence the fact that local collaborations can make an impact despite what may occur at the federal level.
The United States Endangered Species Act still has broad public support, with over 80% of Americans, on average, supporting it across political affiliations (Bruskotter et al., 2018). In summary of 50 years of the Act’s history, we find a consistent effort is made to assess, list, and review invertebrate species; however, compared to the diversity of invertebrates, there is likely a shortfall in conserving relative biodiversity compared to vertebrate taxa. Further, we find that identified threats to listed species vary by aquatic and terrestrial domains, with factors like pollution and small population sizes appearing as common risk factors across groups. Finally, these threats are uniquely geographically distributed, with more threats and taxa listed in the southeastern United States for aquatic organisms and elevated threats, as well as more taxa in the Midwest and Great Plains for terrestrial invertebrates.
The future of invertebrate conservation under federal law in the United States is subject to change. However, our review has clarified its history and identified threats to these unique, diverse groups of organisms. To this end, we strongly encourage using emerging scientific tools, such as eDNA and artificial intelligence, alongside consensus-building strategies to promote the conservation of invertebrate biodiversity. Of course, the successful adoption these technologies is entirely contingent on continued support of fundamental research into the systematics, taxonomy, and natural history of invertebrates. Which is particularly important for hyperdiverse groups, such as most invertebrates (Hopkins and Freckleton, 2002; Drew, 2011; Mcneely, 2002; Agnarsson and Kuntner, 2007). We strongly support the continued resourcing of these domains as well as new approaches so that the Endangered Species Act continues to provide the platform for us to action conservation and preserve our invertebrate natural heritage.
Data availability statement
The original contributions presented in the study are included in the article/Supplementary Material. Further inquiries can be directed to the corresponding author/s.
Author contributions
VS: Conceptualization, Data curation, Formal analysis, Investigation, Methodology, Resources, Software, Validation, Visualization, Writing – original draft, Writing – review & editing. JL: Conceptualization, Data curation, Investigation, Validation, Writing – original draft, Writing – review & editing. AG: Conceptualization, Data curation, Investigation, Validation, Writing – original draft, Writing – review & editing. LG: Conceptualization, Data curation, Formal analysis, Funding acquisition, Investigation, Methodology, Project administration, Resources, Software, Supervision, Validation, Visualization, Writing – original draft, Writing – review & editing.
Funding
The author(s) declare that financial support was received for the research and/or publication of this article. VS and AG were both supported by a David H. Smith Postdoctoral Conservation Research Fellowship. VS was further supported by start-up funding from the Florida Museum of Natural History—University of Florida. JL was supported by a Liber Ero Fellowship. LG was supported by the University of Southern California.
Acknowledgments
The authors thank Tom Sisk and Barry Noon for pitching the special issue topic and inviting us to contribute. Discussions with Rob Guralnick were insightful in producing some of the natural language processing solutions used within this work.
Conflict of interest
The authors declare that the research was conducted in the absence of any commercial or financial relationships that could be construed as a potential conflict of interest.
Generative AI statement
The author(s) declare that Generative AI was used in the creation of this manuscript.
We relied on using a large language model (OpenAI ChatGPT v4-turbo) for data collection but not the writing of this manuscript.
Publisher’s note
All claims expressed in this article are solely those of the authors and do not necessarily represent those of their affiliated organizations, or those of the publisher, the editors and the reviewers. Any product that may be evaluated in this article, or claim that may be made by its manufacturer, is not guaranteed or endorsed by the publisher.
Supplementary material
The Supplementary Material for this article can be found online at: https://www.frontiersin.org/articles/10.3389/fcosc.2025.1505451/full#supplementary-material
References
Agnarsson I., Kuntner M. (2007). Taxonomy in a changing world: Seeking solutions for a science in crisis. Systematic Biol. 56 (3), 531–539.
Andelman S. J., Groves C., Regan H. M. (2004). A review of protocols for selecting species at risk in the context of US Forest Service viability assessments. Acta Oecol. (Montrouge) 26, 75–83. doi: 10.1016/j.actao.2004.04.005
Beng K. C., Corlett R. T. (2020). Applications of environmental DNA (eDNA) in ecology and conservation: Opportunities, challenges and prospects. Biodiversity Conserv. 29 (7), 2089–21215.
Bogan M., Hamdhani H., Eppehimer D., Hollien K., Gill B. (2024). Macroinvertebrate community development and resilience to channel dredging following flow restoration using effluent in an urban desert river. Restor. Ecol. 33 (2). doi: 10.1111/rec.14250
Bossart J. L., Carlton C. E. (2002). Insect conservation in America: Status and perspectives. Am. Entomol. 48 (2), 82–92. doi: 10.1093/ae/48.2.82
Bried J., Ries L., Smith B., Patten M., Abbott J., Ball-Damerow J., et al. (2020). Towards global volunteer monitoring of odonate abundance. Bioscience 70 (10), 914–923.
Bried J., Tear T., Shirer R., Zimmerman C., Gifford N., Campbell S., et al. (2014). A framework to integrate habitat monitoring and restoration with endangered insect recovery. Environ. Manage. 54 (6), 1385–1985.
Bruskotter J. T., Vucetich J. A., Slagle K. M., Berardo R., Singh A. S., Wilson R. S. (2018). Support for the U.S. Endangered Species Act over time and space: Controversial species do not weaken public support for protective legislation. Conserv. Lett. 11 (6), e12595. doi: 10.1111/conl.12595
Cameron S. A., Lozier J. D., Strange J. P., Koch J. B., Cordes N., Solter L. F., et al. (2011). Patterns of widespread decline in north american bumble bees. Proc. Natl. Acad. Sci. United States America 108 (2), 662–675.
Cardoso P., Erwin T. L., Borges P. A. V., Tim R. N. (2011). The seven impediments in invertebrate conservation and how to overcome them. Biol. Conserv. 144 (11), 2647–2655. doi: 10.1016/j.biocon.2011.07.024
Chamberlain S., Arendsee Z., Stirling T., Boettiger C., James T. D., Salmon M., et al. (2023). ropensci/taxizedb: taxizedb v0.3.1 (Zenodo). doi: 10.5281/ZENODO.1158055
Chamberlain S., Boettiger C. (2017). R Python, and Ruby clients for GBIF species occurrence data. PeerJ PrePrints. e3304v1. doi: 10.7287/peerj.preprints.3304v1
Choi J. J., Gaskins L. C., Morton J. P., Bingham J. A., Blawas A. M., Hayes C., et al. (2024). Role of low-impact-factor journals in conservation implementation. Conserv. Biol., e14391. doi: 10.1111/cobi.14391
Colla S. R., Gadallah F., Richardson L., Wagner D., Gall L. (2012). Assessing declines of north american bumble bees (Bombus spp.) using museum specimens. Biodiversity Conserv. 21 (14), 3585–3955.
Didham R. K., Basset Y., Collins C. M., Leather S. R., Littlewood N. A., Menz M. H. M., et al. (2020). Interpreting insect declines: seven challenges and a way forward. Insect Conserv. Divers. 13 (2), 103–114. doi: 10.1111/icad.12408
Dougherty M. M., Larson E. R., Renshaw M. A., Gantz C. A., Egan S. P., Erickson D. M., et al. (2016). Environmental DNA (eDNA) detects the invasive rusty crayfish Orconectes rusticus at low abundances. J. Appl. Ecol. 53 (3), 722–732. doi: 10.1111/1365-2664.12621
Duda R. O., Hart P. E. (1973). Pattern classification and scene analysis. Available online at: https://scholar.google.com/citations?user=aUDICDsAAAAJ&hl=en&oi=sra (Accessed October 2024).
Eberhard E. K., Wilcove D. S., Dobson A. P. (2022). Too few, too late: U.S. Endangered Species Act undermined by inaction and inadequate funding. PloS One 17 (10), e027532. doi: 10.1371/journal.pone.0275322
Ewald J. A., Wheatley C. J., Aebischer N. J., Moreby S. J., Duffield S. J., Crick H. Q. P., et al. (2015). Influences of extreme weather, climate and pesticide use on invertebrates in cereal fields over 42 years. Glob. Change Biol. 21 (10), 3931–3950. doi: 10.1111/gcb.13026
Forister M. L., Halsch C. A., Nice C. C., Fordyce J. A., Dilts T. E., Oliver J. C., et al. (2021). Fewer butterflies seen by community scientists across the warming and drying landscapes of the American West. Science 371 (6533), 1042–1045. doi: 10.1126/science.abe5585
Galka J. (2022). Mollusk loves: Becoming with native and introduced land snails in the hawaiian islands. Island Stud. J. 17 (1), 102–122.
Gerber L. R. (2016). Conservation triage or injurious neglect in endangered species recovery. Proc. Natl. Acad. Sci. United States America 113 (13), 3563–3566.
Gibbs J. P. (1993). Importance of small wetlands for the persistence of local populations of wetland-associated animals. Wetlands (Wilmington N.C.) 13 (1), 25–31.
Gibbs J. P. (2000). Wetland loss and biodiversity conservation. Conserv. Biology: J. Soc. Conserv. Biol. 14 (1), 314–317.
Global Biodiversity Information Facility (2024a). GBIF Occurrence Data Download for ESA Invertebrates. (GBIF). doi: 10.15468/dl.bdxex5
Global Biodiversity Information Facility (2024b). GBIF Occurrence Data Download for North American Invertebrate Species. (GBIF). doi: 10.15468/dl.jwzku8
Global Biodiversity Information Facility (2024c). GBIF Occurrence Data Download for North American Vertebrate Species List. (GBIF). doi: 10.15468/dl.fdsssu
Graves T. A., Janousek W. M., Gaulke S. M., Nicholas A. C., Keinath D. A., Bell C. M., et al. (2020). Western bumble bee: declines in the continental United States and range-wide information gaps. Ecosphere 11 (6). doi: 10.1002/ecs2.3141
Greenwald N., Suckling K. F., Hartl B., Mehrhoff L. A. (2019). Extinction and the U.s. endangered species act. PeerJ 7. doi: 10.7717/peerj.6803
Guzman L. M., Elle E., Morandin L. A., Cobb N. S., Chesshire P. R., McCabe L. M., et al. (2024). Impact of pesticide use on wild bee distributions across the United States. Nat. Sustain. 7 (10), 1–11. doi: 10.1038/s41893-024-01413-8
Haack R. (1993). The endangered Karner blue butterfly (Lepidoptera: Lycaenidae): biology, management considerations, and data gaps. Available online at: https://research.fs.usda.gov/treesearch/15307 (Accessed October 2024).
Hancock J. R., Barrows A. R., Roome T. C., Huffmyer A. S., Matsuda S. B., Munk N. J., et al. (2021). Coral husbandry for ocean futures: leveraging abiotic factors to increase survivorship, growth, and resilience in juvenile Montipora capitata. Mar. Ecol. Prog. Ser. 657, 123–133. doi: 10.3354/meps13534
Hatfield R., Colla S., Jepsen S., Richardson L., Thorp R., Jordan S. F. (2014). IUCN assessments for north american bombus spp.
Hess R. J., Hess A. N. (2015). Conserving Karner blue butterflies in Wisconsin: A development of management techniques. Am. Entomol. 61 (2), 96–113. doi: 10.1093/ae/tmv022
Hopkins G. W., Freckleton R. P. (2002). Declines in the numbers of amateur and professional taxonomists: Implications for conservation. Anim. Conserv. Forum. doi: 10.1017/S1367943002002299
Howedi F., Mohd M. (2014). Text classification for authorship attribution using Naive Bayes classifier with limited training data. Comput. Eng. intelligent Syst. 5 (4), 48–56. Available at: https://www.researchgate.net/profile/Fatma-Howedi-2/publication/347765350_Text_Classification_for_Authorship_Attribution_Using_Naive_Bayes_Classifier_with_Limited_Training_Data/links/5fe3a5cd299bf140883a3e7c/Text-Classification-for-Authorship-Attribution-Using-Naive-Bayes-Classifier-with-Limited-Training-Data.pdf (Accessed October 2024).
Kingsford R. T., Basset A., Jackson L. (2016). Wetlands: Conservation’s poor cousins: Wetland conservation. Aquat. Conservation: Mar. Freshw. Ecosyst. 26 (5), 892–9165.
Kroll S. A., Horwitz R. J., Keller D. H., Sweeney B. W., Jackson J. K., Perez L. B. (2019). Large-scale protection and restoration programs aimed at protecting stream ecosystem integrity: the role of science-based goal-setting, monitoring, and data management. Freshw. Sci. 38 (1), 23–39. doi: 10.1086/701756
Loper Bright Enterprises et al. v. Raimondo, Secretary of Commerce, et al. (2024). Available online at: https://www.supremecourt.gov/opinions/23pdf/22-451_7m58.pdf (Accessed October 2024).
Lydeard C., Cowie R. H., Ponder W. F., Bogan A. E., Bouchet P., Clark S. A., et al. (2004). The global decline of nonmarine mollusks. Bioscience 54 (4), 321.
Lyons T. (2022). The karner blue butterfly: A new hampshire icon (Concord, New Hampshire: New Hampshire State Parks). Available at: https://blog.nhstateparks.org/the-karner-blue-butterfly-a-new-hampshire-icon/ (Accessed October 2024).
Malcom J. W., Li Y.-W. (2018). Missing, delayed, and old: The status of ESA recovery plans. Conserv. Lett. 11 (6), e12601. doi: 10.1111/conl.12601
Mammola S., Nanni V., Pantini P., Isaia M. (2020). Media framing of spiders may exacerbate arachnophobic sentiments. People Nat. (Hoboken) 2 (4), 1145–1157. doi: 10.1002/pan3.10143
Matsuda S. B., Chakravarti L. J., Cunning R., Huffmyer A. S., Nelson C. E., Gates R. D., et al. (2022). Temperature-mediated acquisition of rare heterologous symbionts promotes survival of coral larvae under ocean warming. Glob. Change Biol. 28 (6), 2006–2025. doi: 10.1111/gcb.16057
Miličić M., Popov S., Branco V. V., Cardoso P. (2021). Insect threats and conservation through the lens of global experts. Conserv. Lett. 14. doi: 10.1111/conl.12814
Monfils M. J., Rowe L. M., Cole-Wick A. A., Cuthrell D. L. (2021). Monitoring butterfly species of greatest conservation need in Michigan grasslands and savannas. (Michigan Department of Natural Resources, Wildlife Division in Lansing, Michigan).
Neel M. C., Leidner A. K., Haines A., Goble D. D., Scott J.M. (2012). By the numbers: How is recovery defined by the US endangered species act?”. Bioscience 62 (7), 646–575.
New T. R. (2012). Insect conservation: Past, present and prospects. 2012th Edn (Dordrecht, Netherlands: Springer). Available at: https://books.google.com/books?hl=en&lr=&id=UE-0gBVJGzUC&oi=fnd&pg=PR5&dq=Insect+Conservation:+Past,+Present+and+Prospects&ots=yHs8Xi-Dad&sig=DIXeHqC_fPDncDfViK_YUMmYTwc (Accessed October 2024).
New Hampshire Fish and Game Department (2024). Karner blue butterfly and concord (NH) pine barrens project. Available online at: https://www.wildlife.nh.gov/wildlife-and-habitat/nongame-and-endangered-species/nongame-projects/karner-blue-butterfly-and (Accessed October 2024).
Olden J. D., McCarthy J. M., Maxted J. T., Fetzer W. W., Vander Zanden M. J. (2006). The rapid spread of rusty crayfish (Orconectes rusticus) with observations on native crayfish declines in Wisconsin (U.S.A.) over the past 130 years. Biol. Invasions 8 (8), 1621–1628. doi: 10.1007/s10530-005-7854-2
Oliveira-Junior J. M., Rocha T., Vinagre S. F., Miranda-Filho J. C., Mendoza-Penagos C., Dias-Silva K., et al. (2022). A bibliometric analysis of the global research in odonata: Trends and gaps. Diversity. doi: 10.3390/d14121074
Patterson T. A., Grundel R., Dzurisin J. D. K., Knutson R. L., Hellmann J. J. (2020). Evidence of an extreme weather-induced phenological mismatch and a local extirpation of the endangered Karner blue butterfly. Conserv. Sci. Pract. 2. doi: 10.1111/csp2.147
Pedregosa F., Varoquaux G., Gramfort A., Michel V., Thirion B., Grisel O., et al. (2011). Scikit-learn: Machine learning in Python. J. Mach. Learn. Res. 12, 2825–2830. Available at: https://www.jmlr.org/papers/volume12/pedregosa11a/pedregosa11a.pdf?ref=https:/ (Accessed October 2024).
Pennsylvania Game Commission, Pennsylvania Fish & Boat Commission (2015). Pennsylvania Wildlife Action Plan, (2015-2025). (PA Game and PA Fish and Boat Commission, Harrisburg, Pennsylvania).
Pfluger A. (2023). To amend the Endangered Species Act of 1973 to require consideration of economic impact in making a listing decision with respect to the list of threatened and endangered spceies, and for other purposes. (Proposed legislation, United States Congress in Washington, DC, Bill is H.R.1142, 118th Congress).
Pleasants J. M., Oberhauser K. S. (2013). Milkweed loss in agricultural fields because of herbicide use: effect on the monarch butterfly population. Insect Conserv. Diversity 6 (2), 135–144. doi: 10.1111/j.1752-4598.2012.00196.x
Puckett E. E., Kesler D. C., Greenwald D. N. (2016). Taxa, petitioning agency, and lawsuits affect time spent awaiting listing under the US Endangered Species Act. Biol. Conserv. 201, 220–229. doi: 10.1016/j.biocon.2016.07.005
Pulfer T. L., Evans C. G., Featherstone D., Post R., McCarter J. I., Laverty J. F. (2013). “Not knowing, not recording, not listing: numerous unnoticed mollusk extinctions.” Conserv. Biology: J. Soc. Conserv. Biol. 23 (5), 1214–1221.
Régnier C., Benoît ., Philippe B. (2009). Extinction in a hyperdiverse endemic hawaiian land snail family and implications for the underestimation of invertebrate extinction: Underestimating invertebrate extinction. Conserv. Biology: J. Soc. Conserv. Biol. 23 (5), 1214–122.
Régnier Claire, Bouchet P., Hayes K. A., Yeung N. W., Christensen C. C., Chung D. J. D., et al. (2015). Extinction in a hyperdiverse endemic hawaiian land snail family and implications for the underestimation of invertebrate extinction: Underestimating invertebrate extinction. Conserv. Biology: J. Soc. Conserv. Biol. 29 (6), 1715–1235.
Reinholdt Jensen M., Sigsgaard E. E., Agersnap S., Rasmussen J. J., Baattrup-Pedersen A., Wiberg-Larsen P., et al. (2021). Seasonal turnover in community composition of stream-associated macroinvertebrates inferred from freshwater environmental DNA metabarcoding. Environ. DNA (Hoboken N.J.) 3 (4), 861–765.
Richardson L. (2007). Beautiful soup documentation. April. Available online at: https://tedboy.github.io/bs4_doc/.
Rishan S. T., Kline R. J., Rahman Md S. (2023). Applications of environmental DNA (eDNA) to detect subterranean and aquatic invasive species: A critical review on the challenges and limitations of eDNA metabarcoding. Environ. Adv. 12 (100370), 1003705.
Roberts C. P., Ludwig A. K., Fogarty D. T., Stuber E. F., Uden D. R., Walker T. L. Jr, et al. (2025). Population increases of the threatened american burying beetle (Nicrophorus americanus) linked to large-scale collaborations in a working lands ecoregion. Biol. Conserv. 301 (110865), 1108655.
Salafsky N., Salzer D., Stattersfield A. J., Hilton-Taylor C., Neugarten R., Butchart S. H. M., et al. (2008). A standard lexicon for biodiversity conservation: unified classifications of threats and actions: Classifications of threats & actions. Conserv. Biol. 22 (4), 897–911. doi: 10.1111/j.1523-1739.2008.00937.x
Salvador R. B., Tomotani B. M., O’Donnell K. L., Cavallari D. C., Tomotani J. V., Salmon R. A., et al. (2021). Invertebrates in science communication: Confronting scientists’ practices and the public’s expectations. Front. Environ. Sci. Eng. China 9. doi: 10.3389/fenvs.2021.606416
Sander M., Beermann A. J., Buchner D., Pimentel I. M., Sinclair J. S., Weiss M., et al. (2024). Environmental DNA time series analysis of a temperate stream reveals distinct seasonal community and functional shifts. River Res. Appl. 40 (5), 850–625.
Scott J. M., Goble D. D., Wiens J. A., Wilcove D. S., Bean M., Male T. (2005). Recovery of imperiled species under the endangered species act: The need for a new approach. Front. Ecol. Environ. 3 (7), 383–895.
Shirey V., Ries L. (2023).Population dynamics and drivers of the eastern monarch (Danaus plexippus) across its full annual cycle: a cross-scale synthesis of a model migratory species. Available online at: https://scholar.google.com/citations?view_op=view_citation&hl=en&citation_for_view=ssaexXQAAAAJ:Wp0gIr-vW9MC (Accessed October 2024).
Smith D. R., Allan N. L., McGowan C. P., Szymanski J. A., Oetker S. R., Bell H. M. (2018). Development of a species status assessment process for decisions under the U.s. endangered Species Act. J. Fish Wildl. Manage. 9 (1), 302–320. doi: 10.3996/052017-jfwm-041
Strayer D. L. (2006). Challenges for freshwater invertebrate conservation. J. North Am. Benthological Soc. 25, 271–287. doi: 10.1899/0887-3593(2006)25[271:CFFIC]2.0.CO;2
Strayer D. L. (2009). Twenty years of zebra mussels: lessons from the mollusk that made headlines. Front. Ecol. Environ. 7 (2), 135–141. doi: 10.1890/080020
Swengel A. B., Swengel S. R. (2018). Patterns of long-term population trends of three lupine-feeding butterflies in Wisconsin. Diversity (Basel) 10 (2), 31. doi: 10.3390/d10020031
Taha A. A., Hanbury A. (2015). Metrics for evaluating 3D medical image segmentation: analysis, selection, and tool. BMC Med. Imaging 15 (1), 29. doi: 10.1186/s12880-015-0068-x
Taylor M. F. J., Suckling K. F., Rachlinski J. (2005). The effectiveness of the Endangered Species Act: A quantitative analysis. BioScience 55, 360–367. doi: 10.1641/0006-3568(2005)055[0360:TEOTES]2.0.CO;2
Tear T. H., Scott J. M., Hayward P. H., Griffith B. (1993). Status and prospects for success of the endangered species act: A look at recovery plans. Sci. (New York N.Y.) 262 (5136), 976–977.
United States Fish and Wildlife Service (1989). ETWP; Determination of Threatened Status for the Magazine Mountain Shagreen Mesodon magazinensis; 54 FR 15206 15208 (Washington, District of Columbia: United States Department of Interior).
United States Fish and Wildlife Service (2001). “Hine’s emerald dragonfly (Somatochlora Hineana) recovery plan.” In Fort Snelling, MN. Available online at: https://ecos.fws.gov/docs/recovery_plan/hedplan.pdf.
United States Fish and Wildlife Service (2017). Endangered Species Status for Rusty Patched Bumble Bee; Final Rule (Washington, District of Columbia: United State Department of Interior).
United States Fish and Wildlife Service (2020a). Endangered and Threatened Wildlife and Plants; Designation of Critical Habitat for the Big Sandy Crayfish and Guyandotte River Crayfish (Washington, District of Columbia: United States Department of the Interior).
United States Fish and Wildlife Service (2020b). Monarch (Danaus plexippus) Species Status Assessment Report, Version 2.1 (Washington, District of Columbia: United States Department of Interior).
United States Fish and Wildlife Service (2020c). Species Status Assessment Report for Western Fanshell (Cyprogenia alberti) and “Ouachita” Fanshell (Cyprogenia cf. alberti) (Washington, District of Columbia: United States Department of the Interior).
United States Fish and Wildlife Service (2024a). ECOS: environmental conservation online system. Available online at: https://ecos.fws.gov/ecp0/reports/ad-hoc-species-report (Accessed September 30, 2024).
United States Fish and Wildlife Service (2024b). Monarchs. Available online at: https://www.fws.gov/initiative/pollinators/monarchs (Accessed October 1, 2024).
U.S. Fish & Wildlife Service (2016). Species status assessment framework: an integrated framework for conservation fact sheet. Available online at: https://www.fws.gov/sites/default/files/documents/species-status-assessment-fact-sheet-2016.pdf (Accessed October 2024).
Van Deynze B., Swinton S. M., Hennessy D. A., Haddad N. M., Ries L. (2024). Insecticides, more than herbicides, land use, and climate, are associated with declines in butterfly species richness and abundance in the American Midwest. PloS One 19 (6), e0304319. doi: 10.1371/journal.pone.0304319
Wagner D. L., Van Driesche R. G. (2010). Threats posed to rare or endangered insects by invasions of nonnative species. Annu. Rev. Entomol. 55 (1), 547–568. doi: 10.1146/annurev-ento-112408-085516
Westerman B. (2024). ESA Amendments Act of 2024. (Proposed legislation, United States Congress in Washington, DC, Bill is H.R.9533, 118th Congress)
Wheatley M. (2022). Karner blue butterfly recovery in New Hampshire (United States Fish and Wildlife Service). Available at: https://www.fws.gov/story/karner-blue-butterfly-recovery-new-hampshire (Accessed October 2024).
Williams U. S. S. H. (1973). Endangered Species Act of 1973. (Signed legislation, United States Congress in Washington, DC, S1983 in the 93rd Congress).
Wilson K. A., Magnuson J. J., Lodge D. M., Hill A. M., Kratz T. K., Perry W. L., et al. (2004). A long-term rusty crayfish (Orconectes rusticus) invasion: dispersal patterns and community change in a north temperate lake. Can. J. Fish. Aquat. Sci. 61 (11), 2255–2266. doi: 10.1139/f04-170
Keywords: endangered species act, conservation, insects, invertebrates, mollusks
Citation: Shirey VM, Lewthwaite JMM, Gawel AM and Guzman LM (2025) 50 years of invertebrate conservation under the United States Endangered Species Act—history and threats to species. Front. Conserv. Sci. 6:1505451. doi: 10.3389/fcosc.2025.1505451
Received: 02 October 2024; Accepted: 28 February 2025;
Published: 01 April 2025.
Edited by:
Thomas D. Sisk, Northern Arizona University, United StatesReviewed by:
Ed DeWalt, University of Illinois at Urbana-Champaign, United StatesJason Bried, University of Illinois at Urbana-Champaign, United States
Copyright © 2025 Shirey, Lewthwaite, Gawel and Guzman. This is an open-access article distributed under the terms of the Creative Commons Attribution License (CC BY). The use, distribution or reproduction in other forums is permitted, provided the original author(s) and the copyright owner(s) are credited and that the original publication in this journal is cited, in accordance with accepted academic practice. No use, distribution or reproduction is permitted which does not comply with these terms.
*Correspondence: Vaughn M. Shirey, dm1zaGlyZXlAZ21haWwuY29t
†Present address: Laura Melissa Guzman, Department of Entomology, Cornell University, Ithaca, Ithaca, NY, United States