- 1Anti-Personnel Landmines Detection Product Development (APOPO) Training and Research Centre, Sokoine University of Agriculture, Morogoro, Tanzania
- 2Division of Depression and Anxiety Disorders, McLean Hospital, Belmont, MA, United States
- 3Department of Psychiatry, Harvard Medical School, Boston, MA, United States
- 4Endangered Wildlife Trust, Wildlife in Trade Programme, Midrand, South Africa
- 5Department of Zoology and Entomology, Mammal Research Institute, University of Pretoria, Pretoria, South Africa
- 6Evolutionary Ecology Group, Department of Biology, University of Antwerp, Wilrijk, Belgium
- 7Rutgers Center for Cognitive Science, Rutgers University, Piscataway, NJ, United States
The illegal wildlife trade (IWT) is one of the largest global crime economies, directly threatening species and their habitats, and biodiversity, and indirectly the global climate, and countries’ economies. Syndicates operating within the IWT are often involved in trafficking of humans, weapons, and drugs. IWT takes place in large scale through international ports, with wildlife being smuggled inside shipping containers. Current methods to combat IWT and screen these shipping containers, such as X-ray scans, are expensive and time-consuming. Scent-detection animals present an innovative approach to combatting IWT, as animals may be better suited to distinguish between organic materials and less susceptible to visual concealment methods. Previous scent-detection work has largely focused on conservation dogs (Canis lupus familiaris). Here, we present a series of proof-of-principle studies training African giant pouched rats (Cricetomys ansorgei) to detect illegally trafficked wildlife. As initial proof-of-principle within a controlled laboratory environment, we trained rats (n=11) to detect the scent of pangolin scales (Manis spp.) and a threatened African hardwood (Dalbergia melanoxylon). In subsequent experiments, we trained these rats (n=10) to detect rhinoceros horn (family Rhinocerotidae), and elephant tusks (Loxodonta spp.). At the conclusion of these studies, rats (n=7) accurately identified all four target species (although ivory samples may have been cross-contaminated, and results should be interpreted with caution) while correctly rejecting non-target items commonly used by traffickers to conceal the presence of illicit material. Rats detected the presence of wildlife targets even when these were concealed in mixtures among non-target items. These results provide the foundation to train rats for scent-detection of illegally trafficked wildlife to combat IWT. The unique attributes of rats could allow them to complement existing screening technologies in the fight against IWT. Rats have low training and maintenance costs, flexibly work with multiple handlers, have a long lifespan, and a sophisticated sense of smell. Their small size also offers unique capabilities for the screening of shipping containers, such as being able to navigate densely packed areas or be lifted to assess contents of sealed containers by screening ventilation systems. Future directions include assessing operational feasibility of deploying rats at ports.
1 Introduction
The illegal wildlife trade (IWT) in flora and fauna is considered the fourth largest transnational crime economy, estimated to be worth between $7–23 billion U.S. dollars (USD; Zavagli, 2021). IWT is closely linked to crimes such as money laundering, corruption, and simultaneous trafficking of drugs, weapons, and/or humans (FATF, 2020; UNODC, 2020; Zavagli, 2021). Additionally, the spread of zoonotic diseases can be linked to the unregulated illegal trade of species across countries (de Sadeleer and Godfroid, 2020), exacerbating public health emergencies such as the novel coronavirus pandemic. Although both international government-led treaties and policies, such as the Convention on International Trade in Endangered Species of Wild Fauna and Flora (CITES), and multiple non-governmental/charitable initiatives have worked tirelessly on combatting IWT, experts still estimate that natural resources worth $91–258 billion USD are trafficked annually (Nellemann et al., 2016). This crime economy devastates biodiversity and the environment while threatening human health and national security by disrupting local, regional, and international economic and political stability (Nellemann et al., 2016; UNODC, 2020; Zavagli, 2021). The threat of IWT must be met with innovative approaches while remaining cost-effective and flexible to adapt to ever-changing trafficking routes, techniques, and settings.
Pangolins (genus Manis spp.), elephants (genus Loxodonta), and rhinoceros (rhino; family Rhinocerotidae) are species known to be significantly affected by IWT. All eight species of pangolin (four in Africa and four in Asia) and all species of elephant and rhino are listed as threatened on the International Union for Conservation of Nature’s (IUCN) Red List of Threatened Species (Willcox et al., 2019) and on CITES’ Appendix I (Bale, 2019), indicating a high risk of extinction and heightened requirements for legal trade. Pangolins have acquired the title “world’s most heavily trafficked mammal” (Challender et al., 2014; Heinrich et al., 2017; Ingram et al., 2018, 2019). They are trafficked internationally for meat and scales (consisting of keratin), fetching large sums as luxury products (Challender et al., 2014; Gomez et al., 2016).
The trade of elephant ivory (made of dentine) and rhino horn (composed primarily from keratin) is rooted in traditional and modern South-East and East Asian cultural beliefs, with demand linked to their alleged healing properties as well as use in art (e.g., ivory carvings). The illegal killing (colloquially known as poaching) of elephants and rhinos has been linked to local poverty and exorbitant market prices for their products, however, corruption and organized crime facilitates their continued trade (Hauenstein et al., 2019; Smith et al., 2003). Between 2007 and 2014, there was a 30% decline in African elephant numbers (Chase et al., 2016) and over the past decade, over 11,000 rhinos were lost due to poaching (e.g., Sanchez-Barreiro et al., 2021; Save The Rhino, 2024).
Although wildlife is the most publicized target of IWT, flora, particularly trees, are also at risk. Illegal logging negatively affects on economies and the environment, including loss of suitable habitat of forest-dwelling species and associated biodiversity loss, as well as contributing to climate change (Khalid et al., 2019). Dalbergia melanoxylon, or East African blackwood, is a large genus of small to medium-size trees, shrubs, and lianas, that is commonly affected by illegal logging and unsustainable harvesting. D.melanoxylon is a small, slow-growing tree that does not reach harvestable age until it is around 70 years old. The inner part of the tree, the heartwood, is commonly used in the manufacturing of woodwind musical instruments and decorative objects (Cunningham, 2015; Nakai et al., 2019), fueling unsustainable harvesting. Reportedly, the proportion of illegal timber logging in Tanzania has been as high as 96% and timber imports from Tanzania into China exceeded five times the exports reported from Tanzania (Milledge et al., 2007; Campbell et al., 2007).
International efforts to curtail IWT span the entire trafficking pipeline and have included: preventative measures to stop poachers from procuring wildlife in the field (e.g., anti-poaching units), various methods to detect illegal wildlife products as they are trafficked, and campaigns geared towards reducing consumer demand (e.g., Harrington et al., 2018; ‘t Sas-Rolfes et al., 2019). Authorities also use innovative wildlife forensic tools such as genetic tracing (also known as “DNA barcoding”) and the identification of isotopic signatures to determine where specimens were procured (Brandis et al., 2018; Luczon et al., 2016); however, these techniques are not readily deployed and can only be applied after the specimen has been detected or seized. A recent report by WWF and TRAFFIC (Zavagli, 2021) found that container shipping is the dominant method used to traffic illegal wildlife products in large volumes. Thus, a common screening method in both air and seaports is the X-ray scanner; however, the sheer volume of shipping cargo poses barriers to scanning every container, including imposing substantial delays and increased costs. The purchasing cost alone of these scanners is several hundred thousand USD, and continued operation and maintenance are estimated to cost “tens of thousands to hundreds of thousands of dollars each year” (Congressional Budget Office, 2015). Additionally, X-ray and other visual screening methods are limited by their ability to distinguish types of organic material, allowing illicit items to be hidden within or among licit material (Buffler and Tickner, 2010).
A promising alternative to visual scanners is the use of scent-detection animals (Braun, 2013; Lazarowski et al., 2020). Dogs (Canis lupus familiaris), for example, have been successfully trained to detect wildlife contraband and have been deployed to ports to screen containers (W. Powell, pers. comm.). Canine units offer more logistical flexibility and training cost is cheaper than purchasing and running X-ray scanners. Nevertheless, canine units face additional limitations. Each scent-detection dog requires up to one year of training and is associated with high purchasing and maintenance costs, with some training organizations estimating between $20,000–30,000 USD per dog (King, 2013). A conservation dog requires a skilled handler who further requires training and investment, and the dog may not be easily transferrable between handlers. The efficacy of canine units is also tempered because they cannot search containers loaded onto trucks without the provision of steps to access the seal or penetrate tightly packed areas within shipping parcels. Containers stacked on top of each other further increase the distance between potential targets and the detection dog, leading to odors becoming increasingly diluted (Lazarowski et al., 2020), further reducing the ability of canines to screen additional layers of cargo.
Like dogs, African giant pouched rats (Cricetomys ansorgei, henceforth rats) have been successfully deployed for scent detection work (c.f., Weetjens et al., 2009; Poling et al., 2011a, b; Ellis et al., 2017). These rats have extensive olfactory receptor repertoires and large olfactory bulbs (Freeman et al., 2020) which speaks to their ability to detect and discriminate a large variety of odors. Further, this species has an average life span between seven to nine years which makes them suitable to be trained and subsequently deployed as scent detection animals (Webb et al., 2020). The rats are adapted to the sub-Saharan climate, occurring across East and southern Africa (Olayemi et al., 2012), and can be trained to search for multiple odor targets (Webb et al., 2020). Their small size makes them more suitable for navigating tightly packed areas, and compared to canines, they are inexpensive to train and maintain and can easily be transferred between handlers. These characteristics and abilities make them a model species for scent detection. In fact, APOPO has successfully trained and deployed African giant pouched rats for the detection of explosives in post-conflict zones and for the tuberculosis (TB)-causing pathogen, TB mycobacterium, over the past 25 years.
This project aimed to establish proof-of-principle that rats can be trained to detect illegally smuggled wildlife, specifically, pangolin scales, rhino horn, elephant ivory, and African blackwood. We also tested operational feasibility by examining how the rats generalize to new specimens and their ability to detect targets when they were concealed by items commonly used by smugglers. We hypothesized the rats could be successfully trained to detect the four wildlife targets, retain the targets for long periods, and find the targets even when they were concealed. The research described here served as the first step in a phased approach to train rats as a novel solution to complement existing detection technologies and aid in the detection of illegally trafficked wildlife.
2 Methods
All research was conducted at APOPO’s Training and Research Headquarters in Morogoro, Tanzania. The following procedures were conducted with approval from the Institutional Committee for Research Involving Animals of the Sokoine University of Agriculture. Throughout all experiments, animals were exclusively trained through the use of positive reinforcement.
2.1 Subjects
Eleven rats (five female) approximately one year old at the beginning of the project served as subjects. Unfortunately, one rat (Kirsty) had to be discontinued from training during Phase 2 of Experiment 1 due to health issues. Two rats (Thoreau and Desmond), developed unrelated health issues and passed away during Experiment 2, thus leaving eight rats. Following APOPO’s standard training protocols, rats had previously been socialized to humans and habituated to various environments (e.g., Poling et al., 2011b; Mahoney et al., 2013). All animals were single-, or pair-housed with same-sex littermates in kennels equipped with a clay sleeping pot, an untreated wooden climbing/gnawing structure, wood shaving substrate, and ad libitum drinking water. Free-feeding weight of each rat was maintained by providing daily required amounts during experimental sessions (supplemented in the home cage two hours later, as needed). On days when no experimental sessions took place (holidays and weekends) rats were provided with 20 g of rat chow (Carfil Quality or Specialty Feeds Maintenance Food) and a variety of locally sourced produce (e.g., banana, cucumber, sun-dried fish, etc.).
The sample size was in line with APOPO’s previous experimental work and informed by APOPO’s logistical constraints, established low attrition rates during training, and previously published large effect sizes in experimental and operational studies which suggest studies with 10 rats are sufficiently powered (80% powered to detect large effect at α = 0.05; see Webb et al., 2020; Ellis et al., 2019, 2017). All 11 rats had been previously trained to discriminate between an un-related target odor (Orange Terp BM Citronova Fab; vapor pressure = 133.32 Pa; Firmenich) and five non-targets (see Lewon et al., 2019). Following this experiment, rats were placed on rest for one month before beginning training with wildlife targets.
2.2 Apparatus
APOPO’s custom-built semi-automated line cage (ALC; see Supplementary Figure S1 for a schematic and Ellis et al., 2019 for more details) was used throughout this project. The ALC is a stand-alone aluminium and Perspex glass apparatus fitted with a hinged lid and contains 10 holes (3 cm diameter) along the floor, spaced 10 cm apart. A Perspex glass divider can be inserted to restrict access to fewer holes. Each hole is equipped with a through-beam photoelectric sensor, providing continuous auditory feedback when the beam is broken by a rat poking its nose inside the hole. Scent samples, placed in aluminium cassettes, are positioned underneath the ALC floor and metal sliding plates in the floor can be opened and closed for access to scent samples. Once placed inside, a rat is trained to evaluate all samples placed underneath the holes in order, starting always at hole one. The through-beam sensor reads the time the rat holds their nose within the hole. Once the rat has sniffed the first hole and retrieved its nose, this hole is closed, and the next hole opened and so on. Once the rat has evaluated all 10 samples, the aluminium cassette underneath is replaced with the next one. Reinforcement of flavored pellets (5TCY OmniTreat™) is provided automatically at a fixed location via a dispenser (ENV-203-94, MedAssociates, Georgia, USA) triggered by a pre-determined beam break duration. The apparatus is programmed with a pre-determined and adjustable duration which, if a rat holds their nose for this duration, reads it as an indication of the sample beneath. Known positive samples are programmed to be reinforced with an automatic click sound and release of food reward. Therefore, if a rat is trained to hold their nose within a hole for three consecutive seconds as an indication, and this behavior is performed on a sample which is a reinforced target, the apparatus automatically delivers a click and food reward to reinforce this indication. Once the rat has retrieved their reward, they are trained to resume their evaluation from the hole following on the previously rewarded one. The apparatus is programmed with randomised orders of samples each day and automatically reads and transcribed the exact sniff time for each sample, as well as sample identity and whether a known target was indicated and reinforced.
2.3 Sample preparation
Each item (cut or chopped as needed) was placed in a 3 x 5 cm glass vial (Lenz Laborglasinstrumente, Wertheim, Germany) affixed with an airtight lid when not in use during training. See Supplementary Tables S1 and S2 for list of all items used. Storage of all samples used was in a single room which was also where samples were prepared using gloves and clean tools (cleaned between each sample using methylated spirit). Safes were used for the storage of wildlife targets as well as for non-target materials used within a given week.
2.3.1 Wildlife targets
Unless otherwise noted, all wildlife materials were obtained from the Tanzanian Wildlife Management Authority (TAWA) under authorization from the Tanzanian Ministry of Natural Resources and Tourism. Pangolin scales as well as hardwood were obtained at the beginning of this study in 2017 while rhino horn and elephant ivory were obtained in 2020.
Pangolin Scales (henceforth Pangolin): We were provided with 36 Pangolin (Smutsia temminckii) scales (roughly 500 g) from two different individuals labelled as “Pangolin 1” and “Pangolin 2”. These specimens reportedly died of natural causes at the Dar es Salaam Zoo and were unpreserved aside from freezing. Scales were sundried for one week before being cut and securely stored. Three volumes (0.5, 1, and 1.5 g) were used as low, middle, and high concentrations, respectively of each specimen as samples. These samples were stored within plastic zip-lock bags (separate bag for each specimen) inside an individual safe.
African blackwood (henceforth Wood): Samples were secured from two sources. A piece of treated Wood, seized by local authorities, was used for most sessions and therefore, unless otherwise noted, “Wood” refers to this specimen. The sample was stored inside a plastic zip-lock bag within an individual safe. Additionally, we obtained freshly cut Wood (bark removed) from Sokoine University of Agriculture’s Forestry department (referred to as Fresh Wood where applicable). Each specimen was chopped with a clean axe to produce 1 g pieces of Wood which were stored in sealed sample glass vials throughout the duration of the test. Samples used for sessions contained either 2 g, 3 g, or 4 g (low, medium, and high concentration, respectively).
Rhino horn (henceforth Rhino): Shavings from the horn of nine animals were collected when the animals were outfitted with tracking devices. Unfortunately, collection records from TAWA did not include which Rhino specimen originated from which sub-species but given the area of collection, shavings of at least seven specimens were from black rhino, Diceros bicornis, with geographical range of the remaining two samples also containing white rhino, Ceratotherium simum. Shavings were securely stored in individual plastic zip-lock bags for each animal with approximately 0.5 g used for each sample during training sessions. All samples were stored within a safe alongside elephant ivory (see discussion about the potential implications of this).
Elephant Ivory (henceforth Ivory): Small pieces of Ivory from African savannah elephants, Loxodonta africana, were chipped off larger tusk sections from ten animals using clean bolt cutters. Ivory samples were stored separately in zip-lock bags for each animal with the remaining larger tusks being stored without individual packaging. All samples were stored within a safe alongside rhino horn. After 11 training sessions, sample volume was increased from 0.5 g to 1.3–1.5 g.
2.3.2 Non-targets (masking agents)
The initial ten non-target items were selected from a list maintained by the Endangered Wildlife Trust (EWT) using CITES seizure reports to identify additional contents of packages containing illicit wildlife substances. These initial items were: 1) electrical cables, 2) synthetic/plastic hair wigs (Darling), 3) new cotton socks, 4) coffee beans (Africafe), 5) dengu seeds, 6) cardboard, 7) washing powder (Omo, “original scent”), 8) unshelled raw peanuts, 9) Delonix regia (locally referred to as Christmas or Flame Tree) seeds, and 10) D. regia seedpods. To ensure ample training variability, additional items were added after completion of Experiment 1 (see below), resulting in a total of 165 non-target items, including both organic (e.g., seeds and feathers) and inorganic or synthetic material (e.g., metals, plastics, and fabrics). All non-target items were securely stored separately in zip-lock bags and handled under the same conditions as targets. Only perishable items were replaced as needed with fresh samples, while non-perishable items remained the same throughout to account for the total duration and repeat use of samples throughout the experiment, similar to target items. In addition, the large volume of all non-target items prohibited us from storing them within a safe. However, non-target materials used within a given week were stored in a single designated safe to mimic storage conditions of wildlife targets as closely as possible.
2.4 Behavioral procedures
Sessions were conducted five days a week (barring public holidays or office closures). The experiments described below took place between 5th December 2017 and 7th December 2021 (see Supplementary Table S1 for a breakdown of dates and all training and tests that rats underwent over this period).
Throughout training stages, rats were required to evaluate all samples in a session within 20 minutes. If a rat failed to sniff all samples after 20 minutes, the session was terminated at the last sample the rat had visited. Within a session, if a rat failed to visit the next sample hole in sequence after 3 minutes, the rat was removed from the apparatus and the session was paused while all other rats completed their respective sessions. After the final rat finished for the day, the rat was returned to the ALC, and the session was resumed at the sample hole where it had left off. If the rat again failed to visit a hole within 3 minutes, the session was terminated for the day.
2.4.1 Indication training
Using a Perspex partition in the ALC, access was restricted to three holes containing only target samples. The indication threshold (required nose-poke duration to receive a food reward) was set to 1.0 second (s) during the first session and subsequently increased to 1.5s. The trainers shaped the rat’s indication response by re-opening the same hole until the rat inserted its nose and met the threshold. These shaping trials did not count as a correct indication. During indication training with Pangolin and Wood (Experiment 1), a rat’s threshold was increased by 0.5s increments after it had indicated more than 80% of targets during the preceding sessions. Adjustments continued until all rats met a threshold of 2.5s.
During the first 11 sessions of Experiment 1, two rats (Desmond and Thoreau) failed to indicate ≥80% of the targets or exceeded the time limit. Therefore, during Session 12 they were presented with only their previous target (Orange Terp). Session 13 included four samples of the previous target (varying concentrations), four samples of Pangolin 1 and four samples of Wood. All following sessions included only Pangolin 1 and Wood.
2.4.2 Discrimination training
Non-target samples were introduced in phases to gradually increase sample numbers evaluated during each session. Phase 1 consisted of 30 samples, Phase 2 consisted of 50 or 60 samples, and Phase 3 of 100 samples. Rats advanced through phases as a group. Indications were classified as either hits (on targets) or false alarms (on non-targets).
We included known and reinforced wildlife targets as well as so-called blind targets which were not reinforced at advanced stages of discrimination. Blind targets are samples that are not known to handlers and are not reinforced when correctly indicated by a rat. This not only eliminated unintentional cueing to target location but also prepared rats for operational scenarios where indications on unknown materials cannot be rewarded by their handlers.
Indication thresholds were adjusted individually throughout. During Experiment 1, thresholds were increased by 0.5s if a rat committed >10% false alarms and kept at the new threshold for a minimum of five sessions. Similarly, if a rat failed to indicate ≥75% of targets, their indication threshold was decreased by 0.5s. During Experiment 2, if a rat committed ≥20% false alarms during five consecutive sessions, the threshold was increased by 0.5s. If the rat continued to commit ≥20% false indications for two consecutive sessions, the threshold was further increased by 0.5s. If a rat failed to hit ≥50% of targets during Phase 1, the threshold was decreased by 0.25s. During Phases 2–3, we decreased the threshold by 0.25s if a rat failed to hit ≥66% of targets.
2.5 Experiment 1: can rats be trained to detect wildlife targets?
Following indication training with Pangolin 1 and Wood, rats progressed through discrimination training.
Phase 1: Each session contained 18 non-targets and 12 targets (six Pangolin 1 and six Wood, two of each concentration). Non-target items included six items that rats had previously encountered (cotton socks, electrical cables, cardboard, dengu seeds, coffee beans, and synthetic hair wig; Lewon et al., 2019) and four new items (washing powder, tree seedpods, tree seeds, and peanuts) presented randomly once or twice per session.
Phase 2: Total number of samples per session increased to 50, by increasing non-targets to 38 samples per session. Of 12 targets (six each of Pangolin 1 and Wood), two were non-reinforced blind samples (one each of Pangolin 1 and Wood).
Phase 3: Non-targets increased to 88 samples while all other procedures followed those described for Phase 2. Training continued for three weeks after at least eight out of ten rats correctly indicated ≥80% of targets per session with ≤10% false alarms.
2.5.1 Test 1: generalization to novel specimens of pangolin and hardwood
To determine to what extent discrimination was driven by the odor profiles of the target items rather than memorization of specific training samples, we tested if the rats’ detection accuracy would generalize to novel target specimens. Pangolin 2 and Fresh Wood (at the three different volumes) replaced the six training targets during a single test session otherwise identical to Phase 3. During the rats’ very first encounter with novel specimens within that session we presented these first targets as blinds (mid-concentration), serving as probe trials. This test was followed by eight training sessions to assess whether reinforcing the novel specimens would result in rapid acquisition (“savings”, c.f. Rescorla, 1971) of target detection. During training sessions, one novel target sample (Pangolin 2 or Fresh Wood) and one training target (Pangolin 1 or Wood) served as blinds, alternating each day.
After completing Test 1, we tested if rats could detect Pangolin and Wood concealed with familiar and novel non-target items at varying ratios. The methods of these tests are described in the Supplementary Material, however, a combination of unplanned limitations precluded statistical analyses of the training and testing on ratios. We later repeated and statistically evaluated similar tests (mixtures) described further below.
2.6 Experiment 2: expanding wildlife targets
We sequentially trained nine rats to identify additional targets of Rhino and Ivory (based on Webb et al., 2020). Novel non-target items were introduced weekly throughout training phases and added to the sample library. Unless otherwise specified, all rats advanced through training stages as a group, according to the pre-determined criteria defined for each stage below.
2.6.1 Rhino horn training
Rats advanced to Discrimination Training when ≥7 rats correctly indicated ≥7 of nine samples per session during two consecutive sessions.
2.6.1.1 Discrimination training
Throughout, rats advanced to the next phase if, during two out of three sessions, ≥7 rats did not commit >5 false indications.
Phase 1 (30 samples): Nine targets (three each from Rhino 1, 2, and 3) were included in each session. Six non-target items were randomly selected from the library of materials the rats had previously encountered as well as one novel non-target item. Three samples of each non-target item were presented and rats advanced when they indicated ≥7 of nine targets.
Phase 2 (60 samples): Each session included 12 targets (one sample from each Rhino specimen serving as a non-reinforced blind) and 48 non-targets. Non-targets were comprised of two novel items and four familiar items (eight samples each), randomly selected weekly. Rhino 4 samples were introduced when rats indicated ≥10 of 12 targets. Blind samples were only from Rhino 1, 2, or 3 Rats advanced to Phase 3 when indicating ≥10 of 12 targets.
Phase 3 (100 samples): Initially, each session included 12 target samples (three samples of Rhino 1, 2, 3, 4, one blind sample each) and 88 non-target samples (eight samples each from nine familiar items randomly selected and two novel items identified weekly). When rats met the criterion of indicating ≥10 of 12 targets, Rhino 5 and 6 were introduced. Two samples of each target specimen were included during each session with four blind samples randomly selected (restricted to maximum one blind per Rhino specimen per session). Rats underwent retention testing when they indicated ≥10 of 12 targets.
2.6.1.2 Retention and generalization test 1
Ten sessions were conducted with 100 samples each. Adopting the procedures of Webb et al. (2020), previously learned wildlife targets (Pangolin and Wood) that rats had not encountered for 22 weeks were reintroduced within each test session as well as Rhino. Each session contained 88 non-targets following Phase 3 procedures and 12 targets (four samples each of Pangolin 1, Wood, and Rhino, including one blind each). Samples from a single Rhino specimen were included within each session, with Rhino 1 presented in Session 1, Rhino 2 in Session 2, and so on to effectively introduce novel Rhino specimens 7–9 during Sessions 7–9.
During Session 10, Pangolin 2 samples replaced Pangolin 1 (rats had prior experience with Pangolin 2 during Experiment 1) as well as one sample each of Rhino 8 and 9, and two samples from Rhino 7 (one blind). After this test, rats began Ivory training.
2.6.2 Ivory training
Indication training began with three samples each from Ivory 1, 2, and 3, and rats advanced following the same criteria as during Rhino training.
2.6.2.1 Discrimination training
Phase 1 and Phase 2 followed the same criteria adopted during Rhino Training, but with Ivory 1, 2, and 3 samples during Phase 1, and Ivory 4 introduced during Phase 2. Rats advanced to Phase 3 when they met the criterion as described in Rhino Training.
Phase 3: As with Rhino Training, this phase included 100 samples per session. When rats met the criterion on Ivory 1–4, samples from Ivory 5 and 6 were introduced. Unfortunately, two rats (Desmond, Thoreau) developed health issues and died and did not take part from Sessions 16 and 32 onward, respectively. Therefore, the new criterion was for six out of eight, or five out of seven rats to indicate ≥10 targets with ≤5 false alarms during two out of three consecutive sessions.
2.6.2.1.1 Glass vial test
To ensure the detection accuracy at the conclusion of training was driven by the scent of Ivory rather than the absence of scent, one novel non-target item was replaced by empty glass vials (the same glass vials used for presenting samples throughout training).
2.6.2.2 Retention and generalization test 2
As during Retention and Generalization Test 1, we re-introduced all previously learned wildlife targets (Pangolin, Wood, and Rhino) across sessions. Each session included 100 samples with 12 targets (four blind). Novel Ivory was introduced in Session 1. During Sessions 1–3, only two wildlife items were included per session. Session 1 included six Rhino samples (three each of Rhino 1 and 8, one of each blind) and six Ivory samples (three each of Ivory 1 and 8, one of each blind). Session 2 included six Pangolin samples (three each of Pangolin 1 and 2, one of each blind) and six Ivory samples (three each of Ivory 2 and 7, one of each blind). Session 3 likewise included six Ivory samples (three each of Ivory 3 and 6, one of each blind) and six Wood samples (two blind).
During Session 4 and 5, Pangolin, Rhino, Wood, and Ivory were presented per session (three samples of each of which two were reinforced and one was blind for each species). Sessions consisted of Wood, Pangolin 1 (two samples of which one was blind) Pangolin 2 (one sample), Rhino 4 (two samples of which one was blind) and Rhino 5 (one sample), and Ivory 4 (two samples of which one was blind) and Ivory 5 (one sample). During Sessions 6-9 we replaced Ivory 4 and 5 with Ivory 9 and 10 to evaluate rat accuracy to generalize to new specimens within the context of searching for all wildlife targets.
2.7 Experiment 3: assessing operational feasibility with hidden targets
Prior to Rhino and Ivory Training presented above, rats underwent four months of training during which we expanded the number of non-target items by introducing two novel items per week. Following this we investigated whether rats could detect Pangolin and Wood when they were concealed among known or novel non-targets. Concealed here meaning that these items were presented in the ALC as a mixture within a single glass vial. Later, following Experiment 2 of training to detect Rhino and Ivory, we conducted similar tests, investigating if rats were able to detect all four wildlife items when they had been concealed. The section below describes methods for both of these tests. In all cases, samples that had been used for mixtures were separated again after the session and non-target material was discarded while the wildlife target material was placed into direct sunlight for several hours to allow decontamination.
2.7.1 Simple concealment I of pangolin and hardwood
This took place after Experiment 1 and before training for Experiment 2 took place. After Experiment 1, rats were on break for approximately 7 months due to logistic and staffing constraints. Following their break, we repeated indication and discrimination training for Pangolin and Wood, as well as additional discrimination training during which we increased the total number of non-targets (described in more detail in the Supplementary Material). The 4 sessions described as baseline below, followed immediately after these sessions. Test 1 included targets concealed with familiar non-targets (i.e., items rats were explicitly trained to reject throughout their previous training), and Test 2 included targets concealed with novel non-targets (i.e., never explicitly trained to reject), both at 50:50 volume ratios (Table 1).
Non-target items were changed every test session. During any one session, the familiar and novel non-target item used was the same for all mixture samples presented in this session. Items were not included if they represented an assumed relevance such as preferred foods or conspecific/animal odors. Materials used for non-target mixture samples were discarded after use. For this reason, only materials easily obtained were used for mixtures. Any target material that had been used for a mixture was separated from any non-target material and placed in sunlight following the session for decontamination.
Baseline: Rats completed four sessions with only mixtures of non-targets (Table 1).
Test 1: Rats evaluated targets concealed with familiar non-targets over the course of two weeks. Alternating each session, rats completed four sessions with only Pangolin and four with only Wood (Table 1).
Test 2: Rats evaluated targets concealed among novel non-targets over 16 sessions. Sessions alternated between Pangolin and Wood, such that eight sessions included only Pangolin 1 and 2 and eight sessions included only Wood (Table 1).
2.7.2 Concealment of all wildlife targets
This took place after rats had been trained on Rhino and Ivory targets as described in Experiment 2.
Simple Concealment II (n=3 Sessions): Each session contained Ivory 1 and a second wildlife target (Pangolin 1, Wood, and Rhino 1 during Sessions 1, 2, and 3, respectively; Table 2). Wildlife items were presented in isolation or mixed at a 50:50 ratio with a familiar non-target.
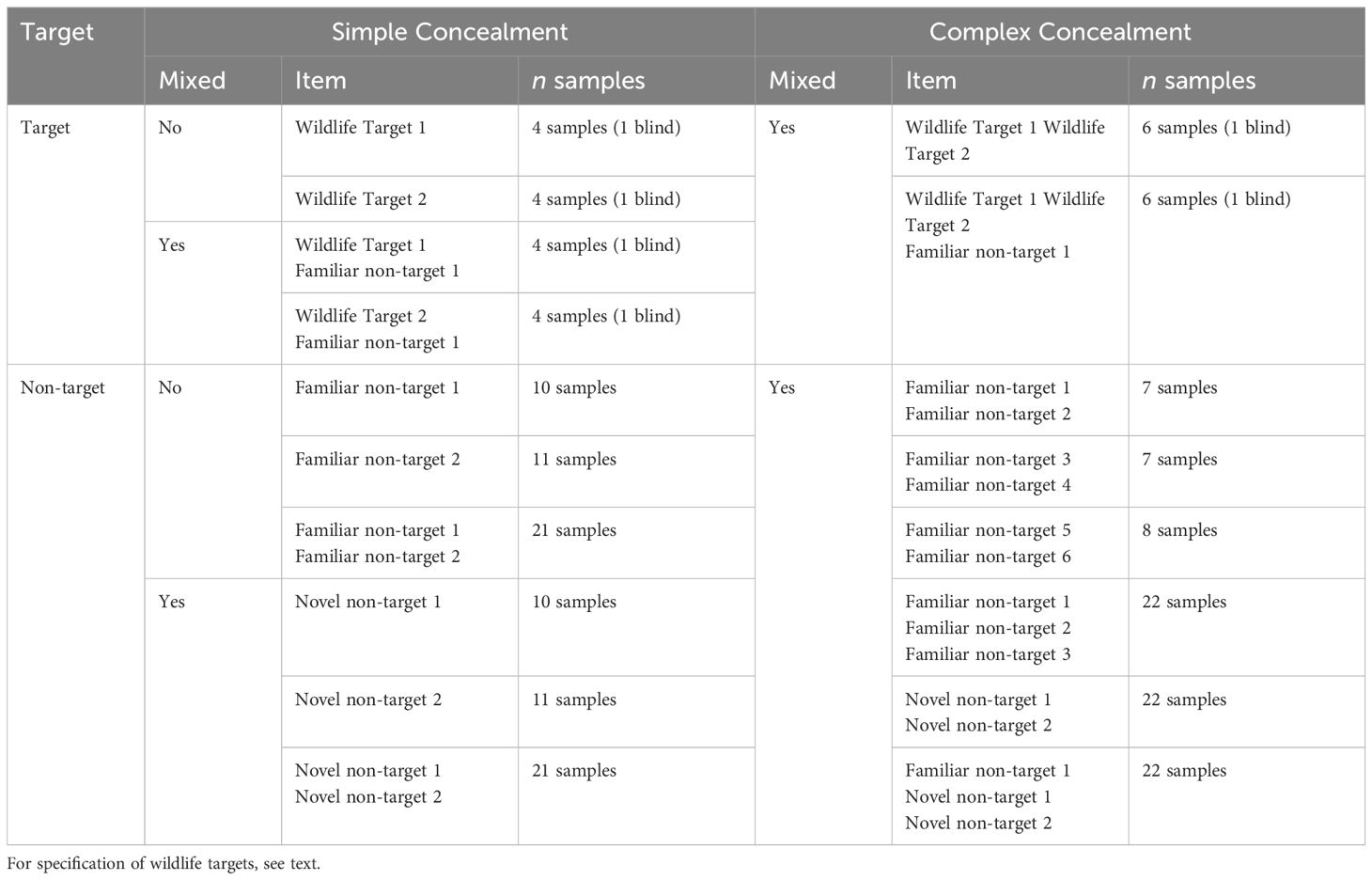
Table 2. Types of targets and non-targets presented during the Concealment Tests as well as number of each sample presented during each session.
Complex Concealment (n=6 Sessions): Wildlife items and non-target items were presented in mixtures only. Either as two different wildlife items mixed together, or as two wildlife items as well as a familiar non-target mixed together. During Session 1, rats encountered Ivory 1 and Pangolin 1, Session 2 included Ivory 1 and Wood, Session 3 included Ivory 1 and Rhino 1, Session 4 included Pangolin 1 and Wood, Session 5 included Pangolin 1 and Rhino 1, and Session 6 included Wood and Rhino 1.
2.8 Statistical analyses
Data were visualized and analyzed in R Statistical Software (v. 4.1.1), using the following packages: ggplot2 (Wickham, 2016), dplyr (Wickham et al., 2021), lme4 (Bates et al., 2015), emmeans (Lenth, 2021), and Rmisc (Hope, 2013). Significance was assigned at p ≤ 0.05. Further details are presented below for each experiment.
An indication (nose-poke duration exceeding the indication threshold) on a wildlife target was classed as a hit, while an incorrect indication of a non-target item was classed as a false alarm. To determine scent detection accuracy, we compared hits to false alarms at the conclusion of each training phase, which included the last three training sessions, or during tests. Generally, for all data presented in this manuscript, we analyzed whether there were significant differences between hits for specific target types (e.g., Pangolin vs. Wood or pure wildlife target vs. concealed wildlife target) or for individual specimens within specific target types (e.g., Pangolin 1 vs. Pangolin 2). If rats were presented with varying concentrations of targets, we assessed if rats hit all concentrations with comparable accuracy. When no differences were found between hits on all specimens within a target type, these were classed as a combined target category. During tests where rats were evaluating the targets most recently trained on as well as prior targets, we assessed whether they hit all of these wildlife targets similarly well to assess whether they retained previously trained targets to a high level. Where rats were presented with familiar as well as novel non-targets, we assessed whether there was a difference in false alarms between these two. If there was no difference between false alarms on familiar and novel non-targets, we assessed these as one category. If rats committed a significant difference of false alarms between the two, we assessed familiar and novel-non-targets separately. To evaluate trainer influence (e.g., cueing; c.f. Lewon et al., 2019), we compared hits on known, reinforced targets compared to hits on blind targets.
For all training, we present descriptive statistics for the final session of indication training and statistical analyses across the last three sessions of each discrimination training phase. Percentages reported are averages across rats and the associated Standard Error of the Mean (Mean ± SEM), unless otherwise noted. For discrimination training and tests, we used General Linear Mixed Effects Models (GLMERs) with a binomial error structure (indication or no indication) including rat ID as a random effect. In cases where models showed convergence warnings, we applied a bobyqa’ optimizer (maxfun=2e5). If this did not address the convergence warning, we used a Poisson error structure instead, as in some cases hits vs. false alarms were at extreme distributions. In cases where we encountered singularity issues, we removed the random effect and ran a General Linear Model (GLM) with a binomial error structure instead. Each of these cases are reported where applicable in the results. Where factors with multiple levels were indicated to have significant effects, we ran a Tukey post-hoc test with package ‘emmeans’ to make corrected pairwise comparisons.
3 Results
3.1 Experiment 1: can rats be trained to detect the scent of wildlife targets?
3.1.1 Indication training of pangolin and wood
Rats completed 20 sessions between 5th December 2017 and 8th January 2018. During the last session, rats detected 71.21% (± 7.1) of Pangolin 1 targets and 51.52% (± 5.3) of Wood targets on average.
3.1.2 Discrimination training with pangolin and wood
Rats completed 152 sessions between 9th January 2018 and 10th October 2018 (Phase 1: 66 sessions, Phase 2: 36 sessions, Phase 3: 50 sessions). From Session 26 of Phase 2, one rat (Kirsty) was removed from this study. Figure 1 shows average proportion and percentage of hits of Pangolin 1 and Wood targets, and false alarms on non-targets at the conclusion of each phase. Rats indicated significantly more targets than non-targets (all p < 0.001; Phase 1: z = 15; Phase 2: z = 21; Phase 3: z = 23.6). Further, rats indicated both target types significantly more compared to non-targets at the conclusion of each phase (all p < 0.001; Pangolin: Phase 1: z = 14.8; Phase 2: z = 18.3; Phase 3: z = 19.5; Wood: Phase 1: z = 12.9; Phase 2: z = 17.4; Phase 3: z = 20.3). At the conclusion of Phase 1 and Phase 3 rats indicated Pangolin significantly more compared to Wood (Phase 1: p < 0.001, z = −5.15, Phase 3: p = 0.002, z = −3.17). However, there was no significant difference between Wood and Pangolin at the conclusion of Phase 2 (p = 0.9, z = −0.13).
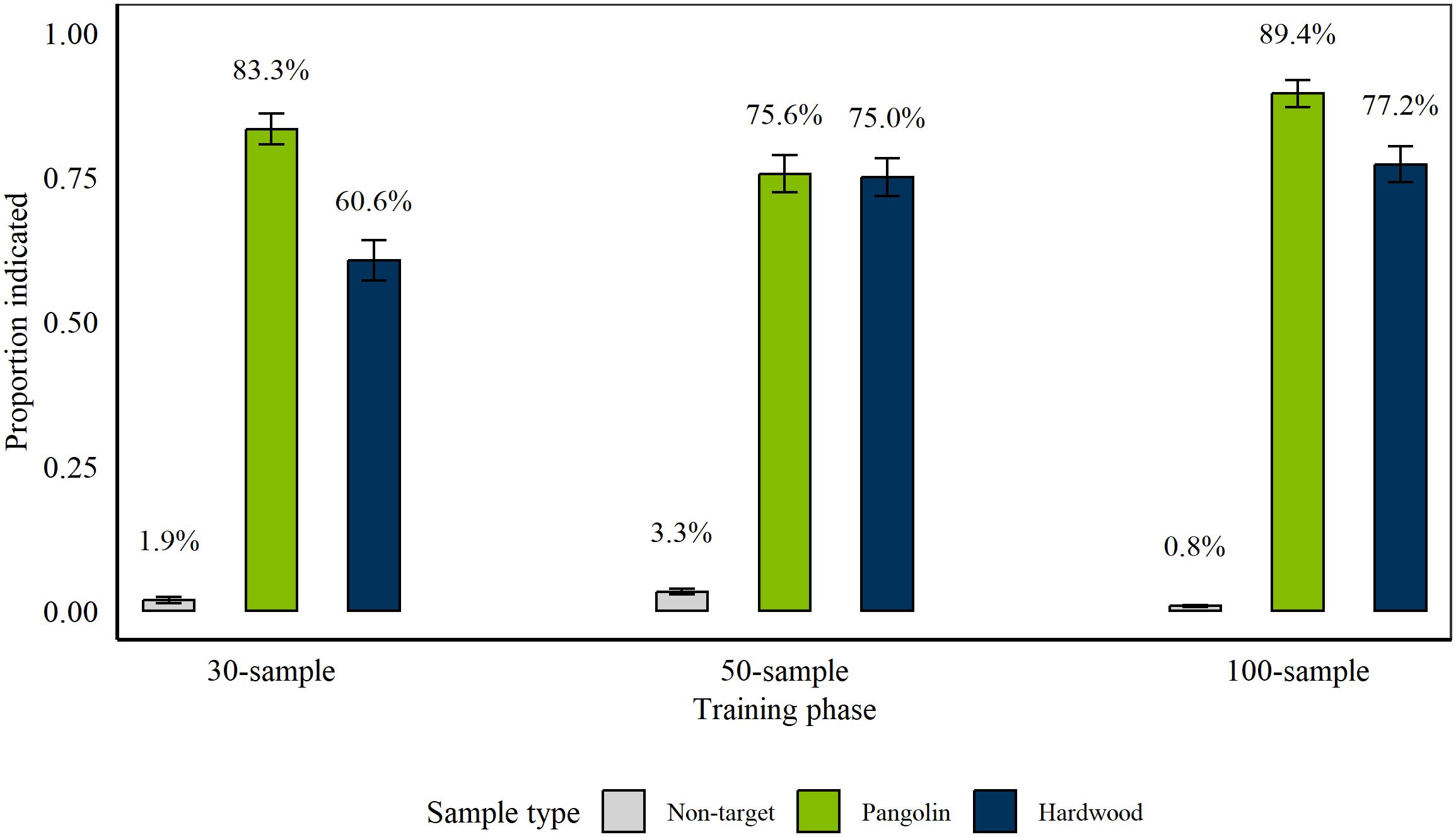
Figure 1. Average proportion of Pangolin (green) and Hardwood (blue) wildlife targets and non-targets (grey) indicated by rats, on average, during the last three sessions of each discrimination training phase. We tested n = 11 rats during 30-sample training (Phase 1) and n = 10 rats during 50- and 100-sample training (Phase 2 and 3). During each phase, we presented a total of 12 wildlife targets (six of each species) alongside 18, 38, or 88 non-target samples, respectively. Error bars are Standard Error of the Mean (SEM) with average percentage displayed above each bar.
There was no effect of target volume (0.5 g, 1 g, or 1.5 g) on rat indications of targets during any of the phases, as assessed by comparing a GLMER including the target volume to the respective Null Model (Phase 1: Pangolin: p = 0.19, X2(2) = 3.28; Wood: p = 0.92, X2(2) = 0.16; Phase 2: Pangolin: p = 0.19, X2(2) = 3.37; Wood: p = 0.17, X2(2) = 3.53; Phase 3: Pangolin: p = 0.31, X2(2) = 2.35; Wood: p = 0.23, X2(2) = 2.94).
At the conclusion of Phase 2, there was no significant difference between hits of blind compared to known Pangolin 1 targets (p = 0.75, z = −0.32; blind 73.33% (± 8.2), known 76% (± 3.5)), but rats indicated significantly more known Wood targets compared to blind ones (p < 0.001, z = −3.52; known 79.33% (± 3.3), blind 53.33% (± 9.3); GLMER using ‘bobyqa’ optimizer). Nonetheless, at the conclusion of Phase 3, there were no significant difference between blind and known targets for either target type (Pangolin 1: p = 0.91, z = 0.11; known 89.33% (± 2.5), blind 90% (± 5.6); Wood: p = 0.11, z = −1.58; known 79.33% (± 3.3), blind 66.67% (± 8.8)).
3.1.3 Generalization to novel pangolin and hardwood specimens
During the test session on 11th October 2018, we introduced Pangolin 2 and Fresh Wood. Rat indications of familiar and novel specimens during the test session and following training sessions are presented in Figure 2. Seven of ten rats correctly indicated the first (blind) sample of the novel Pangolin 2, while only one rat indicated the first (blind) sample of the novel Fresh Wood. Across the session rats indicated significantly more targets (60.83% (± 4.5)) overall, compared to non-targets (1.02% (± 0.5); p < 0.001, z = 13.05). Rats hit both Pangolin specimens similarly well (p = 0.06, z = −1.89) and Pangolin targets, overall, were indicated significantly more compared to non-targets (p < 0.001, z = 10.78). While rats indicated significantly more Wood targets than non-targets (GLM, p < 0.001, z = 10.96), this was driven by responses to the familiar Seized Wood rather than the novel Fresh Wood (GLM with Poisson error structure; p < 0.001, z = 12.06). There was no clear discrimination between novel Fresh Wood (with only a single sample indicated by one rat) and non-targets (p = 0.26, z = 1.13). Rats hit known and blind Pangolin targets similarly well (p = 1) but hit significantly more known than blind Wood targets (p = 0.02, z = −2.38; GLM) likely driven by the fact that all blind samples were of the novel Fresh Wood.
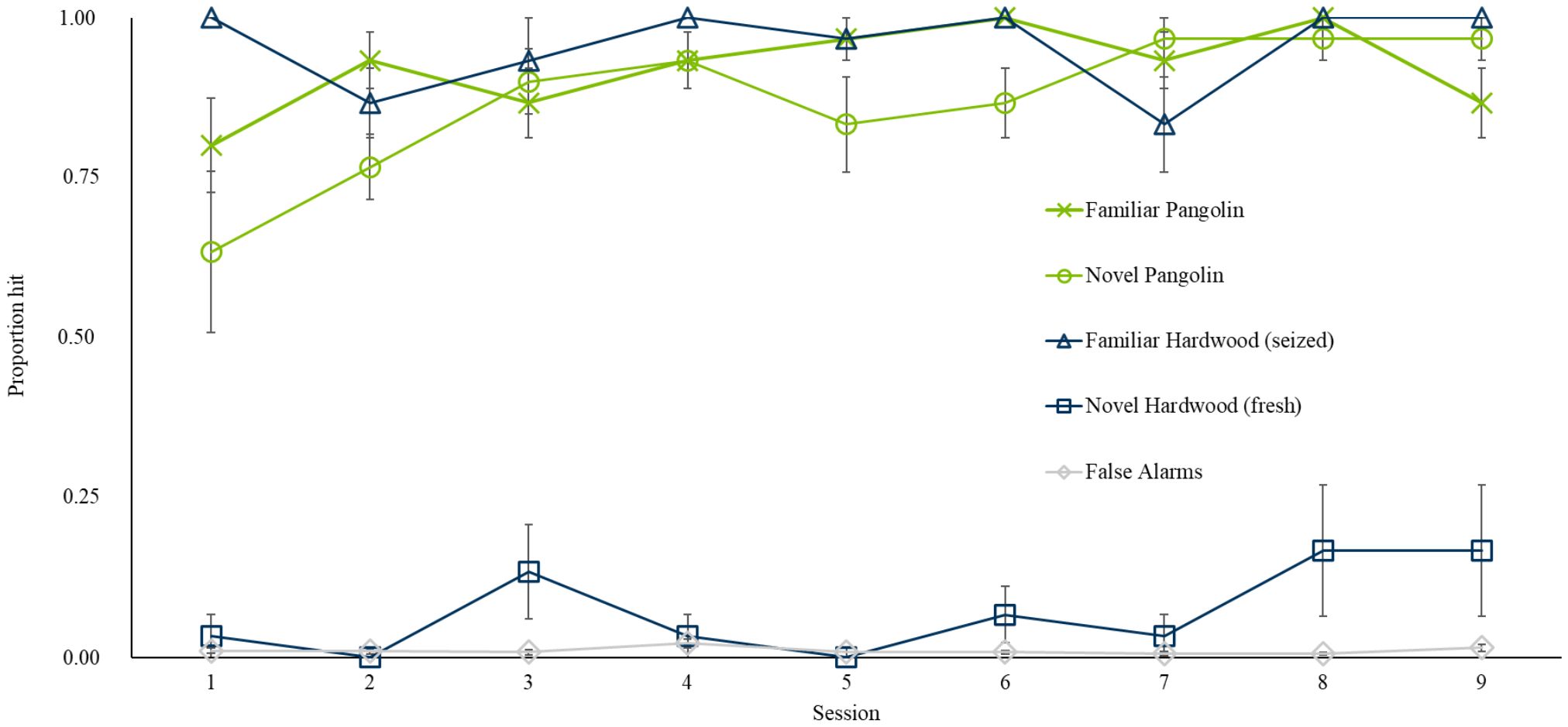
Figure 2. Average proportion of samples indicated by n = 10 rats, on average, among familiar (green line, cross markers) and novel Pangolin (green line, round markers), familiar (blue line, triangular markers) and novel (blue line, square markers) Wood, and familiar non-targets (grey line, diamond markers) during the Generalization to Novel Specimen Test (Session 1) and the following eight sessions of training (Sessions 2–9). Error bars are SEM.
Following the Generalization Test, rats completed an additional eight sessions of training with the novel specimens from 12th October 2018 and 22nd October 2018. Throughout these eight sessions, rats indicated significantly more targets compared to non-targets (p < 0.001, z = 39.08). There was no significant difference between hits on Pangolin 1 vs. Pangolin 2 (p = 0.31, z = −1.5) which were indicated significantly more compared to non-targets (p < 0.001, z = 31.2). Rats also indicated significantly more Wood targets vs. non-targets (p < 0.001, z = 30.55) but continued to hit significantly more Seized Wood vs. novel Fresh Wood (p < 0.001, z = −12.55). However, unlike during the test session, both Wood targets were indicated significantly more compared to non-targets when grouping all eight sessions (both p < 0.001, Seized Wood: z = 23.59; Fresh Wood: z = 7.61, assessed with a GLM). However, as shown in Figure 2, hits on Fresh Wood remained low overall.
3.2 Experiment 2: expanding wildlife targets
3.2.1 Indication training of rhino horn
Rat completed four sessions of indication training between 2nd February 2021 and 5th February 2021 and hit an average of 90.12% (± 4.7) of the nine targets during the last session.
3.2.2 Discrimination training of rhino horn
Figure 3 shows average detection accuracy across stages of discrimination training between 5th February 2021 and 8th March 2021. Rats completed training in 22 sessions, with ten sessions for Phase 1 and six sessions each for Phase 2 and 3. At the conclusion of each phase, rats indicated significantly more familiar non-targets, compared to novel non-targets (Phase 1: familiar: 8.73% (± 1.45), novel: 1.06% (± 0.75), p = 0.004, z = −2.9, Poisson error structure; Phase 2: familiar: 5.56% (± 0.5), novel: 1.62% (± 0.61), p = 0.002, z = −3.15; Phase 3: familiar: 4.99% (± 0.49), novel: 1.85% (± 0.65), p = 0.007, z = −2.72). We therefore assessed indications on targets compared to familiar and novel non-targets separately.
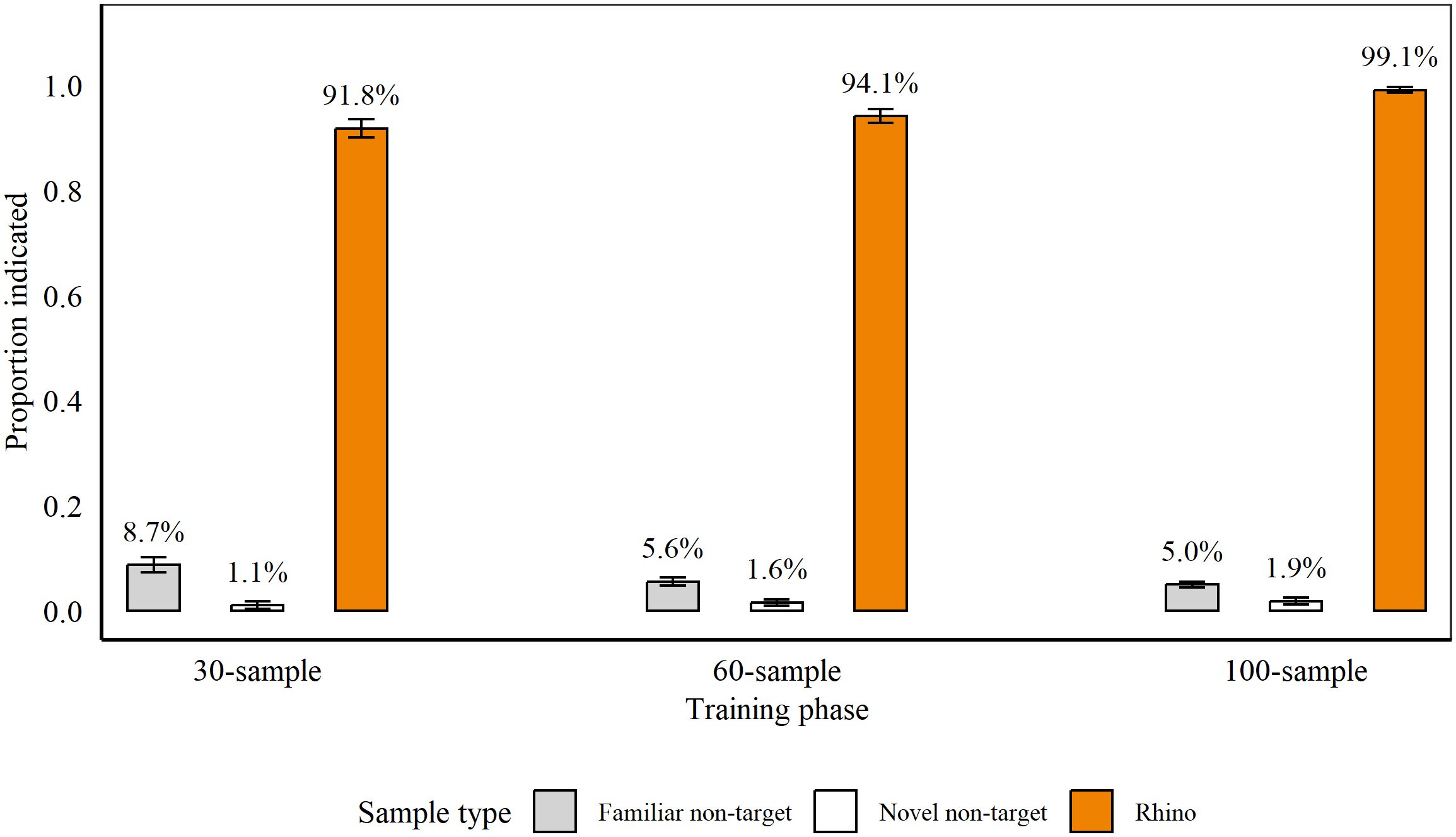
Figure 3. Average proportion of samples indicated by rats (n = 9), on average, during the last three sessions of each phase of discrimination training for Rhino horn. During 30-sample training, we presented nine targets and 21 non-targets, and during 60-sample and 100-sample training, we presented 12 targets and 48 or 88 non-targets, respectively. Average percentage of indications is indicated above each bar. Error bars are SEM.
Rats indicated significantly more Rhino targets vs. familiar non-targets (all p < 0.001; Phase 1: z = 13.95; Phase 2: z = 19.29; Phase 3: z = 12.9) and vs. novel non-targets (all p < 0.001 all GLMs; Phase 1: z = 9.29; Phase 2: z = 15.35; Phase 3: z = 12.69). Importantly, when blind samples were included during Phases 2 and 3, there were no significant differences between blind and known targets (Phase 2: p = 0.21, z = −1.26; Phase 3: p = 0.91, z = −0.12 (GLM)).
3.2.3 Retention test 1 of pangolin, hardwood, and rhino horn
Across ten sessions from 9th March 2021 and 23rd March 2021, we assessed rats’ retention of previously learned targets (Sessions 1–10) as well as generalization to novel Rhino specimens (Sessions 7–10). The average percentage of indications during each session as well as statistical results are presented in Table 3. Within each session, there were no significant differences between rats’ hits on targets (all p > 0.995, all GLMs) showing near-perfect retention of Pangolin and Wood targets from the first session onward. Further, this included generalization to novel Rhino specimens and hits on blind vs. known Pangolin, Wood, and Rhino targets.
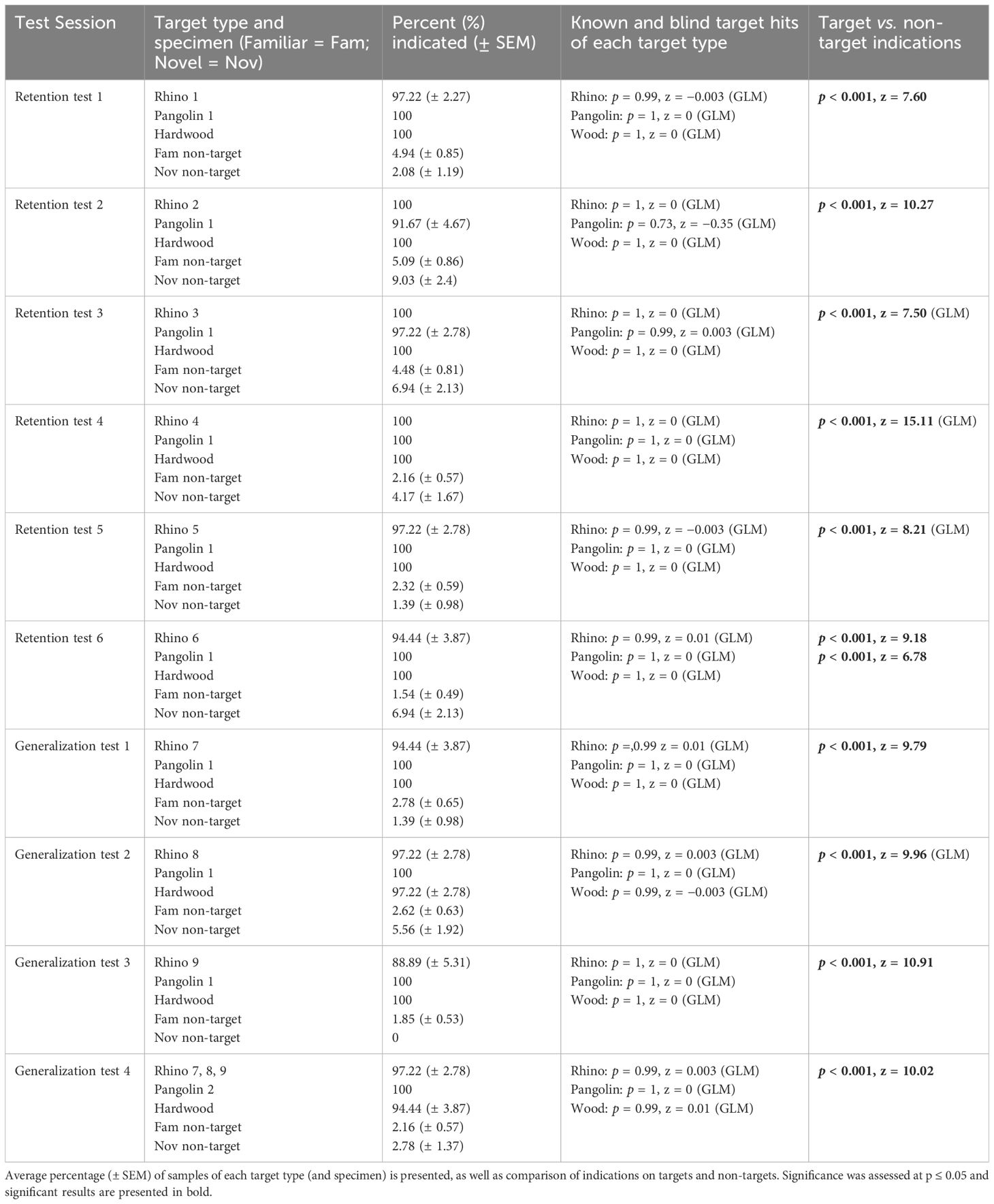
Table 3. Results for indications by African giant pouched rats, Cricetomys ansorgei, on wildlife targets and non-target materials during Retention Test 1.
During each session, there were no significant differences between indications on familiar vs. novel non-targets (all p > 0.06, Sessions 7–8 assessed with a GLM), except for Session 6 (p < 0.001, z = 3.4). Therefore, within each session non-targets were collapsed into one category to compare target vs. all non-targets. The exception was Session 6, in which target indications were separated compared to familiar and to novel non-targets. During each session rats indicated significantly more targets vs. non-targets.
3.2.4 Indication training of Ivory
Indication training required 24 sessions between 5th May 2021 and 9th June 2021 and rats hit an average of 85.19% (± 3.7) of nine ivory targets during the last session.
3.2.5 Discrimination training of Ivory
Detection accuracy at the conclusion of each training phase between 10th June 2021 and 10th November 2021 is presented in Figure 4. Rats completed 24 sessions in Phase 1, 11 sessions in Phase 2, and 67 sessions in Phase 3.
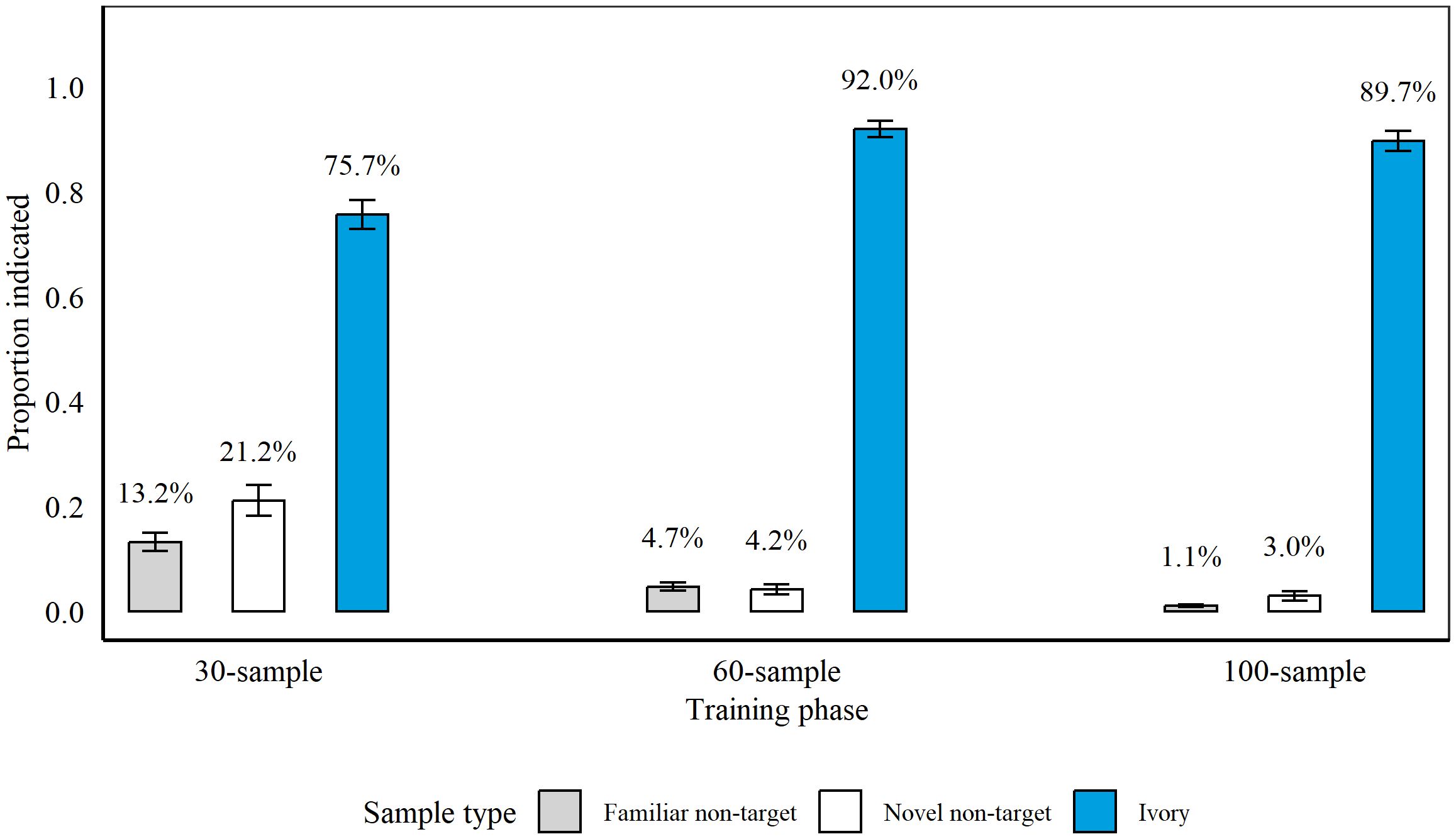
Figure 4. Average proportion of samples indicated by rats (n = 9 during 30- and 60-sample training and n = 8 during 100-sample training) during the final three sessions of each discrimination training phase. During 30-sample training, we presented nine targets and 21 non-targets, and during 60-sample and 100-sample training we presented 12 targets and 48 or 88 non-targets, respectively. The average percentage of samples indicated is presented above each bar. Error bars are SEM.
Similar to Rhino Discrimination Training, rats indicated significantly more Ivory targets compared to non-targets after each phase (all p > 0.001). Rats indicated significantly more novel non-targets compared to familiar non-targets during Phase 1 (p = 0.02, z = 2.42, GLM) and Phase 3 (p = 0.009, z = 2.61, GLM), but not during Phase 2 (p = 0.63, z = −0.48). Therefore, we assessed indications on targets vs. non-targets overall during Phase 2 (all p > 0.001; z = 21.65) but compared to familiar and novel non-targets individually during Phase 1 and 3 (all p > 0.001; vs. familiar non-targets: Phase 1: z = 13.95; Phase 3: z = 19.66; vs. novel non-targets: Phase 1: z = 10.44; Phase 3: z = 14.08).
On the introduction of blind samples during Phase 2, rats hit significantly more blind vs. known targets (p = 0.011, z = −2.53), but there were no significant differences in rats’ hits for known vs. blind targets at the end of Phase 3 (p = 0.47, z = 0.73, GLM).
3.2.5.1 Glass vial testing
During this test session on 11th November 2021, rats indicated significantly more targets (95.24% (± 0.32)) compared to non-targets (0.65% (± 0.32); p < 0.001, z = 8.70) as well as significantly more targets compared to familiar (0.6% (± 0.3); p < 0.001, z = 7.66) and novel (glass vial; 1.79% (± 1.78); p < 0.001, z = 6.83) non-targets. In fact, indications on familiar and novel non-targets were not significantly different (p = 0.73, z = 1.16; GLM). Hits on blind or known ivory targets were also not significantly different (p = 0.11, z = −1.6).
3.2.6 Retention and generalization test 2 of all wildlife targets
Descriptive and statistical results for this test from 12th November 2021 and 24th November 2021 are presented in Table 4. There were no significant differences between indications on novel and familiar non-targets during any of the sessions. Testing generalization to novel Ivory during Sessions 1–2 when novel Ivory Specimens 8 and 7 were introduced, respectively, we found no significant difference between hits on familiar and novel Ivory specimens. Testing retention of previously learned targets during Sessions 1–3, we found that rats indicated significantly more Rhino, Pangolin, and Wood targets compared to non-targets. Rats also hit significantly more Wood compared to Ivory targets (p = 0.05, z = 1.92), and significantly more Ivory compared to Rhino targets (p = 0.009, z = −2.62). There were no significant differences in hits on Ivory and Pangolin targets when Pangolin was first re-introduced (p = 0.7, z = −0.39).
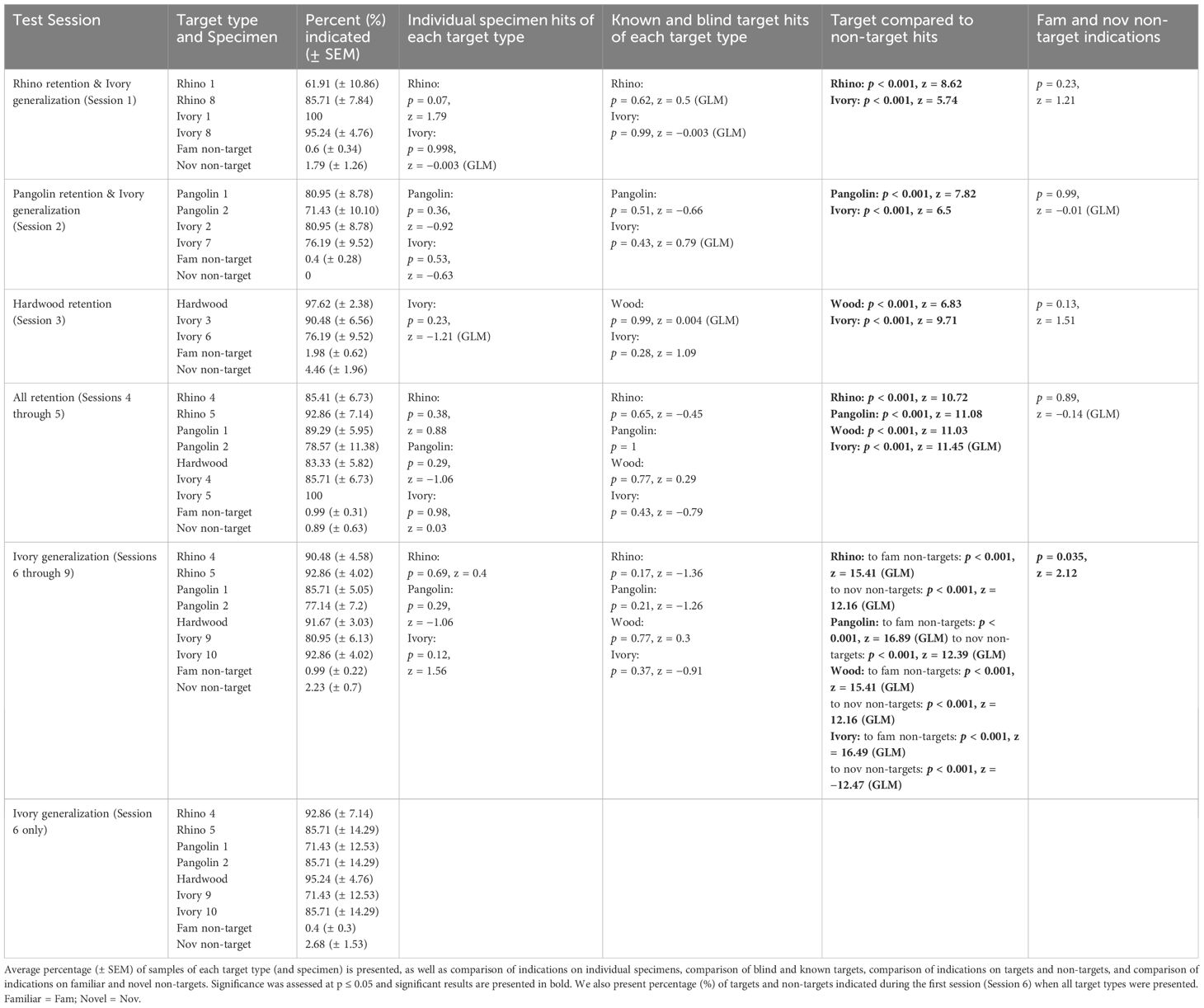
Table 4. Results for indications by African giant pouched rats, Cricetomys ansorgei, on wildlife targets and non-target materials during Retention and Generalization Test 2.
During Sessions 4–5 when all wildlife targets were presented (only familiar Ivory), rats hit significantly more Ivory compared to Wood (p = 0.003, z = 3.51, Tukey post-hoc) but there was no longer a difference between Ivory and Rhino (p = 0.7, z = 1.16; Tukey post-hoc; Figure 5). There were no significant differences between rats’ hits on specimens for any of the target types presented from more than one specimen (Sessions 1–5).
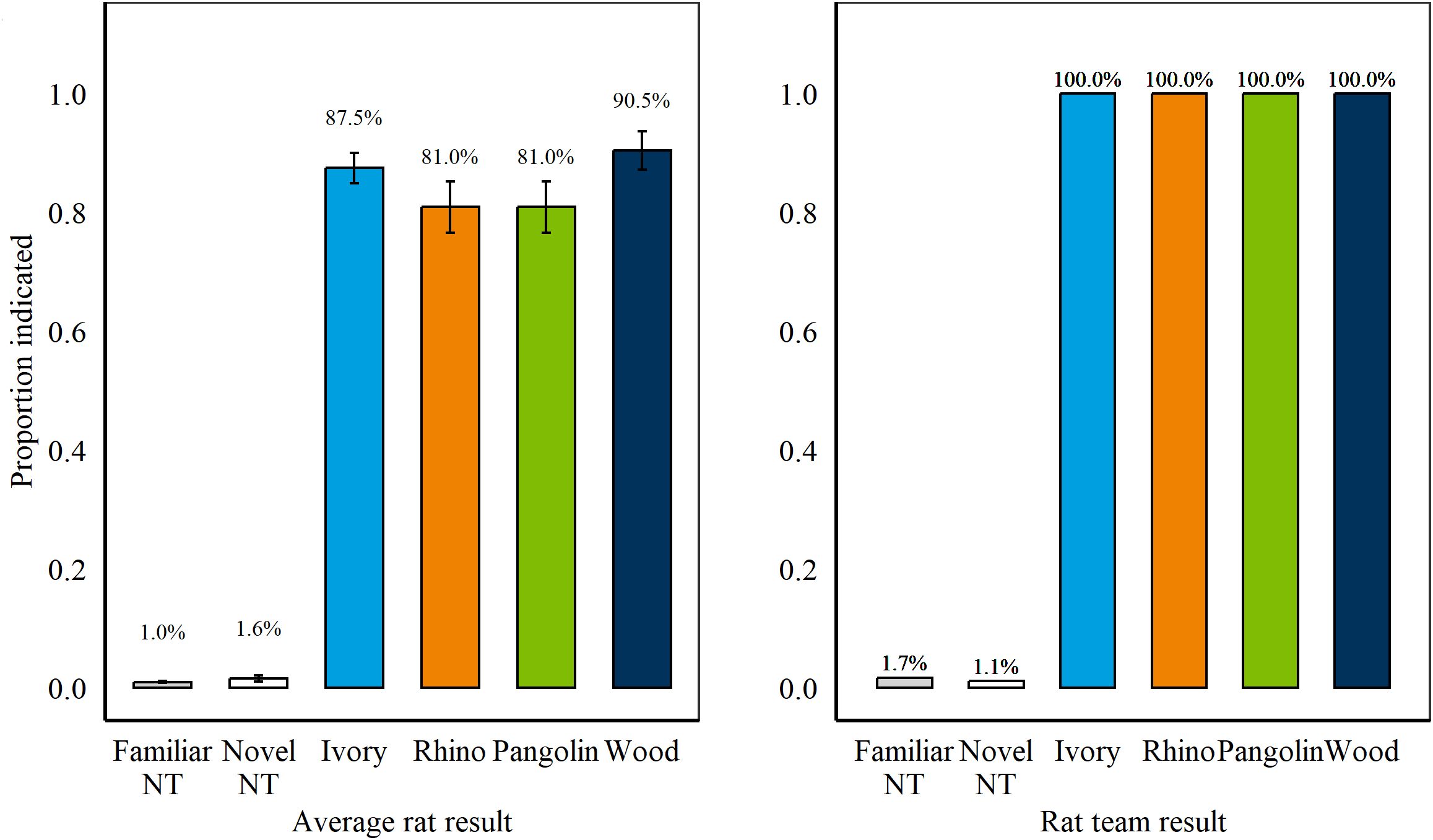
Figure 5. (Left) Average proportion of targets and non-targets (NT) indicated during Retention Test 2, Session 1–5 by n = 8 rats. Error bars are SEM. (Right) Results during Retention Test 2 if applying a two-rat cut-off where at least two rats were required to indicate the same sample for it to be considered hit. As a team, rats correctly indicated 100% of all wildlife targets presented across two sessions.
We further assessed results with a rat-team approach, where at least two individual rats had to indicate a specific sample for it to be considered a hit. Using this approach, rats would have detected 100% of all wildlife targets, boosting detection from average rat results (Figure 5) while committing 1.7% and 1.1% of false alarms on familiar and novel non-targets, respectively (compared to 1.0% and 1.6% for average rat results).
During Sessions 6–9, when introducing novel Ivory Specimens 9–10, rats indicated significantly more novel non-targets compared to familiar non-targets and we assessed all target hits vs. novel and familiar non-target indications separately. There were no significant differences between hits for specimens of each target type (comparing familiar and novel specimens), and rats hit all blind and known targets similarly well (Table 4). Specifically, Ivory specimen had no significant effect on whether rats hit the Ivory target or not (all p > 0.83). All targets were indicated significantly more compared to novel and familiar non-targets. Lastly, we re-ran the models including ‘Session’ as a fixed effect to ensure that grouping sessions together did not conceal an effect of learning over the consecutive sessions (all p > 0.1).
3.3 Experiment 3: assessing operational feasibility with hidden targets
3.3.1 Simple concealment I of pangolin and hardwood
These tests were carried out between 18th August 2020 and 1st October 2020, after discrimination training on Pangolin and Wood, before rats were trained on additional wildlife targets (Rhino and Ivory). A GLMER revealed significant differences in rats’ indications for the non-target types presented during baseline testing (n=4 sessions) when only non-target mixtures were presented and post-hoc tests revealed that rats indicated significantly more novel non-targets vs. familiar non-targets (p = 0.05, z = −2.57) and vs. mixtures of familiar non-targets (p = 0.02, z = −2.86). However, rats hit Pangolin and Wood similarly well (p = 0.33, z = −0.98). We therefore compared overall target indications to novel non-targets separately from all other non-targets (grouped, including mixtures).
Rats indicated significantly more targets compared to novel non-targets (p < 0.001, z = 12.35; GLM) and all other non-targets (p < 0.001, z = 15.01). There were no differences between hits for blind and known Pangolin (p = 0.997, z = 0.003; GLM) nor Wood targets (p = 0.08, z = −1.74).
During the first test of mixing targets with familiar non-targets, rats indicated significantly more novel non-targets than familiar non-target mixtures (p = 0.02, z = −2.84), and significantly more familiar non-targets than familiar non-target mixtures (p = 0.05, z = 2.59; Figure 6). We therefore assessed familiar non-target mixtures separate from other non-target categories in following analyses. No differences were found in rats’ hits on target types, including mixtures (all p ≥ 0.13). Rats indicated significantly more targets than mixtures of familiar non-targets (p < 0.001, z = 13.25) and all other non-targets (p < 0.001, z = 26.1). No significant differences were found in hits of blind and known Pangolin (p = 0.21, z = 1.26) and Wood targets (p = 0.992, z = 0.01; both GLMs).
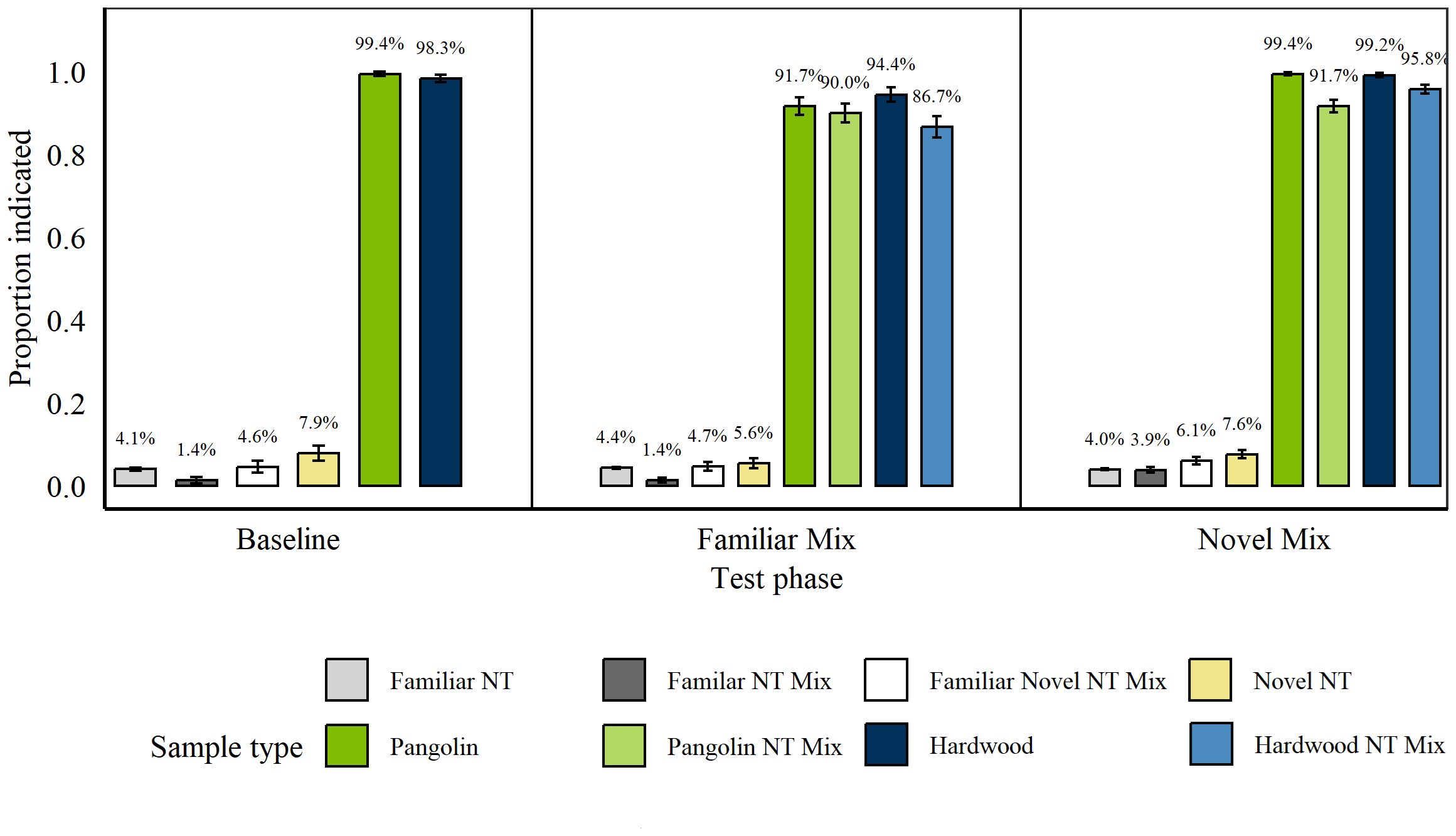
Figure 6. Average proportion of targets and non-targets (NT) indicated during the Simple Concealment Test for Pangolin and Hardwood by n =. Error bars are SEM. (Left) Results at the end of four sessions of baseline testing in which mixtures (Mix) contained either two familiar or one familiar and one novel non-target items. (Middle) Results during the first test session with a target (Pangolin or Hardwood) either presented in isolation or mixed with one familiar non-target item. (Right) Results during the second test session in which target items were presented in isolation or in mixtures with one novel non-target.
During the second test in which Pangolin and Wood targets were mixed with novel non-targets, rats indicated significantly more non-target mixtures of familiar and novel items (p = 0.04, z = −2.7) and significantly more novel non-targets compared to familiar non-targets (p < 0.001, z = −4.59). Further, rats indicated significantly more novel non-targets than mixtures of two familiar non-targets (p = 0.02, z = −3.01). Rats also hit the various target types significantly different. Pangolin samples were hit significantly more when they appeared alone than when mixed with a novel-non-target (p < 0.001, z = 3.84). There were no significant differences in hits of pure Pangolin and Wood (p = 0.97, z = 0.45) nor in hits between Pangolin and Wood targets mixed with a novel non-target (p < 0.001, z = −3.94).
Given these differences, we assessed the following as one category each: familiar non-targets and familiar non-target mixtures (“familiar non-targets”), novel non-targets and novel-familiar non-target mixtures (“novel non-targets”), pure targets, and targets mixed with novel non-targets (“target mixtures”). Rats indicated significantly more pure targets vs “familiar non-targets” (p < 0.001, z = 18.02) and vs. “novel non-targets” (p < 0.001, z = 15.33). Rats also indicated significantly more “target mixtures” vs “familiar non-targets” (p < 0.001, z = 36.3) and vs “novel non-targets” (p < 0.001, z = 22.5).
3.3.2 Simple concealment II of all wildlife targets
These tests were carried out between 25th November 20210 and 29th November 2021, after rats had been trained on the additional wildlife targets (Rhino and Ivory). There were no significant differences between rats’ false alarms for any of the non-target types during three sessions of the Simple Concealment II Test (all p > 0.07). We therefore grouped non-targets. Throughout all sessions rats indicated significantly more targets overall than non-targets (p > 0.001, z = 20.89) and significantly more targets and target mixtures than non-targets (all p < 0.001, Pangolin: z = 12.61; Wood: z = 11.95; Rhino: z = 11.14; Ivory: z = 18.11; Figure 7).
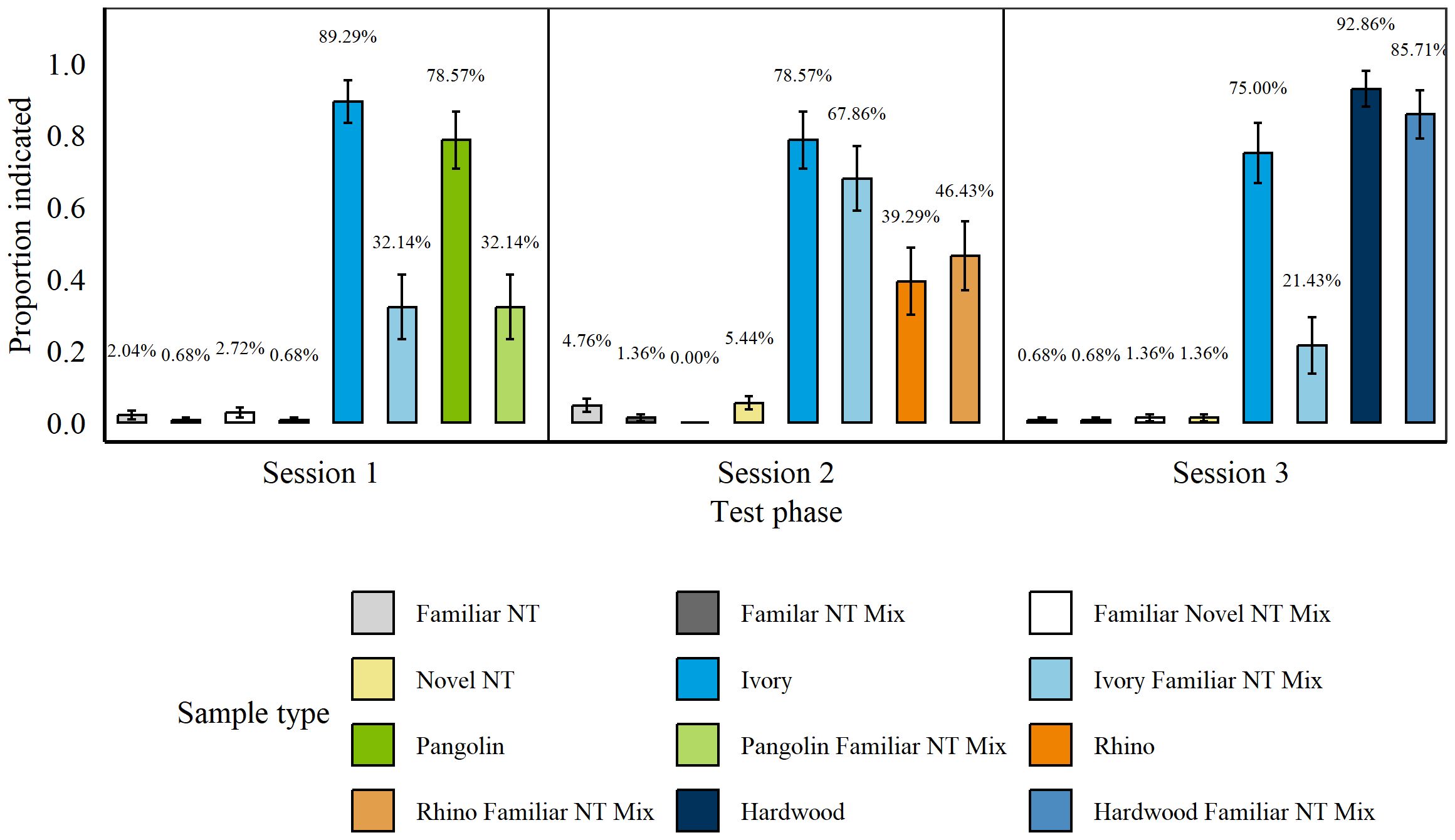
Figure 7. Average proportion of indications for targets and non-targets by n = 8 rats during each session of the Simple Concealment Test for all wildlife targets. Wildlife targets of each species were either presented in isolation, mixed with another species, or mixed with another species as well as a familiar non-target item. Each panel represents one session in order of execution/days. Error bars are SEM.
Rats hit significantly more isolated Pangolin compared to Pangolin mixed with a familiar non-target (p = 0.001, z = −3.23) and significantly more Ivory compared to Ivory mixed with a familiar non-target (p < 0.001, z = −5.11). Nonetheless, rats indicated both isolated and mixed Pangolin significantly more than non-targets (Pure: p < 0.001, z = 10.3; Mixed: p < 0.001, z = 7.3) and isolated and mixed Ivory significantly more than non-targets (Pure: p < 0.001, z = 15.51; Mixed: p < 0.001, z = 12.43). Rats hit isolated and mixed Wood and Rhino similarly well compared to these targets mixed with familiar non-targets (Wood: p = 0.39, z = −0.87; Rhino: p = 0.47, z = 0.73). However, while hits on Wood were relatively high, rats did not hit either of the Rhino targets above 50%, on average. There were no significant differences in rats’ hits for blind and known Pangolin (p = 0.28, z = −1.09), Wood (p = 0.61, z = −0.51), or Rhino (p = 0.35, z = −0.93; Poisson error structure). Rats did, however, hit significantly more known than blind Ivory (p = 0.002, z = −3.03).
3.3.3 Complex mixture concealment of all wildlife targets
These tests followed immediately after the Simple Concealment Tests, between 20th November 2021 and 7th December 2021. There were no significant differences in rats’ indications for the various non-target mixtures (all p > 0.07) and we therefore assessed non-targets as one category. Overall, rats indicated significantly more targets, whether mixed or not, compared to non-targets (p < 0.001, z = 28.44; Figure 8). Specifically, rat hits did not significantly differ between two targets mixed together and those two targets mixed together with a non-target (all p > 0.1), nor were there any significant differences between hits of blind and known targets, regardless of target type (all p > 0.44, all GLMs). The exception was whether the mixture of Pangolin, Wood, and a familiar non-target was presented blind or not, with rats hitting significantly more known vs. blind samples (known: 97.14% (± 2.86), blind: 57.14% (± 20.2), p = 0.011, z = −2.55, GLM).
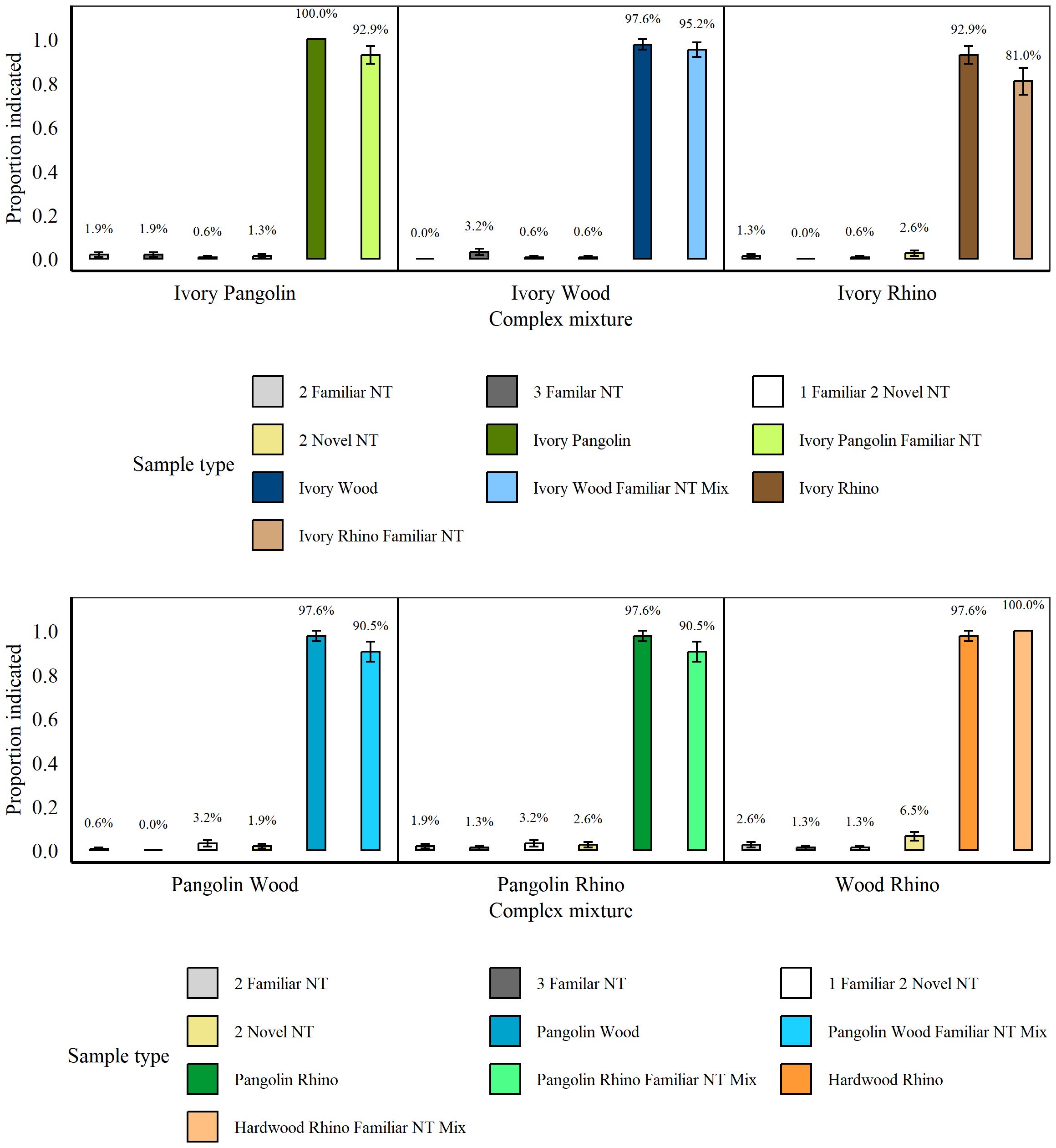
Figure 8. Average proportion of indications by n =8 rats for mixtures containing either two or three non-targets (NT), two different targets (see separate panels for each combination of species), or two targets along with a familiar non-target during the Complex Concealment Test. Panels are presented in same sequence as test sessions. Error bars are SEM.
4 Discussion
Our results demonstrate proof-of-principle that African giant pouched rats can be trained to detect commonly smuggled wildlife species. We successfully trained eight rats to discriminate four wildlife targets from a total of 146 non-target substances, some of which were identified as items commonly used to either mask the odor or otherwise conceal the presence of wildlife contraband in shipping containers. In addition, these results provide additional evidence that our rats can detect multiple targets simultaneously (Webb et al., 2020) and validated a robust training protocol which could be used for various scent detection applications. During the final testing session of Retention Test 2, when all four target types were presented, each rat evaluated 12 targets and 88 non-targets. On average rats detected 85.71% (n = 71) of 84 targets, while only falsely indicating 1.3% (n = 8) out of 616 non-targets. Moreover, rats took an average of 13 minutes to evaluate 100 samples during this session. Overall, the results indicate that rats can efficiently and accurately detect multiple wildlife targets.
While the average rat discriminated targets from non-targets, we also considered results when adopting a rat-team strategy. Here, a sample was only considered as a true hit or false alarm if it had been indicated by a specific number of rats. This is a similar method described by APOPO in other scent detection tasks (Mahoney et al., 2012). By applying a 2-rat threshold during five sessions of Retention and Generalization Test 2 (Session 1–5), we demonstrated improved accuracy in finding 100% of the target samples while only minimally increasing false alarms from 1.3% to 1.4%. Thus, the rat-team approach holds remarkable promise for further enhancing wildlife detection. These results also suggest that through careful selection of rat teams and consideration of individual rat performance metrics, we may optimize the teams and reduce the overall number of rats required to be deployed. In dogs, recent studies found that retention of trained targets decreased if the target was not encountered for 12 months (Lazarowski et al., 2021; Waggoner et al., 2022). In the present study, rats displayed perfect retention of Pangolin and Wood during the first Retention Test (five months since they last experienced those targets). Similarly, the rats displayed perfect retention during Retention Test 2 despite not having encountered Pangolin, Wood, or Rhino for eight months. Although we did not test retention after a 12-month period, these findings suggest that rats’ cognitive performance in retention of targets is on par with that of dogs. Further, Williams and Johnston (2002) have shown that dogs became faster at learning new targets with experience, a result mirrored by our rats previously (Webb et al., 2020) and during their training on Rhino, but not Ivory. Thus, additional research is required to determine if differences between Ivory and Rhino may be due to the presumed differences in odor volatility.
In this study, we first trained rats to successfully discriminate wildlife target samples from non-target items, before conducting generalization tests with new target specimens. During detection tasks, scent detection animals are required to process a large amount of olfactory information and respond to new and changing stimuli. An essential mechanism by which animals do this is stimulus generalization, a phenomenon that allows organisms to categorize perceptually similar stimuli and thus elicit a similar behavioral response (in our case the indication response; Moser et al., 2019; Oldenburg et al., 2016). A rat’s perception of each odor can therefore be shaped by both, discrimination and generalization (Moser et al., 2019). This, in turn, affects how a rat detects odors and responds to variations in a target odor (e.g., different Pangolin specimens) while discriminating it from non-target odors (Moser et al., 2019). Our results show that rats generalized to novel specimens of trained targets, which improved with experience. Rats excelled at detecting the novel Pangolin specimen across the generalization tests, implying that they indicate novel specimens other than those encountered during their training which would be the case with illegally trafficked items. While rats were trained on samples from a single pangolin, they generalized immediately to a novel specimen, mirroring closely what has been observed in dogs (Oldenburg et al., 2016). However, rats did not generalize well to the novel untreated fresh Wood (compared to the treated Seized Wood). It may be that the processing of the Wood fundamentally altered its odor profile, hindering generalization to non-treated samples. In addition, storage of fresh Wood was a challenge given the high humidity settings and resulted in Wood growing moldy within a short amount of time. There may also have been a difference due to the fresh Wood having been obtained more recently hence resulting in a difference in how long this sample had been stored in our laboratory and how often it had been handled, compared to the remainder of target and non-target materials. This highlights the need for adequate training materials when teaching animals to detect a specific wildlife species and the importance of testing closely related materials. Still, these results suggest the rats will generalize across different samples from one target species but the efficacy of this will depend on the species and how material has been treated.
The duration to train rats on specific wildlife species varied. While initial training on Pangolin and Wood took 172 sessions to complete, rats mastered the following training on Rhino horn within only 22 sessions. Ivory training, in comparison, required a total of 126 sessions before reaching detection accuracy similar to that of the other species. However, this may also have been a factor in detection of mixtures of Ivory during the Simple Concealment Test II being better compared to that of Rhino, as rats had more training experience with Ivory. Nonetheless, during the Complex Concealment Tests, which were conducted immediately after the Simple Concealment, detection accuracy for Rhino within mixtures was similar to all other target mixtures. It is not clear whether this was driven by rapid re-acquisition of Rhino or the additional other wildlife species within the mixture.
The concealed mixture tests revealed rats could successfully detect wildlife targets hidden in familiar and novel non-targets. In line with our previous work (Webb et al., 2020) and following additional training on an increased variety of novel non-target materials (see Supplementary Material), we did not see a pronounced novelty effect that has been previously described in rats and canines. In fact, across training there were relatively few differences between novel and familiar non-targets. The absence of a strong novelty effect is a major strength and suggests rats will ignore the wide range of novel non-targets they may encounter in the field. We did observe differences in rats’ ability to detect some isolated wildlife targets compared to these targets mixed with a familiar non-target, which rats have explicitly been trained to reject. When targets were mixed with familiar non-target items, there was a so-called generalization decrement. It is possible that rats were less able to detect the wildlife target within the mixture or that competing excitatory and inhibitory associations demonstrating summation led to a generalization decrement (Brandon et al., 2000). However, applying the rat team strategy and a cut-off of two rats, we again achieved 100% detection of wildlife targets (compared to an overall average of 86.1% for the average rat) and maintained false alarms at 1.7%.
In dogs, training on single target odors results in diminished accuracy with mixtures compared to dogs trained on mixtures (Fischer-Tenhagen et al., 2017). Indeed, dogs trained on mixtures were able to detect the isolated target (Gazit et al., 2021). A recent study investigated the ability of two detection dogs to find pangolin and ivory when concealed among other products (Narayanasamy et al., 2023). The authors observed that dogs performed better at detecting pangolin compared to ivory when concealed (Narayanasamy et al., 2023). They further observed a difference between the two dog breeds in their performance and noted that personality plays an important factor in the selection of individuals. While APOPO’s rats differ in their personality traits (Broecke et al., 2023), we have no evidence of these affecting the performance of any of APOPO’s operational rats across active programs. As such, we suggest that rats may offer an additional advantage of being trained for all targets regardless of personality. We further observed that rats were better at detecting wildlife targets during the complex mixture test compared to the simple mixture test II. This may have been driven by the added experience over time with mixtures in general by the time the rats were tested on complex mixtures, or by more of their trained target odors being present in a complex mixture. While we are unable to comment on the exact reason for this result, this may be beneficial in an operational environment where, more often than not, a variety of wildlife species may be trafficked in a single container (Zavagli, 2021).
4.1 Limitations
Despite numerous strengths of this study, including the presentation of multiple specimens and robust generalization testing, there are several key limitations. First, our study provides proof-of-principle rats can be trained to detect commonly trafficked wildlife. However, training and testing took place in a controlled laboratory environment. This is ultimately not reflective of environments in which wildlife is commonly trafficked or screened by scent-detection animals. Further research is required to assess how rats can operate in real-world environments and whether they maintain their ability to detect wildlife under the less-controlled conditions.
Second, scent detection dogs and rats generalize between different sources or variations of target odors although we only found limited work that has explored how the number of trained targets influences generalization. One study suggested dogs can detect six variations of a target odor after being trained on only two of them (Oldenburg et al., 2016). Understanding how much variation in targets should be offered to ensure generalization to the complete range that may be experienced in an operational environment is a critical aspect of training scent detection animals. Although we made every effort to present rats with a range of specimens, we have yet to assess whether this is sufficient for them to generalize to not only additional specimens but also varying quantities. As wildlife is commonly trafficked in large volumes, our training volumes, limited by the number of samples we had access to, may not have been representative of these. Therefore, future research should consider presenting rats not only with varying specimens of a species but also with varying concentrations of each specimen. Even with small sample quantities, such variation should, theoretically, lead to better generalization ability of rats.
Third, in line with the previous point, samples were obtained at different points in time throughout the experiment. For example, rats were trained on pangolin scales obtained in 2017 until the end of the experiments in 2021. However, as novel non-target materials were introduced weekly, this meant that these items were in use and in storage for shorter periods of time. Such differences in storage duration and handling frequency over the years may also have affected the scent of samples. Nonetheless, while new non-targets and perishable items such as leaves, peanuts, or rodent pellets were replaced when needed, we also utilized non-perishable items such as washing powder, plastic hair wig, and electric cables which remained the same throughout (i.e., the same duration as Pangolin and Wood). When samples were used for concealment tests, we separated the samples immediately after the session and placed wildlife materials into direct sunlight for several hours before storing it separately from the remainder of the stock until the experiments were finished. We are unable to confirm whether this method successfully removed all contamination. Although this is a limitation in the laboratory setting, it is important to note that, in operational environments, wildlife targets will most likely always occur among non-target items for concealment. Therefore, presenting rats with these mixtures and training them to be target-seeking rather than novelty rejecting supports the operational need.
Fourth, the results related to Ivory should be interpreted with caution. Upon completion of the research presented here, we discovered that Ivory and Rhino samples were stored in the same safe at our laboratory due to logistical constraints. We further observed that a separate cohort of rats who had been trained to detect Rhino, but not Ivory, spontaneously indicated Ivory samples when presented for the first time. The shared storage and behavior of Ivory-naïve rats strongly suggest our Ivory may have become contaminated by Rhino-associated scent/s. Therefore, we are unable to conclude that rats can detect the scent of Ivory. Nonetheless, even if rats did, in fact, detect the contamination of Rhino on the Ivory samples, it lends insight into the sensitivity of C.ansorgei’s olfaction to detect slight contamination of a familiar target odor.
4.2 Application for conservation
Current screening methods for illegally trafficked wildlife are expensive and time-consuming (Congressional Budget Office, 2015; Buffler and Tickner, 2010). With the results presented here, we propose that African giant pouched rats could offer a cost-effective addition to these current methods. Although final training cost of wildlife detection rats remains to be determined upon completion of developing this program, we draw this conclusion based on evidence of existing established APOPO programs in mine and Tuberculosis detection. For example, there are no up-front purchase costs of rats unlike in many scent detection dogs, the housing and maintenance cost of APOPO’s rats is extremely low and their dietary requirements can be met by locally available produce rather than requiring expensive custom-made food. Further, unlike many dogs, rats will work with any trained handler allowing for more flexibility in staff deployment and no loss of deployment opportunities linked to (sick) leave of handlers. As such, rats could be deployed alongside other screening methods, complementing existing tools or targeting different areas. This might, for example, be possible where rats screen specific items (e.g., containers) for illegally trafficked wildlife, while canines focus on searching larger open areas.
To increase operational efficiency and reduce the potentially expensive screening of falsely indicated items, deploying rats as teams may further bolster their value as a screening tool (Mahoney et al., 2012). In addition, we have shown that rats can be trained on multiple targets and that rat team strategies can increase sensitivity (ability to detect a target). In future, there is potential to form rat teams of rats with high sensitivity (ability to identify a target) or specificity (ability to reject a non-target) for individual wildlife species, which can further bolster detection. Although there were mixed results when presenting rats with targets concealed with multiple non-target items, these were still significantly more likely to be indicated by rats compared to non-targets. As wildlife is commonly concealed among legal cargo (Zavagli, 2021), training rats on such mixtures is essential for operational relevance.
Next steps include further research into how rats would operate in areas such as seaports to detect trafficked wildlife. This will include development of deployment methods and training rats to detect wildlife targets in environments closely mimicking operational settings. Operational trials will determine whether developed methods are sufficient in bringing APOPO’s wildlife detection rats from a controlled environment into operations.
5 Conclusion
IWT is a multi-faceted threat to biodiversity, economies, and human health and current methods to curtail IWT are limited by high cost and operational demands. The results presented here provide substantial evidence for the development of scent detection rats as a tool to fight the illegal wildlife trade and add to existing methods. Taken together, we show that African giant pouched rats can be trained to detect more than one wildlife species while ignoring non-target materials. We further found that rats generalized between different specimens of the same species, which improved with experience. However, the rats did not generalize between trained Wood which had been treated prior to seizure and pieces of fresh cut Wood. Overall, this study demonstrates the potential usefulness of employing African giant pouched rats for the detection of wildlife contraband, although further research is needed to establish deployment feasibility.
Data availability statement
APOPO will not make the detailed list of non-target materials readily available due to the sensitivity of details on specific masking materials rats have been trained on. This is to ensure every precaution is taken to not share this information with trafficking networks. A randomized version of the data can be made available on request. Requests to access the datasets should be directed to www.apopo.org.
Ethics statement
The animal study was approved by Institutional Committee for Research Involving Animals of the Sokoine University of Agriculture, Morogoro, Tanzania. The study was conducted in accordance with the local legislation and institutional requirements.
Author contributions
IS: Conceptualization, Data curation, Formal analysis, Investigation, Methodology, Project administration, Supervision, Visualization, Writing – original draft, Writing – review & editing. EW: Conceptualization, Data curation, Investigation, Methodology, Project administration, Writing – original draft, Writing – review & editing. SM: Conceptualization, Investigation, Project administration, Writing – review & editing. AM: Investigation, Project administration, Writing – review & editing. WM: Investigation, Project administration, Writing – review & editing. AI: Investigation, Project administration, Writing – review & editing. MSa: Investigation, Project administration, Writing – review & editing. DV: Investigation, Writing – review & editing. MSc: Conceptualization, Funding acquisition, Investigation, Methodology, Project administration, Supervision, Writing – review & editing. AD: Conceptualization, Funding acquisition, Writing – review & editing. KB: Conceptualization, Funding acquisition, Writing – review & editing. HD: Conceptualization, Funding acquisition, Writing – review & editing. CC: Conceptualization, Funding acquisition, Methodology, Writing – review & editing. CF: Conceptualization, Funding acquisition, Investigation, Methodology, Project administration, Supervision, Writing – review & editing.
Funding
The author(s) declare financial support was received for the research, authorship, and/or publication of this article. This project was funded by the U.S. Fish and Wildlife Services (USFWS), the Illegal Wildlife Trade Challenge Fund of the United Kingdom (UK), US AID, the “Partnership against Poaching and Illegal Wildlife Trade”, implemented by GIZ on behalf of the German Federal Ministry for Economic Cooperation and Development (BMZ) and the German Federal Ministry for Environment, Nature Conservation and Nuclear Safety (BMU), as well as the World Conservation Network’s Pangolin Crisis Fund.
Acknowledgments
We thank the donors who have supported this project throughout. Special thanks to the late Dr Georgies Mgode who played a crucial role in obtaining access to training samples and the late Adam Pires who managed the EWT’s Wildlife Trade Program; they are dearly missed by their colleagues We are grateful to APOPO’s trainers and researchers who carried out training sessions throughout the years: Haylee Ellis, Sezari Koba, Karim Chang’aa, Senga Sagang’ha, Nassoro Amani. This project was made possible through support from the Sokoine University of Agriculture (SUA) as well as the Tanzanian Wildlife Management Authority (TAWA) and the Tanzanian Wildlife Research Institute (TAWIRI) who provided training material. The authors also express their gratitude to the rats who have participated in this project and the reviewers for their valuable feedback.
Conflict of interest
The authors declare that the research was conducted in the absence of any commercial or financial relationships that could be construed as a potential conflict of interest.
Publisher’s note
All claims expressed in this article are solely those of the authors and do not necessarily represent those of their affiliated organizations, or those of the publisher, the editors and the reviewers. Any product that may be evaluated in this article, or claim that may be made by its manufacturer, is not guaranteed or endorsed by the publisher.
Supplementary material
The Supplementary Material for this article can be found online at: https://www.frontiersin.org/articles/10.3389/fcosc.2024.1444126/full#supplementary-material
References
‘t Sas-Rolfes M., Challender D. W. S., Hinsley A., Verissimo D., Milner-Gulland E. J. (2019). Illegal wildlife trade: scale, processes, and governance. Annu. Rev. Environ. Resour. 44, 201–228. doi: 10.1146/annurev-environ-101718-033253
Bale R. (2019). “Poaching is sending the shy, elusive pangolin to its doom,” in National geographic magazine (National Geographic Society, Washington, DC). Available at: https://www.nationalgeographic.com/magazine/article/pangolins-poached-for-scales-used-in-chinese-medicine.
Bates D., Maechler M., Bolker B., Walker S. (2015). Fitting linear mixed-effects models using lme4. J. Stat. Software 67, 1–48. doi: 10.18637/jss.v067.i01
Brandis K. J., Meagher P. J. B., Tong L. J., Shaw M., Mazumder D., Gadd P., et al. (2018). Novel detection of provenance in the illegal wildlife trade using elemental data. Sci. Rep. 8, 15380. doi: 10.1038/s41598-018-33786-0
Brandon S. E., Vogel E. H., Wagner A. R. (2000). A componential view of configural cues in generalization and discrimination in Pavlovian conditioning. Behav. Brain Res. 110, 67–72. doi: 10.1016/S0166-4328(99)00185-0
Braun B. (2013). Wildlife detector dogs- A guideline on the training of dogs to detect wildlife in trade (Germany: WWF). Available at: https://www.traffic.org/site/assets/files/2272/wwf-wildlife-detector-dogs-guidelines.pdf.
Broecke B. V., Abraham L., Webb E. K., Schneider M., Fast C. F. (2023). Can animal personalities save human lives? Evidence for repeatable differences in activity and anxiety in African giant pouched rats (Cricetomys ansorgei). Appl. Anim. Behav. Sci. 259, 105848. doi: 10.1016/j.applanim.2023.105848
Buffler A., Tickner J. (2010). Detecting contraband using neutrons: Challenges and future directions. Radiat. Measurements 45, 1186–1192. doi: 10.1016/j.radmeas.2010.06.010
Campbell B. M., Angelsen A., Cunningham A., Katerere Y., Sitoe A., Wunder S. (2007). Miombo woodlands – opportunities and barriers to sustainable forest management (Bogor, Indonesia: CIFOR). Available at: http://www.cifor.cigar.org/miombo/docs/Campbell_BarriersandOpportunities.pdf.
Challender D. W. S., Waterman C., Baillie J. E. M. (2014). Scaling up pangolin conservation (London, UK: IUCN SSC Pangolin Specialist Group Conservation Action Plan. Zoological Society of London). Available at: https://www.pangolinsg.org/wp-content/uploads/sites/4/2016/02/Scaling_up_pangolin_conservation_280714_v4.pdf.
Chase M. J., Schlossberg S., Griffin C. R., Bouché P. J. C., Djene S. W., Elkan P. W., et al. (2016). Continent-wide survey reveals massive decline in African savannah elephants. PeerJ 4, e2354. doi: 10.7717/peerj.2354
Congressional Budget Office (2015). Scanning and imaging shipping containers overseas: costs and alternatives. Available online at: https://www.cbo.gov/sites/default/files/114th-congress-2015-2016/reports/51478-Shipping-Containers-OneCol.pdf (Accessed August 30, 2024).
Cunningham A. B. (2015). More than a music tree: 4400 years of Dalbergia melanoxylon trade in Africa. South Afr. J. Bot. 98, 167. doi: 10.1016/j.sajb.2015.03.004
de Sadeleer N., Godfroid J. (2020). The Story behind COVID-19: Animal Diseases at the Crossroads of Wildlife, Livestock and Human Health. Eur. J. Risk Regul. 11, 210–227. doi: 10.1017/err.2020.45
Ellis H., Mulder C., Valverde E., Poling A., Edwards T. (2017). Reproducibility of African giant pouched rats detecting Mycobacterium tuberculosis. BMC Infect. Dis. 17, 298. doi: 10.1186/s12879-017-2347-3
Ellis H., Watkins E., Cox C., Sargisson R., Edwards T. (2019). Trialling a semi-automated line cage for scent detection by African giant pouched rats. Behav. Analysis: Res. Pract. 19, 150–163. doi: 10.1037/BAR0000095
Financial Action Task Force (2020). FATF annual report 2020-2021. Available online at: https://www.fatf-gafi.org/en/publications/Fatfgeneral/Annual-report-2020-2021.html (Accessed August 30, 2024).
Fischer-Tenhagen C., Johnen D., Heuwieser W., Becker R., Schallschmidt K. (2017). Odor perception by dogs: evaluating two training approaches for odor learning of sniffer dogs. Chem. Senses 42, 435–441. doi: 10.1093/chemse/bjx020
Freeman A. R., Ophir A. G., Sheehan M. J. (2020). The giant pouched rat (Cricetomys ansorgei) olfactory receptor repertoire. PloS One 15, e0221981. doi: 10.1371/journal.pone.0221981
Gazit I., Goldblatt A., Grinstein D., Terkel J. (2021). Dogs can detect the individual odors in a mixture of explosives. Appl. Anim. Behav. Sci. 235, 105212. doi: 10.1016/j.applanim.2020.105212
Gomez L., Leupen B. T. C., Hwa T. K. (2016). The trade of African pangolins to Asia: a brief case study of pangolin shipments from Nigeria Vol. 28 (Cambridge, UK: TRAFFIC Bulletin), 3–5. Available at: https://www.traffic.org/site/assets/files/3012/traffic_pub_bulletin_28_1-africa-asia-pangolin-trade.pdf.
Harrington L. A., D’Cruze N., Macdonald D. W. (2018). Rise to fame: events, media activity and public interest in pangolins and pangolin trade 2005-2016. Nat. Conserv. 30, 107–133. doi: 10.3897/natureconservation.30.28651
Hauenstein S., Kshatriya M., Blanc J., Dorman C. F., Beale C. M. (2019). African elephant poaching rates correlate with local poverty, national corruption and global ivory price. Nat. Commun. 10, 2242. doi: 10.1038/s41467-019-09993-2
Heinrich S., Wittman T. A., Ross J. V., Shepherd C. R., Challender D. W. S., Cassey P. (2017). The global trafficking of pangolins: A comprehensive summary of seizures and trafficking routes from 2010–2015 (Petaling Jaya, Selangor, Malaysia: TRAFFIC, Southeast Asia Regional Office). Available at: https://www.traffic.org/site/assets/files/1606/global-pangolin-assessment.pdf.
Hope R. M. (2013). Rmisc: R package version 1.5. Available online at: https://cran.r-project.org/web/packages/Rmisc/Rmisc.pdf (Accessed August 30, 2024).
Ingram D., Coad L., Abernethy K., Maisels F., Stokes E., Bobo K., et al. (2018). Assessing Africa-wide pangolin exploitation by scaling local data. Conserv. Lett. 11, e12389. doi: 10.1111/conl.12389
Ingram D., Cronin D., Challender D., Venditti D., Gonder M. (2019). Characterising trafficking and trade of pangolins in the Gulf of Guinea. Global Ecol. Conserv. 17, e00576. doi: 10.1016/j.gecco.2019.e00576
Khalid F., Taj M. B., Jamil A., Kamal H., Afzal T., Iqbal M. J., et al. (2019). Multiple impact of illegal logging: a key to deforestation over the globe. Biomed. J. Sci. Tech. Res. 20, 15430–15435. doi: 10.26717/BJSTR.2019.20.003519
King A. (2013). The new canine detectives. New Scientist 219, 40–43. doi: 10.1016/S0262-4079(13)62100-4
Lazarowski L., Krichbaum S., DeGreeff L. E., Simon A., Singletary M., Angle C., et al. (2020). Methodological considerations in canine olfactory detection research. Front. Veterinary Humanities Soc. Sci. 7. doi: 10.3389/fvets.2020.00408
Lazarowski L., Waggoner P., Hutchings B., Angle C., Porritt F. (2021). Maintaining long-term odor memory and detection performance in dogs. Appl. Anim. Behav. Sci. 238, 105301. doi: 10.1016/j.applanim.2021.105301
Lenth R. V. (2021). emmeans: Estimated Marginal Means, aka Least-Squares Means (R package version 1.6.3). Available online at: https://cran.r-project.org/web/packages/emmeans/index.html (Accessed on August 30, 2024).
Lewon M., Webb E. K., Brotheridge S. M., Cox C., Fast C. D. (2019). Behavioral skills training in scent detection research: Interactions between trainer and animal behavior. J. Appl. Behav. Anal. 52, 682–700. doi: 10.1002/jaba.566
Luczon A. U., Ong P. S., Quilang J. P., Fontanilla I. K. C. (2016). Determining species identity from confiscated pangolin remains using DNA barcoding. Mitochondrial DNA Part B 1, 763–766. doi: 10.1080/23802359.2016.1238752
Mahoney A., Edwards T. L., Weetjens B., Cox C., Beyene N., Jubitana M., et al. (2013). Giant African pouched rats (Cricetomys Gambianus) as detectors of Tuberculosis in human sputum: two operational improvements. psychol. Rec. 63, 583–594. doi: 10.11133/j.tpr.2013.63.3.012
Mahoney A., Weetjens B. J., Cox C., Beyene N., Reither K., Makingi G., et al. (2012). Pouched rats’ detection of tuberculosis in human sputum: Comparison to culturing and polymerase chain reaction. Tuberculosis Res. Treat 2012, 716989. doi: 10.1155/2012/716989
Milledge S., Gelvas I., Ahrends A. (2007). Forestry, governance and national development: lessons learned from a logging boom in Southern Tanzania (Dar es Salaam: IUCN: International Union for Conservation of Nature. TRAFFIC East-Southern Africa, Tanzania, Ministry of Natural Resources and Tourism, Tanzania Development Partners Group, TZ).
Moser A. Y., Bizo L., Brown W. Y. (2019). Olfactory generalization in detector dogs. Animals 9, 1–12. doi: 10.3390/ani9090702
Nakai K., Ishizuka M., Ohta S., Timothy J., Jasper M., Lyatura N. M., et al. (2019). Environmental factors and wood qualities of African blackwood, Dalbergia melanoxylon, in Tanzanian Miombo natural forest. J. Wood Sci. 65, 39. doi: 10.1186/210086-019-1818-0
Narayanasamy S. S., Chong E., Aziz S. A., Visscher W., Jaafar S. Z. A., Clements G. R. (2023). Hide-and-sniff: can anti-trafficking dogs detect obfuscated wildlife parts? Conserv. Sci. Pract. 5, e12886. doi: 10.1111/csp2.12886
Nellemann C., Henriksen R., Kreilhuber A., Stewart D., Kotsovou M., Raxter P., et al. (2016). The rise of environmental crime: a growing threat to natural resources, peace, development and security (Nairobi, Kenya: United Nations Environment Programme (UNEP). Available at: https://wedocs.unep.org/20.500.11822/7662.
Olayemi A., Nicolas V., Hulselmans J., Missoup A. D., Fichet-Calvet E., Amundala D., et al. (2012). Taxonomy of the African giant pouched rats (Nesomyidae: Cricetomys): molecular and craniometric evidence support and unexpected high species diversity. Zoological J. Linn. Soc. 165, 200–219. doi: 10.1111/j.1096-3642.2012.00823.x
Oldenburg C. J., Schoon A., Heitkönig I. M. A. (2016). Wildlife detection dog training: A case study on achieving generalization between target odor variations while retaining specificity. J. Veterinary Behav. 13, 34–38. doi: 10.101016/j.jveb.2016.03.008
Poling A., Weetjens B., Cox C., Beyene N. W., Bach H., Sully A. (2011a). Using trained pouched rats to detect land mines: another victory for operant conditioning. J. Appl. Behav. Anal. 44, 351–355. doi: 10.1901/jaba.2011.44-351
Poling A., Weetjens B., Cox C., Beyene N., Durgin A., Mahoney A. (2011b). Tuberculosis detection by giant African pouched rats. Behav. Analyst 34, 47–54. doi: 10.1007/bf03392234
Sánchez-Barreiro F., Gopalakrishnan S., Ramos-Madrigal J., Westbury M. V., de Manuel M., Margaryan A., et al. (2021). Historical population declines prompted significant genomic erosion in the northern and southern white rhinoceros (Ceratotherium simum). Mol. Ecol. 30, 6355–6369. doi: 10.111/mec.16043
Save The Rhino. (2024). Available at: www.savetherhino.org/rhino-info/poaching-stats/ (Accessed January 30, 2024).
Smith R. J., Muir R. D. J., Walpole M. J., Balmford A., Leader-Williams N. (2003). Governance and the loss of biodiversity. Nature 426, 67–70. doi: 10.1038/nature02025
UNODC (2020). United Nations office on drugs and crime world drug report. Available online at: https://wdr.unodc.org/wdr2020/en/exsum.html (Accessed August 30, 2024).
Waggoner P., Lazarowski L., Hutchings B., Angle C., Porritt F. (2022). Effects of learning an increasing number of odors on olfactory learning, memory and generalization in detection dogs. Appl. Anim. Behav. Sci. 247, 105568. doi: 10.1016/j.applanim.2022.105568
Webb E. K., Saccardo C. C., Poling A., Cox C., Fast C. D. (2020). Rapidly training African giant pouched rats (Cricetomys ansorgei) with multiple targets for scent detection. Behav. Processes 174, e104085. doi: 10.1016/j.beproc.2020.104085
Weetjens B. J. C., Mgode G. F., Machang’u E. S., Kazwala R., Lwilla F., Cox C., et al. (2009). African pouched rats for the detection of puylmonary tuberculosis in sputum samples. Int. J. Tuberculosis Lung Dis. 13, 737–743.
Wickham H., François R., Henry L., Müller K. (2021). dplyr: A grammar of data manipulation (R package version 1.0.7). Available online at: https://cran.r-project.org/web/packages/dplyr/index.html (Accessed August 30, 2024).
Willcox D., Nash H., Trageser S., Kim H., Hywood L., Connelly E., et al. (2019). Evaluating methods for detecting and monitoring pangolin populations (Pholidota: Manidae). Global Ecol. Conserv. 17, e00539. doi: 10.1016/j.gecco.2019.e00539
Williams M., Johnston J. M. (2002). Training and maintaining the performance of dogs (Canis familiaris) on an increasing number of odor discriminations in a controlled setting. Appl. Anim. Behav. Sci. 78, 55–65. doi: 10.1016/S0168-1591(02)00081-3
Keywords: animal scent detection, illegal wildlife trade, smuggling detection, anti-trafficking, proof-of-principle, elephant ivory, rhino horn, pangolin
Citation: Szott ID, Webb EK, Mshana S, Mshigeni A, Magesa WS, Iyungu A, Saidi M, Van de Laak D, Schneider M, Dore A, Brebner K, Davies-Mostert H, Cox C and Fast CD (2024) Ratting on wildlife crime: training African giant pouched rats to detect illegally trafficked wildlife. Front. Conserv. Sci. 5:1444126. doi: 10.3389/fcosc.2024.1444126
Received: 05 June 2024; Accepted: 22 August 2024;
Published: 30 October 2024.
Edited by:
David R. Breininger, University of Central Florida, United StatesReviewed by:
Karthikeyan Vasudevan, Centre for Cellular & Molecular Biology (CCMB), IndiaZaira Lizbeth Esparza Rodríguez, Instituto de Ecología (INECOL), Mexico
Copyright © 2024 Szott, Webb, Mshana, Mshigeni, Magesa, Iyungu, Saidi, Van de Laak, Schneider, Dore, Brebner, Davies-Mostert, Cox and Fast. This is an open-access article distributed under the terms of the Creative Commons Attribution License (CC BY). The use, distribution or reproduction in other forums is permitted, provided the original author(s) and the copyright owner(s) are credited and that the original publication in this journal is cited, in accordance with accepted academic practice. No use, distribution or reproduction is permitted which does not comply with these terms.
*Correspondence: Isabelle D. Szott, szott.izzy@gmail.com
†These authors share first authorship