- Conservation Ecology Program, King Mongkut’s University of Technology Thonburi, Bangkok, Thailand
Droughts are expected to become more frequent and severe as the climate changes. Tropical songbirds likely reduce reproductive output during extreme droughts such as El Niño events in Southeast Asia as a tradeoff for reducing mortality risks. However, the timing of these rainfall responses and the extent of these reproductive costs are largely unknown in this region. Here we quantified how the amount and frequency of rainfall impacted nest initiation (first egg dates), and how the amount of rainfall may impact the duration of nesting in a given season (last expected fledging date). We tested these hypotheses on seven species of understory passerines in a secondary dry evergreen forest in northeastern Thailand. Using ten years of data (2014–2023) we examined the relationship between rainfall examining both total amounts occurring at key parts of the year (such as just prior to the breeding season) as well the number of “no-rain” days (rainfall gap) on (1) first egg dates and the (2) last expected fledging date of a given season. More severe dry seasons (longer time gaps in rainfall) caused longer delays in the start of egg laying and gap days tended to have a stronger effect than total rainfall. Lower breeding season rainfall resulted in later fledge dates for four species; lower rainfall amounts during the dry season resulted in later fledge dates for three species. There was no relationship between first egg dates and last expected fledging dates for any target species. Overall, it appears that droughts are likely shortening the breeding seasons of many tropical forest birds, but the reproductive costs are still difficult to estimate precisely. Further investigation is also recommended as to how rainfall is affecting nesting success particularly whether greater rainfall is regularly associated with earlier end of season fledging dates.
Introduction
Droughts are expected to become more severe and frequent as the climate changes (Trenberth et al., 2014; Fu, 2015). For many tropical species, variation in precipitation is used to time breeding (Skagen et al., 2018; Hidalgo Aranzamendi et al., 2019; Halali et al., 2021) and overall is a critically important parameter driving natural selection (Siepielski et al., 2017). It is therefore likely that changes in rainfall could have substantial impacts on seasonal fecundity. Although tropical songbirds likely reduce reproduction during extreme droughts (Brawn et al., 2017; Martin and Mouten, 2020), the few long-term studies available suggest that this behavioral flexibility is critical because it allows songbirds to buffer some of the mortality risks associated with breeding during extreme droughts (Martin and Mouten, 2020), such as El Niño events in Southeast Asia. Furthermore, even without El Niño events, Southeast Asian rainfall patterns are normally highly variable (Chang et al., 2005), and especially so in some seasonal evergreen forests of the region (Bunyavejchewin, 1999). In these forests, El Niño events represent one point on a continuum of rainfall, whereby reproductive effort in response to rainfall also likely lies on a continuum (Cruz‐McDonnell and Wolf, 2016), suggesting that understanding rainfall effects or thresholds will be important for modeling climate change impacts on tropical forest songbirds of this region. For example, if rainfall fails to reach some minimum volume and/or frequency, this may cause a majority of individuals of some species to forgo breeding in a given season (Martin and Mouten, 2020; Boersma et al., 2022), while for other species individuals may only pause breeding for a few weeks or even a few months (Grant et al., 2000).
Precipitation can serve as a reliable predictive cue of food availability, especially in insectivorous birds (Poulin et al., 1992; McKinnon et al., 2015; Hidalgo Aranzamendi et al., 2019). Thus, the demographic impacts of the timing, frequency and amount of rainfall may be dramatically different depending on the details, such as the microhabitat use of the bird species of interest and the forest type (Martin and Mouten, 2020). This may also imply that bird populations in forests which typically receive less rainfall may have less prey available and be particularly vulnerable if there are long-term reductions in rainfall compared to similar forests that typically receive more rainfall (Poulin et al., 1992). Furthermore, the impacts of El Niño events will also likely vary depending on when a species typically breeds, such that early to middle dry season nesters may face more disruption (have to pause reproduction longer) than those species normally nesting later in the dry season. It is also possible that if rains arrive later, that birds will complete the breeding season later, which may mitigate some of the impacts on seasonal female fecundity. The objective of this paper was to quantify how the amount and frequency of rainfall impacts nest initiation (first egg dates), how much first egg dates are delayed by drought and whether rainfall can predict breeding season end dates.
Here we present a long-term nesting study to examine these questions. Most long-term studies in the tropics do not have detailed data on nest initiation dates and the length of breeding seasons because nest finding is typically labor intensive and involves a steep learning curve (Martin and Mouten, 2020); but here we provide an analysis based on 10 years of nesting data initially used to study nest predation and nest-site selection (e.g., Khamcha et al., 2018; Somsiri et al., 2019; Khamcha and Gale, 2020; Phringphroh et al., 2024). We predicted that decreases in rainfall would be associated with delays in first egg dates at the start of the season and that increases in rainfall during the breeding season would promote more nesting, indicating later expected fledging dates at the end of a given season.
Methods
Study area
The study was conducted in the Sakaerat Environmental Research Station (hereafter, Sakaerat), Nakhon Ratchasima Province, northeastern Thailand (14.4928°N, 101.9150°E). Sakaerat is a 78 km2 UNESCO biosphere reserve connected to the Phuluang Non-hunting forest reserve of 92 km2. Sakaerat’s elevation ranges from 280 to 762 m above sea level. A five-lane highway (Route 304), rural communities, and agricultural lands surround Sakaerat. Sakaerat has two major forest types: dry evergreen forest and dry dipterocarp forest, plus patches of tree plantations, as well as small patches of grassland and bamboo. The investigation was carried out in secondary (~40 years post logging) dry evergreen forest in a long-term research plot (Figure 1), which was established in 2012 as a 36-ha plot and expanded to 56-ha in 2020. Between 2013 to 2023, the average annual rainfall and temperature were 1,214 mm (range 798–1,530 mm) and 28°C (range 9–40°C), respectively. The rainfall was highly variable, but typically less than half of the amount reported from the previous forest bird long-term study site in the region (Kinabalu Malaysia) (Martin and Mouten, 2020). The dry season in Sakaerat occurs roughly from November to April (Thompson and Landsberg, 1975). The plot has a relatively high density of regenerating woody stems and small trees ~1,064 stems/ha (Somsiri et al., 2019). The research plot was set up using a 50 × 50 m grid marked with ~60 cm tall PVC pipes. We also collected data on the nest predator community in our study area during this time period, which included both arboreal and terrestrial mammals, snakes and birds, the most dominant being northern pig-tailed macaque Macaca leonina, accipiters Accipiter spp., cat snakes Boiga spp. and Blanford’s bridle snake Lycodon cf. davisonii (Khamcha et al., 2018; Somsiri et al., 2019; Khamcha and Gale, 2020; Phringphroh et al., 2024).
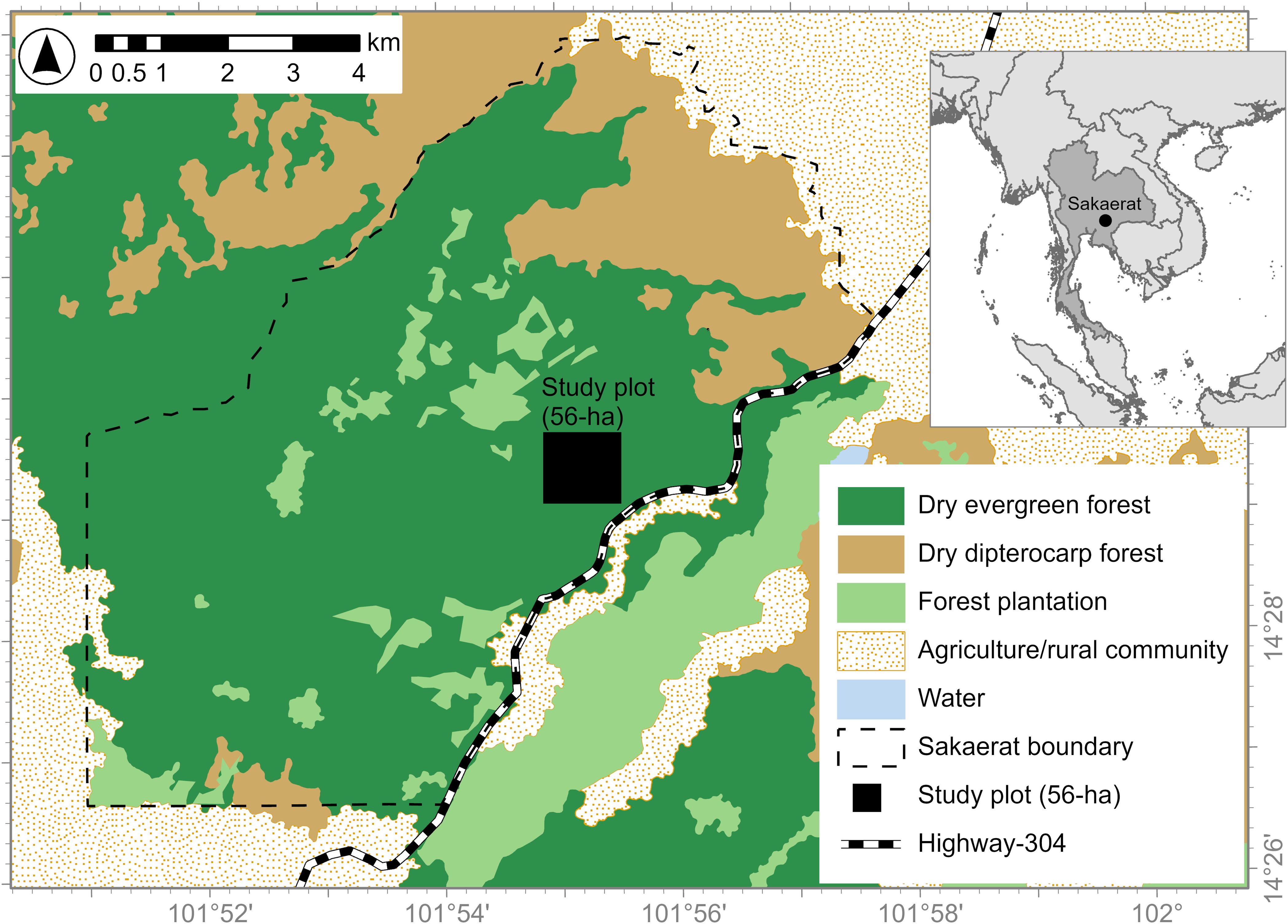
Figure 1. Study area map with the location of the 56-ha study plot in the Sakaerat Environmental Research Station, Nakhon Ratchasima Province, northeastern Thailand.
Nest finding and monitoring
Nest searching was conducted throughout the breeding seasons (January to August) of 2013 to 2023 on the plot following the methods described in Pierce et al. (2019) and Khamcha and Gale (2020). The dry and rainy seasons overlap with the breeding season of our focal species (the dry season typically runs from November to April, and the wet season May to October) (Khamcha et al., 2018). We systematically searched for nests using behavioral cues of adult birds as well as direct visual searches. We prioritized eight relatively common resident species for which larger numbers of nests could be located per season. We focused primarily on eight species of understory-nesting birds, with first egg dates typically ranging from early January to late March, with two species in average rainfall years initiating nesting in January, three species in February, and three species in March (Supplementary Table S1, Supplementary Figure S1). Nests included three types: dome on the ground (Puff-throated Babbler Pellorneum ruficeps), open cavity (shallow cavities in rotten stumps or branches in which an incubating or brooding adult bird is at least partly visible) (White-rumped Shama Copsychus malabaricus, and Indochinese Blue Flycatcher Cyornis sumatrensis), and open cup nests (Black-naped Monarch Hypothymis azurea, Stripe-throated Bulbul Pycnonotus finlaysoni, Puff-throated Bulbul Alophoixus pallidus, Abbott’s Babbler Malacocincla abbotti, and Scaly-crowned Babbler Malacopteron cinereum). Once nests were found, we recorded the species, type of nest, date found, first active date (first laying date), stage of each nest (egg versus nestling), and nest height (measured using measuring tape). We checked all active nests every two to three days until failure or success was determined. Ground nests or low nests (< ~1.5 m) were directly examined, whereas higher nests (>1.5 m height) were checked using a mirror or mobile phone on a pole or observed indirectly through parental activity at the nest (e.g., adults carrying food or nest material). There was very little variation in clutch sizes within a species (Supplementary Table S1). Due to manpower limitations, we had relatively few females of our target species color-marked, although we did have a small number of Puff-throated Babbler, Scaly-crowned Babbler, and White-rumped Shama marked. Thus, we were usually unable to confirm the number of clutches produced by a given female in a given season. We also did not have data on female body condition. We did have access to data from color-marked individuals of Abbott’s Babbler, Puff-throated Bulbul, and Black-naped Monarch from a previous study in Khao Yai National Park ~60 km to the southwest of our Sakaerat plot. Khao Yai receives nearly twice as much annual rainfall than Sakaerat. Nevertheless, based on these data from Sakaerat and Khao Yai most of our target species were confirmed to be capable of producing multiple clutches in a given season (Supplementary Table S1). In addition, waterproof infrared surveillance video cameras (placed ≥1.5 m away from nests) were used to keep track of active nests (defined as having at least one egg). Cameras operated continuously, capturing 24-hour video footage. The recordings were used to determine nest fates, adult behavior, and identify potential predators. Cameras remained in place until nest fates (success or failure) were confirmed.
Rainfall data
The rainfall data were obtained from five permanent weather stations located within a 2-km radius of the study plot. Rainfall was measured hourly using research-grade Delta-T Devices WS-GP1 compact weather stations (Delta-T Devices Ltd., Cambridge, UK). The hourly rainfall data from each station were summed to obtain daily totals, which were then averaged across the five stations.
Statistical analysis
We assessed effects of two rainfall parameters as indexes of the severity of the dry season on nest initiation (first egg dates): (a) the early season rainfall (cumulative rainfall from just before the start of the dry season (1 October) up until one week prior to the first egg date of a given species for that year) and (b) the rainfall gap (the largest number of consecutive “no-rain” days from 1 October to 31 March) which typically occurred towards the end of the dry season. For assessing the effects of rainfall on the timing of the end of breeding season (latest fledging date of the season), (c) we used the total amount of rainfall from 1 October up until 31 March to represent overall dry season rainfall, and (d) the total amount of rainfall from October 1 up until 31 July (typically the end of the breeding season for most species) to represent total rainfall during the breeding season (Figure 2). As we never found an active nest prior to December 1st in any of the study years, we labeled day 1 of each season as 1 December for counting egg days (Julian dates). We also tested the potential impact of any delays in first egg days on the end of breeding season using the last expected fledging date of the latest nest found in a given season. Due to limited manpower, we were unable to ring most adult females of the target species, making it difficult to determine clutch order. We were limited to assessing the likely first and last clutches of each species within a given season. Therefore, the first nest found during the season was used as the indicator of the start date for that species for that year and the last predicted fledging date was used to estimate the end of a given season. Although using mean (or median) first egg dates for this analysis would have been more robust, our dataset presented challenges. The extremely high predation rates and rapid renesting rates we observed made it possible for a female to lay an egg, have it depredated, rebuild a nest, and re-lay within a few days (~less than one week). Because most females were not uniquely marked, therefore to ensure that only first nests of a season were included in this assessment, we limited our analysis to the first active nest found in a given season for a given species, although we did check these dates for the presence of outliers.
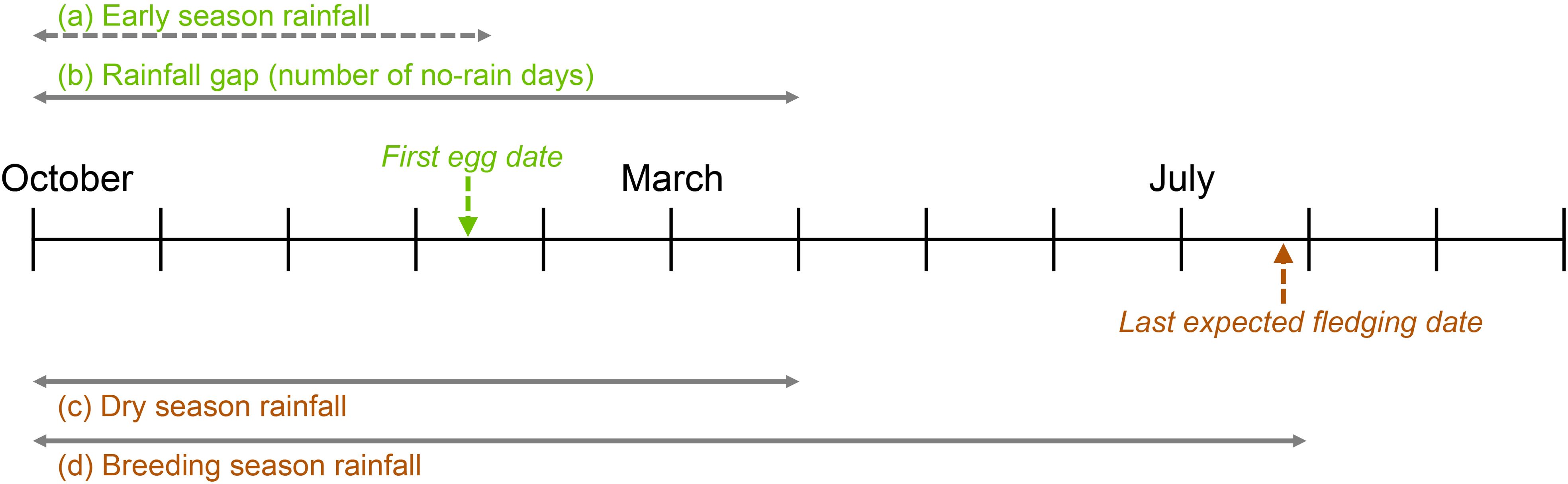
Figure 2. A graphical depiction of the variables being assessed on an annual timeline. The dashed line indicates that the data will vary for different species.
Prior to running the statistical analysis, we calculated the nesting period of each focal species using data from the nests for which the exact first egg date, and/or hatching and fledging dates are indicated (Supplementary Table S1). The first egg dates for nests without this data were extrapolated from hatching or fledging dates, or, when not possible, by using mid-points of the earliest and latest possible first egg dates, based on average incubation and fledging periods. The last expected fledging dates were also calculated using the same approach.
Following Martin and Mouten, 2020, we removed the data from the year 2013 to account for the learning curve for nest finding. After we removed 2013 all models improved. To assess the influence of rainfall parameters, early season rainfall (cumulative rainfall from 1 October to one week prior to the first egg date), rainfall gap (the largest number of consecutive “no-rain” days from 1 October to 31 March), dry season rainfall (cumulative rainfall from 1 October to 31 March), and breeding season rainfall (cumulative rainfall from 1 October up until 31 July) on nest initiation (first egg date) and last expected fledging date of each focal species, we constructed Generalized Linear Models (GLMs) with appropriate error structures (Poisson/negative binomial) using the package glmmTMB (Brooks et al., 2023). The decision for selecting a given model distribution depended on the assumption of equal mean and variance for the Poisson distribution. If the variance exceeded the mean (indicating overdispersion), the negative binomial distribution was considered more suitable as it accounts for this overdispersion. To assess the overall effects of rainfall parameters on nest initiation and the last expected fledging date for all species combined, we used Generalized Linear Mixed Models (GLMMs), with species assigned as a random effect. To evaluate the influence of rainfall parameters on first egg dates, each set of models for each species included a single-variable model of either rainfall gap or early season rainfall and a constant model. Similarly, to assess the influence of rainfall parameters on last expected fledging date of each focal species, each set of models included a single-variable model of either dry season rainfall or breeding season rainfall and a constant model. We used Akaike’s Information Criterion adjusted for small sample size (AICc) for ranking models (Akaike, 1973; Burnham and Anderson, 2004). We considered the strength of evidence for the two rainfall parameters influencing the delays in first egg days and last fledging days using beta coefficients and the 85% confidence intervals following Arnold (2010). We evaluated the data for outliers, overdispersion using the DHARMa package (Hartig, 2022) and all models passed all assumptions. Abbott’s Babbler is scarce in the study plot and was the only species that had insufficient data for analysis, probably due to our inability to consistently find nests of this species each year. For each target species in a given season, to assess the correlation between the first egg date of the first nest found and the expected fledging date of the last nest found, we used the Kendall rank correlation coefficient. We tested the relationship between rainfall gap and total dry season rainfall on expected fledging date also using the Kendall rank correlation coefficient. Additionally, we examined the relationship between total dry season rainfall and total number of active nests for each focal species for a given season; however, our results indicated that there was no significant correlation between rainfall and the number of active nests observed per species (P> 0. 07). All statistical analyses were performed in program R version 4.3.1 (R Core Team, 2023).
In this paper, we did not include an analysis of the impact of rainfall on nest success and failure; however, these aspects have been thoroughly addressed in Phringphroh et al. (2024). Following Phringphroh et al. (2024), lower nest success was associated with periods of higher rainfall for Indochinese Blue Flycatcher, Scaly-crowned Babbler, and Puff-throated Babbler. Additionally, Phringphroh et al. (2024) found that increased rainfall correlated with a higher rate of nest predation by the top four nest predators.
Results
Over the 11 breeding seasons from 2013 to 2023, we used > 10,000 hours for nest searching; we located 1,314 active nests from 32 species including 1,159 nests of our focal species (Supplementary Tables S1, S2). The study period overlapped one “very strong” (2015–16) and one “strong” (2023–24) El Niño event and two “moderate” La Niña events in 2020–21 and 2021–22 as well as weak El Niño and La Niña events in five other years (https://ggweather.com/enso/oni.htm); overall, there was notable variation in annual rainfall in Sakaerat (Figure 3A).
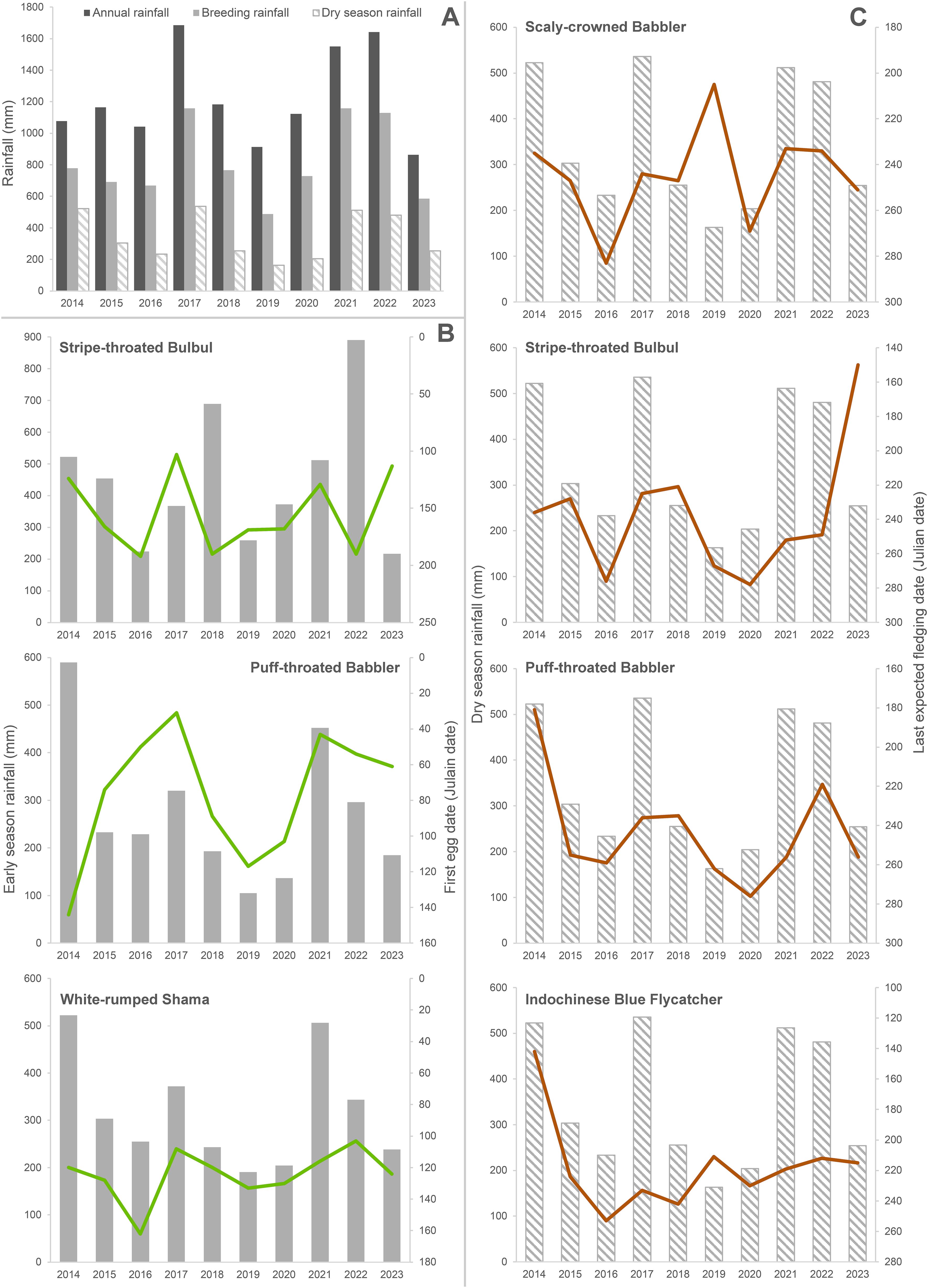
Figure 3. (A) Total annual rainfall (1 October–30 September), cumulative dry season rainfall (1 October–31 March), and breeding season rainfall (1 October–31 July) for years 2014–2023 at Sakaerat Environmental Research Station, Nakhon Ratchasima, Thailand. (B) Cumulative early season rainfall significantly influenced first egg dates of three species: Stripe-throated Bulbul, Puff-throated Babbler, and White-rumped Shama. (C) Cumulative dry season rainfall significantly influenced last expected fledging dates of four species: Scaly-crowned Babbler, Stripe-throated Bulbul, Puff-throated Babbler, and Indochinese Blue Flycatcher. Lines indicate the Julian dates for the first egg dates and the last expected fledging dates for each species for each year.
First egg date
Overall, we found significant effects of rainfall gap (β = 0.00247, SE = 0.00037, 85% CIs = 0.00193 – 0.00301) and early season rainfall (β = -0.00062, SE = 0.00013, 85% CIs = -0.00082 – -0.00043) on the timing of nest initiation across all species combined (Figure 4A). All seven focal species (excluding Abbott’s Babbler) had the same trends in which lower early season rainfall was associated with delays in first egg dates (Table 1). Likewise, longer gaps in dry season rainfall were associated with increases (delays) in first egg dates (Table 1; Figure 5A). Rainfall gap reached statistical significance for four species (Puff-throated Babbler, Puff-throated Bulbul, Stripe-throated Bulbul, and White-rumped Shama), whereas only three of these species (Puff-throated Bulbul was the exception) showed significant trends with early season rainfall (Figures 3B, 5A). Altogether, rainfall gap was a consistently better predictor of first egg date than early season rainfall.
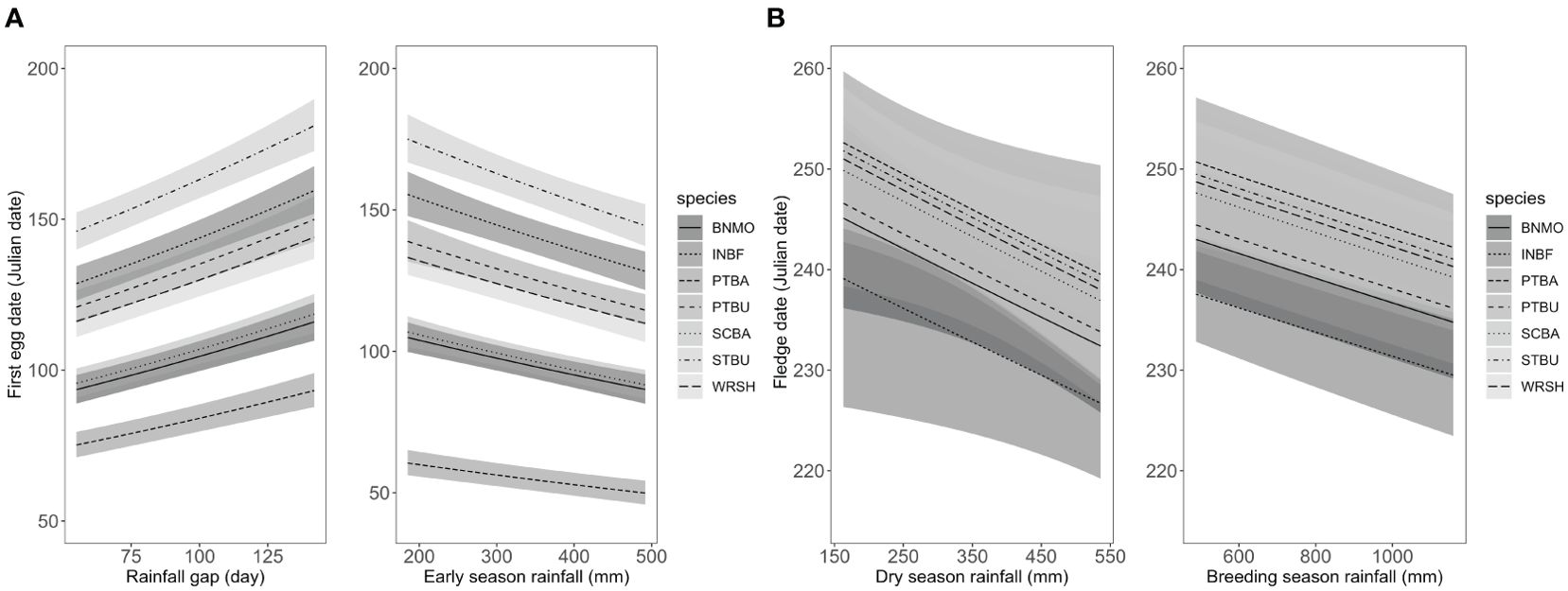
Figure 4. (A) Predicted first egg dates for the community of seven focal songbird species (all seven focal species combined) in relation to rainfall gap (the largest number of consecutive “no-rain” days from 1 October to 31 March) and early season rainfall (cumulative rainfall from 1 October until one week before the first egg date). Mean predicted estimates for each species are shown as distinct black lines, with their 85% confidence intervals in gray. (B) Predicted last expected fledging dates for the community of seven focal songbird species (all seven focal species combined) in relation to dry season rainfall (cumulative rainfall from 1 October to 31 March) and breeding season rainfall (cumulative rainfall from 1 October to 31 July). Mean predicted estimates for each species are shown as distinct black lines, with their 85% confidence intervals in gray. BNMO, Black-naped Monarch Hypothymis azurea, INBF, Indochinese Blue Flycatcher Cyornis sumatrensis, PTBA, Puff-throated Babbler Pellorneum ruficeps, PTBU, Puff-throated Bulbul Alophoixus pallidus, SCBA, Scaly-crowned Babbler Malacopteron cinereum, STBU, Stripe-throated Bulbul Pycnonotus finlaysoni, WRSH, White-rumped Shama Copsychus malabaricus.
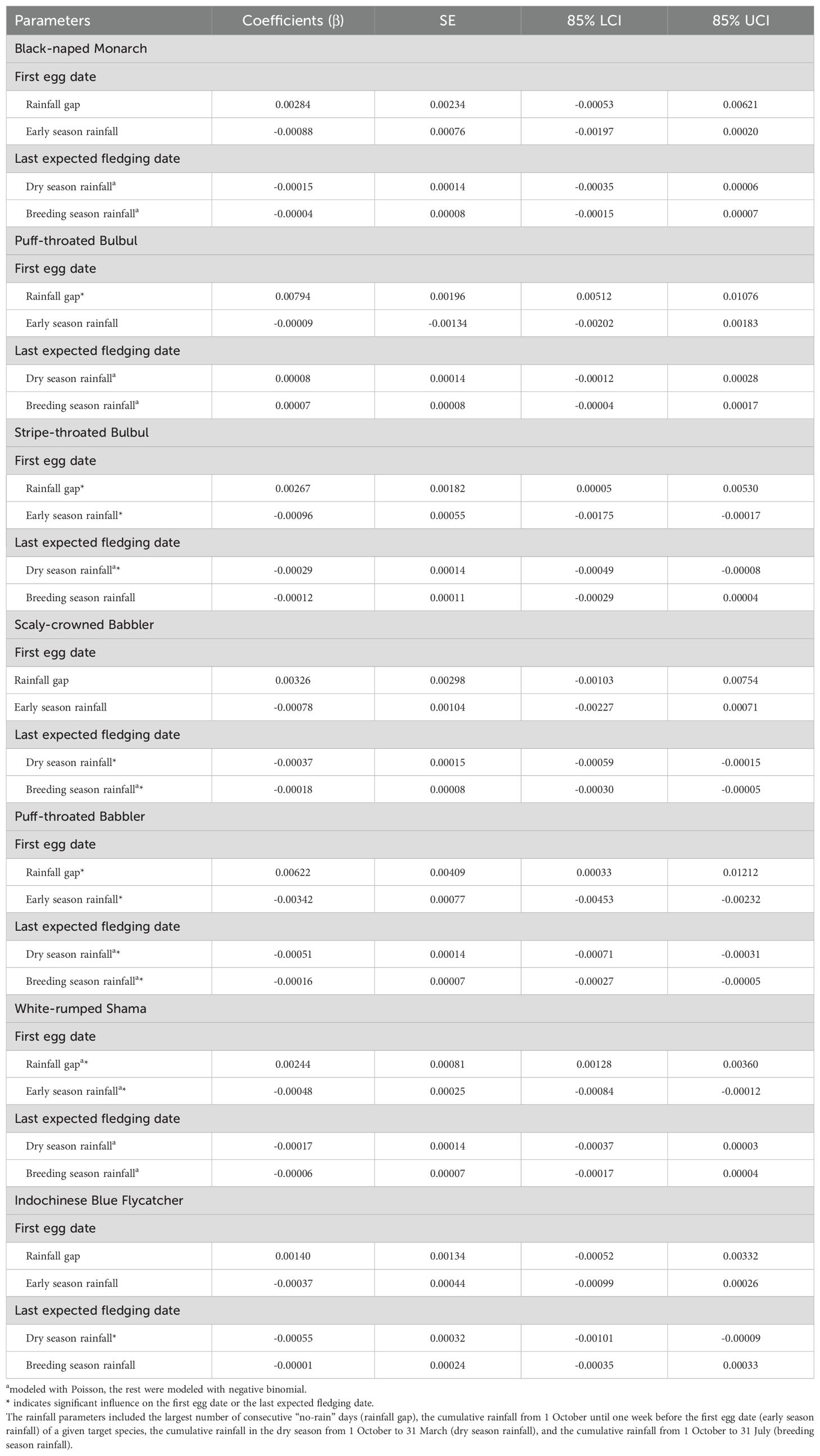
Table 1. Coefficient estimates of rainfall parameters with standard errors (SE) and 85% lower (85%LCI) and upper (85% UCI) confidence intervals influencing the first egg dates and the last expected fledging dates of focal species during the breeding seasons of 2014–2023 at Sakaerat Environmental Research Station, Nakhon Ratchasima, Thailand.
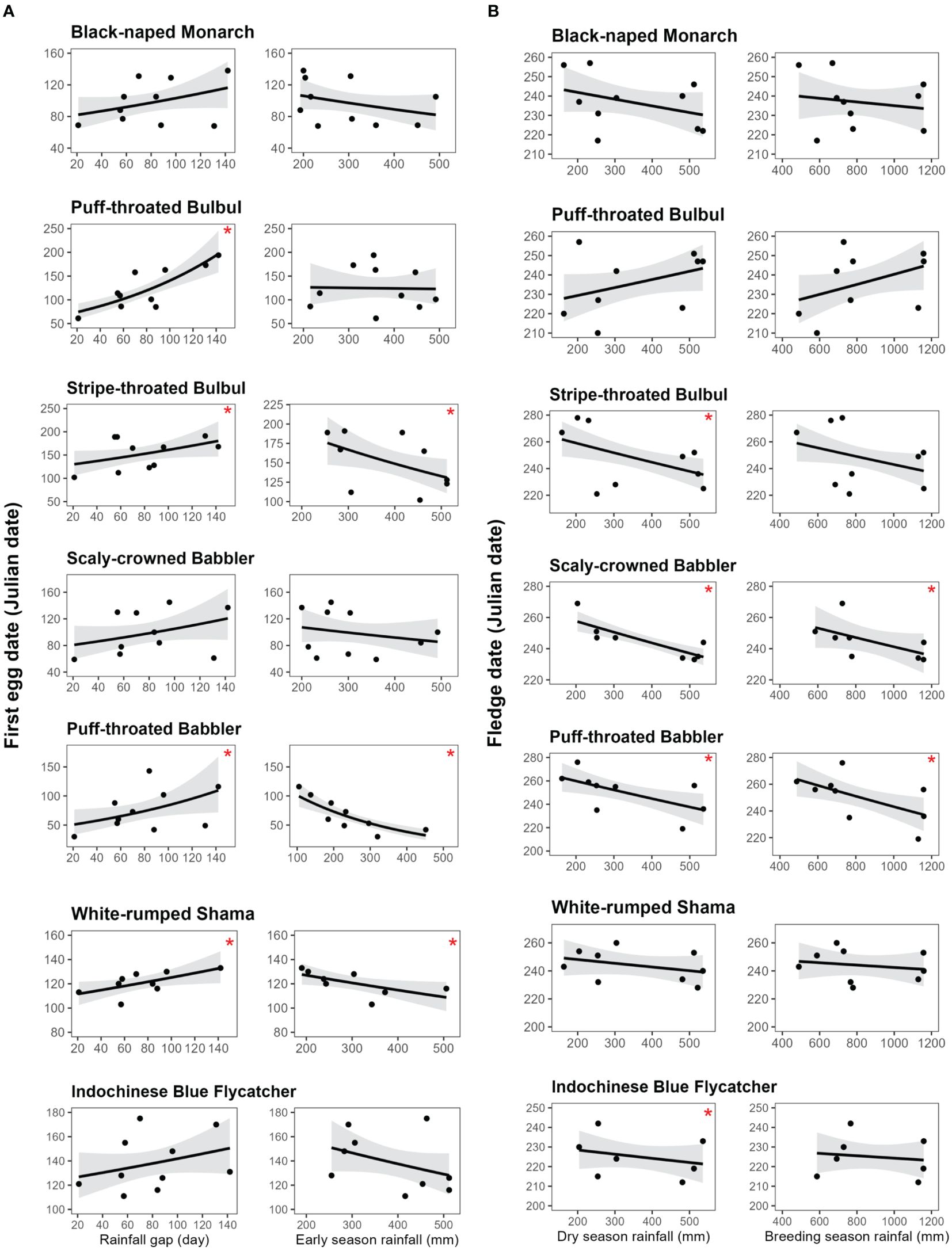
Figure 5. (A) Predicted first egg dates of seven focal songbird species relative to rainfall gap (the largest number of consecutive “no-rain” days from 1 October to 31 March) and early season rainfall (cumulative rainfall from 1 October until one week before the first egg date). Mean predicted estimates are shown as black lines, with their 85% confidence intervals in light gray. * indicates significant influence on the first egg date. (B) Predicted last expected fledging dates of seven focal songbird species relative to dry season rainfall (cumulative rainfall from 1 October to 31 March) and breeding season rainfall (cumulative rainfall from 1 October to 31 July). Mean predicted estimates are shown as black lines with their 85% confidence intervals in light gray. * indicates significant influence on the last expected fledging date. .
Last expected fledging date
Rainfall during the dry and breeding seasons appeared to have significant, yet moderate, effects on the last expected fledging dates for all species combined (β = -0.00017, SE = 0.00001, 85% CIs = -0.00026 – -0.00001 for dry season rainfall and β = -0.00001, SE = 0.00037, 85% CIs = -0.00001 – -0.000003 for breeding season rainfall; Figure 4B). For six of our seven focal species with sufficient data, dry season and breeding season rainfall were negatively associated with last expected fledging date (more rainfall was associated with earlier expected end of season fledging dates), with four species showing significant trends for dry season rainfall (Figure 3C) and two of the same four showing significant trends for breeding season rainfall (Table 1; Figure 5B).
First egg date vs. last expected fledging date
For all seven focal species there was no correlation between first egg date and last expected fledging date (Table 2) indicating that the relative timing of the end of the season typically could not be predicted from the date the nesting season started.
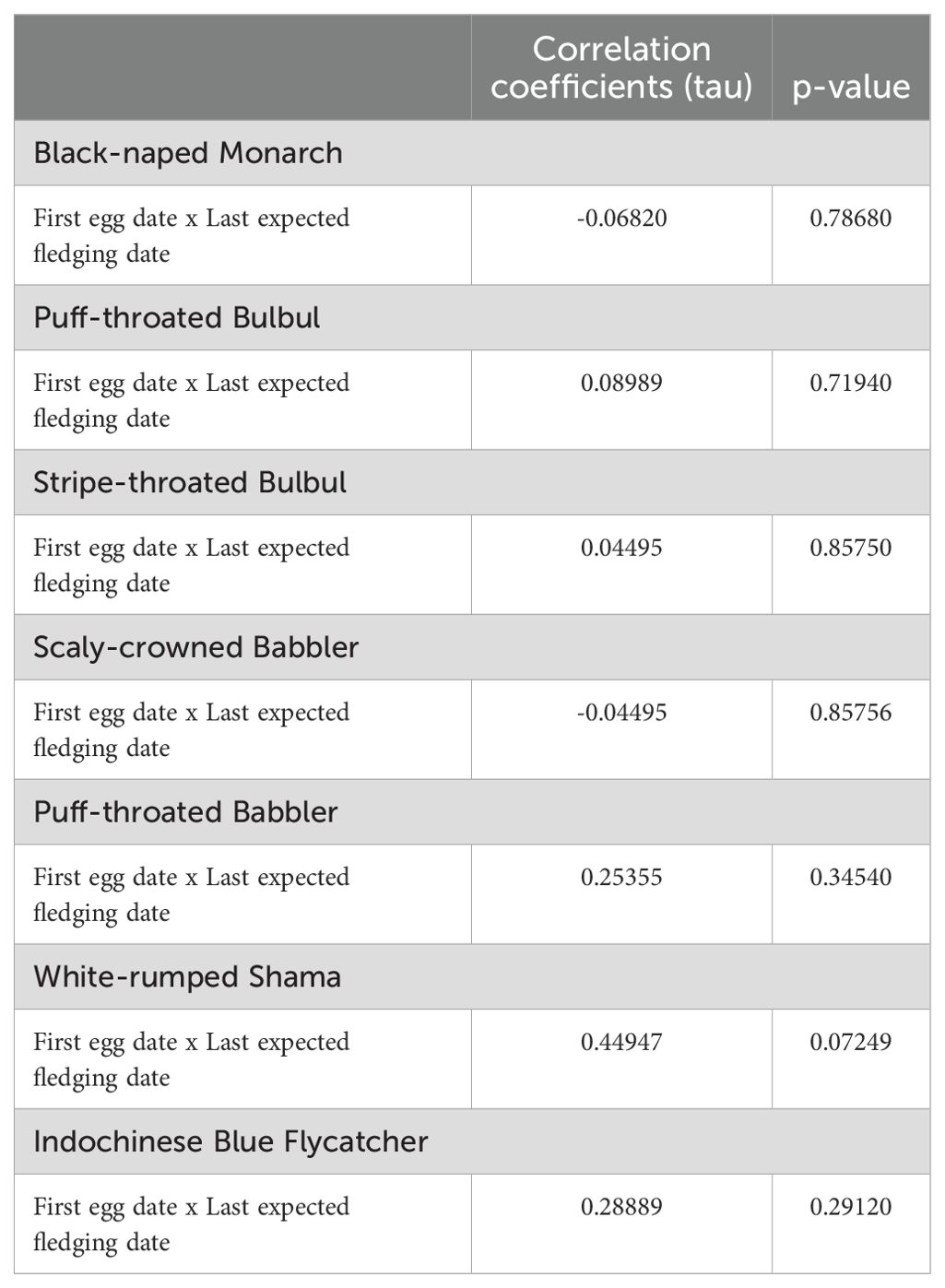
Table 2. The correlation between first egg dates and the last expected fledging dates of the latest nests found in a given season for 10 breeding seasons from 2014–2023 at Sakaerat Environmental Research Station, Nakhon Ratchasima, Thailand.
Discussion
Our ten years of data suggest longer gaps in rainfall led to longer delays in nest initiation dates for our community of seven species overall. The same pattern was observed at the individual species level for at least four of the target species. Lower dry season rainfall and breeding season rainfall were both correlated with last expected fledging dates for the community overall. Individually, lower dry season rainfall affected four species and lower breeding season rainfall affected three species in predicting later last expected fledging dates. There was no relationship between first egg dates and last expected fledging dates for any of the seven target species. Interestingly, for predicting first egg dates, rainfall gap performed slightly better than total rainfall as a predictor for the community as well as for individual species, suggesting that the duration and likely the timing of rainfall is at least as important or likely more important than the overall amount of rainfall. There were no clear patterns as to which of the seven focal species showed statistically significant effects from rainfall in the timing of first egg dates. However, the coefficient for the effect of rainfall gap was positive in all seven focal species and likewise the total rainfall coefficient was negative for all species, this suggested that all of our target species were likely affected similarly but that a combination of issues related to uncertainties in estimating first egg dates and small sample sizes of both nests and years limited the statistical power of our tests for some species. Interestingly, the trends were likewise consistent but in the opposite direction for the last fledging dates, whereby more rainfall during the dry season (including months prior to normal breeding) or more rainfall later in the breeding season was associated with negative coefficients (earlier last fledging dates for six of our seven species). Dry season rainfall was an overall somewhat better predictor of last fledging date compared to breeding season rainfall.
Currently we do not have arthropod or fruit availability data to assess quantitatively and in detail how rainfall is impacting the likely food resources of our target species, but numerous studies have documented that rainfall and length of dry periods have a large effect on local arthropod (e.g., Newell et al., 2023) and fruit abundance (e.g., Wright et al., 1999). Nevertheless, four of our seven species with sufficient data did show significant associations with rainfall, although there was no clear pattern as to why these four showed significant effects and the other three did not, but as we noted above, our samples were limited by a number of factors. One of the main uncertainties was related to our limited ability to reliably find the first nests of the season of a given species; i.e., there was some possibility that first egg dates may have been influenced by the nest-finding experience of the field crew of the particular year. Nest finding, and especially in tropical forests, has a steep learning curve (Martin and Mouton, 2020) and a few species (e.g., Indochinese Blue Flycatcher) were particularly challenging to find. Anecdotally, we noted clear differences in nest-finding abilities among observers and that some observers were uniquely adept at finding a small subset (two to three) of the focal species, but typically found few of the other species in a given season.
We detected a negative relationship between rainfall and the last expected fledging date, with higher rainfall in the dry season (and to some extent breeding season rainfall) correlated with an earlier expected fledging date. One hypothesis is that higher rainfall years may be associated with higher reproductive success across a season, which in turn may allow for adults to finish the season earlier (i.e., rainfall may reduce the number of nesting attempts required to fledge young). In contrast, it is also possible that increased rainfall may actually be having the opposite effect in which recently published work from our plot suggests that increased rainfall was associated with increased activity of the top four predators in the study area (Phringphroh et al., 2024) and that perhaps adults may quit re-nesting after several failed re-nesting attempts. However, our data from nearly the exact same set of species from a nearby study site (Khao Yai) suggests that individual females of at least one of our target species (Puff-throated Bulbul) can attempt up to seven clutches in a single season (Pierce et al., 2019) also suggesting that many individuals may persist with nesting regardless of the predation pressure. Nevertheless, this nearby site was notably wetter than Sakaerat, so it is possible that fecundity rates are lower at Sakaerat compared to Khao Yai.
We found a complex relationship between rainfall and nest productivity that will require further study to untangle. In general, rainfall quantity and timing affected the fecundity of our focal species. Overall, decreased rainfall appears to be reducing the length of breeding seasons in particularly dry years, but that during wetter years breeding also seems to finish earlier. To quantify the demographic effects we will need to accurately measure individual female fecundity. Furthermore, rainfall undoubtedly is having similar effects elsewhere in the tropics, particularly in drier forests such as Sakaerat. We also suspect that forests (such as Khao Yai) with a similar community of birds but which receive significantly more rainfall may be more “buffered” from ENSO events for example (Poulin et al., 1992) and would therefore also be useful for additional comparative studies.
Data availability statement
The raw data supporting the conclusions of this article will be made available by the authors, without undue reservation.
Ethics statement
The animal study was approved by King Mongkut’s University of Technology Thonburi Institutional Animal Care and Use Committee and the Department of National Park, Wildlife and Plant Conservation, Thailand. The study was conducted in accordance with the local legislation and institutional requirements.
Author contributions
GG: Conceptualization, Data curation, Funding acquisition, Investigation, Methodology, Project administration, Resources, Supervision, Validation, Writing – original draft, Writing – review & editing. RA: Data curation, Investigation, Methodology, Resources, Validation, Visualization, Writing – review & editing. DK: Data curation, Formal analysis, Funding acquisition, Investigation, Methodology, Project administration, Resources, Validation, Visualization, Writing – review & editing.
Funding
The author(s) declare financial support was received for the research, authorship, and/or publication of this article. This long-term study was supported by The National Science and Technology Development Agency (NSTDA) (CPMO P-13-00367 & P-14-51347), The National Research Council of Thailand (NRCT) (59 000 190, 60 000 088 & 62 000 277), Thailand Science Research and Innovation (TSRI) (FRB640008, FRB650048/164 & FRB660073/164), King Mongkut’s University of Technology Thonburi (58 000 312 & 64 000 254).
Acknowledgments
We thank T. Artchawakom and S. Weaengsothorn, the former and current directors of Sakaerat Environmental Research Station, for their permission to conduct research and their staff for facilitating this study. We are grateful to A. J. Pierce and W. Sankamethawee, who co-initiated this long-term study and provided invaluable support throughout the study. We also thank the Sakaerat bird team, especially M. Phringphroh, P. Doungkomna, K. Somsiri, and P. Luekhamhan for their valued assistance in nest finding and monitoring. We also thank our internship students from Khon Kaen University, Naresuan University, and Mahidol University for their assistance with the project.
Conflict of interest
The authors declare that the research was conducted in the absence of any commercial or financial relationships that could be construed as a potential conflict of interest.
Publisher’s note
All claims expressed in this article are solely those of the authors and do not necessarily represent those of their affiliated organizations, or those of the publisher, the editors and the reviewers. Any product that may be evaluated in this article, or claim that may be made by its manufacturer, is not guaranteed or endorsed by the publisher.
Supplementary material
The Supplementary Material for this article can be found online at: https://www.frontiersin.org/articles/10.3389/fcosc.2024.1457478/full#supplementary-material
References
Akaike H. (1973). “Information theory and an extension of the maximum likelihood principle,” in Second International Symposium on Information Theory, vol. 267 . Eds. Petrovand B. N., Csaki F. (Akademiai Kiado, Budapest), e281.
Arnold T. W. (2010). Uninformative parameters and model selection using Akaike's Information Criterion. J. Wildl. Manage. 74, 1175–1178. doi: 10.1111/j.1937-2817.2010.tb01236.x
Boersma J., Enbody E. D., Karubian J., Watts H. E., Schwabl H. (2022). Drought disrupts year-round breeding readiness in a tropical songbird. Avian Conserv. Ecol. 17, 44. doi: 10.5751/ACE-2343-170244
Brawn J. D., Benson T. J., Stager M., Sly N. D., Tarwater C. E. (2017). Impacts of changing rainfall regime on the demography of tropical birds. Nat. Clim. Change. 7, 133–136. doi: 10.1038/nclimate3183
Brooks M., Bolker B., Kristensen K., Martin M., Magnusson A., McGillycuddy M., et al. (2023). GlmmTMB: generalized linear mixed models using template model builder. Available online at: https://cran.r-project.org/web/packages/glmmTMB/index.html. (accessed September 10, 2023)
Bunyavejchewin S. (1999). Structure and dynamics in seasonal dry evergreen forest in northeastern Thailand. J. Veg. Sci. 10, 787–792. doi: 10.2307/3237303
Burnham K. P., Anderson D. R. (2004). Multimodel inference: understanding AIC and BIC in model selection. Sociological Methods Res. 33, 261–304. doi: 10.1177/0049124104268644
Chang C. P., Wang Z., McBride J., Liu C. H. (2005). Annual cycle of Southeast Asia—Maritime Continent rainfall and the asymmetric monsoon transition. J. Clim. 18, 287–301. doi: 10.1175/JCLI-3257.1
Cruz-McDonnell K. K., Wolf B. O. (2016). Rapid warming and drought negatively impact population size and reproductive dynamics of an avian predator in the arid southwest. Glob. Change Biol. 22, 237–253. doi: 10.1111/gcb.2016.22.issue-1
Fu R. (2015). Global warming-accelerated drying in the tropics. Proc. Natl. Acad. Sci. U. S. A. 112, 3593–3594. doi: 10.1073/pnas.1503231112a
Grant P. R., Grant B. R., Keller L. F., Petren K. (2000). Effects of El Niño events on Darwin's finch productivity. Ecol. 81, 2442–2457. doi: 10.2307/177466
Halali S., Halali D., Barlow H. S., Molleman F., Kodandaramaiah U., Brakefield P. M., et al. (2021). Predictability of temporal variation in climate and the evolution of seasonal polyphenism in tropical butterfly communities. J. Evol. Biol. 34, 1362–1375. doi: 10.1111/jeb.13895
Hartig F. (2022). DHARMa: residual diagnostics for hierarchical (multi-level/mixed) regression models. Available online at: https://cran.r-project.org/web/packages/DHARMa/vignettes/DHARMa.html. (accessed September 10, 2023)
Hidalgo Aranzamendi N., Hall M. L., Kingma S. A., van de Pol M., Peters A. (2019). Rapid plastic breeding response to rain matches peak prey abundance in a tropical savanna bird. J. Anim. Ecol. 88, 1799–1811. doi: 10.1111/1365-2656.13068
Khamcha D., Gale G. A. (2020). Predation behaviour of the bridle snake (Lycodon cf. davisonii) on Asian tropical evergreen forest bird nests. Raffles Bull. Zool. 68, 803–809. doi: 10.26107/RBZ-2020-0091
Khamcha D., Powell L. A., Gale G. A. (2018). Effects of roadside edge on nest predators and nest survival of Asian tropical forest birds. Glob. Ecol. Conserv. 16, e00450. doi: 10.1016/j.gecco.2018.e00450
Martin T. E., Mouton. J. C. (2020). Longer-lived tropical songbirds reduce breeding activity as they buffer impacts of drought. Nat. Clim. Change 10, 953–958. doi: 10.1038/s41558-020-0864-3
McKinnon E. A., Rotenberg J. A., Stutchbury B. J. (2015). Seasonal change in tropical habitat quality and body condition for a declining migratory songbird. Oecologia 179, 363–375. doi: 10.1007/s00442-015-3343-1
Newell F. L., Ausprey I. J., Robinson S. K. (2023). Wet and dry extremes reduce arthropod biomass independently of leaf phenology in the wet tropics. Glob. Change Biol. 29, 308–323. doi: 10.1111/gcb.16379
Phringphroh M., Khamcha D., Sankamethawee W., Powell L. A., Angkaew R., Pierce A. J., et al. (2024). Nest site vegetation structure influences nest predators and nesting success of understory birds in a dry evergreen forest in northeastern Thailand. Ornithol. 141, 12. doi: 10.1093/ornithology/ukae031
Pierce A. J., Sankamethawee W., Powell L. A., Gale G. A. (2019). Patterns of nesting and nest success in an evergreen forest in Southeast Asia. Emu-Austral Ornithol. 120, 46–55. doi: 10.1080/01584197.2019.1686645
Poulin B., Lefebvre G., McNeil R. (1992). Tropical avian phenology in relation to abundance and exploitation of food resources. Ecol. 73, 2295–2309. doi: 10.2307/1941476
R Core Team (2023). R: A language and environment for statistical computing (Vienna, Austria: R Foundation for Statistical Computing). Available at: https://www.R-project.org/.
Siepielski A. M., Morrissey M. B., Buoro M., Carlson S. M., Caruso C. M., Clegg S. M., et al. (2017). Precipitation drives global variation in natural selection. Science 355, 959–962. doi: 10.1126/science.aag2773
Skagen S. K., Augustine D. J., Derner J. D. (2018). Semi-arid grassland bird responses to patch-burn grazing and drought. J. Wildl. Manage. 82, 445–456. doi: 10.1002/jwmg.21379
Somsiri K., Gale G. A., Pierce A. J., Khamcha D., Sankamethawee W. (2019). Habitat structure affects nest predation of the Scaly-crowned Babbler (Malacopteron cinereum) by macaques and snakes in a Thai-seasonal evergreen forest. J. Ornithol. 161, 389–398. doi: 10.1007/s10336-019-01724-0
Thompson O. E., Landsberg H. E. (1975). Climatological conditions in the Sakaerat forest, Thailand. Geografiska Annaler: Series A, Phys. Geogr 57, 247–260. doi: 10.1080/04353676.1975.11879919
Trenberth K. E., Dai A., van der Schrier G., Jones P. D., Barichivich J., Briffa K. R., et al. (2014). Global warming and changes in drought. Nat. Clim. Change. 4, 17–22. doi: 10.1038/nclimate2067
Keywords: climatic variability, drought, expected fledging dates, first egg dates, long-term study, tropical birds
Citation: Gale GA, Angkaew R and Khamcha D (2024) Changes in breeding activity of Southeast Asian songbirds in response to the severity of the dry season. Front. Conserv. Sci. 5:1457478. doi: 10.3389/fcosc.2024.1457478
Received: 30 June 2024; Accepted: 13 November 2024;
Published: 04 December 2024.
Edited by:
Monte Neate-Clegg, University of California, Los Angeles, United StatesReviewed by:
Nicola J. Van Wilgen, South African National Parks, South AfricaJames Cox, Tall Timbers Research Station and Land Conservancy, United States
Copyright © 2024 Gale, Angkaew and Khamcha. This is an open-access article distributed under the terms of the Creative Commons Attribution License (CC BY). The use, distribution or reproduction in other forums is permitted, provided the original author(s) and the copyright owner(s) are credited and that the original publication in this journal is cited, in accordance with accepted academic practice. No use, distribution or reproduction is permitted which does not comply with these terms.
*Correspondence: Daphawan Khamcha, ZGFwaGF3YW5AZ21haWwuY29t