- 1Centro Nacional de Pesquisa e Conservação da Biodiversidade Amazônica, Instituto Chico Mendes de Conservação da Biodiversidade (ICMBio), Manaus, Brazil
- 2Finnish Museum of Natural History, University of Helsinki, Helsinki, Finland
- 3Department of Biology, University of Turku, Turku, Finland
- 4Laboratório de Genética e Evolução Animal, Departamento de Genética, Universidade Federal do Amazonas, Manaus, AM, Brazil
- 5Department of Environmental Science and Policy, George Mason University, Fairfax, VA, United States
- 6Department of Biology, Trinity University, San Antonio, TX, United States
- 7Instituto de Ciências Biológicas, Departamento de Biologia, Universidade Federal do Amazonas, Manaus, Brazil
Saguinus bicolor is one of the most endangered primates in the Amazon, mainly due to threats related to human activities, such as deforestation and habitat fragmentation. Moreover, the encroachment of Saguinus midas, a widely distributed species, into the restricted range of S. bicolor, poses further conservation challenges and extinction risks. This study focuses on the potential niche overlap and competition between these two species, which are closely related callitrichid primates with a parapatric distribution. We employed the support vector machine (SVM) algorithm to investigate the potential of invasion by S. midas into the area of occurrence of S. bicolor by quantifying and comparing the habitat suitability for both species. We used environmental variables that are known to be relevant descriptors of callitrichid niches and a similarity test was applied to assess niche overlap between the two species. Our analysis reveals that the majority of the area occupied by S. bicolor exhibits higher habitat suitability for S. midas than for S. bicolor. Furthermore, we found a high niche similarity between the species. These results suggest a significant invasive potential of S. midas into the range of S. bicolor. The research highlights the concerning prospects for the long-term persistence of S. bicolor, emphasizing the urgent need for conservation measures. The conservation and maintenance of an ecologically functional urban forest matrix, along with the preservation of habitat quality and connectivity in rural areas, are crucial. Monitoring the current contact areas between the two species and the areas indicated as highly and moderately suitable for S.midas in the range of S. bicolor is also essential, and the observed trends in the occupation of these areas need to be incorporated into adaptive conservation strategies to protect less anthropogenically impacted areas. The findings are expected to contribute to the ongoing National Action Plan for the conservation of S. bicolor and support the management and conservation efforts for the species.
1 Introduction
The role of niche overlap and interspecific competition in determining species ranges and coexistence is vigorously debated (Lehman, 2000; Schreier et al., 2009; Kamilar and Ledogar, 2011; Wisz et al., 2013; Weber and Strauss, 2016). In a comprehensive review, Schreier et al. (2009) found that niche partitioning among co-existing primate species was driven by differences in diet (28%), use of different forest substrates (25%), and use of different forest types (14%). Stable coexistence between species is determined by factors such as fitness traits and niche differences, and we would expect that at a local scale, the more diverged the two species’ niches are, the greater the chances of coexistence (Chesson, 2000). However, as a result of ecological niche conservatism, the more closely phylogenetically related two species are, the greater the niche overlap between them (Peterson et al., 1999; Webb et al., 2002). Likewise, it is expected that high niche similarity between species leads to greater chances of interspecific competition (Gavilanez and Stevens, 2013; Weber and Strauss, 2016). We know, for example, that for many sympatric primate species, shared characteristics (e.g. similar diet and body mass) can increase interspecific competition (Ganzhorn, 1999). Lehman (2000) suggested that closely related species of Cebus in Guyana may exhibit negative patterns of interspecific association, potentially influencing their coexistence in the same habitats due to similarities in ecological requirements and resource use. Kamilar and Ledogar (2011) indicate that some primate species pairs may never be found in the same community due to competitive exclusion, even in areas where the environmental conditions are suitable for both species. Interspecific competition can also lead to parapatric distributions, potentially resulting in areas of sympatry within narrow contact zones (Coyne and Orr, 2004).
The pied tamarin (Saguinus bicolor) and the red-handed tamarin (Saguinus midas) are two closely related callitrichid primates (Lopes et al., 2023) with a parapatric distributions (Röhe, 2006). Saguinus bicolor is recognized as one of the 25 most endangered primate species worldwide (in press, 2024) and exhibits a notable degree of endemism among Amazonian primates, with its range estimated at approximately 7,500 km2 (Röhe, 2006). The species is assessed as critically endangered by both the IUCN and the Brazilian Ministry of Environment, with main threats stemming from deforestation and habitat fragmentation, mainly related to expanding rural settlements, agricultural and urban development (Gordo et al., 2021). Saguinus bicolor groups range from 2 to 13 individuals and are extremely territorial, with low population densities (1-2 groups/km2) throughout their distribution (Gordo, 2012). Saguinus bicolor occurs in a densely populated area, largely within the greater metropolitan area of Manaus, the capital of the Brazilian state of Amazonas. The distribution of S. bicolor is limited by the confluence of the Amazon and Negro rivers in the south and southwest, and the Cuieiras and Urubu rivers in the northwest and east, respectively. The northern boundary does not constitute a physical barrier but rather is demarcated by a narrow contact zone with S. midas, a species widely distributed through the southern Guiana Shield (Röhe, 2006).
Saguinus midas is classified as least concern by the IUCN, with a stable population trend. Saguinus midas groups range from 2 to 7 individuals, with population densities varying between 0.4 and 5.4 groups/km² throughout their distribution (Mittermeier et al., 2021). Its known distribution covers nearly the entirety of the region north of the Amazon River and east of the Branco, Essequibo, and Negro rivers, occurring in Brazil, Suriname, Guyana, and French Guiana (Mittermeier et al., 2021). The species has been described as highly adaptable and appears to be expanding its range (Ayres et al., 1982). Saguinus midas and S. bicolor share certain common characteristics. Both species show tolerance to environmental degradation and fragmentation (Ferrari, 1993; Gordo et al., 2013), occurring over a great diversity of habitats (e.g., primary forests: Gordo et al., 2008; Rodrigues and Vidal, 2011; edge habitats, dense understories and secondary growth: Rylands and Keuroghlian, 1989; Ferrari, 1993; disturbed, anthropized and peripheral habitat: Johns and Skorupa, 1987; Ferrari, 1993; Gordo et al., 2013). Furthermore, these two species are morphologically similar (Hershkovitz, 1977), showing similarity in food habits and seasonal balance in the use of resources (Egler, 1992; Pack et al., 1999). Additionally, the recent evolutionary divergence between S. bicolor and S. midas, estimated to have taken place approximately 2.97 million to 4.16 million years ago (Brcko et al., 2022; Lopes et al., 2023), further supports the notion of niche conservatism and potential niche overlap between these species.
Since the 1990s, studies have indicated that S. midas has been expanding its range specifically into areas previously occupied by S. bicolor, which consequently suffered decrease in its range (Subirá, 1998; Röhe, 2006; Gordo et al., 2021). The encroachment of S. midas into the range of S. bicolor, combined with evidence of hybridization between the species (Röhe, 2006; Gordo et al., 2021) suggests that S. bicolor is potentially threatened by competition with S. midas. However, in the two instances where we observed encounters between groups of the two species during this study, we did not observe any aggressive interactions between S. midas and S. bicolor. This is consistent with Röhe (2006), who reported four episodes of encounters between groups of these species with no aggressive interactions, suggesting that it is unlikely that this type of interaction is determining the spatial segregation observed between these two species. Nonetheless, Sobroza et al. (2021a) found that S. midas displayed more long calls while listening to S. bicolor calls than to its own species in sympatric areas, indicating a potentially higher level of aggressiveness or territorial behavior. This contrasts with findings in allopatric areas, where S. midas responded more to calls of its own species than to those of the congener. Observations of specimens exhibiting phenotypic characteristics indicative of hybrid animals have already been made. During field campaigns in an area of contact between the species, a group of three S. midas individuals was observed accompanying a group of seven S. bicolor, with one S. midas individual exhibiting phenotypic characteristics of a hybrid, including lighter fur and larger size. Another indication of hybridization between the species was found in the phylogenetic analyses of de Oliveira (2014), where one of the individuals that presented phenotypes corresponding to S. midas, originating from the contact zone between the two species, was grouped with S. bicolor.
We know that the range expansion of species, a well-documented phenomenon, can be driven by multiple factors including inter and intraspecific competition, favorable climatic conditions, genetic factors, resource availability, ecosystem modifications, stochastic colonization processes, and anthropogenic activities, among others (Excoffier et al., 2009; Gurevitch et al., 2011; Lowry et al., 2013; Wisz et al., 2013; Svenning et al., 2014; Pacifici et al., 2020). The demographic success of these species (Gurevitch et al., 2011) and the compatibility of the species’ niche with the invaded area (Shea and Chesson, 2002), are also important factors to consider. Additionally, species with large geographic ranges are more likely to establish themselves outside their original areas, particularly if they encounter climatic conditions similar to those in their original range of occurrence (Duncan et al., 2003; Clout and Russell, 2008). These characteristics raise concerns that S. midas has great potential to invade areas currently occupied by S. bicolor.
In this study, we employed ecological niche modeling (ENM) to investigate the invasive potential of S. midas within the current extent of occurrence (EOO) of S. bicolor. Habitat suitability for both species was evaluated using a set of relevant environmental variables known to describe the principal dimensions of niches of the family Callitrichidae. Our goal was to predict the areas of high habitat suitability for S. midas within the current EOO of S. bicolor. We also estimated common areas with high and moderate habitat suitability for both species, in order to identify potential areas of coexistence. This approach can contribute to understanding the potential invasion of S. midas into the EOO of S. bicolor and assess the conservation needs and strategies for areas suitable for S. bicolor.
2 Methods
2.1 Study area
The study area is located in central Amazonia, between the Uatumã River to the east, the Apuaú River to the west, the Negro and Amazonas rivers to the south and 2°0’ S latitude to the north (Figure 1). This area covers the entire historical (Ayres et al., 1982) and current (Gordo et al., 2021) known distribution of S. bicolor, plus a 60 to 80 km buffer beyond the species’ distribution limits and encompasses an area of approximately 318 km wide and 140 km long (28,020 km2).
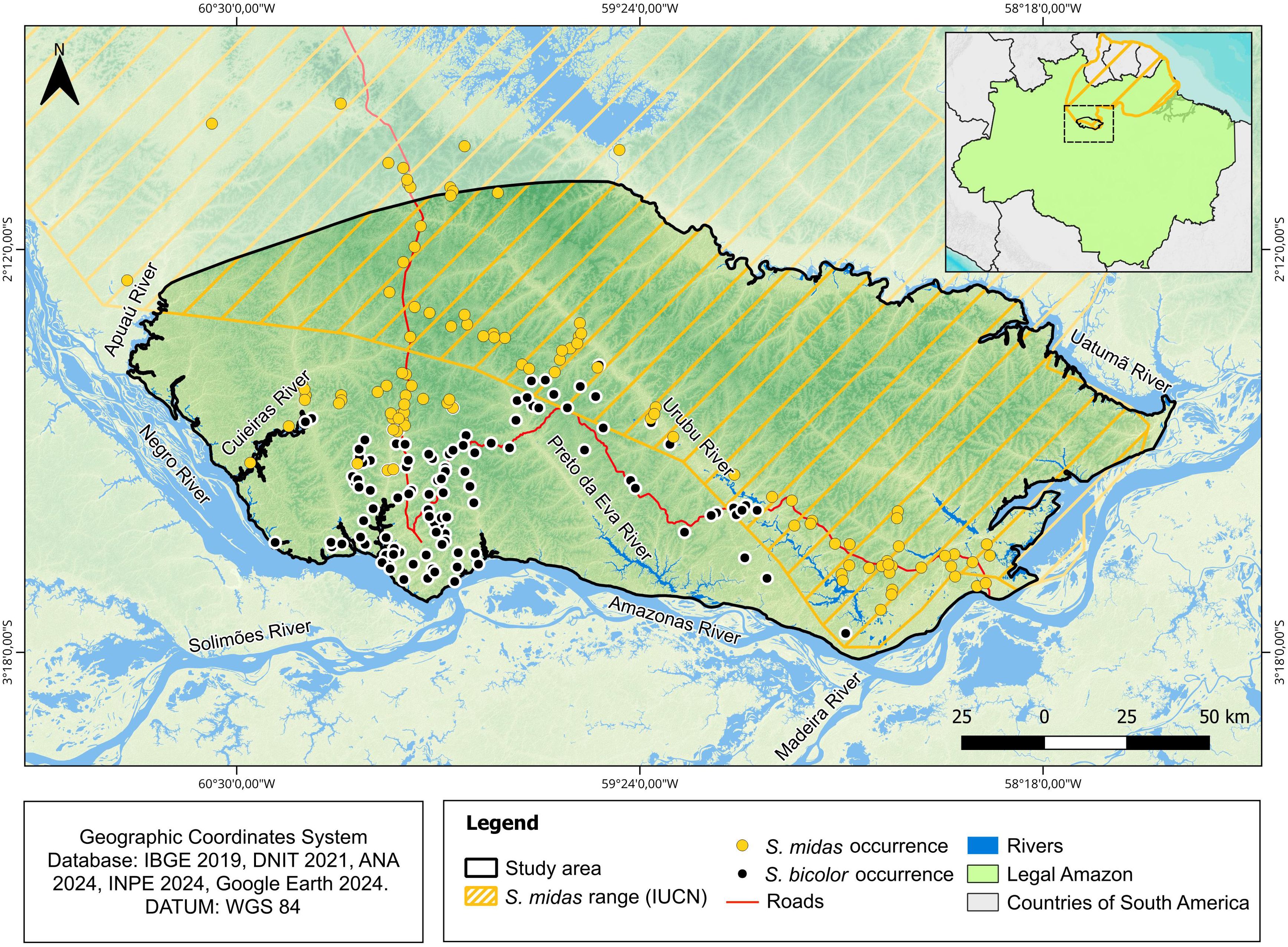
Figure 1. Location of the study area and the presence data of Saguinus bicolor (black dots) and Saguinus midas (yellow dots).
The vegetation consists of tropical humid terra firme forest in primary or secondary stages of succession. However, the rapid and disorderly occupation of urban and peri-urban areas of Manaus and surrounding regions turned the area into a deforestation hotspot in the Brazilian Amazon. This was triggered by the establishment of a free trade zone in the 1960s by the federal government as part of a strategy for the occupation and development of the Brazilian Amazon region, along with rural area occupation for agricultural activities (Gordo et al., 2013).
The study region features a tropical monsoon climate (“Am” according to the Köppen-Geiger classification), characterized by distinct dry and rainy seasons (Peel et al., 2007). During the drier months (from June to November), the average temperature is approximately 26.3 ± 1.3°C, while during the wetter months (from December to May) it is around 24.9 ± 1.1°C, with an annual average rainfall exceeding 2500 mm. Monthly rainfall averages 136 mm during the driest months and 288 mm during the wettest months (Aleixo et al., 2019).
2.2 Occurrence data
We obtained records of S. bicolor and S. midas from field observations in accessible areas that were pre-selected through satellite images. These areas were chosen to cover sampling gaps, especially in potential contact zones of the two species. In total, 818 areas were selected and visited between September 2019 and August 2021. These areas varied in size, ranging from small fragments of 1 hectare to extensive areas of continuous forest. Sampling was performed for at least 20 minutes in the smaller fragments and for at least 60 minutes in the continuous forest areas, where an observer conducted searches at 0.5 km/hour looking for Saguinus species. All sites were at least 1 km apart, and some sites were visited more than once if initial attempts to detect the animals were unsuccessful. We also sampled twelve 3 km trails opened in primary and secondary forest areas, six on each bank of the Urubu River, which in principle limits the distribution of S. bicolor to the east and S. midas to the west, respectively. These trails were surveyed monthly, until at least one occurrence record was obtained. The total number of occurrences registered for S. bicolor groups was 106 and for S. midas, 115.
In addition, we compiled occurrence records of S. bicolor (n=5) and S. midas (n=14) from gray literature (de Oliveira, 2014) and online datasets (n=15 for each species) from the Global Biodiversity Information Facility - GBIF (www.gbif.org) and SpeciesLink (splink.cria.org.br). Since S. midas has a wider distribution, we also used records from outside the focal studied area. However, in order to avoid including different lineages of S. midas that might have different niche requirements (e.g. Machado et al., 2019), we excluded records beyond the Trombetas River to the east and above the coordinate 1°50’ N to the north. This decision stems from genetic studies revealing two distinct intraspecific lineages of S. midas, separated by the Trombetas River (de Oliveira, 2014). Additionally, Vallinoto et al. (2006) observed genetic divergence between samples of S. midas from the Uatumã River and those from the Trombetas River, approximately 200 km apart. Moreover, we excluded records that were obtained before 1980 to avoid georeferencing inaccuracies and observations from localities that are currently deforested. We verified georeferencing errors by visually inspecting coordinates on the map. In cases of inconsistencies, we contacted the observer. Using these strategies, we were able to resolve inconsistencies for 3 records collected after the year 2000. Occurrence data outside the known distribution range for the species were excluded.
We used a randomization approach, through the ‘thin’ function in spThin R package, which returned a set of data with the maximum number of records with a minimal distance of 1 km between records to control the sampling bias from highly sampled areas while simultaneously retained the greatest amount of useful information (Aiello-Lammens et al., 2015). At the end of this process, we obtained 232 validated occurrence records, 123 for S. midas and 109 for S. bicolor. Out of the 232 records used in the analysis, 229 correspond to the period between 2005 and 2021. The three previous occurrences date back to 1983 and 2001 for S. bicolor and 1984 for S. midas. These occurrences were retained as they originate from regions where obtaining recent records for the species was not possible.
2.3 Environmental data
We compiled a set of 23 environmental variables that describe variations in temperature, precipitation, forest structure, habitat characteristics vegetation, soil and human impact. These factors have been shown to affect the distribution of our study species (Vidal and Cintra, 2006; Rodrigues and Vidal, 2011; Gordo, 2012; Gordo et al., 2013; Mittermeier et al., 2021). Furthermore, human activities have important implications for the invasive potential of generalist species (Gallardo et al., 2015).
2.3.1 Climatic data
We obtained bioclimatic variables from Worldclim V. 2.0 (http://www.worldclim.org/; Fick and Hijmans, 2017), for the period between 1970 and 2000, at 30 arc-seconds resolution. Since several of the 19 available bioclimatic variables are strongly related, we selected a subset of climatic variables for which we had evidence or which were strong candidates to be ecologically important for both species. The included bioclimatic variables were isothermality (BIO3), temperature seasonality (BIO4), precipitation of the wettest month (BIO13), precipitation of the driest month (BIO14), precipitation seasonality (BIO15) and precipitation of the warmest quarter (BIO18). Precipitation and temperature are important factors driving platyrrhine primate distributions and density in general (Wiederholt and Post, 2010; Duran et al., 2013; Cavalcante et al., 2020; Rabelo et al., 2020), including callitrichids specifically (Ochoa-Quintero et al., 2017; Braz et al., 2019; Rezende et al., 2020; Arias-Gonzalez et al., 2021). Furthermore, variations in temperature and precipitation influence insect biomass (Hernández-Zul et al., 2013; Ribeiro et al., 2013) and fruit productivity (Peres, 1994; Wiederholt and Post, 2010; Gordo, 2012), which form the basis of the diet of S. midas (Pack et al., 1999) and S. bicolor (Gordo, 2012). For example, for some grasshoppers (Orthoptera: Acrididae), an important item in the diet of species of Saguinus (Sussman and Kinzey, 1984; Ferrari, 1993), precipitation and temperature are the most important factors explaining variations in population density (Scattolini et al., 2020; Kistner-Thomas et al., 2021).
2.3.2 Vegetation index and forest structure
Vegetation traits influence the distribution of different Saguinus species, a group with wide amplitude of habitat use (e.g., primary forests: Gordo et al., 2008; Rodrigues and Vidal, 2011; edge habitats, dense understories and second growth: Rylands and Keuroghlian, 1989; Ferrari, 1993; disturbed, anthropized and peripheral habitat: Ferrari, 1993; Gordo et al., 2013). Therefore, we used the Normalized Difference Vegetation Index (NDVI) as a proxy for vegetation density and quality within each raster cell (Ponzoni and e Shimabukuro, 2007). Values of NDVI can range from -1 (water or bare ground) to 1 (healthy and dense vegetation) (Fitzgerald et al., 2018). This satellite-derived remote sensing metric has proven very useful in ecological studies, helping to improve our understanding of species-environment relationships (He et al., 2015). In some ecological niche models, for example, NDVI was an important variable in discriminating suitable from unsuitable habitat for primate species (Willems et al., 2009; Fitzgerald et al., 2018). This variable also accounts for the great variability in habitat use described for Saguinus species. To represent the current vegetation conditions, we downloaded a mosaic of raster images available at NASA’s Land Processes Distributed Active Archive Center (LP DAAC) website (lpdaac.usgs.gov/products/mod13a2v006/). The images were obtained by the MODIS sensor and are available with the NDVI values already processed at 30 arc-second spatial resolution (Didan, 2015). We used images from June 2020 to May 2021 to capture annual phenological variation (Bradley et al., 2011), calculating an arithmetic mean to create a final NDVI raster for the entire study area. Final raster values ranged from -0.19 to 0.89, indicating sparse to dense vegetation cover, respectively.
Canopy height (CH) was another factor that we considered ecologically relevant for the study species. The ability of arboreal mammals to travel, access resources or avoid predation, is highly influenced by the three-dimensional space of their habitat. Some studies have already demonstrated the importance of canopy height in describing habitat suitability for Neotropical primates (Palminteri et al., 2012; Gouveia et al., 2014; McLean et al., 2016), including callitrichids (Pyritz et al., 2010). Specifically for our two study species, Sobroza et al. (2021c) reported that S. midas shifted its vertical occurrence to higher strata in response to increased precipitation; this phenomenon was observed in areas of allopatry as well as sympatry with S. bicolor. However, this pattern was not evident in S. bicolor. To represent forest height and structure, we used a 30-m spatial resolution global forest canopy height map available at the Global Land Analysis and Discovery (GLAD) laboratory (https://glad.umd.edu/dataset/gedi/).
2.3.3 Human footprint
Human activities are also important drivers of species distributions, especially when predicting a species’ invasive potential (Acevedo et al., 2007; Gallardo et al., 2015; Pacifici et al., 2020). Saguinus bicolor and S. midas are known to occupy habitats with relatively high anthropogenic pressure and are known to adapt to landscape modifications (Ayres et al., 1982; Rylands and Keuroghlian, 1989; Ferrari, 1993; Röhe, 2006; Gordo et al., 2013). Previous studies hypothesized that S. midas is invading areas previously occupied only by S. bicolor and this invasion has been favored by the opening of roads and consequent changes in structure and availability of habitat (Ayres et al., 1982; Röhe, 2006).
To assess the influence of anthropogenic pressure and habitat modification on species distribution, we used the Global Human Footprint as a proxy to represent the extent of anthropogenic modification in our study area. We used the Dataset of the Last of the Wild Project, Version 2, 2005 (LWP-2) (WCS and CIESIN, 2005) available at 30 arc-second grid cell sizes. This variable is the Human Influence Index (HII) normalized by biome and realm. HII is based on nine layers of global data including population density patterns, land configurations (built-up areas, land use and land cover), electrical infrastructure and proxies to human access (i.e., coastlines, roads, railroads and navigable rivers). The dataset was produced by the Wildlife Conservation Society (WCS) and the Columbia University Center for International Earth Science Information Network (CIESIN) and can be accessed at https://sedac.ciesin.columbia.edu/data/set/wildareas-v2-human-footprint-geographic.
2.3.4 Soil nutrient concentration
Soil properties have also been reported to affect habitat use of Saguinus species at the local scale (Cárdenas Ramírez et al., 2021). Among the chemical characteristics of soils, the sum of the concentrations of exchangeable bases (or Sum of Bases; hereafter SB) has been shown to be a relevant indicator of nutrient concentration in soils. SB has been consistently related to floristic variation across the Amazonian lowland forests (Phillips et al., 2003; Pitman et al., 2008; Higgins et al., 2011; Tuomisto et al., 2016). Therefore, it determines forest characteristics, productivity and resource availability, which are potential drivers of the distribution of Saguinus species at the regional scale.
The SB maps availables for Amazonia are based on models that are fitted for the whole bioma (Zuquim et al., 2019, 2023). To improve local accuracy, we used the same approach of broad scale models, but restricting the model fit to our study region. Therefore, we created a raster of SB concentration focused on our study region. We applied a method proposed by Zuquim et al. (2019) to generate maps of the SB (Ca + Mg + K measured in cmol(+)/kg) that minimizes soil sampling data paucity by complementing field measurements with soil values estimated using indicator species records (Zuquim et al., 2014). We used the same direct and indirect soil estimates locations available in Zuquim et al. (2019) study to generate a soil map for our study area at 30 arc-second resolution. We evaluated map accuracy by using a 5-fold leave-one-out cross-validation procedure to access the mean squared deviation ratio (MSDR), the root mean squared error (RMSE) and the mean error (ME) (Brus et al., 2011; Vašát et al., 2013).
The mean square deviation ratio (MSDR) was 1.03, where values close to 1 indicate accuracy. The root-mean-square error (RMSE) was 0.54 and the mean error (ME) was 0.001945757. Smaller values of RMSE and ME indicate greater accuracy. The raster of soil cation concentration accuracy measures were satisfactory and therefore were used in ecological niche models.
2.4 Data analyses
We adopted a 30 arc-second resolution (~1 km near the Equator) in the analysis to match the original resolution of most of the environmental variables and because Saguinus species are known to have small home range sizes. Available home range estimates fluctuate between 32 and 145 ha (e.g. S. geoffroyi 32ha: Dawson, 1979; S. inustus 35ha: Palacios et al., 2004; S. midas 39ha: Kessler, 1998; S. labiatus 56ha: Rehg, 2006; S. bicolor 110ha: Gordo et al., 2008; S. mystax 145ha: Peres, 1993). We also expect that this resolution reflects the level at which biotic interactions between the species play a role (Acevedo et al., 2007; Sobroza et al., 2021a, b). Furthermore, the spatial resolution of our cleaned occurrence data is also at 1 km2. Canopy Height (CH) was rescaled to 30 arc-second resolution using the bilinear interpolation method, with the resample function in the raster package (Hijmans et al., 2018). All the other variables were already obtained at 30 arc-seconds resolution.
2.4.1 Multicollinearity among predictors
After defining our set of variables (n = 10: Table 1), we conducted a statistical variable selection procedure to ensure high predictability and minimize multicollinearity among predictor variables which is known to cause overfitting (Sillero et al., 2021). For this purpose, we calculated the variance inflation factor (VIF) with the vifcor function of the usdm R package (Naimi et al., 2014). This function first finds the pair of variables that has the maximum linear correlation (greater than a threshold), and exclude the one variable that has the greater VIF value. The function repeats the procedure until no covariates with high correlation coefficients are retained (Naimi et al., 2014). We set our correlation threshold to 0.5 and VIF criterion lower than 3. At the end, we retained seven variables that were used to model species distributions (Table 1).
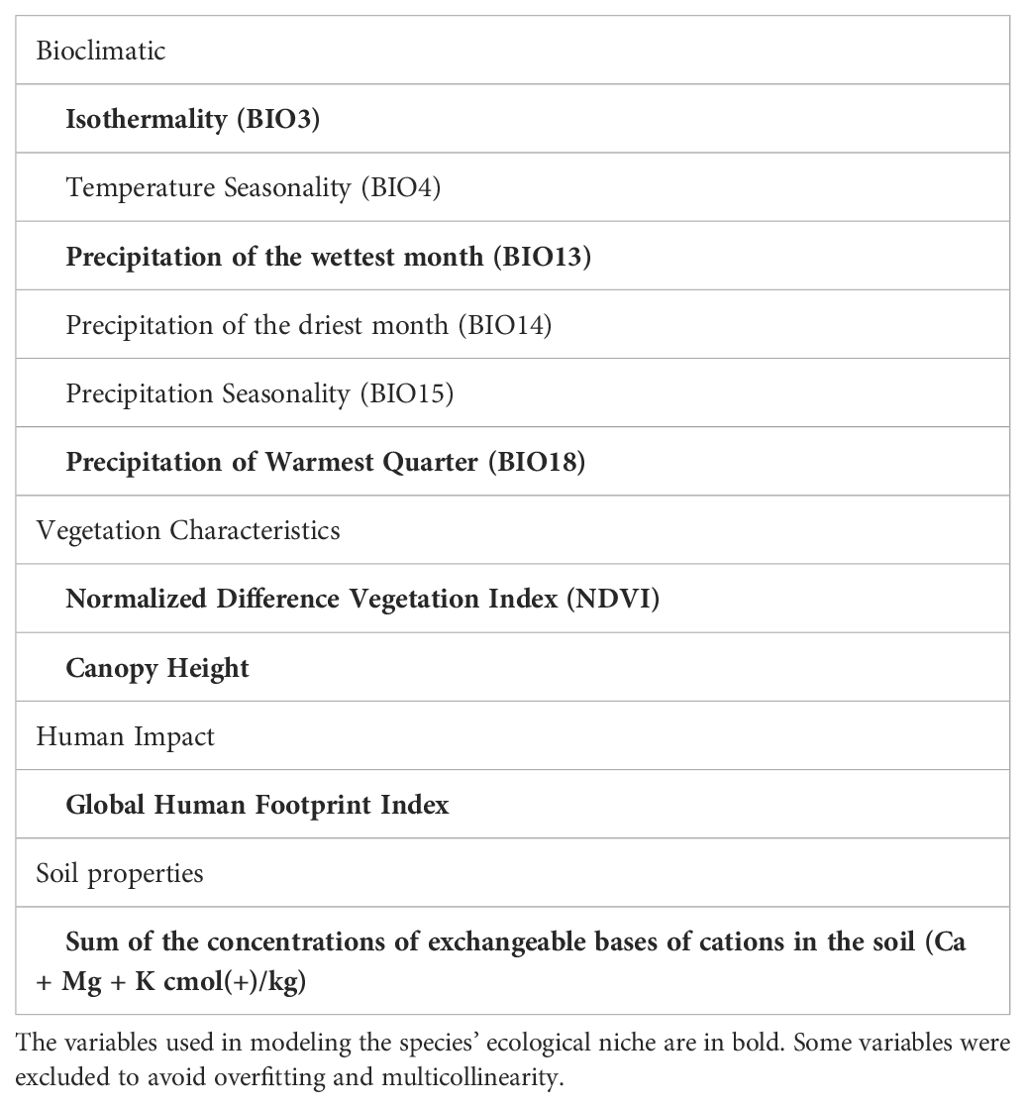
Table 1. Environmental variables that presumably influence the geographic distribution of S. bicolor and S. midas.
2.4.2 Niche modelling and model evaluation
We used the support vector machine (SVM) algorithm to model environmentally suitable areas for both species. SVM stands out as a nonparametric machine learning technique utilized for both regression and classification tasks, notably prevalent in ecological niche modeling (Guo et al., 2005; Drake et al., 2006; Giovanelli et al., 2010; Ashraf et al., 2017). Furthermore, its efficacy extends to enhancing the accuracy of range boundary estimations for closely-related parapatric species (Kass et al., 2021). The accuracy and reliability of ENMs built with SVMs have been examined extensively in prior studies (Drake et al., 2006; Giovanelli et al., 2010; Duan et al., 2014; Valavi et al., 2021).
Machine learning method results are slightly different every time a model is calibrated for the same dataset (Phillips et al., 2006, 2017). Consequently, it is important to train the model several times for the same dataset to evaluate if the results are stable across iterations, especially when using random selection of training and testing records (Guisan et al., 2017; Sillero and Barbosa, 2020). Additionally, the spatial configuration of the training data can affect the performance of species distribution models (Chaves et al., 2022). For this reason, we ran 100 model replicates for each species, and randomly divided our occurrence data for each run into 70% training and 30% testing data partitions.
For the sample selection of background points, we used the modelling strategy proposed by Liu et al. (2019) for SVM algorithms, which is expected to produce models with higher accuracy, specially for rare species (low prevalence) with few true presences. It is based on a small multiplier strategy, i.e. setting the number of random points a few times greater than the training dataset. For both species, we used a ratio of 4 random points for each true presence (see Liu et al., 2019 for details).
We used two evaluation metrics to assess the predictive performance of the models: the area under the receiver operating characteristic curve (AUC) and true skill statistic (TSS) metrics. All models with AUC values above the mean of the 100 replicates were retained to obtain the final probabilistic predictions (see Supplementary Figures 1, 2 of the Supplementary Material). We also calculated the standard deviation of the predicted suitability values for the 100 runs for each taxon (see Supplementary Figure 3 of the Supplementary Material).
2.4.3 Areas of potential coexistence and potential invasion scenarios of Saguinus midas
We reclassified the suitability maps for each species into three categories (following Acevedo et al., 2007) according to the habitat suitability model scores: low habitat suitability (0–0.33); moderate habitat suitability (0.34–0.66); and high habitat suitability (0.67–1). We summed these classified maps to identify areas of potential coexistence. Areas of overlapping high habitat suitability for both species, were classified as having a high potential for coexistence, whereas areas with high suitability for one species and moderate suitability for the other were classified as having a medium potential for coexistence. The sum of these areas was considered an area of potential coexistence of the two species.
Subsequently, we delineated the EOO for S. bicolor. According to the IUCN (2019), the EOO “must encompass all known, inferred or projected sites of present occurrence of a taxon”. Following these guidelines, we used all known occurrence records for the species, the habitat suitability maps and expert knowledge of the target species and study area. First, we drew a minimum convex polygon (MCP) around all occurrence records, plus a 4.5 km buffer (Gutiérrez et al., 2014; IUCN, 2019). We chose this buffer distance considering known home range sizes for the species (Gordo et al., 2008), and its dispersal ability (M. Gordo found a 3.1 km dispersal ability for S. bicolor, unpublished data from 2021; Dawson, 1979, found an average daily path length of 2061 ± 402 m for Saguinus geoffroyi). Then, we added to this convex hull, areas identified as having moderate or high habitat suitability for the species (IUCN, 2019; Kass et al., 2021). Finally, we drew the boundaries for the EOO polygon at the east, west and south of the distribution of S. bicolor using rivers that are known to limit its occurrence. We used the same MCP + buffer procedure to delineate the southern limits for the EOO polygon of S. midas. We added to this polygon a potential area of contact between S. midas and S. bicolor based on expert knowledge. We did not consider any other areas for S. midas beyond its respective MCP and buffer to allow a realistic assessment of the potential invasion scenarios.
For the potential invasion scenarios, we quantified areas of moderate and high habitat suitability for S. midas within the EOO of S. bicolor. We defined as a conservative invasion scenario when only areas of high habitat suitability for S. midas were within the EOO of S. bicolor, and a worst-case scenario, in which the sum of the moderate + high suitability areas for S. midas were within the EOO of S. bicolor. For spatial analyses, boundary delimitation, and area calculations, we employed QGIS 3.4.13, coordinate system SIRGAS 2000, Zona UTM 20S (QGIS Development Team, 2024).
2.4.4 Niche overlap and similarity test
We used the similarity test, as proposed by Broennimann et al. (2012), to assess the niche overlap between S. bicolor and S. midas across our study area. This method is based on the density of species occurrences in gridded environmental space. For this, we performed a Principal Component Analysis (PCA) with the 7 variables and all species occurrences used in the modeling and selected the first two principal components which we then used to calculate the Schoener D index (Schoener, 1968). This index ranges from 0 (= equivalent to no overlap in the environmental space) to 1 (= the two species completely sharing the same environmental space). The hypothesis of niche similarity is accepted when these empirically observed niche overlap estimates are significantly different from the values expected from 1000 randomly generated simulated values (see Warren et al., 2008; Broennimann et al., 2012). For this analysis, we used the functions available from the ecospat package, version 3.0 (Di Cola et al., 2017). Niche overlap visualizations were obtained using the niceOverPlot function for R, developed by J. Fernández-López (Real Jardín Botánico, Madrid, Spain). We finally used the ecospat.grid.clim.dyn function in the ecospat R package to compare ecological differences and similarities along individual gridded environmental gradients (R Core Team, 2021).
3 Results
We delimited the southeastern limit of the EOO of S. bicolor using a new occurrence record found during this study. We used the Urubu and Cuieiras rivers to delimit the eastern and western limits of the S. bicolor EOO, respectively. These rivers are considered geographic barriers for S. bicolor (Röhe, 2006; Gordo, 2012), but this is not true for S. midas, as this study recorded the species on both banks of Cuieiras (west) and Urubu rivers (east). The northern limit has no natural geographic barrier, but was delimited by a minimum convex polygon connecting all occurrence points, areas considered highly and moderately suitable and accessible, plus a 4.5 km wide buffer. The resulting EOO of S. bicolor had 8,354 km2 (Figure 2).
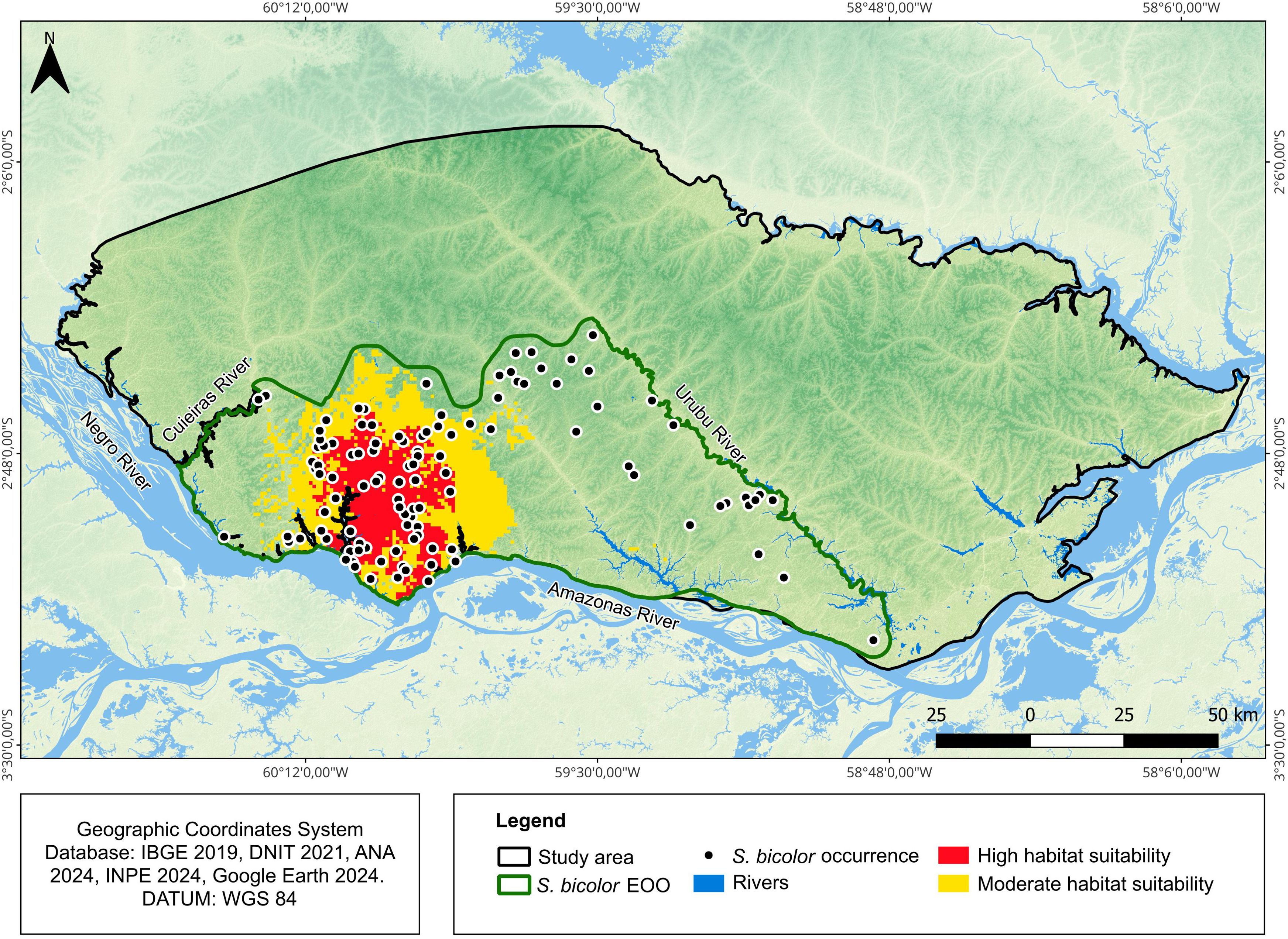
Figure 2. Map of the extension of occurrence (EOO) of S. bicolor (in green). The background image is based on satellite images from Google Earth and watercourse metadata from the Brazilian National Water Agency.
3.1 Ecological niche models
ENMs predicted that most of our study area was environmentally more suitable for S. midas than for S. bicolor. Areas with higher habitat suitability for S. bicolor were mostly concentrated within and around the city of Manaus, while S. midas showed broader areas of highly suitable habitats, mainly in the northwestern and southeastern limits of our study area (Figure 3). The models showed good accuracy and predictive performance based on the average score of AUC and TSS. For S. bicolor, AUC = 0.814 ± 0.045 and TSS = 0.574 ± 0.077 and for S. midas, AUC = 0.898 ± 0.030 and TSS = 0.733 ± 0.060 (mean ± SD).
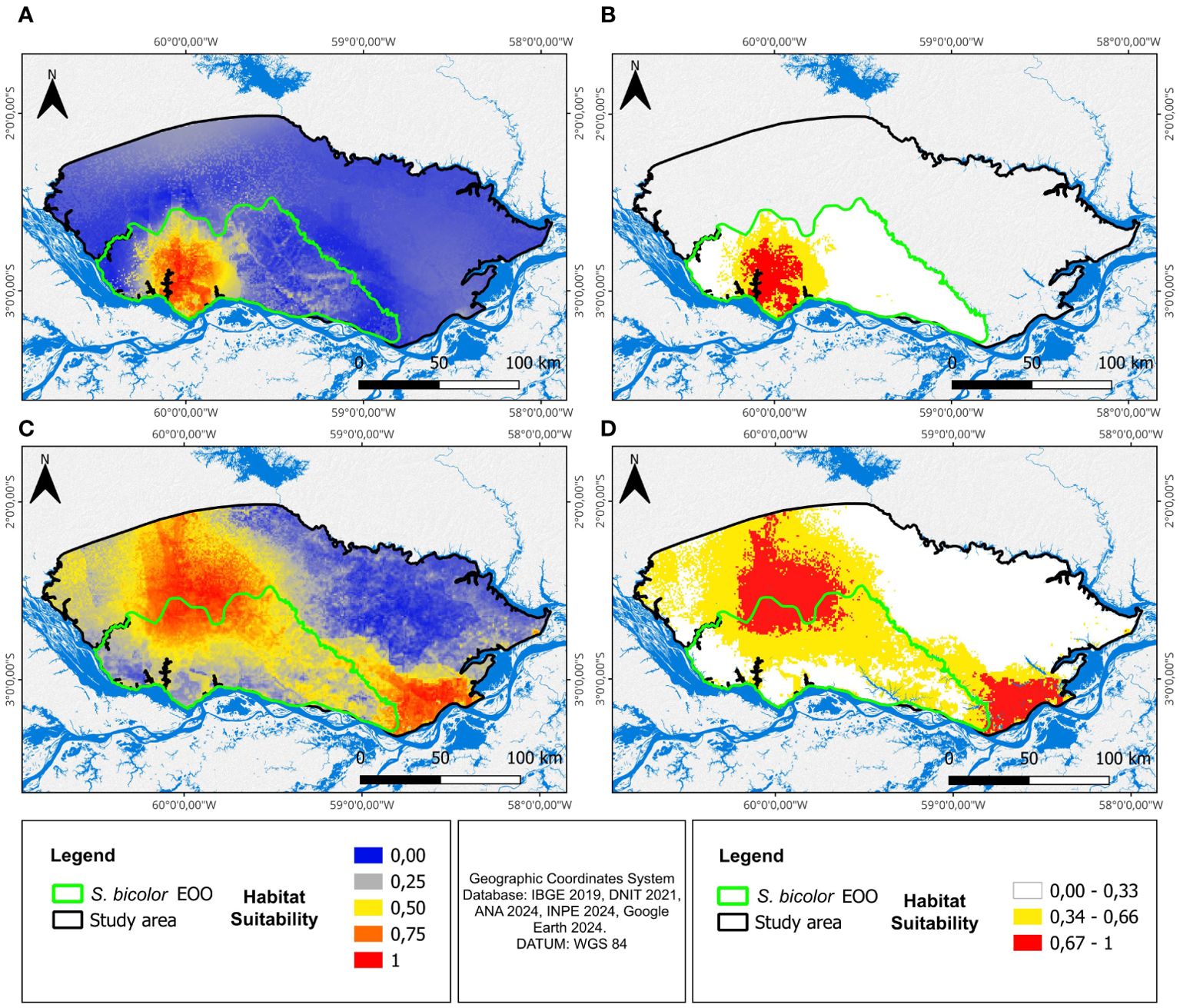
Figure 3. Habitat suitability maps. (A) General habitat suitability for S. bicolor; (B) Reclassified habitat suitability map for S. bicolor; (C) General habitat suitability for S. midas; (D) Reclassified habitat suitability map for S. midas.
The reclassified maps predicted an area of approximately 2,482 km2 with moderate (1,517 km2) and high (965 km2) habitat suitability for S. bicolor, which constitutes approximately 8.9% of our study area of 28,020 km2 and 29.7% of the EOO of S. bicolor (Figure 2). However, 1,609 km2 of the 2,482 km2 area with moderate and high habitat suitability for S. bicolor (64.8% of the area of moderate and high suitability EOO of S. bicolor) also has moderate or high habitat suitability for S. midas. Only 873 km2 (35.2% of moderate and high suitability EOO of S. bicolor and 10.5% of the total EOO of S. bicolor) are areas exclusively suitable for S. bicolor. Out of the 873 km2 of areas exclusively suitable for S. bicolor, 498 km2 are located in urban or projected urban expansion areas, as foreseen in the Master Plan of the City of Manaus (2021) and only 375 km2 are found in rural areas. For S. midas, the areas estimated as having moderate and high habitat suitability comprises approximately 49.2% of our study area (13,787 km2) and 63.8% of S. bicolor EOO (5,328 km2).
3.2 Potential coexistence and invasion scenarios
The models predicted an area of potential coexistence of 996,4 km2 (11.9% of S. bicolor EOO). These areas are intersected by one of the main federal roads (BR-174) in a north-south direction. Out of this total, only a small area at the northern limit of the S. bicolor EOO was classified with a high potential of coexistence (30 km2 or 0.36% of S. bicolor EOO, Figure 4).
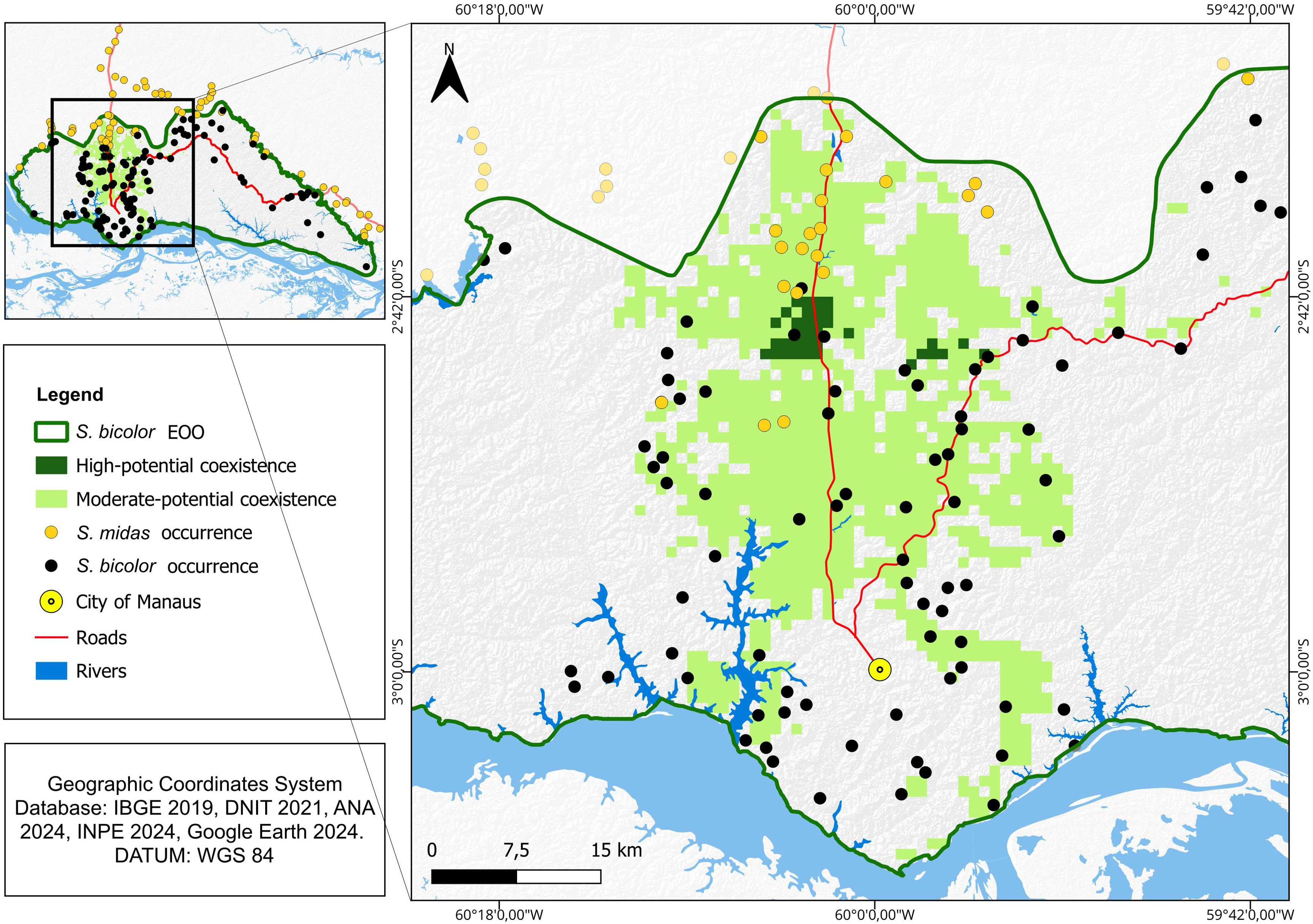
Figure 4. Areas of potential coexistence between Saguinus midas and Saguinus bicolor. In dark green the areas of high probability of coexistence (highly suitable for both species).
The ENM predicted that large areas of the EOO of S. bicolor had low habitat suitability for S. bicolor (5,872 km2 or 70.3%). In addition, 3,721 km2 (44.5%) of the EOO had low habitat suitability for S. bicolor, but medium or high habitat suitability for S. midas, which could facilitate invasion by the latter species. When considering S. midas as having the capacity to invade only areas with high habitat suitability (i.e., the conservative invasion scenario), we found that an area of 857.3 km2 within the S. bicolor EOO is potentially colonizable by S. midas. These areas are mainly located in the northern limits of the EOO of S. bicolor and constitute 10.3% of its EOO (Figure 5). There is also a small area of high habitat suitability for S. midas located at the southeastern limit of the EOO of S. bicolor, on the right bank of the Urubu River.
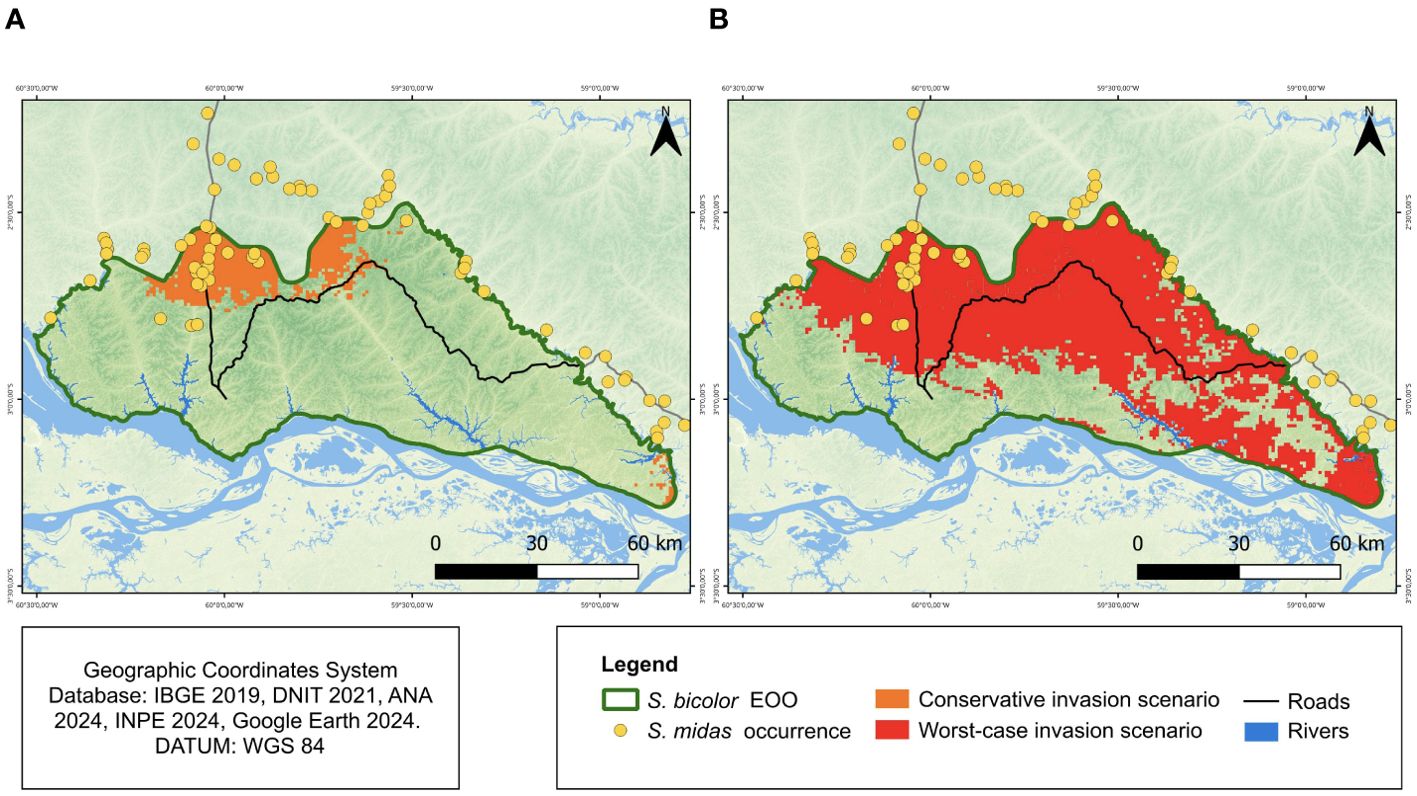
Figure 5. Areas of potential invasion of S. midas in the extent of occurrence of S. bicolor. (A) Conservative scenario, including areas of high suitability of habitat for S. midas and (B) Worst scenario, including areas of medium and high suitability of habitat for S. midas. The yellow dots are the occurrence records for S. midas groups.
The area of the EOO of S. bicolor potentially suitable for invasion increases considerably when we include areas of moderate habitat suitability for S. midas (i.e., the worst-case scenario). In this case, we calculated a total area of 5,113 km2, which constitutes 61.2% of the EOO of S. bicolor. These areas are located mainly in the northern segment of the EOO of S. bicolor, extending towards the city of Manaus, on the left bank of the Cuieiras River and the right bank of the Urubu River. The areas of moderate habitat suitability for S. midas also include the eastern limit of the EOO of S. bicolor, on the right bank of the Urubu River.
3.3 Niche overlap
PCA axes 1 and 2 accounted for 38% and 22% of the ecological variation in the occurrence records of both species (Figure 6). In axis 1, the variables that most influenced the ecological variation were human footprint and forest height. In axis 2, the variables that most influenced the ecological variation were NDVI and Bio18 (precipitation of warmest quarter), (see Supplementary Table 1 in the Supplementary Material).
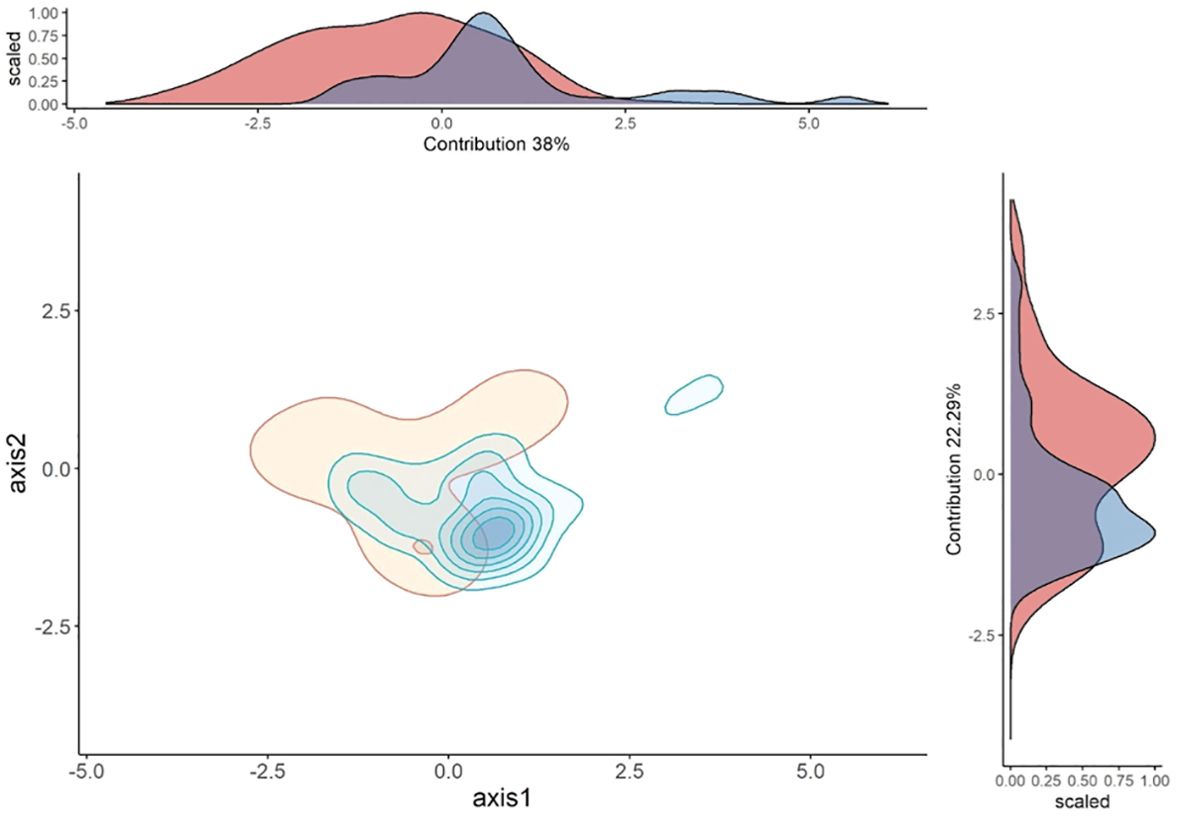
Figure 6. Niche overlap between S. bicolor (in blue) and S. midas (in red). The central graph represents the niche overlap between the two species in the two main axes of the PCA. In the upper margin we have a graph representing the niche overlap on axis 1 and on the right margin a graph representing the niche overlap on axis 2.
The similarity test indicated that the two niches were more similar to each other than to randomly selected niches (Schoener’s D = 0.39, p=0.013, see Supplementary Figure 4 in the Supplementary Material). The occurrence densities for S. bicolor along the anthropogenic gradient (human footprint variable) were higher in areas with higher anthropogenic pressure when compared to what we found for S. midas. In the gradient representing canopy height, the occurrence densities for S. midas were higher in the higher strata of the canopy, reaching 40 meters, while for S. bicolor it ranged from 0 meters (anthropized areas) to a maximum of 30 meters. In the soil properties gradient, the occurrence densities for S. midas were higher in soils with higher exchangeable base cations when compared to S. bicolor, where the occurrence densities were higher in soils with lower exchangeable base cations (See Supplementary Figures 5–7 in the Supplementary Material).
4 Discussion
In this study, we used ENMs to assess habitat suitability and predict the geographic distribution of the critically endangered primate Saguinus bicolor, and its potential competitor S. midas. We also estimated the current extent of occurrence of S. bicolor, and, in order to investigate the invasive potential of S. midas, we evaluated the habitat suitability in this area for the latter.
Our findings revealed that extensive areas of the EOO of S. bicolor (44.5%) has greater habitat suitability for S. midas then S. bicolor, which suggests a high potential for invasion by the former. Furthermore, S. midas has traits characteristic of species that have experienced recent range expansions, including being small-bodied and a habitat and resource generalist (Pacifici et al., 2020). Additionally, it occupies a large area of the Guiana Shield across which it experiences a broad range of habitats and resources. However, the two species are known to exhibit similar morphological, life history, and reproductive characteristics. Saguinus bicolor has an average weight of 513g with a standard deviation of 47.8g (n=41) (Gordo, 2012), while S. midas averages 590g with a standard deviation of ±70.7g (n=23) (Pack et al., 1999). The average head-body length for S. bicolor is 280-320mm (Gordo, 2012), compared to 230mm for S. midas (Hershkovitz, 1977). Typically, only one female per group breeds during a given season, with births usually being twins. Both species reach sexual maturity at around 2 years of age and have a generation time of approximately 6 years (Harvey et al., 1987; Gordo, 2012). The diet of the two species also appears to be highly similar (Pack et al., 1999; Gordo, 2012). These similarities make the species functionally analogous within their ecosystems, suggesting that differences in the use of their ecological niches may be influencing their distribution patterns. Nonetheless, previous studies have already suggested that S. midas is the more adaptable species (Ayres et al., 1982), and is likely to have a competitive advantage over S. bicolor (Ayres et al., 1982; Röhe, 2006; Sobroza et al., 2021a). This is supported by de Oliveira (2014) who reported that S. midas has a population growth rate three times greater than that observed for S. bicolor. Similarly, Farias et al. (2015) carried out a conservation genetic study that indicated a decreasing demographic trend for S. bicolor populations, which started at least 13,000 years ago.
The current EOO of S. bicolor (8,354 km2) estimated in this study is 854 km2 larger than the EOO proposed by Röhe (2006). This expansion of EOO includes an area of approximately 450 km2 located in the northeastern section of our study area, where we documented eight new occurrence records for S. bicolor. Due to difficulties in accessing this area, this region was not sampled in previous studies, but with the recent opening of new dirt roads - a concerning factor since this is a vector for anthropogenic occupation and deforestation - it was possible to observe S. bicolor groups in this area. However, even with the occurrence records of S. bicolor, our model did not predict it as an area of medium or high habitat suitability for the species. Similarly, despite records of groups of S. midas, our models were not able to predict it as an area of potential coexistence. The lack of prediction for these areas as medium or highly suitable habitats or potential coexistence zones may be attributed to several factors (Kass et al., 2021; Sillero et al., 2021). Despite our careful efforts to avoid this type of bias in our sampling design, the data used to construct the models may have underrepresented areas that are indeed suitable but difficult to access, favoring occurrences in easily accessible areas. Additionally, species can disperse beyond their theoretical habitat suitability due to factors such as competition or human-mediated movement (Broennimann et al., 2012; Wisz et al., 2013). This means that even if an area is not predicted as suitable by the model, species might still be present due to their ability to move and adapt to different conditions. Similarly, variables not accounted for in the model could influence the presence of species in areas of low suitability (He et al., 2015; Kass et al., 2021). Environmental factors such as microclimate variations or specific interspecies interactions might lead to inaccuracies in predicting suitable habitats.
Even with this increase in area, S. bicolor still has one of the lowest EOOs of any Neotropical primate, which makes it particularly susceptible to anthropogenic threats such as deforestation and global warming, as well as ecological pressures like interspecific competition and stochastic events (Gaston and Spicer, 2001). Furthermore, it is concerning that only a small part (29.7%) of EOO of S. bicolor has high or moderate habitat suitability, of which only 35.2% (873 km2) is more suitable for S. bicolor than S. midas.
We did not record groups of S. bicolor on the east bank of the Urubu River, corroborating the findings of Subirá (1998) and Röhe (2006). This, however, contradicts the inferred presence of S. bicolor between the Urubu and Uatumã rivers by Ayres et al. (1982), who, however, did not report how occurance records were collected (i.e. direct observations by the author or citizen reports). In this area, the squirrel monkey (Saimiri sp.) is commonly called ‘suim,’ which is also the common name for S. bicolor. Therefore, it is likely that the name ‘suim’ may have been incorrectly recorded as S. bicolor by Ayres et al. (1982). Given this and that no other researcher besides Ayres et al. (1982) reported or registered S. bicolor between the Urubu and Uatumã rivers, we considered the records of S. bicolor in this interfluve as doubtful.
We recorded 19 groups of S. midas and two mixed groups in the areas indicated as highly suitable for S. midas within the EOO of S. bicolor. The areas of moderate and high suitability together have a total of 23 records of S. midas (Figure 5). The fact that S. midas is already occupying part of the EOO of S. bicolor associated with the fact that the areas of the EOO of S. bicolor previously occupied by S. bicolor (Ayres et al., 1982; Subirá, 1998; Röhe, 2006) are now occupied by S. midas demonstrates that the invasion process is underway. These areas are located inside the northern portion of the EOO of S. bicolor and are areas relatively anthropized, intersected by the federal BR 174 road and associated land use change. The invasion process could also be taking place in primary forest areas, but detecting this is more difficult. Peaceful associations between the species were recorded in two mixed groups recorded in this study, as Röhe (2006) also reported (four cases), but in contrast to Subirá (1998). This brings a new perspective regarding the skittish interaction between the two species as a conditioning factor for the observed spatial segregation. The latter author suggests that interactions are aggressive, where S. midas invariably attacks S. bicolor, being the competitively dominant species, which was not observed in this study.
The right (western) bank of the Urubu River is the current eastern limit of distribution of S. bicolor. However, we also registered nine occurrences of S. midas on the west bank of the Urubu River in areas of high and moderate suitability for S. midas. This is an observation that deserves attention, since the southern portion of the Urubu River is an Amazonian “várzea” area, considered a geographic barrier to westward movement of S. midas. At the other extreme of the distribution of S. bicolor, we also registered several occurrences of S. midas. These were on the left (eastern) bank of the Cuieiras River in areas of both high and moderate habitat suitability for the species and within the EOO of S. bicolor. Therefore, while S. midas is present on both banks of the Cuieiras and Urubu rivers, they appear to act as barriers to the movement of S. bicolor and delineate its EOO.
Contrary to our expectation, we did not identify extensive areas of coexistence between the species. The few areas of coexistence we identified in this study are just outside the northern city limit of Manaus (Figure 4). We registered 37 occurrences of S. bicolor and 15 occurrences of S. midas within the area of potential coexistence. We documented five occurrences of both species groups co-occurring within the potential coexistence area, two of which were reported by Röhe (2006) and three observed in this study. This area is intersected in the north-south direction by the federal BR-174 road, which serves as a conduit for human occupation and deforestation. Ayres et al. (1982) suggested that anthropogenic changes in the landscape could be favoring the occupation of these areas by S. midas. However, we cannot exclude the possibility that the documentation of these occurrences is caused by a greater sampling effort, since these are areas that are routinely inspected. We cannot rule out the possibility that the same is occurring in continuous forest areas, where this type of observation is more difficult to detect. These records reinforce the ecological significance of our models by indicating that the species are indeed sharing the areas designated as potential zones for coexistence. However, it is worth noting that the models did not predict areas of potential coexistence in the northeast portion of the contact area, underscoring the need for more sampling data in the region to enhance the accuracy of our models.
As expected, due to their recent evolutionary divergence (Brcko et al., 2022; Lopes et al., 2023), we found high niche similarity between the two species explained. Despite substantial niche overlap, S. midas was observed in more diverse environments, and its occurrences were registered in habitats with greater amplitude of soil quality and canopy height. Saguinus bicolor were less tolerant to variation in soil nutrient concentration and in canopy height, but were observed in areas with greater human impact. Given the ecological and biological traits of S. bicolor, we can hypothesize several factors that may favor the species in human-impacted areas. As a species with a wide range of habitat use (Gordo et al., 2013), S. bicolor may be capable of exploiting new resources and tolerate or even thrive in human-altered environments. Habitat fragmentation can create edge habitats that some species exploit more effectively than continuous forests, providing unique resources or conditions they are adapted to use (Ries et al., 2004). Additionally, the species is often observed moving through human-impacted areas, which may serve as corridors or stepping stones between more conserved habitats, even if they are not ideal permanent habitats (Gordo et al., 2013). Furthermore, human-altered environments can sometimes reduce competition from other species, including potential predators (Ries et al., 2004). In conserved areas, more specialized and dominant species might outcompete generalists like S. bicolor, which can then find niches in human-impacted areas. Sobroza et al. (2021c) also found that S. midas expanded its vertical niche when in sympatry with S. bicolor relative to areas of allopatry, suggesting some level of ecological adjustment in response to shared habitats. These results reinforce the idea of S. midas being a more generalist species in contrast to S. bicolor.
4.1 Implications for conservation of S. bicolor
Assessing the potential of a species to expand its range into the range of another endangered species is crucial for science-based conservation and management. This is especially critical when the endangered species’ distribution comprises urban areas and the demographic expansion of the expanding species is driven by anthropogenic factors (Pacifici et al., 2020). Saguinus bicolor is one of the most threatened primate species in the Amazon (Schwitzer et al., 2019; Gordo et al., 2021), mainly due to threats related to human activities, such as deforestation and habitat fragmentation, in particular, the rapid expansion of the city of Manaus. Unfortunately, the entire area of urban and agricultural expansion in Manaus, facilitated by the expansion of the road network, has historically occurred in areas of occurrence and with greater habitat suitability for S. bicolor than for S. midas, and these trends are unlikely to change. Recent studies (Soares-Filho et al., 2005; Fearnside and de Alencastro Graça, 2009) indicate that these areas will continue to suffer intense anthropogenic pressure, which will result in further landscape changes and additional loss of habitat for S. bicolor. Additionally, the occupation of the small EOO of S. bicolor by S. midas, already pointed out in previous studies (Ayres et al., 1982; Röhe, 2006) and, as the present study indicates, with potential to increase, can also be considered a serious threat for S. bicolor. In view of this, conservation actions must be taken urgently, ensuring the maintenance and quality of the species’ habitat and natural ecological processes. Controlling deforestation becomes the most immediate measure to be considered. Within the 8,354 km2 EOO of S. bicolor only 873 km2 are of high and moderate suitability for S. bicolor and of low suitability for S. midas. However, excluding the urbanized areas of Manaus and the area destined for urban expansion by the municipal growth plan, only 375 km2 of areas that combine suitable habitats, low human pressure and low probability of invasion by S. midas remain. While relatively small, these strategic areas are located in the rural area of Manaus and represent strongholds that could greatly favor the long-term survival of S. bicolor. Therefore, the implementation of measures to prioritize these areas for conservation is essential.
While the prospects of long-term survival of S. bicolor are precarious, they are possible. Long-term survival can be achieved through a multi-pronged approach that involves all stakeholders, is proactive and long-term. We view as minimally necessary:
1) Creation of protected areas aimed at conserving the main habitat of S. bicolor and fostering the establishment of ecological corridors.
2) Connecting fragments of areas with high and moderate suitability for the species and promoting safety for animals moving between the fragments. Prioritizing connectivity efforts in rural areas north of Manaus and along roadways is essential to maintaining habitat connectivity for these species.
3) Developing strategies that ensure that public and mainly private areas have their use effectively regulated, keeping their federally legislated legal reserves and permanent protected areas connected and forming ecological corridors.
4) Developing strategies for owners of private areas to participate in conservation actions.
5) Monitor and prevent deforestation and the opening of illegal roads and its branches in priority areas for the conservation of the species.
6) Implementing an awareness campaign for the general public, and an education campaign in public and private schools.
Currently, the National Action Plan for the conservation of Saguinus bicolor is in progress, implementing several of these essential actions (Jerusalinsky et al., 2017). This strategic plan, started in 2011, is coordinated by the Instituto Chico Mendes for Biodiversity Conservation (ICMBio) and follows the guidelines established by the International Union for Conservation of Nature (IUCN). It has a general objective of “promoting the conservation of Saguinus bicolor and its habitat, implementing actions to revert the current trend of population decline of the species’’. Through studies followed by a proposal from the Pied Tamarin National Plan articulators, for example, the municipal government of Manaus was able to create a protected area of 1,010 hectares in 2018, strategically located in an urban area crucial for maintaining local populations of S. bicolor.
In June 2024, the Brazilian government created an important integral protected area for the species. The Sauim-de-coleira Wildlife Refuge, encompassing 15,300 hectares within the Rio Preto da Eva – Rio Urubu interfluve, is considered strategic for maintaining the connectivity of the S. bicolor habitat. This protected area was planned based on studies coordinated by the Pied Tamarin National Plan, which initially, through a Population Viability Analysis (PVA), established that a minimum viable population for S. bicolor consists of 500 individuals in an area of at least 10,000 hectares (Campos et al., 2017). Following this, habitat connectivity analyzes indicated some target areas, one of which was chosen and converted into a protected area. It is now necessary to implement adequate management of the area, which includes actions that guarantee the protection of the species with the needs of the community members who live in and use the area.
In addition to the progress made in creating protected areas, it is worth highlighting the role of the action plan in structuring the captive program for the species. In 2016, there were only 24 individuals of S. bicolor being kept in 5 Brazilian institutions, most of which had difficulty in properly managing these animals. After a series of agreements and training, in 2024 we reached 75 individuals in 13 institutions, with the prospect of increasing the number of partners, including foreign institutions. The action plan also contributed to improving licensing processes in areas where S. bicolor occurs by providing licensing agencies with essential technical information. This includes identifying priority areas for the species’ conservation and proposing mitigation and compensation measures for locations where interventions and human impacts are necessary.
The researchers involved in the action plan emphasize the critical importance of improving habitat quality and connectivity for the species, mitigating human-induced mortality, upholding and restoring legal reserves and permanent protected areas, and implementing environmental education initiatives as key priorities for conserving this iconic Amazonian species. The findings of this study can support policy decisions on land use, prioritize areas for monitoring the advancement of S. midas into regions currently occupied by S. bicolor, and identify recommended areas for establishing new protected zones. In this way, we can prevent further population declines of one of the most threatened primate species in the entire Amazon.
Data availability statement
The raw data supporting the conclusions of this article and the original contributions presented in the study are publicly available. These data can be found here: https://github.com/legalLab/publications/tree/master/Lagroteria_et_al_2024.
Ethics statement
The study was authorized by the ICMBio Biodiversity Authorization and Information System, SISBIO no. 74022/2019. Ethical approval was not required for the study involving animals in accordance with the local legislation and institutional requirements.
Author contributions
DL: Conceptualization, Data curation, Formal analysis, Funding acquisition, Investigation, Methodology, Project administration, Resources, Software, Supervision, Validation, Visualization, Writing – original draft, Writing – review & editing. TC: Conceptualization, Data curation, Formal analysis, Methodology, Software, Supervision, Validation, Visualization, Writing – review & editing. GZ: Formal analysis, Methodology, Software, Validation, Visualization, Writing – review & editing. FR: Conceptualization, Data curation, Investigation, Methodology, Writing – review & editing. AM: Data curation, Investigation, Visualization, Writing – review & editing. TH: Conceptualization, Investigation, Methodology, Software, Supervision, Validation, Visualization, Writing – review & editing. MG: Conceptualization, Data curation, Formal analysis, Funding acquisition, Investigation, Methodology, Project administration, Resources, Supervision, Visualization, Writing – review & editing.
Funding
The author(s) declare financial support was received for the research, authorship, and/or publication of this article. The author declares financial support was received for the development of this research. This project was financed in part by the Coordenação de Aperfeiçoamento de Pessoal de Nível Superior - Brazil (CAPES) - PROEX n. 0742/2020, and by the Fundação de Amparo à Pesquisa do Estado do Amazonas “FAPEAM Universal Amazonas” call for proposals, grant no. 006/2019, and by CLAMBIO, consortium funded through the BiodivERsA 2019-2020 Joint COFUND Call on “Biodiversity and Climate Change” (Academy of Finland grant no. 344733 to HT).
Acknowledgments
We are grateful to all field assistants and other collaborators who helped obtain the occurrence records, as well as to the owners of private properties for facilitating fieldwork. We also extend our gratitude to the Chico Mendes Institute for Biodiversity Conservation (ICMBio), the Projeto Sauim-de-Coleira staff, Amazonas Federal University, the National Institute for Research in the Amazon (INPA), and the National Center for Research and Conservation of Amazonian Biodiversity (CEPAM). We would like to thank Edson Rodrigues Costa, André Nóbrega and Diogo Ribeiro for their assistance in field activities and image production. We would like to thank Tiergarten Kleve Zoo, Shaldon Wildlife Trust, and The Tamarin Trust for supporting field activities. We also thank the two reviewers for their valuable comments that improved our work.
Conflict of interest
The authors declare that the research was conducted in the absence of any commercial or financial relationships that could be construed as a potential conflict of interest.
Publisher’s note
All claims expressed in this article are solely those of the authors and do not necessarily represent those of their affiliated organizations, or those of the publisher, the editors and the reviewers. Any product that may be evaluated in this article, or claim that may be made by its manufacturer, is not guaranteed or endorsed by the publisher.
Supplementary material
The Supplementary Material for this article can be found online at: https://www.frontiersin.org/articles/10.3389/fcosc.2024.1426488/full#supplementary-material
References
Acevedo P., Cassinello J., Hortal J., Gortázar C. (2007). Invasive exotic aoudad (Ammotragus lervia) as a major threat to native Iberian ibex (Capra pyrenaica): A habitat suitability model approach. Diversity Distributions 13, 587–597. doi: 10.1111/j.1472-4642.2007.00374.x
Aiello-Lammens M. E., Boria R. A., Radosavljevic A., Vilela B., Anderson R. P. (2015). spThin: an R package for spatial thinning of species occurrence records for use in ecological niche models. Ecography 38, 541–545. doi: 10.1111/ecog.01132
Aleixo I., Norris D., Hemerik L., Barbosa A., Prata E., Costa F., et al. (2019). Amazonian rainforest tree mortality driven by climate and functional traits. Nat. Clim. Change 9, 384–388. doi: 10.1038/s41558-019-0458-0
Arias-Gonzalez C., González-Maya J. F., Gonzalez Zamorano P., Ortega Rubio A. (2021). Climate refugia for two Colombian endemic tamarin primates are critically under-protected. Mamm. Biol. 101, 531–543. doi: 10.1007/s42991-021-00151-0
Ashraf U., Peterson A. T., Chaudhry M. N., Ashraf I., Saqib Z., Rashid Ahmad S., et al. (2017). Ecological niche model comparison under different climate scenarios: a case study of Olea spp. in Asia. Ecosphere 8, e01825. doi: 10.1002/ecs2.1825
Ayres J. M., Mittermeier R. A., Constable I. D. (1982). Brazilian tamarins on the way to extinction? Oryx 16, 329–333. doi: 10.1017/S0030605300017786
Bradley A. V., Gerard F. F., Barbier N., Weedon G. P., Anderson L. O., Huntingford C., et al. (2011). Relationships between phenology, radiation and precipitation in the Amazon region. Global Change Biol. 17, 2245–2260. doi: 10.1111/gcb.2011.17.issue-6
Braz A. G., Lorini M. L., Vale M. M. (2019). Climate change is likely to affect the distribution but not parapatry of the Brazilian marmoset monkeys (Callithrix spp.). Diversity Distributions 25, 536–550. doi: 10.1111/ddi.12872
Brcko I. C., Carneiro J., Ruiz-García M., Boubli J. P., e Silva-Júnior J. D. S., Farias I., et al. (2022). Phylogenetics and an updated taxonomic status of the Tamarins (CallitriChinae, Cebidae). Mol. Phylogenet. Evol. 173, 107504. doi: 10.1016/j.ympev.2022.107504
Broennimann O., Fitzpatrick M. C., Pearman P. B., Petitpierre B., Pellissier L., Yoccoz N. G., et al. (2012). Measuring ecological niche overlap from occurrence and spatial environmental data. – Global Ecol. Biogeogr. 21, 481–497. doi: 10.1111/j.1466-8238.2011.00698.x
Brus D., Kempen B., Heuvelink G. (2011). Sampling for validation of digital soil maps. Eur. J. Soil Sci. 62, 394–407. doi: 10.1111/j.1365-2389.2011.01364.x
Campos I. B., Gordo M., Vidal ,. M. D. (2017). “Análise de viabilidade populacional (AVP) de Saguinus bicolor,” in Plano de ação nacional para a conservaçáo do sauim-de-coleira. Eds. Jerusalinsky L., de Azevedo R. B., Gordo M. (Brasília, DF: Instituto Chico Mendes de Conservação da Biodiversidade), 45–58.
Cárdenas Ramírez G. G., Jones M. M., Heymann E. W., Tuomisto H. (2021). Characterizing primate home-ranges in Amazonia: Using ferns and lycophytes as indicators of site quality. Biotropica 00, 1–11. doi: 10.1111/btp.12935
Cavalcante T., Jesus A. S. J., Rabelo R., Messias M. R., Valsecchi J., Ferraz D., et al. (2020). Niche overlap between two sympatric frugivorous Neotropical primates: improving ecological niche models using closely-related taxa. Biodiversity Conserv. 2020, 1–15. doi: 10.1007/s10531-020-01997-5
Chaves P. P., Ruokolainen K., Tuomisto H. (2022). Impact of spatial configuration of training data on the performance of Amazonian tree species distribution models. For. Ecol. Manage. 504, 119838. doi: 10.1016/j.foreco.2021.119838
Chesson P. (2000). Mechanisms of maintenance of species diversity. Annu. Rev. Ecol. Syst. 31, 343–366. doi: 10.1146/annurev.ecolsys.31.1.343
Clout M. N., Russell J. C. (2008). The invasion ecology of mammals: a global perspective. Wildlife Res. 35, 180–184. doi: 10.1071/WR07091
Dawson G. A. (1979). The use of time and space by the Panamanian tamarin, Saguinus oedipus. Folia Primatologica 31, 253–284. doi: 10.1159/000155891
de Oliveira J. B. (2014). Filogeografia e Demografia Histórica de Saguinus bicolor. Master Degree, Instituto Nacional de Pesquisas da Amazônia, Manaus, AM.
Di Cola V., Broennimann O., Petitpierre B., Breiner F. T., D'Amen M., Randin C., et al. (2017). ecospat: an R package to support spatial analyses and modeling of species niches and distributions. Ecography 40, 774–787. doi: 10.1111/ecog.02671
Didan K. (2015). MOD13A2 MODIS/Terra Vegetation Indices 16-Day L3 Global 1km SIN Grid V006 (NASA EOSDIS Land Processes DAAC) (Accessed 2021-07-29).
Drake J. M., Randin C., Guisan A. (2006). Modelling ecological niches with support vector machines. J. Appl. Ecol. 43, 424–432. doi: 10.1111/j.1365-2664.2006.01141.x
Duan R. Y., Kong X. Q., Huang M. Y., Fan W. Y., Wang Z. G. (2014). The predictive performance and stability of six species distribution models. PloS One 9, e112764. doi: 10.1371/journal.pone.0112764
Duncan R. P., Blackburn T. M., Sol D. (2003). The ecology of bird introductions. Annu. Rev. Ecol. Evolution Systematics 34, 71–98. doi: 10.1146/annurev.ecolsys.34.011802.132353
Duran A., Meyer A. L. S., Pie M. R. (2013). Climatic niche evolution in new world monkeys (Platyrrhini). PloS One 8, 8–13. doi: 10.1371/journal.pone.0083684
Egler S. G. (1992). Feeding ecology of Saguinus bicolor bicolor (Callitrichidae: Primates) in a relict forest in Manaus, Brazilian Amazonia. Folia Primatologica 59, 61–76. doi: 10.1159/000156644
Excoffier L., Foll M., Petit R. J. (2009). Genetic consequences of range expansions. Annu. Rev. Ecol. Evolution Systematics 40, 481–501. doi: 10.1146/annurev.ecolsys.39.110707.173414
Farias I. P., Santos W. G., Gordo M., Hrbek T. (2015). Effects of forest fragmentation on genetic diversity of the Critically Endangered primate, the pied tamarin (Saguinus bicolor): Implications for conservation. J. Heredity 106, 512–521. doi: 10.1093/jhered/esv048
Fearnside P. M., de Alencastro Graça P. M. L. (2009). BR-319: A rodovia Manaus-Porto Velho e o impacto potencial de conectar o arco de desmatamento à Amazônia central. Novos cadernos NAEA 12, 19–50. doi: 10.5801/ncn.v12i1.241
Ferrari S. F. (1993). “Ecological differentiation in the Callitrichidae,” in Marmosets and Tamarins, systematics, behaviour, and ecology. Ed. Rylands A. B. (Oxford: Oxford University Press), 314–328. doi: 10.1093/oso/9780198540229.003.0016
Fick S. E., Hijmans R. J. (2017). WorldClim 2: new 1km spatial resolution climate surfaces for global land areas. Int. J. Climatol. 37, 4302–4315. doi: 10.1002/joc.5086
Fitzgerald M., Coulson R., Lawing A. M., Matsuzawa T., Koops K. (2018). Modeling habitat suitability for chimpanzees (Pan troglodytes verus) in the Greater Nimba Landscape, Guinea, West Africa. Primates 59, 361–375. doi: 10.1007/s10329-018-0657-8
Gallardo B., Zieritz A., Aldridge D. C. (2015). The importance of the human footprint in shaping the global distribution of terrestrial, freshwater and marine invaders. PloS One 10, e0125801. doi: 10.1371/journal.pone.0125801
Ganzhorn J. U. (1999). “Body mass, competition and the structure of primate communities,” in Primate communities. Eds. Fleagle J. G., Janson C. H., Reed K. E. (Cambridge University Press, Cambridge, UK), 141–157.
Gaston K. J., Spicer J. I. (2001). The relationship between range size and niche breadth: a test using five species of Gammarus (Amphipoda). Glob. Ecol. Biogeogr. 10, 179–188. doi: 10.1046/j.1466-822x.2001.00225.x
Gavilanez M. M., Stevens R. D. (2013). Role of environmental, historical and spatial processes in the structure of Neotropical primate communities: contrasting taxonomic and phylogenetic perspectives. Global Ecol. Biogeogr. 22, 607–619. doi: 10.1111/geb.12011
Giovanelli J. G., de Siqueira M. F., Haddad C. F., Alexandrino J. (2010). Modeling a spatially restricted distribution in the Neotropics: How the size of calibration area affects the performance of five presence-only methods. Ecol. Model. 221, 215–224. doi: 10.1016/j.ecolmodel.2009.10.009
Gordo M. (2012). Ecologia e conservação do sauim-de-coleira, Saguinus bicolor (Primates; Callitrichidae). PhD thesis. Museu Paraense Emílio Goeldi, Belém do Pará/PA.
Gordo M., Calleia F. O., Vasconcelos S. A., Leite J. J., Ferrari S. F. (2013). “The challenges of survival in a concrete jungle: conservation of the pied tamarin (Saguinus bicolor) in the urban landscape of Manaus, Brazil,” in Primates in Fragments. Eds. Marsh L. K., Chapman C. (Springer, New York), 357–370. 532p.
Gordo M., Rodrigues L. F., Vidal M. D., Spironello W. R. (2008). “Primatas,” in Reserva Ducke – a Biodiversidade amazônica através de uma grade. Eds. Oliveira M., Baccaro F. B., Braga-Neto R., Magnusson W. E. (Manaus, AM: Áttema design editorial), 39–49.
Gordo M., Röhe F., Vidal M. D., Subirá R., Boubli J. P., Mittermeier R. A., et al. (2021). Saguinus bicolor (amended version of 2019 assessment) (The IUCN Red List of Threatened Species). Available at: https://www.iucnredlist.org/species/40644/192551696. (Accessed 26 February 2022).
Gouveia S. F., Villalobos F., Dobrovolski R., Beltrão-Mendes R., Ferrari S. F. (2014). Forest structure drives global diversity of primates. J. Anim. Ecol. 83, 1523–1530. doi: 10.1111/1365-2656.12241
Guisan A., Wilfried T., Niklaus E. Z. (2017). Habitat suitability and distribution models: with applications in R (Cambridge: Cambridge University Press (Ecology, Biodiversity and Conservation)). doi: 10.1017/9781139028271
Guo Q., Kelly M., Graham C. H. (2005). Support vector machines for predicting distribution of Sudden Oak Death in California. Ecol. Model. 182, 75–90. doi: 10.1016/j.ecolmodel.2004.07.012
Gurevitch J., Fox G. A., Wardle G. M., Taub D. (2011). Emergent insights from the synthesis of conceptual frameworks for biological invasions. Ecol. Lett. 14, 407–418. doi: 10.1111/ele.2011.14.issue-4
Gutiérrez E. E., Boria R. A., Anderson R. P. (2014). Can biotic interactions cause allopatry? Niche models, competition, and distributions of South American mouse opossums. Ecography 37, 741–753. doi: 10.1111/ecog.00620
Harvey P. H., Martin R. D., Clutton-Brock T. H. (1987). “Life histories in comparative perspective,” in Primate Societies. Eds. Smuts B. B., Cheyney D. L., Seyfarth R. M., Wrangham R. W., Struhsaker T. T. (Chicago: University of Chicago Press), 181–196.
He K. S., Bradley B. A., Cord A. F., Rocchini D., Tuanmu M. N., Schmidtlein S., et al. (2015). Will remote sensing shape the next generation of species distribution models? Remote Sens. Ecol. Conserv. 1, 4–18. doi: 10.1002/rse2.7
Hernández-Zul M. I., Quijano-Carranza J. A., Yañez-López R., Ocampo-Velazquez R. V., Torres-Pacheco I., Guevara-Gonzalez R. G., et al. (2013). Dynamic simulation model of Central American Locust Schistocerca piceifrons (Orthoptera: Acrididae). Florida Entomologist 96, 1274–1283. doi: 10.1653/024.096.0405
Hershkovitz P. (1977). Living new world monkeys (Platyrrhini) (Chicago: University of Chicago Press), v. 1.
Higgins M. A., Ruokolainen K., Tuomisto H., Llerena N., Cardenas G., Phillips O. L., et al. (2011). Geological control of floristic composition in Amazonian forests. J. Biogeogr. 38, 2136–2149. doi: 10.1111/j.1365-2699.2011.02585.x
Hijmans R. J., Van Etten J., Cheng J., Mattiuzzi M., Sumner M., Greenberg J. A., et al. (2018). Raster: geographic data analysis and modeling (R package version), v. 2.
IUCN (2019). Guidelines for Using the IUCN Red List Categories and Criteria, Version 14 (IUCN Standards and Petitions Subcommittee). Available at: http://www.iucnredlist.org/documents/RedListGuidelines.pdf.
Jerusalinsky L., Azevedo R. B., Gordo M. (2017). Plano de ação nacional para a conservação do sauim-de-coleira (Brasília: Instituto Chico Mendes de Conservação da Biodiversidade, Icmbio), 207.
Johns A. D., Skorupa J. P. (1987). Responses of rain-forest primates to habitat disturbance: a review. Int. J. primatol. 8, 157–191. doi: 10.1007/BF02735162
Kamilar J. M., Ledogar J. A. (2011). Species co-occurrence patterns and dietary resource competition in primates. Am. J. Phys. Anthropol. 144, 131–139. doi: 10.1002/ajpa.21380
Kass J. M., Meenan S. I., Tinoco N., Burneo S. F., Anderson R. P. (2021). Improving area of occupancy estimates for parapatric species using distribution models and support vector machines. Ecol. Appl. 31, e02228. doi: 10.1002/eap.2228
Kessler P. (1998). Primate densities in the natural reserve of Nouragues, French Guiana. Neotropical Primates 6, 45–46. doi: 10.62015/np.1998.v6.396
Kistner-Thomas E., Kumar S., Jech L., Woller D. A. (2021). Modeling rangeland grasshopper (Orthoptera: acrididae) population density using a landscape-level predictive mapping approach. J. Economic Entomol. 114 (4), 1557–1567. doi: 10.1093/jee/toab119
Lehman S. M. (2000). Primate community structure in Guyana: a biogeographic analysis. Int. J. Primatol. 21, 333–351. doi: 10.1023/A:1005454317333
Liu C., Newell G., White M. (2019). The effect of sample size on the accuracy of species distribution models: considering both presences and pseudo-absences or background sites. Ecography 42, 535–548. doi: 10.1111/ecog.03188
Lopes G. P., Rohe F., Bertuol F., Polo É., Valsecchi J., Santos T. C. M., et al. (2023). Molecular systematics of tamarins with emphasis on genus Tamarinus (Primates, Callitrichidae). Zoologica Scripta 52, 556–570. doi: 10.1111/zsc.12617
Lowry E., Rollinson E. J., Laybourn A. J., Scott T. E., Aiello-Lammens M. E., Gray S. M., et al. (2013). Biological invasions: a field synopsis, systematic review, and database of the literature. Ecol. Evol. 3, 182–196. doi: 10.1002/ece3.431
Machado A. F., Nunes M. S., Silva C. R., Dos Santos M. A., Farias I. P., da Silva M. N. F., et al. (2019). Integrating phylogeography and ecological niche modeling to test diversification hypotheses using a Neotropical rodent. Evolutionary Ecol. 33, 111–148. doi: 10.1007/s10682-019-09968-1
Master Plan for Manaus (2021). Municipal institute of urban planning (IMPLURB). Available online at: https://www2.manaus.am.gov.br/docs/portal/secretarias/implurb/PLANO%20DIRETOR%20-%20LIVRO%20DIGITAL/LEGISLA%c3%87%c3%83O%20URBAN%c3%8dSTICA%20MUNICIPAL%20-%20PLANO%20DIRETOR%20E%20AMBIENTAL%20DE%20MANAUS%20E%20SUAS%20LEIS%20COMPLEMENTARES%20-%20Vers%c3%a3o%2001.pdf (Accessed July 26, 2022).
McLean K. A., Trainor A. M., Asner G. P., Crofoot M. C., Hopkins M. E., Campbell C. J., et al. (2016). Movement patterns of three arboreal primates in a Neotropical moist forest explained by LiDAR-estimated canopy structure. Landscape Ecol. 31, 1849–1862. doi: 10.1007/s10980-016-0367-9
Mittermeier R. A., Urbani B., Rylands A. B., Régis T. (2021). Saguinus midas (amended version of 2018 assessment) Vol. 2021 (The IUCN Red List of Threatened Species), e.T41525A192552538. doi: 10.2305/IUCN.UK.2021-1.RLTS.T41525A192552538.en
Naimi B., Hamm N. A., Groen T. A., Skidmore A. K., Toxopeus A. G. (2014). Where is positional uncertainty a problem for species distribution modelling? Ecography 37, 191–203. doi: 10.1111/j.1600-0587.2013.00205.x
Ochoa-Quintero J., Chang C., Gardner T., Rezende-Messias M., Sutherland W., Delben F. (2017). Habitat loss on Rondon’s marmoset potential distribution. Land 6, 8. doi: 10.3390/land6010008
Pacifici M., Rondinini C., Rhodes J. R., Burbidge A. A., Cristiano A., Watson J. E. M., et al. (2020). Global correlates of range contractions and expansions in terrestrial mammals. Nat. Commun. 11, 2840. doi: 10.1038/s41467-020-16684
Pack K. S., Henry O., Sabatier D. (1999). The Insectivorous-Frugivorous Diet of the Golden-Handed Tamarin (Saguinus midas midas) in French Guiana. Folia Primatol. 70, 1–7. doi: 10.1159/000021668
Palacios E., Rodríguez A., Castillo C. (2004). Preliminary observations on the mottledface tamarin (Saguinus inustus) on the lower río caquetá, Colombian amazonia. Neotropical Primates 12, 123–126. doi: 10.1896/1413-4705.12.3.123
Palminteri S., Powell G. V. N., Asner G. P., Peres C. A. (2012). LiDAR measurements of canopy structure predict spatial distribution of a tropical mature forest primate. Remote Sens. Environ. 127, 98–105. doi: 10.1016/j.rse.2012.08.014
Peel M. C., Finlayson B. L., McMahon T. A. (2007). Updated world map of the Köppen-Geiger climate classification. Hydrol. Earth system Sci. 11, 1633–1644. doi: 10.5194/hess-11-1633-2007
Peres C. (1993). Structure and spatial organization of an Amazonian terra firme forest primate community. J. Trop. Ecol. 9, 259–276. doi: 10.1017/S026646740000729X
Peres C. A. (1994). Primate responses to phenological changes in an Amazonian terra firme forest. Biotropica 26, 98–112. doi: 10.2307/2389114
Peterson A., Soberon J., Sanchez-Cordero V. (1999). Conservatism of ecological niches in evolutionary time. Science 285, 1265–1267. doi: 10.1126/science.285.5431.1265
Phillips S. J., Anderson R. P., Schapire R. E. (2006). Maximum entropy modeling of species geographic distributions. Ecol. Model. 190, 231–259. doi: 10.1016/j.ecolmodel.2005.03.026
Phillips S. J., Anderson R. P., Dudík M., Schapire R. E., Blair M. E. (2017). Opening the black box: an open-source release of Maxent. Ecography 40, 887–893. doi: 10.1111/ecog.03049
Phillips O. L., Vargas P. N., Monteagudo A. L., Cruz A. P., Zans M.-E. C., Sánchez W. G., et al. (2003). Habitat association among Amazonian tree species: a landscape-scale approach. J. Ecol. 91, 757–775. doi: 10.1046/j.1365-2745.2003.00815.x
Pitman N. C. A., Mogollón H., Dávila N., Ríos M., García-Villacorta R., Guevara J., et al. (2008). Tree community change across 700 km of lowland Amazonian forest from the Andean foothills to Brazil. Biotropica 40, 525–535. doi: 10.1111/j.1744-7429.2008.00424.x
Ponzoni F. J., e Shimabukuro Y. E. (2007). Sensoriamento remoto no estudo da vegetação (São José dos Campos, SP: Editora Parêntese), 135.
Pyritz L. W., Büntge A. B. S., Herzog S. K., Kessler M. (2010). Effects of habitat structure and fragmentation on diversity and abundance of primates in tropical deciduous forests in Bolivia. Int. J. Primatol. 31, 796–812. doi: 10.1007/s10764-010-9429-z
QGIS Development Team (2024). QGIS Geographic Information System (Open Source Geospatial Foundation Project). Available at: http://qgis.osgeo.org.
Rabelo R. M., Goncalves J. R., Silva F. E., Rocha D. G., Canale G. R., Bernardo C. S. S., et al. (2020). Predicted distribution and habitat loss for the Endangered black-faced black spider monkey (Ateles chamek) in the Amazon. Oryx 54.5, 699–705. doi: 10.1017/S0030605318000522
R Core Team (2021). R: a language and environment for statistical computing (Vienna: R Foundation for Statistical Computing).
Rehg J. A. (2006). Seasonal variation in polyspecific associations among Callimico goeldii, Saguinus labiatus, and S. fuscicollis in Acre, Brazil. Int. J. Primatol. 27, 1399. doi: 10.1007/s10764-006-9076-6
Rezende G. C., Sobral-Souza T., Culot L. (2020). Integrating climate and landscape models to prioritize areas and conservation strategies for an endangered arboreal primate. Am. J. Primatol. 82 (12), e23202. doi: 10.1002/ajp.23202
Ribeiro R. C., Lemos W., De P., Poderoso J. C. M., Pikart T. G., Zanuncio J. C. (2013). New record of grasshopper (Orthoptera: Acrididae & Romaleidae) defoliators and population dynamics of insects on crops of Heliconia spp. in the Amazon. Florida Entomologist 96, 225–228. doi: 10.1653/024.096.0130
Ries L., Fletcher R. J. Jr., Battin J., Sisk T. D. (2004). Ecological responses to habitat edges: mechanisms, models, and variability explained. Annu. Rev. Ecol. Evol. Syst. 35, 491–522. doi: 10.1146/annurev.ecolsys.35.112202.130148
Rodrigues L. F., Vidal M. D. (2011). Densidade e tamanho populacional de primatas em uma área de terra firme na Amazônia Central. Neotropical Primates 18, 9–16. doi: 10.1896/044.018.0102
Röhe F. (2006). Área de contato entre as distribuições geográficas de Saguinus midas e Saguinus bicolor (Callitrichidae-Primates): a importância de interações e fatores ecológicos. Manaus, AM: Instituto Nacional de Pesquisas da Amazônia.
Rylands A. B., Keuroghlian A. (1989). Primate populations in continuous forest and forest fragments in central Amazonian. Acta Amazonica Manaus 18, 291–307. doi: 10.1590/1809-43921988183307
Scattolini M. C., Lira-Noriega A., Cigliano M. M. (2020). Species richness, range size, and wing development in South American melanopline grasshoppers (Orthoptera, Acrididae). Ecol. Entomol. 45, 840–853. doi: 10.1111/een.12859
Schoener T. W. (1968). The Anolis lizards of Bimini: Resource partitioning in a complex fauna. Ecology 49, 704–726. doi: 10.1111/j.1744-7429.2008.00486.x
Schreier B. M., Harcourt A. H., Coppeto S. A., Somi M. F. (2009). Interspecific competition and niche separation in primates: a global analysis. Biotropica 41, 283–291. doi: 10.1111/j.1744-7429.2008.00486.x
Schwitzer C., Mittermeier R. A., Rylands A. B., Chiozza F., Williamson E. A., Byler D., et al. (2019). Primates in Peril: The World’s 25 Most Endangered Primates 2018–2020 (Washington, DC: IUCN SSC Primate Specialist Group, International Primatological Society, Global Wildlife Conservation, and Bristol Zoological Society), 130.
Shea K., Chesson P. (2002). Community ecology theory as a framework for biological invasions. Trends Ecol. Evol. 17, 170–176. doi: 10.1016/S0169-5347(02)02495-3
Sillero N., Arenas-Castro S., Enriquez-Urzelai U., Vale C. G., Sousa-Guedes D., Martínez-Freiría F., et al. (2021). Want to model a species niche? A step-by-step guideline on correlative ecological niche modelling. Ecol. Model. 456, 109671. doi: 10.1016/j.ecolmodel.2021.109671
Sillero N., Barbosa A. M. (2020). Common mistakes in ecological niche models. Int. J. Geographical Inf. Sci. 35, 213–226. doi: 10.1080/13658816.2020.1798
Soares-Filho B. S., Nepstad D. C., Curran L., Cerqueira G. C., Garcia R. A., Ramos C. A., et al. (2005). Cenários de desmatamento para a Amazônia. Estudos Avançados 19, 137–152. doi: 10.1590/S0103-40142005000200008
Sobroza T. V., Gordo M., Barnett A. P. A., Boubli J. P., Spironello W. R. (2021a). Parapatric pied and red-handed tamarin responses to congeneric and conspecific calls. Acta Oecol. 10, 103688. doi: 10.1016/j.actao.2020.103688
Sobroza T. V., Gordo M., Pequeno P. A. C. L., Dunn J. C., Spironello W. R., Rabelo R. M., et al. (2021b). Convergent character displacement in sympatric tamarin calls (Saguinus spp.). Behav. Ecol. Sociobiol. 75, 88. doi: 10.1007/s00265-021-03028-x
Sobroza T. V., Pequeno P. A. C. L., Gordo M., Kinap N. M., Barnett A. P. A., Spironello W. R. (2021c). Does co-occurrence drive vertical niche partitioning in parapatric tamarins (Saguinus spp.)? Austral Ecol. 46, 1335–1342. doi: 10.1111/aec.13085
Subirá R. J. (1998). Avaliação da situação atual das populações selvagens do Sauim-de- coleira Saguinus b. bicolor (Spix 1823). University of Brasília Foundation, Brasília, DF.
Sussman R. W., Kinzey W. G. (1984). The ecological role of the Callitrichidae: a review. Am. J. Phys. Anthropol. 64, 419–449. doi: 10.1002/ajpa.1330640407
Svenning J. C., Gravel D., Holt R. D., Schurr F. M., Thuiller W., Münkemüller T., et al. (2014). The influence of interspecific interactions on species range expansion rates. Ecography 37, 1198–1209. doi: 10.1111/j.1600-0587.2013.00574.x
Tuomisto H., Moulatlet G. M., Balslev H., Emilio T., Figueiredo F. O. G., Pedersen D., et al. (2016). A compositional turnover zone of biogeographical magnitude within lowland Amazonia. J. Biogeogr. 43, 2400–2411. doi: 10.1111/jbi.12864
Valavi R., Guillera-Arroita G., Lahoz-Monfort J. J., Elith J. (2021). Predictive performance of presence-only species distribution models: a benchmark study with reproducible code. Ecol. Monogr. 00, e01486. doi: 10.1002/ecm.1486
Vallinoto M., Araripe J., do Rego P. S., Tagliaro C. H., Sampaio I., Schneider H. (2006). Tocantins River as an effective barrier to gene flow in Saguinus Niger populations. Genet. Mol. Biol. 29, 215–219. doi: 10.1590/S1415-47572006000200005
Vašát R., Pavlů L., Borůvka L., Drábek O., Nikodem A. (2013). Mapping the topsoil pH and humus quality of forest soils in the North Bohemian Jizerské Hory Mts. region with ordinary, universal, and regression kriging: Cross-validation comparison. Soil Water Res. 8, 97–104. doi: 10.17221/62/2012-SWR
Vidal M. D., Cintra R. (2006). Effects of forest structure components on the occurrence, groups and density of groups of bare-face tamarins (Saguinus bicolor - Primates: CallitriChinae) in central Amazonia. Acta Amazonica 36, 237–248. doi: 10.1590/S0044-59672006000200014
Warren D. L., Glor R. E., Turelli M. (2008). Environmental niche equivalency versus conservatism: Quantitative approaches to niche evolution. Evolution 62, 2868–2883. doi: 10.1111/evo.2008.62.issue-11
Webb C. O., Ackerly D. D., Mc Peek M. A., Donoghue M. J. (2002). Phylogenies and community ecology. Annu. Rev. Ecol. Systematics 33, 475–505. doi: 10.1146/annurev.ecolsys.33.010802.150448
Weber M. G., Strauss S. Y. (2016). Coexistence in close relatives: beyond competition and reproductive isolation in sister taxa. Annu. Rev. Ecol. Evolution Systematics 47, 359–381. doi: 10.1146/annurev-ecolsys-112414-054048
Wiederholt R., Post E. (2010). Tropical warming and the dynamics of endangered primates. Biol. Lett. 6, 256–260. doi: 10.1098/rsbl.2009.0710
Wildlife Conservation Society - WCS, and Center for International Earth Science Information Network - CIESIN - Columbia University (2005). Last of the Wild Project, Version 2, 2005 (LWP-2): Global Human Footprint Dataset (Geographic) (Palisades, NY: NASA Socioeconomic Data and Applications Center (SEDAC) (Accessed 04 August 2021).
Willems E. P., Barton R. A., Hill R. A. (2009). Remotely sensed productivity, regional home range selection, and local range use by an omnivorous primate. Behav. Ecol. 20, 985–992. doi: 10.1093/beheco/arp087
Wisz M. S., Pottier J., Kissling W. D., Pellissier L., Lenoir J., Damgaard C. F., et al. (2013). The role of biotic interactions in shaping distributions and realized assemblages of species: implications for species distribution modelling. Biol. Rev. 88, 15–30. doi: 10.1111/j.1469-185X.2012.00235.x
Zuquim G., Chaves P. P., Quesada C. A., Ruokolainen K., Tuomisto H. (2023). Introducing a map of soil base cation concentration, an ecologically relevant GIS-layer for Amazonian forests. Geoderma Regional 33, e00645. doi: 10.1016/j.geodrs.2023.e00645
Zuquim G., Stropp J., Moulatlet G. M., Van Doninck J., Quesada C. A., Figueiredo F. O., et al. (2019). Making the most of scarce data: mapping soil gradients in data-poor areas using species occurrence records. Methods Ecol. Evol. 10, 788–801. doi: 10.1111/2041-210X.13178
Keywords: range expansion, interspecific competition, Callitrichidae, pied tamarin, niche overlap, habitat suitability, coexistence, primate conservation
Citation: Lagroteria D, Cavalcante T, Zuquim G, Röhe F, Medeiros ASM, Hrbek T and Gordo M (2024) Assessing the invasive potential of Saguinus midas in the extent of occurrence of the critically endangered Saguinus bicolor. Front. Conserv. Sci. 5:1426488. doi: 10.3389/fcosc.2024.1426488
Received: 01 May 2024; Accepted: 01 July 2024;
Published: 05 September 2024.
Edited by:
Karen B. Strier, University of Wisconsin-Madison, United StatesReviewed by:
Stella De La Torre, Universidad San Francisco de Quito, EcuadorCarla Possamai, Muriqui Institute of Biodiversity, Brazil
Copyright © 2024 Lagroteria, Cavalcante, Zuquim, Röhe, Medeiros, Hrbek and Gordo. This is an open-access article distributed under the terms of the Creative Commons Attribution License (CC BY). The use, distribution or reproduction in other forums is permitted, provided the original author(s) and the copyright owner(s) are credited and that the original publication in this journal is cited, in accordance with accepted academic practice. No use, distribution or reproduction is permitted which does not comply with these terms.
*Correspondence: Diogo Lagroteria, ZGlvZ28uZmF1bmFAZ21haWwuY29t
†Present address: Gabriela Zuquim, CSC – IT Center for Science, Espoo, Finland