- 1U.S. Geological Survey, Oregon Cooperative Fish and Wildlife Research Unit, Department of Fisheries, Wildlife, and Conservation Sciences, Oregon State University, Corvallis, OR, United States
- 2Pacific Northwest Research Station, U.S.D.A. Forest Service, Olympia, WA, United States
Introduction: Habitat loss and degradation pose significant threats to global fish and wildlife populations, prompting substantial investments in habitat creation and restoration efforts. Not all habitats provide equal benefits, leading to challenges in prioritizing restoration actions. For example, juvenile anadromous salmonids require high quality rearing aquatic habitats to achieve the physiological requirements needed to successfully migrate to the ocean. However, there are profound disagreements among anadromous salmon restoration managers whether it is best to focus efforts on restoring in-channel habitats that are available for the entire rearing period or floodplain habitats that, while facilitating greater growth and survival than in-channel habitats, are only available for a few weeks at a time and are typically only activated every two-to-three years.
Methods: We used an existing fall-run Chinook salmon decision-support model to evaluate under what conditions floodplain restoration would provide greater benefits than in-channel habitat restoration. The simulations included a wide range of floodplain inundation frequencies and durations and floodplain benefits in the form of increased survival and growth relative to in-channel habitats.
Results: The simulations results indicated that in-channel habitat restoration was always the best habitat restoration action when there was no existing in-channel habitat despite simulating a wide range of flood frequency, duration, and growth and survival benefits. Floodplain restoration was generally best when there was sufficient in-channel habitat available to successfully rear most of the juveniles produced by the returning adult salmon.
Discussion: We hypothesize that in-channel and floodplain habitats have different roles in salmon population maintenance with in-channel habitats regulating the overall population size and floodplains acting as recurrent resource pulses. Our study provides a quantitative framework to evaluate the benefit of these two habitat types and provides generalizable rulesets that can be used by managers when implementing habitat restoration strategies for species that inhabit both in-channel and floodplain habitats.
1 Introduction
Habitat loss and degradation are among the primary threats to many fish and wildlife populations across the globe. In response, managers have invested considerable resources into habitat creation or restoration efforts to conserve animal populations (BenDor et al., 2015; Höhl et al., 2020; Bodin et al., 2022). Not all habitats provide the same benefits to the species they are intended to support. In some cases, the relative benefits are more easily distinguishable, such as habitats to support reproduction (i.e., spawning or nesting habitats) versus habitat to support foraging (i.e., rearing and foraging habitats). However, the relative benefits are much more difficult to ascertain when different habitat types are intended to support the same life stage or activity, but the quality and availability of the habitats varies. For example, juvenile anadromous salmonids require perennial in-channel aquatic habitats to rear to achieve the physiological requirements needed to successfully migrate to the ocean (Honea et al., 2009; Bourret et al., 2016). Although floodplain habitats serve similar functions as their in-channel counterparts, these habitats are also widely documented to facilitate increased somatic growth in salmonids due to an increase in food availability (Jeffres et al., 2008; Opperman et al., 2017). Importantly, these increased somatic growth rates have been linked to increased survival of juvenile salmonids. Therefore, floodplain habitats provide important benefits over in-channel habitats. However, floodplain habitats are ephemeral, where they are often only available every two-to-three years and are typically only activated for a few weeks at a time (Williams et al., 2009).
Given the often-limited resources available to invest into habitat restoration efforts, managers are regularly assigned the difficult task of prioritizing which habitat types to invest in (and where) to promote healthy salmonid populations. To help address these challenges, we developed quantitative decision-support models (DSMs) to evaluate alternative habitat restoration actions for salmonids in the Central Valley of California, U.S.A (Peterson and Duarte, 2020). These DSMs were used by a stakeholder group, the Science Integration Team (SIT), as they participated in the structured decision-making process to help inform the development of a five-year habitat restoration strategy for the Central Valley Project Improvement Act (CVPIA) Fisheries Program (USBR and USFWS, 2020). At the time, the DSMs indicated that in most cases increasing the amounts of perennial in-channel habitat led to substantially increased fish abundances when compared to scenarios that increased the availability of ephemeral floodplain habitats. By extension, the five-year habitat restoration strategy prioritized the creation of perennial in-channel habitats over ephemeral floodplain habitats to restore anadromous salmon populations.
As they continue to participate in the structured decision-making process, the SIT recently reinitiated the conversations that will likely inform the development of the next five-year CVPIA Fisheries Program restoration strategy aimed at promoting salmonid populations in the region. Over the past five years, the SIT has gathered improved information on current conditions in the Central Valley, and we have subsequently revised the DSMs to more accurately capture the best available science and hypotheses on system dynamics in the region. However, there is significant disagreement among stakeholders, with some advocating for the restoration of ephemeral floodplain habitats as the most effective means to restore anadromous salmon populations despite previous model runs. Much of this conflict arises from the uncertainty regarding the greater somatic growth and survival benefits to juvenile salmonids that use floodplain habitats and a belief among some SIT members that created/restored floodplains can be engineered to increase the frequency of inundation over more natural floodplain habitats.
Herein, we focused our study on fall-run Chinook salmon (Oncorhynchus tshawytscha) because they occur in all of the natal tributaries in the DSMs and modified this DSM to more explicitly evaluate tradeoffs in restoring ephemeral floodplain habitats versus perennial in-channel habitats to promote salmonid populations. Similar to Peterson and Duarte (2020), we focused on the modules that simulate juvenile salmonids as they rear in freshwater environments before making their migration to oceanic waters. Our goal of this study was to use the DSM to evaluate under what conditions ephemeral floodplain habitats provide greater benefits than perennial in-channel habitats when the objective is to maximize the number of juvenile salmonids that return as adults. Thus, we considered a broad range of possible environmental conditions and effect sizes within our evaluation. Given our previous evaluations of these habitat types, we hypothesized that floodplain habitats would likely only be considered more beneficial than in-channel habitats when the survival of juvenile fish rearing in in-channel habitats was low and the availability of existing rearing habitat (either in-channel or floodplain) during the early outmigration window (when floodplains are typically activated) was relatively limited.
2 Methods
2.1 Restoration area
The eponymously named California Central Valley is situated between the Coast Ranges and the Sierra Nevada mountain range of California, USA. It is made up primarily of two watersheds, the Sacramento River to the north and the San Joaquin River to the south. The Central Valley climate is characterized by Mediterranean-like conditions, with hot, dry summers and mild, wet winters (Deitch et al., 2017). Streamflows in the Central Valley are derived principally from the snowpack in the Sierra Nevada and are greatest in the winter and lowest in the summer. The spatial extent of the restoration area includes the Sacramento and San Joaquin Rivers and their tributaries and the major tributaries that drain into the central portion of the Sacramento-San Joaquin Delta. Candidate restoration areas included only stream segments and adjacent riparian areas downstream of major obstructions to upstream migration, such as Shasta Dam on the Sacramento River. The spatial grain of the restoration area was individual tributaries, except the San Joaquin River below the Merced River was the only section included in these DSMs, and the mainstem Sacramento River was subdivided into four sections.
2.2 Model overview
The Chinook salmon DSMs are thoroughly documented in Peterson and Duarte (2020) and available at https://github.com/CVPIA-OSC/fallRunDSM. We briefly described them here. These stochastic DSMs tracked the number of juvenile salmon in four size groups: small, < 42 mm total length; medium, 42–72 mm; large, 72–110 mm; and very large, > 110 mm and the returning adult stage. Transitions between stages were estimated using survival and growth parameters that varied with simulation and represented conditions in the natal tributaries, migratory corridors, delta, and ocean. The DSMs originally operated on a monthly time step but we adjusted the parameters for this study to coincide with a weekly time step to facilitate evaluations of different floodplain inundation durations. Again, we focused the evaluation on fall-run Chinook salmon because they occur in all of the natal tributaries in the DSMs. Therefore, juvenile rearing occurred over a 30-week period from January to August. All simulation modeling was conducted using R statistical software v 4.3.2, “Eye Holes” (R Core Team, 2023).
The DSM simulations began with 10,000 adults in a natal tributary. Because we were primarily focused on identifying the relative benefits of juvenile rearing habitat restoration (i.e., in-channel and floodplain habitats), we assumed that there was sufficient spawning habitat for all spawning adult salmon in our evaluations. The number of small-sized juvenile salmon produced was estimated as a function of the number of spawning adults, adult prespawn survival, adult sex ratio, female fecundity, and egg-to-fry survival (Table 1; Figure 1). Juvenile salmon reared in their natal tributaries until habitat capacity was exceeded and capacity was a function of the number of juvenile fish and fish body size (i.e., juvenile territory size; Table 1). Juvenile salmon habitat use and movement out of a natal tributary were modeled using a habitat and body sized-based ruleset. For each weekly time step, juvenile fish used all available habitat with larger fish occupying available habitat first. Floodplain habitats were filled first when they were inundated/activated; when capacity was exceeded in floodplains, the remaining fish were assigned to in-channel habitats until in-channel habitat capacity was exceeded. Excess fish that were not assigned to habitats (i.e., habitat capacity was exceeded) left the natal tributary. Fish that remained in a natal tributary survived and grew as a function of habitat type and body size that varied with simulation scenario, as detailed below. Fish that transitioned to the very large size class left the natal tributary the next time step. Similarly, all juvenile salmon that remained in their natal tributaries at the start of week 30 left the natal tributary. Juveniles leaving their natal tributaries migrated to the delta, the bay, then the ocean and survived as a function of time and migration distance that varied with simulation scenario. Juveniles entering the ocean survived as a function of fish body size and the timing of ocean entry relative to ocean productivity transition (Satterthwaite et al., 2014). This ocean entry survival estimates the probability that a juvenile salmon entering the ocean successfully returns to freshwater as an adult (Supplementary Information). We defined these fish as adult equivalents and used this measure to quantify the relative benefits of perennial in-channel and ephemeral floodplain habitat restoration (Figure 1).
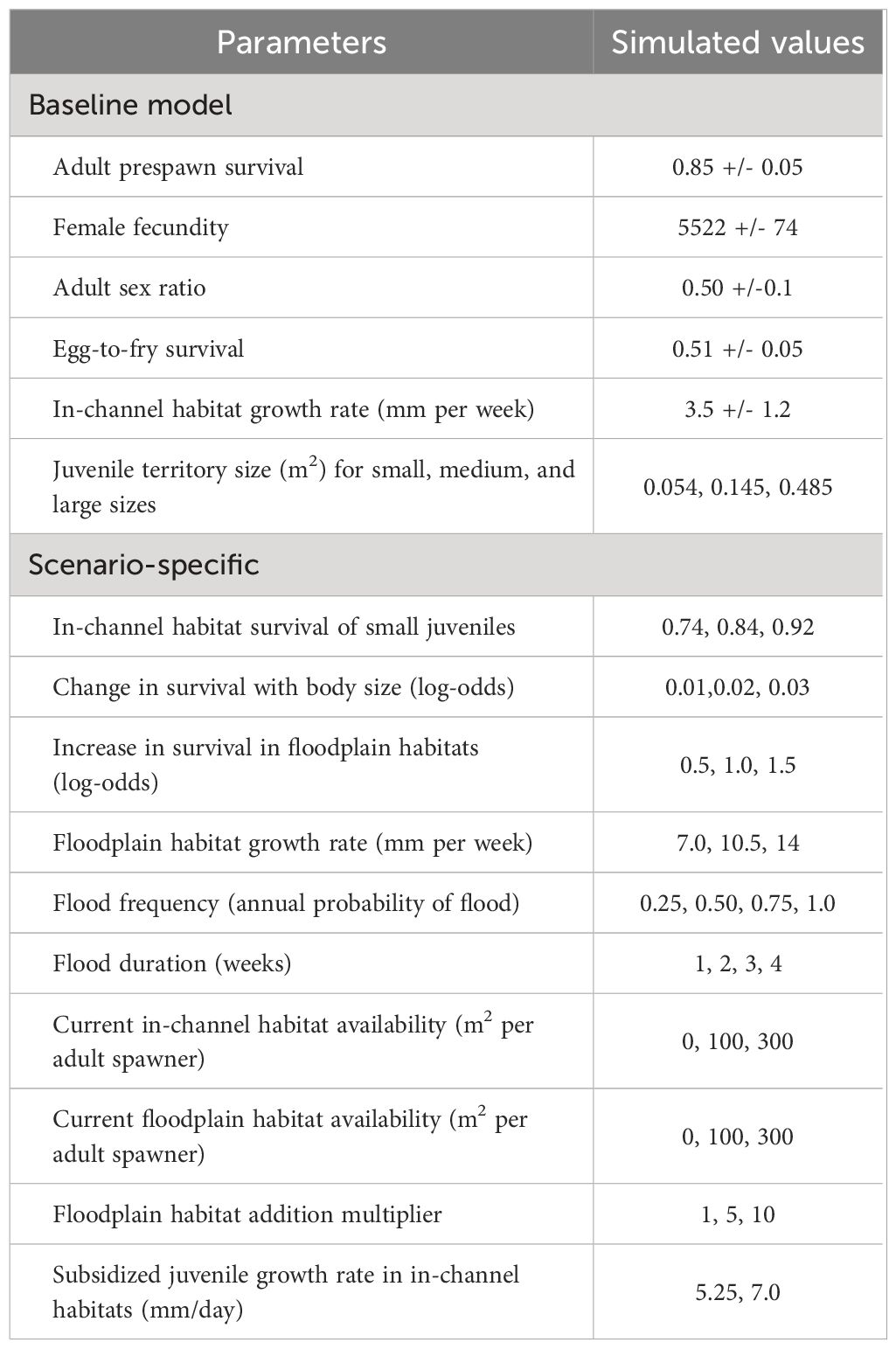
Table 1 Fall-run Chinook salmon (Oncorhynchus tshawytscha) decision-support model baseline parameters with (+/-) standard deviations and scenario specific values used in evaluation of the relative value of restoring in-channel and floodplain juvenile salmon habitats.
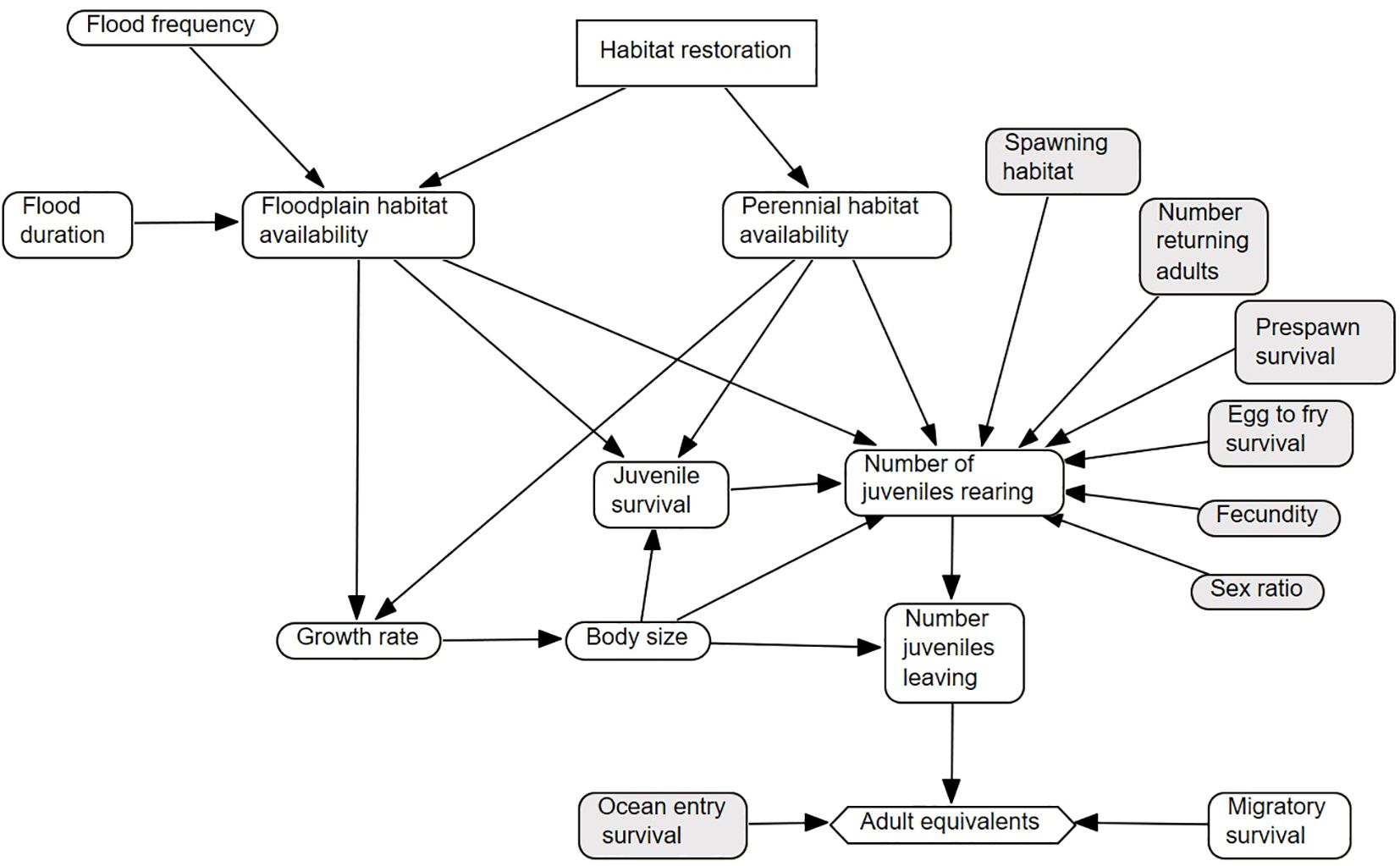
Figure 1 Influence diagram representing the fall-run Chinook salmon (Oncorhynchus tshawytscha) decision-support model for evaluating the relative benefits of restoring in-channel and floodplain habitats. Shaded components were the same across simulation scenarios.
2.3 Evaluation scenarios
Again, our main goal was to identify the conditions whereby floodplain habitat restoration would result in a greater number of expected adult equivalents than in-channel habitat restoration. Previous sensitivity analyses of the DSMs indicated that the rankings of best Chinook salmon habitat restoration actions were most sensitive to juvenile survival and growth rate and habitat availability (Peterson and Duarte, 2020). Therefore, we identified low, medium, and high weekly survival rates of the small size group based on estimated natal rearing survival in Central Valley tributaries (Table 1). Floodplain habitats are believed to have higher survival and growth rates, so we evaluated floodplain survival at rates 1.6, 2.7, and 4.5 times greater and growth rates 2, 3, and 4 times greater than in-channel habitats (Table 1). The smallest of these growth and survival increases in floodplain habitats were used in the DSM for informing the development of the five-year habitat restoration strategy for the CVPIA Fisheries Program (Peterson and Duarte, 2020). The greater sizes attained by fishes rearing in floodplain habitats may impart a survival benefit after the floods recede so we identified three increasing levels of body size effects on survival in rearing juvenile salmon (Table 1). Overbank flows required to inundate floodplains typically occur once every other year (Wolman and Leopold, 1957) but floodplain activation flows (i.e., the smallest 7-d flows that initiate ecological processes in the floodplain) occur two of every three years (Williams et al., 2009). We therefore evaluated the effect of flood frequency at four levels: one lower and two higher than overbank and floodplain activation flows (Table 1). Floodplains can be activated for intervals ranging from days to weeks, but generally require about 7 d to initiate the ecological processes that produce food subsidies for fish (Williams et al., 2009). We evaluated the effects of floodplain duration at four levels ranging from 1 to 4 weeks (Table 1). Existing habitat availability was also simulated at four levels of availability that represented no (zero) habitat, medium, and high levels of habitat availability for both in-channel and floodplain habitats (Table 1). The high in-channel habitat availability value is approximately the value when juvenile rearing habitat is no longer limiting under average conditions in the Central Valley tributaries (e.g., state-dependent policy plots in Peterson and Duarte, 2020). Based on the CVPIA Fisheries Program internal records, juvenile rearing habitat restoration creates, on average, 0.8 ha of in-channel or floodplain habitat in the DSM under the assumption that both actions require similar expenditure of resources to complete. To evaluate circumstances when similar effort can produce more floodplain habitat, we evaluated the relative benefits of floodplain restoration for three multiples applied to 0.8 ha: one (equal to in-channel habitat), five, and ten times (Table 1). Note that these restoration area scenarios can also be interpreted as greater fish holding capacity for the same unit area restored.
Perennial in-channel habitat availability fluctuated systematically through time in the natal tributaries in Central Valley based on flow regimes and the fluctuations can affect the efficacy of habitat restoration actions (Peterson and Duarte, 2020). Therefore, we used three functions to simulate situations representing increasing, decreasing, and stable habitat availability through time (Figure 2). The probability a juvenile salmon that was migrating to the ocean survived (outmigrant survival) also varied among natal tributaries due to their distance from the ocean and location. To incorporate these effects, we simulated under four outmigrant survival patterns (Figure 2) that represented the typical patterns in the Central Valley DSMs.
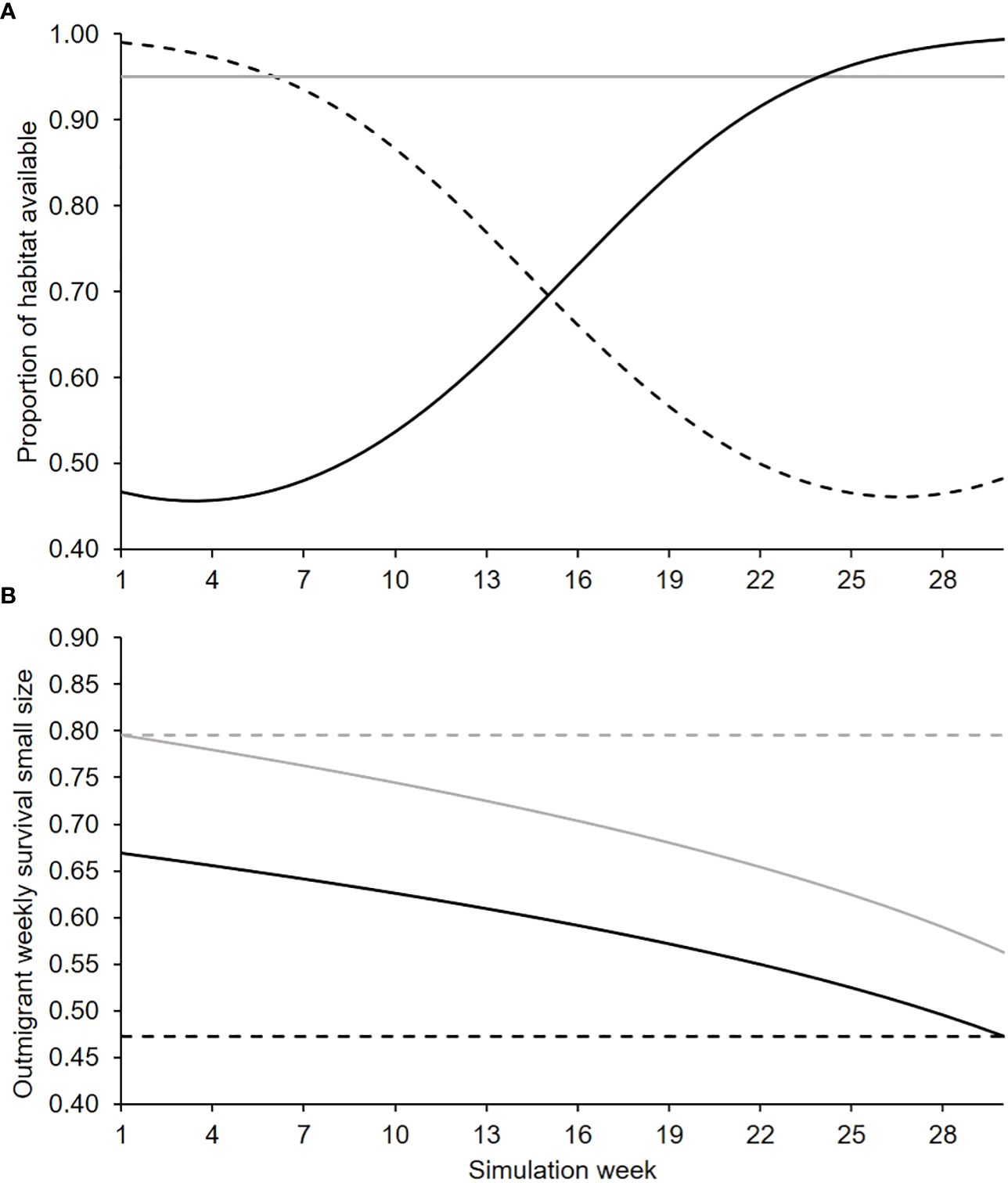
Figure 2 Change in in-channel habitat availability across the 30-week simulation (A) under decreasing (broken line), constant (solid gray), and increasing (solid black) trends and (B) outmigrant survival under low (black) and high (gray) survival with constant (broken line) and decreasing trends (solid).
Finally, floodplains are also believed to provide a food subsidy to fishes that are not using the floodplain during inundation (i.e., fish rearing in adjacent in-channel habitats), and there is some evidence to support this hypothesis (Górski et al., 2013; Farly et al., 2019). However, the magnitude and extent of the food subsidy are largely uncertain. To incorporate this uncertainty, in a post hoc evaluation we applied a food subsidy under two assumptions of the spatial extent. The first applied the food subsidy to all juvenile salmon rearing in the in-channel habitat within the tributary during floodplain inundation. The second applied the subsidy to a portion of fish rearing the adjacent in-channel habitats that equaled the fish rearing capacity of the floodplain habitat in the tributary. Thus, the extent of the subsidy under the second assumption was proportional to the amount of floodplain in a tributary. We also evaluated two growth rates for food subsidized fish at 1.5 and 2 times that of baseline in-channel habitat rates (Table 1).
2.4 Scenario simulation
We conducted two sets of simulations. The first set of simulations focused on evaluating the relative benefits of in-channel and floodplain habitat restoration without floodplain food subsidies. The second set of simulations examined the same tradeoffs, but included the additional benefits of food subsidies. For both sets of simulations, the expected number of adult equivalent salmon was estimated under three candidate actions: no restoration, 0.8 ha in-channel habitat restoration, and floodplain habitat restoration that varied with scenario (i.e., 0.8–8.0 ha). During the simulations, stochasticity was imposed by drawing parameters from statistical distributions with means and standard deviations in Table 1. The flood frequency was simulated using a Bernoulli distribution and the week the flood event was initiated was randomly assigned to one of the first 12 weeks of the year with equal probability (i.e., a unform distribution). The number of fish that transitioned from one state to another (e.g., surviving week to week) were modeled using a binomial distribution. The first set of simulations evaluated all combinations of scenario specific parameters (without the subsidy), the three in-channel habitat dynamics trends, and the four outmigrant survival patterns. The food subsidy simulations also included all combinations of the scenario specific values except for current in-channel habitat availability that was fixed at the middle value (100 m2 per adult spawner), habitat dynamics was fixed at constant, juvenile survival was evaluated for the two lowest values (Table 1), and the outmigrant survival pattern was fixed at the high and decreasing pattern (Figure 2). We simulated 10,000 replicates for each scenario and estimated the mean number of adult equivalent salmon. For each combination, we considered the best restoration action to be the action that resulted in the greatest number of adult equivalent salmon.
The large number of simulation combinations and potentially interacting factors would make it difficult to identify the conditions that floodplain habitat restoration was preferable to in-channel habitat restoration. Therefore, we created binary indicators (0,1) for observations that had no action as the best restoration action and another indicator for observations when floodplain habitat restoration was the best restoration action. We then used logistic regression to fit all combinations of scenario parameters and two-way interactions and selected the best fitting model using Akaike’s Information Criteria (AIC; Akaike, 1973) with the small-sample bias adjustment (AICc; Hurvich and Tsai, 1989). The no food subsidy and food subsidy simulations were analyzed separately. Given the scenarios were simulated using discrete values, we treated all covariates as factor variables in our analyses. The no action regression models were fit using all of the scenarios, whereas the floodplain action regression models were fit to simulation data that excluded the combinations when no action was the best alternative. Thus, predictions under the floodplain restoration action should be interpreted as the probability that floodplain habitat restoration is the best restoration action relative to in-channel habitat restoration.
3 Results
Perennial in-channel habitat restoration was the best restoration action for a vast majority of simulation combinations (73%) compared to no action (13%) and floodplain restoration (14%) under the no subsidy scenarios. Similarly, in-channel habitat restoration was best restoration action in 83% of food subsidy simulations followed by floodplain restoration (14%), and no action (3%). On average, 295 and 215 more adult equivalent salmon were produced relative to no action when in-channel and floodplain restoration, respectively were the best restoration action. When current in-channel habitat availability was zero, in-channel habitat restoration was always the best restoration action. Therefore, simulations with zero current in-channel habitat availability were removed from the data prior to logistic regression model fitting to avoid quasi-complete separation.
The best fitting no restoration action model fit to the simulated no subsidy data contained all but two simulation parameters, flood frequency and outmigrant survival, and 103 two-way interactions (Supplementary Material). Again, no action was never selected when there was zero current in-channel habitat availability. The main effects parameters suggested that seasonal change in in-channel habitat availability and current in-channel and floodplain habitat availability had the greatest influence on the probability that no action was the best restoration action. The interactions suggested that those factors in combination with in-channel habitat survival of juveniles and change in survival with body size largely drove the no action decision. Plots of predicted probabilities for combinations of simulation parameters suggested that the probability that no action was the best was greatest when current in-channel and floodplain habitat availabilities were high (albeit there was a stronger and more consistent effect of current in-channel habitat availability), in-channel habitat survival of juveniles was high, and seasonal change in in-channel habitat availability was decreasing through the rearing period (Figure 3). However, probability that no action was the best when seasonal change in in-channel habitat availability was increasing tended to be higher when in-channel habitat was low.
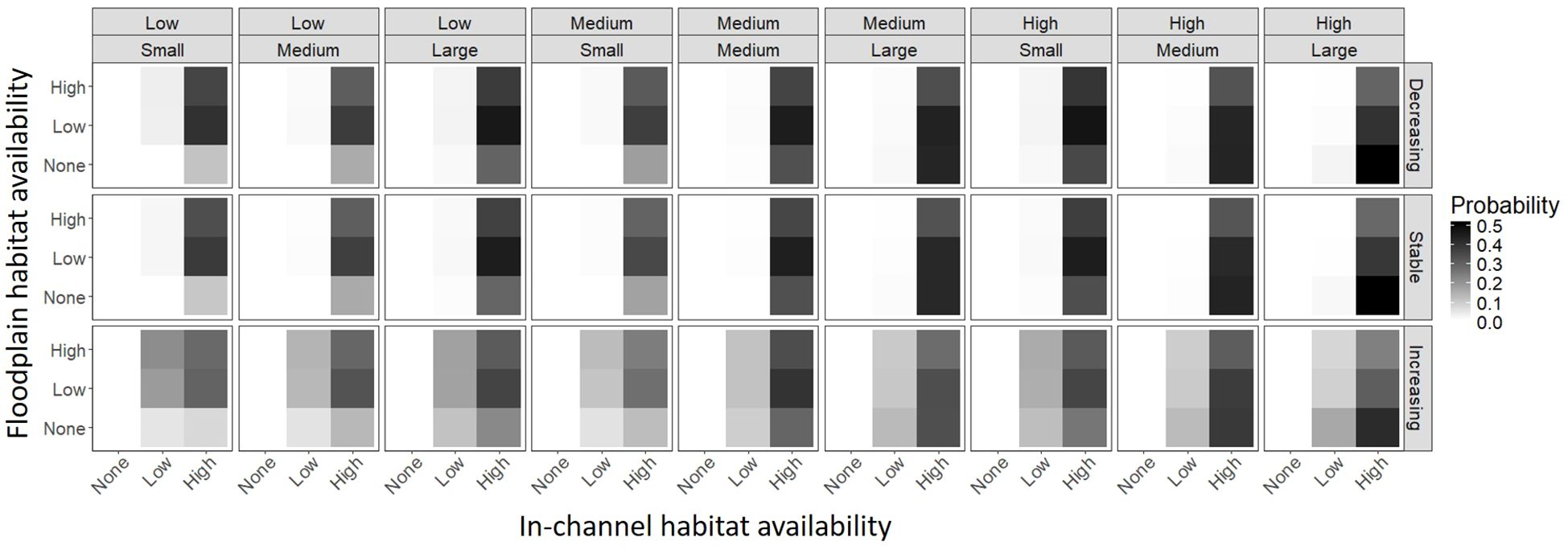
Figure 3 The mean predicted probability that no action was the best restoration action for combinations of juvenile in-channel habitat survival (top above), change in survival with body size (top inset), seasonal change in in-channel habitat availability (right), current in-channel habitat availability (bottom), and current floodplain habitat availability (left).
The best fitting floodplain restoration action model fitted with the no subsidy data also contained most of the simulation parameters except the floodplain habitat addition multiplier and there were 129 two-way interactions (Supplementary Material). Similar to the no restoration action model, current in-channel and floodplain habitat availability, in-channel habitat survival of juveniles, and the change in survival with body size were the most influential factors on the probability that a floodplain restoration action was the best. Again, floodplain habitat restoration was never selected when there was zero current in-channel habitat availability. The predicted probability of floodplain was greatest when there was no current floodplain habitat availability, high in-channel habitat availability, and low in-channel habitat survival of juveniles (Figure 4). Floodplain restoration actions also tended to be best when the change in survival with body size was small and seasonal change in in-channel habitat availability was increasing through the rearing period (Supplementary Material). Flood duration and the floodplain growth rate were also related to the probability that floodplain restoration action was best, but the effects were much lower than current floodplain habitat availability and flood frequency (Figure 5). Specifically, the probability floodplain restoration was the best action increased with lower current floodplain habitat availability.
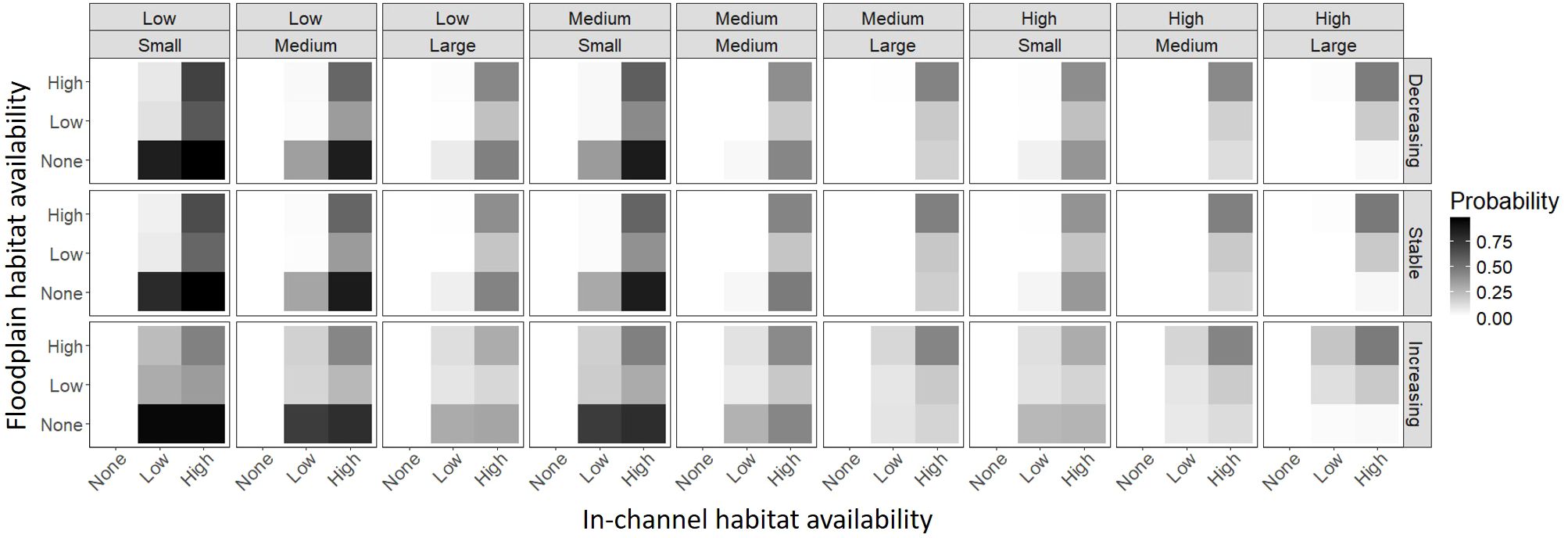
Figure 4 The mean predicted probability that floodplain restoration was the best restoration action (given the best restoration action was not “no action”) for combinations of in-channel habitat survival of juveniles (top above), change in survival with body size (top inset), seasonal change in in-channel habitat availability (right), current in-channel habitat availability (bottom), and current floodplain habitat availability (left).
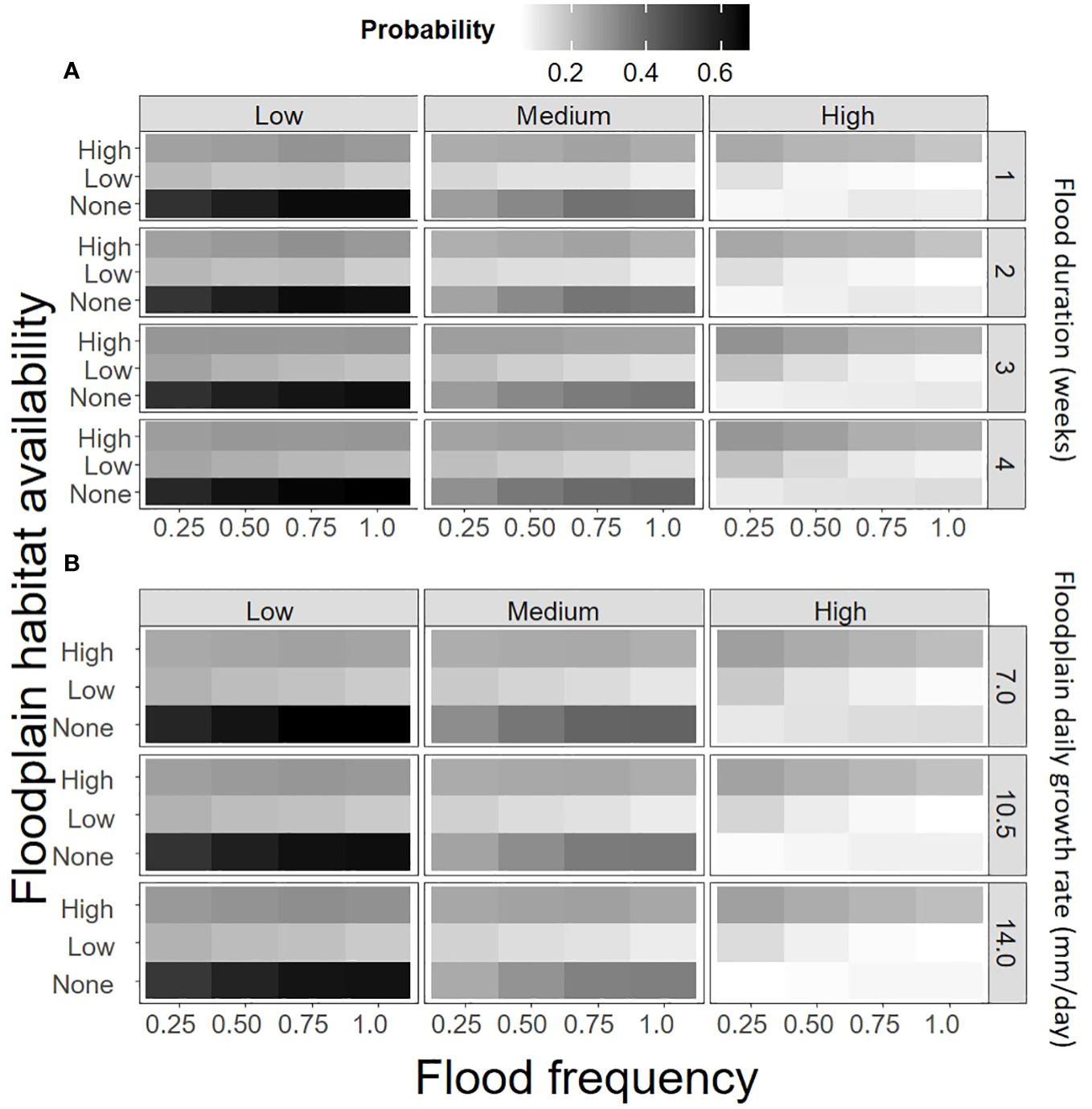
Figure 5 The mean predicted probability that floodplain restoration was the best restoration action (given the best restoration action was not “no action”) for combinations of in-channel habitat survival of juveniles (top), flood frequency (bottom), and current floodplain habitat availability (left) for (A) flood duration and (B) floodplain growth rates.
Model selection of the floodplain food subsidy simulated data indicated similar relationships with current floodplain habitat availability, in-channel habitat survival of juveniles, and the change in survival with body size (Supplementary Material). However, it was also strongly influenced by the extent of the subsidy, flood frequency and duration, and the floodplain habitat addition multiplier. Under the subsidy simulations, the floodplain restoration action was generally the best restoration action when there was none-to-low current floodplain habitat availability and in-channel habitat survival of juveniles was low (Figure 6, top). The probability was also positively related to the extent of the floodplain food subsidy and flood frequency (Figure 6, bottom). Interestingly, the relationship with flood duration was not monotonic and appeared to peak at two-weeks.
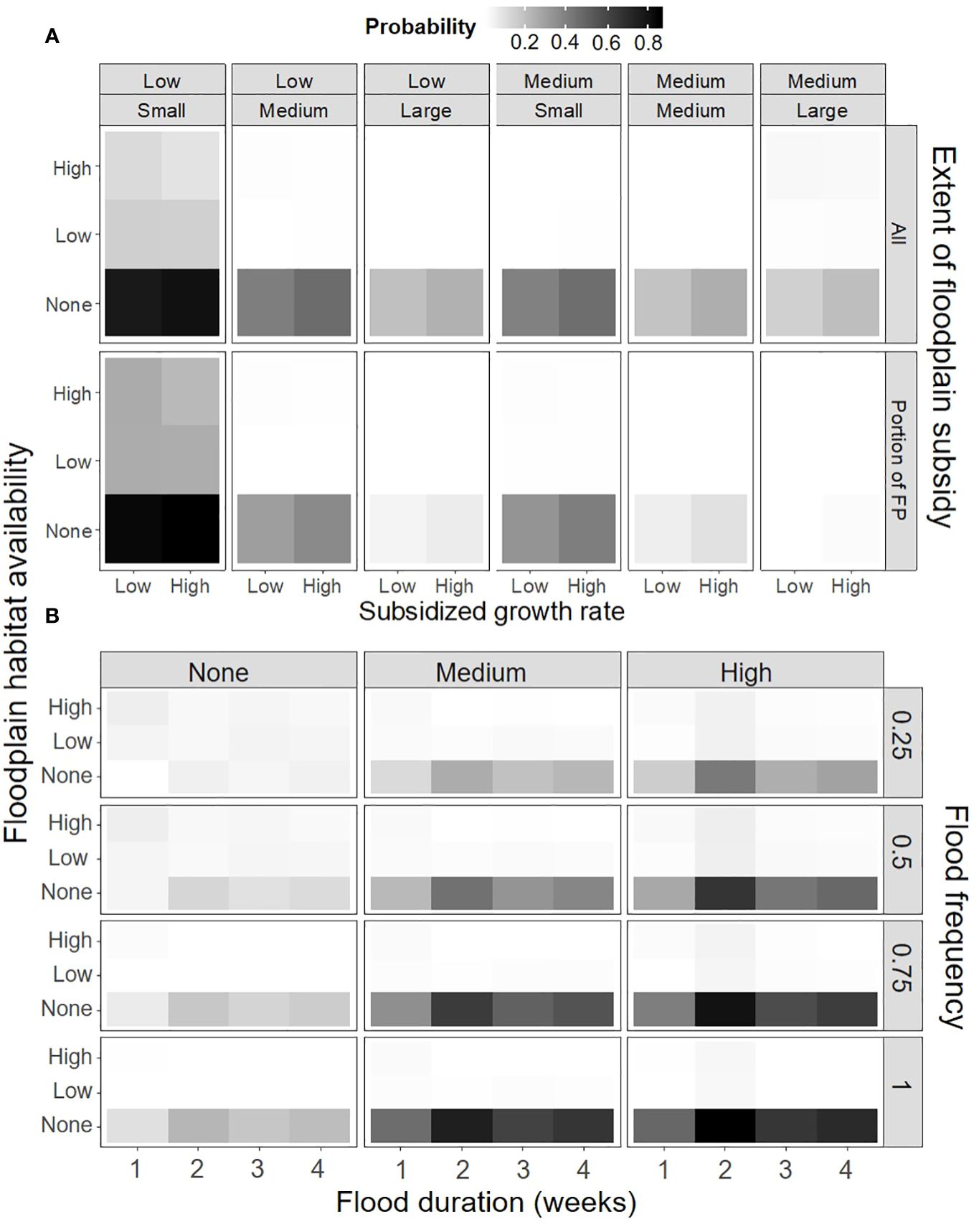
Figure 6 The mean predicted probability that floodplain restoration with a food subsidy was the best restoration action (given the best restoration action was not “no action”) for combinations of (A) in-channel habitat survival of juveniles (top label), change in survival with body size (top inset label), the extent of the subsidy, subsidized growth rate and current floodplain habitat availability and (B) floodplain habitat addition multiplier (top label), flood frequency, flood duration and current floodplain habitat availability.
4 Discussion
The fall-run Chinook salmon DSM used in this evaluation was a relatively simple model covering a portion of the salmon life cycle. Here, both habitat types served to hold and grow juvenile fish. However, fish in floodplain habitats had higher survival and growth rates compared to their counterparts rearing in in-channel habitat. Nonetheless, in-channel habitat restoration was always the best action when there was zero in-channel habitat available across a wide range of increased floodplain growth and survival benefits relative to those for fish rearing in in-channel habitat. Conversely, floodplain restoration tended to be the best restoration action when the existing in-channel habitat availability was near or exceeded the amount of in-channel habitat needed to rear most of the juveniles produced. This suggests that the two habitats have different, albeit interacting, roles with respect to salmon population maintenance. Because in-channel habitats are predictably available throughout the salmon rearing period and across years, we hypothesize that the availability of these perennial habitats largely determines the overall population size within a tributary (sensu Gibson, 1994). Floodplain habitats temporally increase the capacity of the system to support juvenile salmon, but they are typically not available all years and are not available throughout the entire 30-week rearing period. Thus, floodplain habitats can be viewed as a recurrent resource pulse that may alter the dynamics of fish populations (Holt, 2008).
Recurrent resource pulses can have a profound effect on population size and stability depending on how the resources affect population demographics. Mathematical evaluations of the effects of recurrent resource pulses suggest that pulses that temporarily increase population growth rate can increase population size (Cushing, 1987), whereas pulses that temporarily increase carrying capacity ultimately result in population decreases (Nisbet and Gurney, 1976) relative to stable environments. Experimental manipulations of captive populations exposed to recurrent resource pulses have shown that the pulses can maintain population sizes through increasing storage of energy (e.g., increased lipid reserves) that may act as a buffer during resource poor conditions (Costantino et al., 1998; Orland and Lawler, 2004). Such mechanisms are unlikely to occur in fall-run Chinook salmon, a semelparous species that spends a relatively small amount of their life-cycle in freshwater environments. Rather, the pulses increase habitat capacity, survival, and growth for the duration of the pulse resulting in more and larger individuals migrating to the ocean. The greater body size increases the survival in the ocean, depending on the timing of ocean entry (Satterthwaite et al., 2014). Thus, collectively floodplain habitats should result in greater number of returning adults in future years. Over multiple years, given Chinook salmon are known to have high fidelity to their natal tributaries, it seems plausible that increases in returning adults associated with resource pulses could lead to the population exceeding capacity. If this occurs on the spawning grounds, this may lead to increased prespawn mortality. Although not ideal, it should be noted that one benefit of this potential outcome would be a temporary source of increased marine derived nutrients that would likely increase juvenile salmon body growth and condition downstream (Kaylor et al., 2020). If capacity is exceeded in the perennial in-channel habitats, however, this may lead to a deficit of rearing habitat resources (i.e., space and food), which may unintentionally lead to lower juvenile survival during rearing or juveniles outmigrating to the ocean too soon or at smaller body sizes (i.e., reduced ocean entry survival). To our knowledge, there have been no empirical assessments of the population-level effects of restoring resource pulses on salmon populations over multiple years. Without complete understanding of habitat deficits, it seems plausible that restoring ephemeral floodplain habitats rather than in-channel habitats may carry some additional risk.
The greater survival, growth, and increased carrying capacity associated with floodplain habitat restoration was usually not sufficient to produce a greater number of adult equivalent salmon when compared to in-channel habitat restoration. This pattern generally held even under simulations with growth subsidies for fish rearing in in-channel habitats and a flood frequency and duration of every year and four weeks, respectively. We believe that the near dominance of an in-channel habitat restoration action was primarily due to the duration and timing of the floodplain inundation and the timing of the juveniles entering the ocean. The floodplain growth rates we used in the simulations ranged from values similar to those observed in floodplains in the Central Valley (1 mm/day, Dudley et al., 2023) to twice that value. Nonetheless, this would only grow approximately 25% of fish in the small size group (on average) to very large size group over a four-week inundation period. Importantly, the habitat capacity for rearing juveniles decreased at the end of the flood event, resulting in a greater number of juveniles leaving the tributary either as very large fish outmigrating or as fish that were pushed downstream by larger rearing fish. This can translate into having a greater number of juvenile fish entering the ocean when they were too small and when ocean conditions were poor. This rationale is further supported by the higher probabilities of floodplain restoration being the best action when seasonal in-channel habitat availability increased through time (i.e., habitat dynamics increasing). The increased in-channel habitats were able to support some of the excess juveniles after the simulated floods ended. This suggests that the unpredictable and ephemeral nature of floodplain habitat availability relative to in-channel habitats limit their value when in-channel habitat is limiting, particularly when in-channel habitat is relatively limited later in the rearing period. Thus, it makes logical sense that restoring floodplain habitats was never more advantageous when in-channel habitat availability is limiting.
Juvenile survival during rearing in freshwater also strongly affected the best restoration action. When existing in-channel habitat was insufficient for supporting most of the juveniles, floodplain habitat restoration tended to be the best restoration action when small juvenile survival was low and the effect of body size on survival was small (i.e., all juvenile fish tended to have low survival regardless of body size). This was particularly evident when there was no existing floodplain habitat. Juvenile Chinook salmon in the Sacramento River Basin rear in freshwater for 12 to 20 weeks, on average (Kjelson et al., 1982). Thus, survival of juveniles rearing in in-channel habitats under the low survival and small body size effect on survival for 12 and 20 weeks was on average 8% and 2% (respectively), whereas survival averaged 11% and 3% (respectively) for those juveniles that reared in floodplain habitats for two of the weeks. This small increase in survival may be sufficient to result in a greater number of adult equivalent salmon under the floodplain restoration action. However, floodplain habitat also holds rearing juvenile fish and grows them at a higher rate, which can alter the timing and size of fish leaving the rearing areas and entering the ocean. Simulated juvenile survival was less than 0.5% for any size juvenile that entered the ocean less than seven weeks after the ocean productivity transition occurred and was less than 1% for small juveniles at any time after the transition. Thus, we believe that the advantage of floodplain habitat restoration over in-channel habitat restoration under low juvenile survival was that it provided a higher survival environment that held and grew juvenile salmon early in the rearing period and provided an advantage when making the transition to the ocean environment.
Model assumptions undoubtedly affected our results. Sensitivity analysis of the full DSM indicated that the model was very sensitive to fish territory size and growth (Peterson and Duarte, 2020), two factors that govern habitat capacity and movement in the DSM. We incorporated these same mechanisms in this evaluation. Here, habitat capacity was inversely related to fish body size with capacity greater for smaller fish than larger fish and any fish that exceeded capacity left the natal tributary. Habitat capacity for juvenile salmonids is highly uncertain and can vary substantially in space and time (Beakes et al., 2014; See et al., 2021; Hall et al., 2023). Thus, unsurprisingly a variety of methods have been used to model juvenile salmon habitat capacity ranging from territory size (Grant and Kramer, 1990; this study), fixed numerical estimates based on published field observations (Hendrix et al., 2019), and density dependent functions calibrated using sample data (Perry et al., 2018). Similar to our DSM, many of these approaches postulate that fish that exceed habitat capacity leave the tributary and there is some empirical support for this mechanism (Connor et al., 2013). Were this the true mechanism governing habitat use by salmon in the natural environment, we expect our results to only differ qualitatively relative to the upper limit defining the amount of habitat sufficient to support most rearing juveniles (i.e., high existing in-channel habitat availability). However, there is also evidence of density dependent mortality in rearing juvenile salmon (Achord et al., 2003). This density dependent mechanism has been incorporated into some salmon life cycle models as a function of habitat capacity (Lee and Hyman, 1992; Greene and Beechie, 2004), so that fish died at a higher rate when capacity was exceeded rather than leaving the system. If this was the true density dependent response in the natural environment, we would expect our efforts to overestimate the benefits of floodplain habitat restoration because the individuals that experienced the benefits associated with a with floodplain inundation would remain in the tributary post-flooding and die at a higher rate rather than leave and potentially contribute to future adults. Within this evaluation, we only considered natal tributaries and assumed all fish that moved downstream were actively outmigrating to the ocean. In reality, fish leaving their natal tributaries have access to rearing habitats in the mainstem sections of the Sacramento and San Joaquin Rivers. We chose to make this simplifying assumption to focus on the tradeoffs in restoring perennial (in-channel) vs ephemeral (floodplain) habitats to support rearing fish. However, it is worth noting that the inclusion of this process within the modeled scenarios is equivalent to the scenarios that included increased current in-channel and floodplain habitat availability. Thus, the patterns uncovered would not change. Finally, we fully recognize that our evaluations do not consider all the current and potential future conditions that these fish will likely encounter throughout their life cycle (i.e., climate change, contaminants, etc.). Although such extensions could certainly be included in future evaluations, we stress that DSMs are meant to be abstractions of reality to help guide the conversations within the decision-making process. Requisite DSMs that only include the processes relevant to the decision(s) at hand are often preferred in order to avoid unnecessary complexity and, by extension, increase the interpretability of the simulation results and a decision makers ability to understand system dynamics (Phillips, 1984).
Natural resource managers are often faced with difficult decisions on how to best allocate resources to most efficiently and effectively meet restoration goals. Our evaluation suggests that directing resources to restoring perennial in-channel habitats that are reliably available for the duration of the rearing period is optimal when rearing habitats are limiting. This is not to imply that floodplain habitats are unimportant. There is extensive evidence that floodplains serve important roles in lotic ecosystems (Junk et al., 1989; Petsch et al., 2023). Rather, our modeling efforts suggest floodplain habitats do provide additional benefits provided there is sufficient in-channel habitats to support the greater number and larger sized juvenile fish. Importantly, similar to Peterson and Duarte (2020), our results provide quantitative evidence that there are some situations where restoring habitat may have unintended negative impacts on fish populations and the optimal restoration action is no action at all. Thus, our study reinforces that the context of the decision space matters and that no action alternatives are a worthwhile scenario to consider within any decision analytic process.
Data availability statement
Publicly available datasets were analyzed in this study. This data can be found here: https://github.com/CVPIA-OSC.
Author contributions
JP: Conceptualization, Data curation, Formal analysis, Funding acquisition, Investigation, Methodology, Project administration, Resources, Software, Supervision, Validation, Visualization, Writing – original draft, Writing – review & editing. AD: Conceptualization, Data curation, Formal analysis, Funding acquisition, Investigation, Methodology, Software, Validation, Visualization, Writing – original draft, Writing – review & editing.
Funding
The author(s) declare financial support was received for the research, authorship, and/or publication of this article. Funding for this project was provided by the U.S. Bureau of Reclamation under agreement number R23PG00088.
Acknowledgments
We appreciate the efforts of the all the SIT members over the years and especially thank R. Wittler, H. Casillas, S. Whiteman, and N. Wandelear. We also thank M. Urkov, M. Thompkins, S. Gill, M. Wright, and E. Rodriguez for support in developing the DSM, and SIT website; and reviewers for providing a thorough review of an earlier version of this manuscript. AD would also like to acknowledge support from the U.S.D.A. Forest Service, Pacific Northwest Research Station. The Oregon Cooperative Fish and Wildlife Research Unit is jointly sponsored by the U.S. Geological Survey, the U.S. Fish and Wildlife Service, the Oregon Department of Fish and Wildlife, Oregon State University, and the Wildlife Management Institute.
Conflict of interest
The authors declare that the research was conducted in the absence of any commercial or financial relationships that could be construed as a potential conflict of interest.
Publisher’s note
All claims expressed in this article are solely those of the authors and do not necessarily represent those of their affiliated organizations, or those of the publisher, the editors and the reviewers. Any product that may be evaluated in this article, or claim that may be made by its manufacturer, is not guaranteed or endorsed by the publisher.
Author disclaimer
Any use of trade, firm, or product names is for descriptive purposes only and does not imply endorsement by the U.S. Government.
Supplementary material
The Supplementary Material for this article can be found online at: https://www.frontiersin.org/articles/10.3389/fcosc.2024.1428697/full#supplementary-material
References
Achord S., Levin P., Zabel R. (2003). Density−dependent mortality in Pacific salmon: the ghost of impacts past? Ecol. Lett. 6, 335–342. doi: 10.1046/j.1461-0248.2003.00438.x
Akaike H. (1973). ““Information theory and an extension of the maximum likelihood principle,”,” in Second international symposium on information theory. Eds. Petrov B. N., Csaki F. (Akademiai Kiado, Budapest, Hungary), 267–281.
Beakes M., Moore J., Retford N., Brown R., Merz J., Sogard S. (2014). Evaluating statistical approaches to quantifying juvenile Chinook salmon habitat in a regulated California river. River Res. Appl. 30, 180–191. doi: 10.1002/rra.2632
BenDor T., Lester T., Livengood A., Davis A., Yonavjak L. (2015). Estimating the size and impact of the ecological restoration economy. PloS One 10, e0128339. doi: 10.1371/journal.pone.0128339
Bodin B., Garavaglia V., Pingault N., Ding H., Wilson S., Meybeck A., et al. (2022). A standard framework for assessing the costs and benefits of restoration: introducing The Economics of Ecosystem Restoration. Restor. Ecol. 30, e13515. doi: 10.1111/rec.13515
Bourret S., Caudill C., Keefer M. (2016). Diversity of juvenile Chinook salmon life history pathways. Rev. Fish Biol. Fish. 26, 375–403. doi: 10.1007/s11160-016-9432-3
Connor W., Tiffan K., Plumb J., Moffitt C. (2013). Evidence for density-dependent changes in growth, downstream movement, and size of Chinook Salmon subyearlings in a large-river landscape. Trans. Am. Fish. Soc 142, 1453–1468. doi: 10.1080/00028487.2013.806953
Costantino R., Cushing J., Dennis B., Desharnais R., Henson S. (1998). Resonant population cycles in temporally fluctuating habitats. Bull. Math. Biol. 60, 247–273. doi: 10.1006/bulm.1997.0017
Cushing J. (1987). Oscillatory population growth in periodic environments. Theor. Popul Biol. 30, 289–308. doi: 10.1016/0040-5809(86)90038-9
Deitch M., Sapundjieff M., Feirer S. (2017). Characterizing precipitation variability and trends in the world’s Mediterranean-climate areas. Water. 9, 259. doi: 10.3390/w9040259
Dudley P., Hendrix A., Osterback A. (2023). A meta-analysis and model comparison of juvenile salmon growth across different habitat types. River Res. Appl. 39, 177–188. doi: 10.1002/rra.4078
Farly L., Hudon C., Cattaneo A., Cabana G. (2019). Seasonality of a floodplain subsidy to the fish community of a large temperate river. Ecosyst. 22, 1823–1837. doi: 10.1007/s10021-019-00374-w
Gibson R. (1994). Impact of habitat quality and quantity on the recruitment of juvenile flatfishes. Neth. J. Sea Res. 32, 191–206. doi: 10.1016/0077-7579(94)90040-X
Górski K., Collier K., Duggan I., Taylor C., Hamilton D. (2013). Connectivity and complexity of floodplain habitats govern zooplankton dynamics in a large temperate river system. Freshw. Biol. 58, 1458–1470. doi: 10.1111/fwb.12144
Grant J., Kramer D. (1990). Territory size as a predictor of the upper limit to population density of juvenile salmonids in streams. Can. J. Fish. Aquat. Sci. 47, 1724–1737. doi: 10.1139/f90-197
Greene C., Beechie T. (2004). Consequences of potential density-dependent mechanisms on recovery of ocean-type Chinook salmon (Oncorhynchus tshawytscha). Can. J. Fish. Aquat. Sci. 61, 590–602. doi: 10.1139/f04-024
Hall J., Roni P., Ross K., Camp M., Nuckols J., Ruffing C. (2023). Estimating juvenile salmon estuarine carrying capacities to support restoration planning and evaluation. Estuaries Coasts. 46, 1046–1066. doi: 10.1007/s12237-023-01185-y
Hendrix N., Osterback A., Jennings E., Danner E., Sridharan V., Greene C., et al. (2019). Model description for the Sacramento River winter-run Chinook salmon life cycle model (National Marine Fisheries Service Southwest Fisheries Science Center Fisheries Ecology Division). Available online at: https://oceanview.pfeg.noaa.gov/wrlcm/documents/publications/Hendrix%20et%20al%202019_WRLCM%20Description.pdf (Accessed March 10, 2024).
Höhl M., Ahimbisibwe V., Stanturf J., Elsasser P., Kleine M., Bolte A. (2020). Forest landscape restoration—What generates failure and success? Forests. 11, 938. doi: 10.3390/f11090938
Holt R. (2008). Theoretical perspectives on resource pulses. Ecology. 89, 671–681. doi: 10.1890/07-0348.1
Honea J., Jorgensen J., McClure M., Cooney T., Engie K., Holzer D., et al. (2009). Evaluating habitat effects on population status: influence of habitat restoration on spring-run Chinook Salmon. Freshw. Biol. 54, 1576–1592. doi: 10.1111/j.1365-2427.2009.02208.x
Hurvich C., Tsai C. (1989). Regression and time series model selection in small samples. Biometrika. 76, 297–307. doi: 10.1093/biomet/76.2.297
Jeffres C., Opperman J., Moyle P. (2008). Ephemeral floodplain habitats provide best growth conditions for juvenile Chinook salmon in a California river. Environ. Biol. Fishes. 83, 449–458. doi: 10.1007/s10641-008-9367-1
Junk W., Bayley P., Sparks R. (1989). ““The flood pulse concept in river-floodplain systems,”,” in Proceedings of the international large river symposium (LARS), vol. 106 . Ed. Dodge D. (Ottawa, Ontario: Can. Spec. Publ. Fish. Aquat. Sci), 110–127.
Kaylor M., White S., Sedell E., Warren D. (2020). Carcass additions increase juvenile salmonid growth, condition, and size in an interior Columbia River Basin tributary. Can. J. Fish. Aquat. Sci. 77, 703–715. doi: 10.1139/cjfas-2019-0215
Kjelson M., Raquel P., Fisher F. (1982). ““Life history of fall-run juvenile chinook salmon, Oncorhynchus tshawytscha, in the Sacramento-San Joaquin estuary, California,”,” in Estuarine comparisons. Ed. Kennedy V. (Academic Press, New York, NY), 393–411.
Lee D., Hyman J. (1992). The stochastic life-cycle model (SLCM): simulating the population dynamics of anadromous salmonids (Boise ID: U.S. Department of Agriculture, Forest Service, Intermountain Research Station). Research Paper INT-459. doi: 10.5962/bhl.title.68863
Nisbet R., Gurney W. (1976). Population dynamics in a periodically varying environment. J. Theor. Biol. 56, 459–475. doi: 10.1016/S0022-5193(76)80086-0
Opperman J., Moyle P., Larsen E., Florsheim J., Manfree A. (2017). Floodplains: processes and management for ecosystem services (Berkeley, CA: Univ. of California Press).
Orland M., Lawler S. (2004). Resonance inflates carrying capacity in protist populations with periodic resource pulses. Ecology. 85, 150–157. doi: 10.1890/02-0372
Perry R., Plumb J., Jones E., Som N., Hetrick N., Hardy T. (2018). Model structure of the stream salmonid simulator (S3) - A dynamic model for simulating growth, movement, and survival of juvenile salmonids (Reston, VA: U.S. Geological Survey Open-File Report 2018-1056).
Peterson J., Duarte A. (2020). Decision analysis for greater insights into the development and evaluation of Chinook salmon restoration strategies in California's Central Valley. Restor. Ecol. 28, 1596–1609. doi: 10.1111/rec.13244
Petsch D., Cionek V., Thomaz S., Dos Santos N. (2023). Ecosystem services provided by river-floodplain ecosystems. Hydrobiologia. 850, 2563–2584. doi: 10.1007/s10750-022-04916-7
Phillips L. (1984). A theory of requisite decision models. Acta Psychologica 56, 29–48. doi: 10.1016/0001-6918(84)90005-2
R Core Team (2023). R: A language and environment for statistical computing (Vienna, Austria: R Foundation for Statistical Computing). Available at: https://www.R-project.org/.
Satterthwaite W., Carlson S., Allen-Moran S., Vincenzi S., Bograd S., Wells B. (2014). Mach-mismatch dynamics and the relationship between ocean-entry timing and relative ocean recoveries of Central Valley fall-run Chinook Salmon. Mar. Ecol. Prog. Ser. 511, 237–248. doi: 10.3354/meps10934
See K., Ackerman M., Carmichael R., Hoffmann S., Beasley C. (2021). Estimating carrying capacity for juvenile salmon using quantile random forest models. Ecosphere 12, e03404. doi: 10.1002/ecs2.3404
USBR, USFWS (2020). Near-term restoration strategy for the central valley project improvement act fish resource area FY2021–FY2025. Available online at: https://cvpia-documents.s3-us-west-1.amazonaws.com/CVPIA_Near-term-Restoration-Strategy_FY21-FY25_FINAL.pdf (Accessed March 10, 2024).
Williams P., Andrews E., Opperman J., Bozkurt S., Moyle P. (2009). Quantifying activated floodplains on a lowland regulated river: its application to floodplain restoration in the Sacramento Valley. San Francisco Estuary Watershed Sci. 7. doi: 10.15447/sfews.2009v7iss1art4
Keywords: Chinook salmon, Central Valley, pulsed resource, decision model, simulation
Citation: Peterson JT and Duarte A (2024) An evaluation of tradeoffs in restoring ephemeral vs. perennial habitats to conserve animal populations. Front. Conserv. Sci. 5:1428697. doi: 10.3389/fcosc.2024.1428697
Received: 06 May 2024; Accepted: 18 June 2024;
Published: 02 July 2024.
Edited by:
James Nichols, University of Florida, United StatesReviewed by:
Jason T. Magnuson, United States Geological Survey, United StatesBrett Harvey, California Department of Water Resources, United States
Copyright © 2024 Peterson and Duarte. This is an open-access article distributed under the terms of the Creative Commons Attribution License (CC BY). The use, distribution or reproduction in other forums is permitted, provided the original author(s) and the copyright owner(s) are credited and that the original publication in this journal is cited, in accordance with accepted academic practice. No use, distribution or reproduction is permitted which does not comply with these terms.
*Correspondence: James T. Peterson, jt.peterson@oregonstate.edu