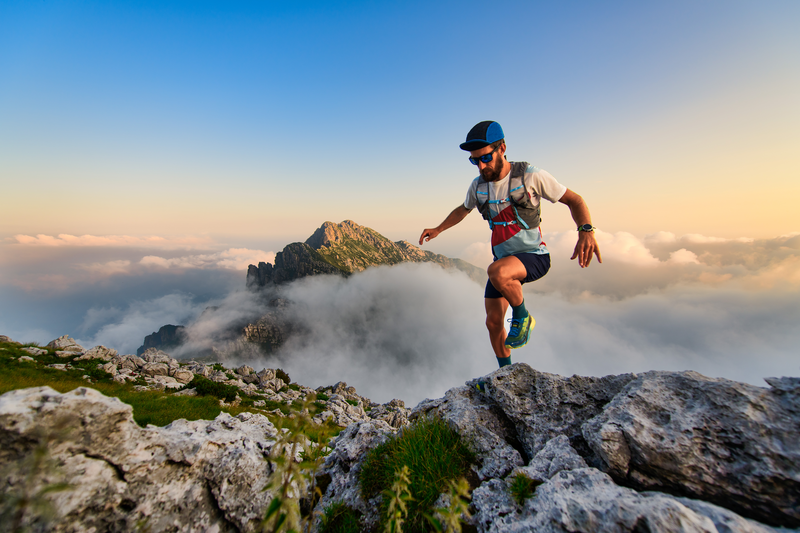
95% of researchers rate our articles as excellent or good
Learn more about the work of our research integrity team to safeguard the quality of each article we publish.
Find out more
ORIGINAL RESEARCH article
Front. Conserv. Sci. , 30 January 2025
Sec. Animal Conservation
Volume 5 - 2024 | https://doi.org/10.3389/fcosc.2024.1423321
This article is part of the Research Topic Linking Habitat Quality to Population Dynamics for Conservation Decision Making View all 10 articles
Wild populations across the globe face an escalating risk of decline and potential extinction due to a variety of threats. Key among these are habitat loss and degradation, which results in smaller, isolated populations that are vulnerable to stochastic effects. The Endangered black lion tamarin (Leontopithecus chrysopygus) survives in 17 fragments of the Atlantic Forest within the Paranapanema River basin, in southeast Brazil, with an estimated 2,255 individuals. Life history and threat data from the 2005 Population Viability Analysis (PVA) for this species were updated and augmented, including new estimates of environmental resistance factors present in, or projected for, their habitat. Notably, improved estimates of carrying capacity for this species were developed using a plant-based energetic model. Climate change and fire risk data were incorporated to project future carrying capacity, and habitat connectivity supported estimates of black lion tamarin dispersal across this fragmented landscape. The resulting population viability projections using Vortex simulation software identify core subpopulations with low extinction risk and high gene diversity, as well as smaller subpopulations with low long-term viability, highlighting the need for targeted conservation strategies across the fragmented metapopulation.
Wild populations are at risk of decline or even extinction due to many threats, including habitat loss or degradation that can fragment populations. Small, isolated populations are impacted more significantly by the synergistic impacts of stochastic processes. As a result, ecological, demographic, and genetic population consequences may interact in a feedback loop, leading the species into an extinction vortex (Gilpin and Soulé, 1986). To assess these risks for the black lion tamarin (Leontopithecus chrysopygus), categorized as Endangered (EN) on both the IUCN Red List (Rezende et al., 2020) and the Brazilian national assessment (Brasil, Ministério do Meio Ambiente (MMA), 2022), a Population Viability Analysis (PVA) was completed in 2005 (Holst et al., 2006) using the best available data for lion tamarin species and expert opinion. Interdisciplinary actions have been implemented from the resulting Action Plan and the subsequent Brazilian National Actions Plans (NAP) developed for this species. Since 2005, this PVA has guided the conservation of the species and informed decisions regarding habitat management and conservation actions, with the management goal of retaining >98% gene diversity and having <2% probability of extinction for 100 years. However, with accumulated results from various research and conservation activities, including the emergence of new knowledge about additional populations and threats affecting both habitat and wild populations, the need for an updated PVA became essential to re-evaluate projected viability and needed management actions. The aim of this modeling effort was to update the black lion tamarin PVA by incorporating the most current population information and to improve the model parameters by incorporating habitat-based data and risks imposed by fire and climate change. This will provide an improved tool for future conservation planning by managers and other stakeholders. We also explored the importance of connectivity across this fragmented landscape for this species to help guide management efforts.
Wild populations of the black lion tamarin (BLT) are currently present in 17 locations within the Atlantic Forest in the Paranapanema River basin, in the state of São Paulo, in southeast Brazil. These locations were historically connected; therefore, for management purposes, the populations that live there fall under the concept of metapopulation and will be treated here as subpopulations, subject to a dynamic of interdependence. These subpopulations were modeled using Vortex software v10.4 (Lacy and Pollak, 2020), a Monte Carlo individual-based simulation that can incorporate both stochastic and deterministic processes on demography and genetics (Lacy, 1993, 2000a, 2000b; Lacy et al., 2019; Lacy, 2019). Vortex is well suited to model the life history and stochastic processes of small vertebrate populations such as the BLT metapopulation.
The value and appropriate use of PVA projections is directly related to the quality of data used to estimate model parameter inputs, including demogaphic rates, population status, genetics, and projected threats. Habitat carrying capacity (K) for the target species can be a crucial model parameter, as it places a limit on population size that, in turn, affects population viability, and also affects density-dependent demographic rates. However, this parameter can be among the most challenging to estimate.
The relationship between wild BLT subpopulations and the conditions and characteristics of the vegetation cover and habitat (i.e., the environmental resistance) are key, as these define the habitat carrying capacity for the species and affect demographic rates. Consequently, for this PVA we sought to improve estimates of current habitat carrying capacity and BLT subpopulation sizes based on an energetics model study. Additional data sources were used to project future carrying capacity for BLTs based on projections of climate change effects and estimated fire risk, accounting for the regeneration and recovery capacity of the vegetation in affected areas. Projected progressive increases in suitable BLT habitat due to restoration efforts underway in the ecological cores of the Pontal do Paranapanema region – the subbasin at the mouth of the Paranapanema River – were considered in estimating future dispersal patterns of the species. We offer this as an example of how to incorporate data and methodology from other research into PVA to improve its effectiveness for evaluating conservation needs and actions. By incorporating additional data (e.g., updated population census), knowledge (e.g., new subpopulations), parameterization tools (e.g., plant-based energetics model to estimate K), and risk factors (e.g., habitat-based estimation of fire risk, projected climate change impacts), we endeavor to develop a more current and comprehensive model that can support future conservation decision making for this species. The resulting model also enables us to assess the relative importance that connectivity may play in population viability.
The area of this study corresponds to the Atlantic Forest fragments of the current BLT occurrence (Figure 1), within the hydrological basin of the Paranapanema River (Garbino et al., 2016) that can be considered as three regions for BLTs. About 60% of the BLT wild population lives in western state of São Paulo, a region called Pontal do Paranapanema (Pontal metapopulation), divided in four fragments: the Morro do Diabo State Park, two private fragments, and the Black Lion Tamarin Ecological Station (Holst et al., 2006; Garbino et al., 2016). In the center-east of the state, the Médio Paranapanema region (Médio metapopulation), are the two subpopulations of Caetetus Ecological Station and Fazenda Rio Claro/Turvinho, and a reintroduced population in Mosquito Private Natural Heritage Reserve (RPPN in Portuguese, acronym for Reserva Particular do Patrimônio Natural). In south-eastern São Paulo, the Alto Paranapanema region (Alto metapopulation), the species occurs in fragments in Guareí, Buri, Itapeva, Paranapanema, Taquarivaí and Angatuba municipalities (Culot et al., 2015; Lima et al., 2003). A recent record of BLT groups in the Paranaciacaba Mountain chain, in the municipalities of Pilar do Sul and São Miguel Arcanjo, could significantly influence the population dynamics and conservation status of the species (Röhe et al., 2003; Rodrigues et al., 2016).
Figure 1. Black lion tamarin distribution map showing each of the 17 known subpopulations for the species (red) and the possible occupancy areas (green) considered in this study. Each subpopulation is numbered and named. Data source: IPÊ, Institute for Ecological Research.
Black lion tamarins belong to the family Callitrichidae, the smallest primates in the Neotropics, are diurnal, and live in family groups of usually two to eight individuals (Coimbra-Filho and Mittermeier, 1977). They have frugivorous-insectivorous diets, but consume other food items like nectar, exudates (gum and sap), and small vertebrates (Valladares-Pádua, 1993; Passos, 1999). BLTs consume at least 53 fruit species across 24 families; consumed fruits are typically small, soft, sweet, and with a lot of pulp, and are collected more from trees than from vines or lianas, most of which belong to the Myrtaceae family (Valladares-Pádua, 1993). One of the most frequently eaten fruits throughout the year, Syagrus romanzoffiana (Arecaceae), has been identified as the most important resource in many areas where BLTs occur, as it is available throughout the dry season when most other fruits are not (Valladares-Pádua, 1993; Mamede-Costa and Gobbi, 1998). The consumption of tree exudates comprised 0 to 55% of the observations, mainly during the dry season (Passos, 1999). Foraging occurs in natural hollows of trees, bark, palm fronds and sheaths of fronds, flowers, and other similar places (Valladares-Pádua, 1993; Passos, 1999). Tree hollows are the predominant places for night shelter, and all these sites belong to or are close to areas of intensive use by the groups (Valladares-Pádua, 1993). The BLT occupancy areas total ~480 km2 (Valladares-Pádua, 1993; Baker et al., 2002), and the number of individuals in the wild was estimated at 1,500 in 2005 (Paranhos, 2006; Holst et al., 2006).
The Vortex simulation program tracks an age- and sex-structured population, stepping each individual through a series of life events (reproduction, survival, inter-population dispersal) for each time step (typically one year). Age- and sex-specific demographic rates are entered as means that represent probabilities and are applied by generating a random number from 0 to 1 for each individual and event to determine the stochastic result (e.g., survival or death; breed or not breed), a process that inherently incorporates demographic stochasticity into the results. Means can be entered as a fixed value or as a distribution to incorporate parametric uncertainty. Year-to-year environmental variation (EV) is inputted as a standard deviation around the mean rate to create a binomial distribution used for the entire iteration, from which the year-specific mean rate to be used for all individuals in a given year is selected. Catastrophes are treated as outlier events with a specified annual risk of occurrence (by generating a random number from 0 to 1 at the beginning of each year and comparing it to the specified annual probability of occurrence) that can be specified to affect survival (e.g., disease), reproduction and/or carrying capacity (e.g., fire). Initial individuals are given two unique alleles at one neutral locus to track genetic diversity through a gene drop process, while kinships are used to track individual inbreeding coefficients. Inbreeding depression can be applied through recessive lethal alleles and/or decreased survival of inbred individuals (see Supplementary Material and Lacy et al., 2019 for further details on Vortex model structure and processes).
Life history model inputs were taken from the 2005 PVA black lion tamarin model (Holst et al., 2006), which was based upon the best available field data for lion tamarin species and opinion of species experts at that time. Baseline life history data were taken primarily from a 21-year dataset for wild golden lion tamarins (L. rosalia) by Baker and Dietz (Baker and Dietz, 1996; Baker et al., 1993, 2002; Dietz and Baker, 1993; Dietz and Baker, unpublished data; Dietz et al., 1997) and used to calculate reproductive and survival parameters, mating system, inbreeding effects, environmental variation, and density-dependent predation effects (Holst et al., 2006). These inputs are generally described below and more fully in the Supplementary Material (Supplementary Table 1 and accompanying text) and Holst et al. (2006).
A base scenario was developed with the best available estimates for BLT demographic rates and inbreeding sensitivity (Supplementary Table 1) but with no catastrophes or habitat loss. Sensitivity testing scenarios were developed using populations of 500 individuals (N0=K=500) and varying inputs by +/-10% of the base value, where appropriate (calculated on survival, not mortality) to assess the sensitivity of model results to these model inputs. For age-based inputs (e.g., first age of reproduction), which must be entered as integers, we tested ages within 1-2 years of the base value that were biologically plausible. The Current scenario represents the current BLT metapopulation and habitat estimates, incorporates additional threats (catastrophes and habitat loss due to climate change), and represents the best available estimates for current and future conditions to project future viability of BLT subpopulations and metapopulation (Supplementary Table 1). Additional scenarios (Isolated) were developed by removing all connectivity between subpopulations from the Current scenario. Each scenario was run (1000 iterations) for 100 years (starting in 2020); this represents approximately 14 generations for this species (Baker et al., 2002 apud Kleiman and Rylands, 2008; Rezende et al., 2020) and is sufficient to observe instabilities in populations and long-term population trends (Lacy et al., 2019). Model outputs included mean and standard deviation for population size (N), gene diversity (GD, i.e., expected heterozygosity based on a single neutral locus), and stochastic growth (r), as well as estimated extinction risk (PE), for each subpopulation and for combined subpopulations within each region, over the 100-year projections. In this model, extinction is defined as the absence of at least one sex.
Although field and genetic data show that lion tamarins are not entirely monogamous (Baker et al., 2002 apud Kleiman and Rylands, 2008; Garcia et al., 2021; P. D. Freitas, personal communication), long-term monogamy represents the best description of the mating system of the species (Holst et al., 2006). Reproductive lifespan was set at age 4-13 years for females and 4-16 years for males (Holst et al., 2006). Data for wild lion tamarins tracked in the studbook indicate that few animals survive beyond 16 years (Valladares-Pádua, 1993; Baker et al., 2002; Holst et al., 2006). Captive studbook data suggest that females may become post-reproductive at age 13, while males remain reproducing throughout their lives.
The mean percent of adult females breeding in a given year was set at 73% (with 9.4% EV as a standard deviation), based on a 21-year dataset of wild golden lion tamarins (Baker et al., 2002) and used in the 2005 PVA (Holst et al., 2006). Of those adult females that breed in a given year, reproduction was limited to a maximum of two litters per breeding pair per year, with a maximum of two offspring per litter (at equal sex ratio). Reproduction was not considered to be density-dependent. Mean number of offspring (births) per pair per year was estimated by applying the observed ratio from captive litter size/mean # offspring per year in the wild for golden lion tamarins (1.9/2.14) and applying this to captive BLT mean litter size (1.76). The resulting average of 1.99 offspring per year was applied to reproducing pairs, as follows: 82.5% (1 litter) and 17.5% (2 litters), with one (31%) or two (69%) offspring. These percentages were applied stochastically by drawing a random number from 0 to 1 for each female that breeds in a given year to determine the number of litters she produces that year, and then drawing another random number for each litter to determine if 1 or 2 offspring are born in that litter.
Age- and sex-specific mortality rates were taken from the 2005 PVA (see Supplementary Table 1). While we recognize that mortality causes may differ between protected and non-protected areas, there is no evidence that mortality rates are different. Mortality rates were modelled as density-dependent, increasing when N is over 50% of the habitat’s carrying capacity (K, i.e., the upper limit of BLT population size for that habitat). This was estimated by classifying a greater percentage of observed disappearances of individuals in the field as mortality events as density increases, as species experts agree it is unlikely such individuals successfully found vacancies, especially at high density (see Supplementary Material and Holst et al., 2006 for details). These demographic rates result in a deterministic growth rate of r = 0.1074 at low densities (N < 50% K) and r = 0.0461 at saturation (N = K), prior to the influence of stochastic processes that lower observed growth. Additional mortality is applied in the model via truncation to carrying capacity at the end of each year if N exceeds K, which brings final population growth to approximately zero when the population is at capacity.
The reduction in fitness in inbred individuals is known as inbreeding depression and can be estimated using the function S=S0e-bF, where S0 is the survival of non-inbred individuals, F is the inbreeding coefficient, b is the average number of lethal alleles per haploid genome, and S is the resulting survival rate (Morton et al., 1956). The value b is a measure of the severity of inbreeding effects through both lethal and non-lethal mechanisms. For diploid species, 2b represents the number of “lethal equivalents” that account for inbreeding impacts (see Supplementary Material for details). Inbreeding impacts were modeled as 4.07 lethal equivalents (LEs) applied to first-year survival and 4 LEs to sub-adult survival. These estimates represent the best available data for wild lion tamarins and were based on pedigree data from wild golden lion tamarin populations and used in previous PVA models for other lion tamarin species (Ballou et al., 1998; Holst et al., 2006). The combined value of 8.07 LEs represents 65% of the average impacts estimated for wild vertebrate populations (O’Grady et al., 2006) and are considered by species experts to be a reasonable, conservative estimate for lion tamarins.
Small BLT subpopulations with fewer than 50 individuals (1-Ponte Branca/BLT Ecological Station, 3-San Maria, 4-Santa Monica, 5-RPPN Mosquito, 6-Caetetus Ecological Station 8-Paranapanema Ecological Station, 13-Angatuba Ecological Station, 14-Riparian forests Guareí, 15-Guareí/fragment, and 16-Guareí/Santo Antônio Farm; see Table 1 for population sizes) are believed to have been isolated for at least 10-20 years, with the current individuals more likely to be related to each other than in the larger subpopulations. To account for this, both initial kinships and individual inbreeding coefficients in these small subpopulations were set to 0.01 based on modeling of inbreeding accumulation in similarly sized hypothetical BLT subpopulations (~ mean inbreeding after 10-15 years). Results for mean gene diversity (GD) and mean inbreeding (F) were calculated based on these kinships instead of on allele frequencies (software default option).
Seventeen habitat fragments harboring BLTs were identified (Figure 1, population numbers 1-17), as well as three additional adjacent habitat fragments with no records of BLT presence but in the process of being connected to BLT-occupied habitat through forest corridor restoration (Figure 1, population numbers 18-20). These 17 occupied fragments and 3 empty fragments are located in three sub-watersheds of Paranapanema river basin, here called regions: Pontal (1-2-3-4-18-19-20); Medio (5-6-7); and Alto (8-9-10-11-12-13-14-15-16-17). These fragments, and the size of each fragment, were defined as follows:
a. If the fragment is a Brazilian protected area, the official size was used. Fragments: 1-Ponte Branca (BLT Ecological Station); 5-RPPN Mosquito; 6-Caetetus Ecological Station; 8-Paranapanema Ecological Station; 13-Angatuba Ecological Station; 18-Tucano (BLT Ecological Station); 19-Água Sumida (BLT Ecological Station); 20-Santa Maria (BLT Ecological Station).
b. If BLT fieldwork has been carried out within a fragment, the areas identified as suitable habitat for the species were used as a baseline and summed to adjacent (connected) areas given by the MapBiomas database of forest remnants (Souza et al., 2020). Fragments: 2-Morro do Diabo State Park; 7-Rio Claro/Turvinho; 12-Capão Bonito National Forest (FLONA)/Apiaí-Mirim River.
c. If no information exists on fragment size, the MapBiomas database of forest remnants (Souza et al., 2020) was used to estimate the areas occupied by BLTs. Fragments: 3-San Maria; 4-Santa Mônica; 9-Itapeva; 10-Buri; 11-Riparian forests Apiaí-Guaçu/Taquarivaí; 14-Riparian forests Guareí; 15-Guareí (fragment); 16-Guareí (Santo Antônio Farm).
d. For the population of the Carlos Botelho State Park/Serra de Paranapiacaba, where the species occupancy is unknown, a buffer zone was estimated between the two occurrence records (32 km apart) within this continuous forest. Fragment: 17-Serra de Paranaciacaba (Carlos Botelho State Park and João XXIII).
The starting BLT population size for each of the 17 occupied habitat fragments (see corresponding numbers in Figure 1) was derived as follows:
a. If a population density study was carried out, the available information was used. Fragments: 2-Morro do Diabo State Park (Paranhos, 2006), 4-Santa Mônica (Culot et al., 2018), 5-RPPN Mosquito (Culot et al., 2018), 6-Caetetus Ecological Station (Passos, 1999), 7-Rio Claro/Turvinho (Mamede-Costa, 1997), 12-FLONA Capão Bonito/Apiaí-Mirim River (Caldano, 2014), 13-Angatuba Ecological Station (Culot et al., 2018), 14-Riparian forests Guareí, 15-Guareí/fragment, 16-Guareí/Santo Antônio Farm (Pinto, 2017).
b. If population density study was not conducted, but the fragment was in close proximity to one where this information is known and has similar characteristics (size, successional state, vegetation type, and potential connectivity), the density value was extrapolated with high or low confidence, according to the available knowledge from fieldwork activities in those areas. Fragments: 1-Ponte Branca/BLT Ecological Station and 3-San Maria (2-Morro do Diabo State Park values), 11-Riparian forests Apiaí-Guaçu/Taquarivaí, 10-Buri and 9-Itapeva (12-FLONA Capão Bonito/Apiaí-Mirim River values).
c. If there are no studies and the fragment characteristics are unknown, the lowest population density recorded for the species was used to estimate population size (Ruiz-Miranda et al., 2019), in this case, 0.01 individuals/ha (Passos, 1999). Fragments: 17-Carlos Botelho State Park/Serra de Paranapiacaba.
Because of the uncertainty in the current population size for each subpopulation, it was decided to start each simulation with an initial population size drawn from a shifted and scaled beta distribution defined by the minimum, maximum, and the best estimate of the current N for each of the 17 subpopulations (Table 1). In technical terms, for each population we changed the support of the beta function beta(x;α,β) from x∈[0,1] to y∈[Min N, Max N] and adjusted α and β so that the mode of beta(y;α,β) is equivalent to the Estimate of current N (with Min N, Max N, and Estimate of current N according to Table 1). This incorporates our uncertainty in the current number of BLTs in each subpopulation. Draws from this composed beta distribution (10,000 iterations) result in a right-skewed distribution of the current number of black lion tamarins in the wild, ranging from approximately 2,000 to 3,100, with a mode of 2,255 and a mean of 2,453 (SD = ± 186). This value may be overestimated, due to the extrapolation of the population density for Carlos Botelho State Park/Serra de Paranapiacaba, whose occupied area is completely unknown, but since it is an extensive continuous forest, its estimated population size raised the total population by at least 256 individuals (based on Min N; Table 1).
Distribution of initial individuals into age and sex classes followed an estimated stable age distribution by Vortex based on demographic rates when N/K = 0.75. All initial individuals were assumed to be unrelated (kinships = 0) except for small subpopulations as described above under Species-based Model Inputs.
While the wild BLT metapopulation is fragmented, some habitat connectivity is estimated to exist among some of these fragments, due in part to habitat corridor restoration efforts or to existing riparian forests. To estimate long-distance dispersal rates, information on the use of the habitat matrix by the species (Pinto, 2017; Santos, 2016) and the current state of the landscape connectivity were considered, as these factors directly influence the dispersion of individuals between one subpopulation and another. Four sets of habitat fragments with potential connectivity were identified, falling in the Pontal and Alto regions: Fragments 1-Ponte Branca (BLT Ecological Station)/2-Morro do Diabo State Park/18-Tucano (BLT Ecological Station); fragments 3-San Maria/4-Santa Mônica/19-Água Sumida (BLT Ecological Station)/20-Santa Maria (BLT Ecological Station); fragments 8-Paranapanema Ecological Station/9-Itapeva/10-Buri/11-Riparian forests Apiaí-Guaçu/Taquarivaí/12-FLONA Capão Bonito/Apiaí-Mirim River; and fragments 13-Angatuba Ecological Station/14-Riparian forests Guareí/15-Guareí (fragment)/16-Guareí (Santo Antônio farm). For connected fragments, the rate of inter-population movement in each direction was classified into one of three categories, defined as follows: low = 1 black lion tamarin every ~10 years; moderate = 1 black lion tamarin every ~2.5 years; high = ~2 black lion tamarins every year, based on field observations and habitat corridor characteristics (Table 2).
Inter-population dispersal was restricted in the model to individuals between 2-4 years of age, with males (~85%) dispersing in a greater proportion than females (~15%), according to studies of the black lion tamarin (Perez-Sweeney et al., 2008) and the behaviorally similar golden lion tamarin (Kleiman and Rylands, 2008). Inter-population dispersal was achieved through probabilistic risk of dispersal for each individual age 2-4 years, with sex-biased dispersal achieved by applying a higher probability of dispersal to males than to females. Sub-adults disperse from their natal area in search of habitat and available mates; in this model, only dispersal between subpopulations is relevant and needed to be estimated.
Dispersal was limited in the model to years when population size is greater than 90% of K (N > 0.9*K) to simulate the need for individuals to search areas outside of the subpopulation. Mortality (10%) was applied to dispersing animals to simulate risk of death before reaching another subpopulation. Dispersers were not allowed to enter saturated subpopulations (N ≥ K). Individuals from adjacent areas were allowed to disperse into the three empty habitat patches included in the Pontal metapopulation (#18-20) when connectivity is estimated to exist.
Additional scenarios with no connectivity (Isolated scenarios) were run for the Alto and the Pontal metapopulations to assess the relative impact of existing connectivity on BLT population viability.
Values for K0 for the habitat currently available to each subpopulation were estimated based on an energetic model developed with specific parameters for the species, considering the abundance of a key resource in the black lion tamarin’s diet, i.e., fruits of the palm Jerivá, Syagrus romanzoffiana (Valladares-Pádua, 1993; Passos, 1999; Mamede-Costa and Gobbi, 1998). We used information on the abundance of S. romanzoffiana in areas where this information was available from field studies (i.e., 1-Ponte Branca/BLT Ecological Station, 2-Morro do Diabo State Park, 3-San Maria, 6-Caetetus Ecological Station, 7-Rio Claro/Turvinho, 10-Buri, 13-Angatuba Ecological Station, 16-Guareí/Santo Antônio Farm, 18-Tucano/BLT Ecological Station, 19-Água Sumida/BLT Ecological Station) and estimated the abundance for all other areas through a simple regression between fragment size and abundance. Finally, based on these values, we estimated the carrying capacity for each subpopulation using an energetic model (Rezende et al., in prep.).
There are several methods to determine K. In this case, we used the model of nutritional contribution and energy methods (McCall et al., 1997), where the nutritional components required by the animal (dry matter, digestible energy, metabolizable energy and crude protein) are related with the kg of vegetable matter and/or the nutritional components available for animal consumption. This type of model shows a good approximation with population density and carrying capacity (McCall et al., 1997).
Considering that the Morro do Diabo population is large and believed to be self-sustaining, we assumed that the population in that area approaches carrying capacity. Based on the energetic model developed, the estimated K for this population was very close to the estimated population for this area (85%; estimated N0 = 1142, estimated K0 = 1340), which gave us confidence in using this model for estimating K for the other areas.
To incorporate the effects of climate change into K, we examined the estimates of changes in habitat suitability, based on niche models that included projections for the years 2050 and 2080 (Meyer et al., 2014), for the protected areas inhabited by BLTs in each region (Figure 1, Populations #1, 2, 18, 19, 20 in Pontal, #6 in Médio and #17 in Alto). We extrapolated these trends in habitat suitability values to the other populations in each respective region (Alto Paranapanema, Medio Paranapanema, and Pontal do Paranapanema); however, the resulting degree of change was thought by species specialists to be overestimated, with some areas losing all capacity to harbor black lion tamarins (K=0).
Colombo and Joly (2010) projected a 20% reduction of the possible occurrence of 38 plant species of the Atlantic Forest (including many genera that are key resources for black lion tamarins) due to climate change. We used this as a proxy of reduction in the carrying capacity of habitat for BLTs. Therefore, to incorporate effects of climate change into our model, we considered 20% as the maximum reduction in K that would occur by 2080 (next 60 years). We rescaled the projected losses proposed by Meyer et al. (2014) to 20% of the rates they proposed for the BLT subpopulations, following the same trend from present to 2050 to 2080. All changes were modeled as linear. For the second set of scenarios, we extended the same rate of loss from 2050 to 2080 to the end of the 100-year simulation (Table 3).
Table 3. Initial carrying capacity (K0) and climate change impacts on K after 30 (KCC30) and 60 (KCC60) years for each BLT subpopulation used in the Vortex model.
In the model, the Catastrophe option was used to incorporate the occurrence of fires (local to each subpopulation) based on annual probabilities. The impact of fire on K and the recovery of the habitat and K were tracked through the use of custom population variables.
The Kernel Density Estimator is an interpolation technique that generates a density surface for visual identification of areas called “hotspots”, which are a concentration of events that indicates the clustering of a phenomenon in a spatial distribution. Thus, to estimate the fire risk for each fragment, a kernel map was produced using as raw data heat detection events generated and provided by the Brazilian Institute for Space Research Instituto Nacional De Pesquisas Espaciais (INPE) (2020) for the period from 2015 to 2020, within the extent of occurrence of BLTs (Figure 2). These events have, in their attribute table, a fire risk index (FRI), already calculated by INPE, using as a principle the meteorological estimate of the vegetation burning index, in which the more consecutive days without rain in a location, the greater its risk of burning. Additionally, the local effects of the vegetation type and the natural cycle of its defoliation, the maximum daily temperature and minimum relative humidity, the topographic elevation and latitude, as well as the presence of fire in the area of interest are considered in the calculation ( (Instituto Nacional De Pesquisas Espaciais (INPE), 2019). These FRI values are organized into five categories, within a scale of 0 to 1: Minimum, below 0.15; Low, from 0.15 to 0.4; Medium, from 0.4 to 0.7; High, from 0.7 to 0.95; and Critical, above 0.95. For the present analysis, only those events whose FRI was equal to or greater than 0.5 were used. Therefore, we were conservative and more precise to ensure the quality of the heat detection event that was selected to produce our Kernel map.
Figure 2. Fire risk hotspots within the extent of occurrence of black lion tamarins. Data source: INPE/MCTIC; CPB/ICMBio/MMA; IPÊ.
From these selected data, we spatially modeled the distribution of known heat detection events for the years studied within our area of interest, thus observing the spatial and temporal patterns of this phenomenon. Kernel assigned a value for each pixel according to the concentration of hotspots and combined with a function that counts all points within an area of influence. Based on this value, extracted for the pixel corresponding to each record of BLT groups within a given subpopulation, we calculated the fire risk for each subpopulation - for subpopulations with more than one record, a simple average between the values was calculated. These values were then converted to annual risk of fire and added into the model as a catastrophe that results in a reduction in K, with a linear recovery of K over the length of time (Table 4). The number of years for K to return to pre-fire K considered the size and shape of the fragments (whether it is a large continuous area, smaller round/square fragment or riparian forest), since this directly influences the resilience and recovery capacity of this type of forest.
No impacts of fire on survival or reproduction were included in the model. When the population is below K, it is assumed that black lion tamarins can escape to empty adjacent (unburned) habitat in the same habitat fragment; if N is close to or at K, then reduced K in the model will cause a corresponding reduction in N due to truncation of N to K at the end of each model year.
Custom population variables were used to incorporate the interaction of fire and climate change impacts on BLT habitat (K). Vortex first calculated the estimated K for the year due to climate change. The model then applied any loss of K due to fire in that year and any habitat recovery following fires in previous fires. Finally, Vortex compared the two K values, i.e., the K based on climate change (KCC) and the K based on fires and recovery (Kfire), and used the lower value for K for that year. This prevents habitat (K) from recovering to a level higher than the K based on climate change.
Meso-predator events (i.e., brief periods of intense predation) have been observed in golden lion tamarin populations and can have a severe short-term impact in small populations (see lion tamarin PVA in Holst et al., 2006). Such events were included in this model for the six small subpopulations currently estimated with fewer than 20 individuals; for subpopulations of 20 or more individuals, predation is considered part of environmental variation. Since these events have not been observed in this species, and to be conservative, the risk used in this model was 20% of that calculated for golden lion tamarins in the 2005 PVA (i.e., reduced from 10% to 2% risk of occurrence per year). This is a frequency of about once per 50 years or about 14% chance per generation. The severity of such events was modeled as a 50% reduction in survival across all sex and age classes. These values align with the default values suggested for generic severe catastrophes in wild vertebrate populations (Reed et al., 2003).
Stochastic growth (r) was selected as the most informative measure of the sensitivity testing results. Of the ten parameters tested, all of them resulted in positive growth of at least r=0.015 over the range of values tested, suggesting that none is highly critical to model results. Age of first reproduction for females in the model (3 vs 4 years tested) was the most sensitive parameter, but this value is based on good field data and is not subject to management intervention. Other relatively sensitive parameters are the percent of adult females breeding each year, the mean number of offspring produced, and the survival rates for juveniles and subadults. Good data exist for reproduction, while survival rates are more uncertain and can be subject to various threats. Taken together, this highlights the importance of the production of surviving offspring for black lion tamarins and suggests potentially important data gaps. Adult survival also is of some, but less, influence on population growth. Maximum age (16-17 years tested) and maximum reproductive age for females (14-16 years tested) have little effect over the ranges tested and do not represent important data gaps. A generic catastrophe with default Vortex settings (50% reduction in survival, with 1.85% annual risk, based on Reed et al., 2003) has relatively little effect in large populations not under threat given the high positive growth rate. Finally, inbreeding (base values vs no inbreeding) has little impact in an initial genetically diverse population of this size and over 100 years. These results suggest that the primary area in which uncertainty may impact viability projections is for mortality rates, especially for immature individuals. This could be important if there are undetected threats that are not incorporated into the Current model scenario.
Results from the Current scenario show that, taken as a whole, the wild metapopulation of black lion tamarins is projected to retain 99.5% gene diversity and have no risk of extinction over the next 100 years under the conditions modeled, which includes projected connectivity levels. This meets the management goal for the species of retaining >98% gene diversity and having <2% probability of extinction for 100 years. However, the viability of the different BLT subpopulations varies greatly, leading to a projected loss of many smaller fragments and reduction of the species’ range over time.
If we examine the viability projections for the 17 current BLT subpopulations separately, only the two large subpopulations (i.e., 2-Morro do Diabo State Park in Pontal region; 17-Carlos Botelho State Park/Serra de Paranapiacaba in Alto region) meet the same viability criteria given above for long-term persistence and genetic health (Table 5). An additional three subpopulations (i.e., 9-Itapeva, 10-Buri, 11-Riparian forests Apiaí-Guaçu/Taquarivaí) exhibit good viability, with at least 90% gene diversity retained and no risk of being extinct at the end of 100 years. Of the remaining 12 subpopulations, five are projected to have moderate extinction risk (~5-18%) and loss of GD (i.e., 1-Ponte Branca/BLT Ecological Station, 6-Caetetus Ecological Station, 7-Rio Claro/Turvinho, 12-FLONA Capão Bonito/Apiaí-Mirim River, 13-Angatuba Ecological Station), and seven are almost certain to go extinct within 100 years (i.e., 3-San Maria, 4-Santa Monica, 5-RPPN Mosquito, 8-Paranapanema Ecological Station, 14-Guareí/Santo Antônio Farm, 15-Riparian forests Guarei, 16-Guareí/fragment) (Figure 3; Table 5). These results suggest that, while this species may persist in the wild in core areas, many of the smaller fragmented subpopulations will disappear under the model assumptions of future threats and management, despite the possibility of recolonization through current connectivity. If survival rates are moderately lower, as explored in sensitivity testing, this might lower the projected viability of medium-sized subpopulations (such as #9-11) and hasten the extinction of small fragments.
Table 5. Probability of Extinction (PE100), mean population size (N100), and Gene Diversity (GD100) of black lion tamarin subpopulations and metapopulation at year 100, for scenarios with projected current connectivity (Current) and scenarios with no connectivity (Isolated).
Figure 3. Projected mean probability of surviving over 100 years for each of the 12 current subpopulations with extinction risk >0.
The three empty habitat fragments have a low probability of recolonizing naturally given the connectivity assumptions used in the model: <4% of the iterations resulted in some recolonization for subpopulation #18, and <1% for subpopulations #19 and #20. The mean maximum population size for recolonized subpopulations was 6-7 BLTs. These recolonizations cannot be sustained in the long term (100 years) without additional immigrants, underscoring the necessity of implementing management interventions to support the persistence of these subpopulations.
Projections with no connectivity (Isolated scenarios) show the same overall long-term viability results, with large subpopulations remaining viable and the smallest subpopulations going extinct. In general, subpopulations are somewhat smaller, lose gene diversity a bit faster, and may go extinct sooner when isolated compared to the estimated low level of connectivity (Table 5).
Figure 4 shows projections for the Alto and Pontal regional metapopulations, with (Current) and without connectivity (Isolated). Both regional metapopulations show initial growth, which can be explained due to current estimated Ns being below K. For the Pontal region, current subpopulations may be below K due to stochastic events in the small populations plus three empty habitat patches, although undetected threats lowering population size cannot be ruled out. For the Alto region, early growth in the model is driven primarily by the initial low density in the large Paranapiacaba fragment (N0 = 320; K0 = 1314). The reason for the discrepancy between current estimated N and estimated K in this region is not understood and is a substantial source of uncertainty for the projected growth of this subpopulation.
Figure 4. Projected mean population size for Alto and Pontal regional metapopulations, with estimated connectivity (solid) and with no connectivity between fragments (dashed).
Despite the initial growth of these populations toward carrying capacity in the model, these scenarios are useful to demonstrate the decline in BLT numbers over time as suitable habitat is lost through climate change and fire events. Isolation increases demographic stochasticity and inbreeding impacts, which in turn increase the likelihood that more subpopulations in small fragments will go extinct and result in lower mean population size for the species in the wild.
For decades, PVA models have served as invaluable tools for evaluating species population viability and guiding conservation strategies across diverse ecosystems (Lacy, 2019). The efficacy of PVA in informing conservation decisions hinges upon the precision and validity of its underlying inputs. Accurate parameter estimation is crucial, encompassing not only species-specific biological traits and immediate threats like disease or poaching, but also factors related to habitat characteristics and associated risks. This becomes particularly vital when modeling small, isolated populations with restricted connectivity and limited prospects for expansion, as is the case with the black lion tamarin and the scenario we analyzed.
Model parameterization can be improved by utilizing diverse data sources, comprehensive and continuous field studies, and stakeholder contributions. The use of vegetative projections, energetic models and mapping of fire risk hotspots enabled us to develop an improved PVA model for wild BLT subpopulations, incorporating even climate change impacts. This model provides a baseline for testing management actions, such as conservation translocations and the establishment of forest corridors to re-establish gene flow and reduce the extinction risk of small BLT subpopulations (Valladares-Pádua, 1993; Frankham et al., 2004; Rezende, 2014).
Our results suggest the persistence of BLT core populations in the western (Pontal do Paranapanema) and eastern (Alto Paranapanema) points of the species’ range, supported primarily by the two large fragments #2 and #17. Caution should be exercised, however, given the uncertainty in model inputs and assumptions. Data used for the Paranapiacaba (#17) projections in the east, in particular, include substantial uncertainty in density and carrying capacity, and field studies are required to address data gaps for this subpopulation in the Alto Paranapanema region.
The western portion of the current BLT known distribution harbor the remaining stronghold for this species. Except for Morro do Diabo State Park, all subpopulations that comprise this region are small, semi-isolated, and not viable in the long term, with projected accelerated loss of gene diversity, inbreeding accumulation, and risk of extinction. Genetic studies on the small subpopulations of the Pontal do Paranapanema demonstrate the importance of their conservation and long-term maintenance to preserve genetic variation, important for the metapopulation to adapt to environmental changes or pressures and, therefore, to survive (Perez-Sweeney et al., 2008). Current estimated connectivity in this region, and in the Alto Paranapanema region, is projected to be insufficient in itself to ensure long-term viability of most BLT subpopulations outside of these two large core fragments. However, to better evaluate the impact of these corridors in dispersion and recolonization processes, studies on the rate of inter-fragment movement, and the success rate (survival and reproduction) of migrants are needed.
In Pontal do Paranapanema, niche-based models project a 94% reduction of suitable habitat for the species in the Morro do Diabo State Park by 2080 (Meyer et al., 2014). We chose to use a more conservative estimate of habitat loss in this fragment for our projections, as we are unaware of the potential response and adaptation of the BLTs to these changes (Korstjens and Hillyer, 2016). Long-term studies to evaluate the impact of climate change on these primates, particularly focused on the impact of phenological shifts in food sources on the species reproductive cycle and the increased disease risk due to climate change, are indicated to provide improved parameters to the model.
Small population size is the most important factor affecting long-term viability of black lion tamarin subpopulations based on these model results. To increase population viability beyond the few larger subpopulations, it will be important to prioritize the evaluation and planning of management strategies (habitat and individuals), such as increasing protected areas, reconnecting isolated populations with corridors through forest restoration, fire prevention in areas of greatest risk, and translocations (wild-to-wild and/or captive-wild) for population supplementation aiming at increasing their genetic diversity and reducing demographic instability, such as unbalanced age structure or sex ratio. Conservation translocations may favor their adaptive potential to climate change and can be a short-term preventive action to avoid extinction while habitat connectivity is established, both strategies backed by strict forest fire control to increase their immediate and long-term chances of success. For better results in this regard, translocations must be planned and implemented considering the results from population genetic studies.
Our model indicates that restoring and reconnecting small fragments of habitat to promote the dispersal of individuals and restoring genetic flow among subpopulations can contribute to the persistence of the species across this fragmented landscape. To this end, ecological corridors are being reforested to connect small fragments to the large subpopulation of black lion tamarins found in Morro do Diabo State Park (Rezende, 2014; IPÊ, 2019). Supplementation via conservation translocation of individuals may be needed to maintain the size of these subpopulations (i.e., San Maria and Santa Monica) and slow the loss of genetic diversity while these restored corridors become functional forest areas for black lion tamarins. Moreover, reintroducing the species in empty fragments subject to natural recolonization (i.e., Tucano, Água Sumida, and Santa Maria, all belonging to the BLT Ecological Station) may enhance dispersal and recolonization throughout the metapopulation by conspecific attraction (Smith and Peacock, 1990; Zeigler et al., 2011). Additional modeling of such management scenarios, using this baseline PVA and informed by stakeholder input, would be valuable in developing an effective long-term BLT metapopulation management strategy.
In conclusion, this study demonstrates the importance of incorporating new data sources into a Population Viability Analysis (PVA) model of an endangered primate species, the black lion tamarin (Leontopithecus chrysopygus), and reinforce the importance and usefulness of such models in planning actions for its management and conservation. By enhancing the model with habitat-based inputs, such as estimates of fire risk, climate change impacts, and habitat connectivity, we have advanced the understanding of subpopulation dynamics and provided new viability projections for the species metapopulation. This work presents improved models, which resulted in critical information to plan and implement conservation measures for the black lion tamarin, serving as a valuable example for how PVA can be improved for other species facing similar environmental challenges (i.e., fragmentation, climate change, fires and other stochastic effects due to small population size). Thus, this research offers an innovative PVA modeling approach not only to the community focused on the conservation of lion tamarins, but also to researchers, policy makers, managers and other stakeholders involved in decision-making for species conservation.
The raw data supporting the conclusions of this article will be made available by the authors, without undue reservation.
Ethical approval was not required for the study involving animals in accordance with the local legislation and institutional requirements because no data used was collected specifically for this manuscript. Data from other research or published data were used.
FF-S: Conceptualization, Data curation, Formal analysis, Funding acquisition, Investigation, Methodology, Software, Validation, Visualization, Writing – original draft, Writing – review & editing. GR: Conceptualization, Data curation, Formal analysis, Funding acquisition, Investigation, Methodology, Project administration, Resources, Supervision, Validation, Visualization, Writing – original draft, Writing – review & editing. CV-P: Conceptualization, Funding acquisition, Investigation, Resources, Supervision, Validation, Writing – review & editing. FV: Data curation, Formal analysis, Methodology, Software, Visualization, Writing – review & editing. LJ: Conceptualization, Formal analysis, Methodology, Supervision, Validation, Visualization, Writing – review & editing. LP: Data curation, Formal analysis, Investigation, Methodology, Software, Validation, Visualization, Writing – review & editing. KT-H: Conceptualization, Data curation, Formal analysis, Investigation, Methodology, Project administration, Resources, Software, Supervision, Validation, Visualization, Writing – original draft, Writing – review & editing.
The author(s) declare financial support was received for the research, authorship, and/or publication of this article. Wildlife Conservation Network (WCN) and WWF’s Russell E. Train Education for Nature Program (EFN) provided Masters scholarship for FFS. IUCN SSC CPSG Brasil, Disney Conservation Fund and Copenhagen Zoo supported the online workshops for discussion and construction of the BLT base model.
This study is the result of a joint collaboration among different specialists, including biologists, physicists, ecologists, and geneticists, and represents an interdisciplinary effort to support the development of conservation strategies for the black lion tamarins. We extend our sincere gratitude to Mathias Dislich (Parque das Aves/CPSG Brasil), Laurence Culot (Laboratory of Primatology UNESP-Rio Claro), Ariovaldo Pereira da Cruz Neto (Laboratory of Animal Physiology UNESP-Rio Claro), Mara Cristina Marques (São Paulo Zoo), Cauê Monticelli (CECFAU-SEMIL-SP), Paola Andrea Ayala Burbano and Patrícia Freitas (UFSCar-São Carlos), and Fabiana Lopes Rocha and Eugenia Cordero Schmidt (IUCN SSC CPSG Brasil), for their valuable contributions and support in the process of building the base model. Special thanks to the Wildlife Conservation Network (WCN) and the WWF’s Russell E. Train Education for Nature Program (EFN) for their generous financial support of FFS’s Master’s degree studies.
The authors declare that the research was conducted in the absence of any commercial or financial relationships that could be construed as a potential conflict of interest.
All claims expressed in this article are solely those of the authors and do not necessarily represent those of their affiliated organizations, or those of the publisher, the editors and the reviewers. Any product that may be evaluated in this article, or claim that may be made by its manufacturer, is not guaranteed or endorsed by the publisher.
The Supplementary Material for this article can be found online at: https://www.frontiersin.org/articles/10.3389/fcosc.2024.1423321/full#supplementary-material
Baker A., Bales K., Dietz J. (2002). “Mating system and group dynamics in lion tamarins,” in Lion Tamarins: Biology and Conservation. Eds. Kleiman D. G., Rylands A. B. (Smithsonian Institution Press, Washington DC), 188–212.
Baker A., Dietz J. (1996). Immigration in wild groups of golden lion tamarins (Leontopithecus rosalia). Am. J. Primatology 38, 47–56. doi: 10.1002/(SICI)1098-2345(1996)38:1<47::AID-AJP5>3.0.CO;2-T
Baker A., Dietz J., Kleiman D. (1993). Behavioral evidence for monopolization of paternity in multi-male groups of golden lion tamarins. Anim. Behav. 46, 1091–1103. doi: 10.1006/anbe.1993.1299
Ballou J., Lacy R., Kleiman D., Rylands A., Ellis S. (1998). Leontopithecus II: The Second Population and Habitat Viability Assessment for Lion Tamarins (Leontopithecus): Final Report (Apple Valley, MN.: Conservation Breeding Specialist Group (SSC/IUCN).
Brasil, Ministério do Meio Ambiente (MMA). (2022). Portaria MMA n° 148, de 7 de junho de 2022 74. DOU 108.
Caldano L. (2014). Censo populacional e avaliação da variabilidade genética das populações de mico-leão-preto (Leontopithecus chrysopygus, Mikan 1823) na Floresta Nacional de Capão Bonito- SP. Universidade Federal de São Carlos, São Carlos- São Paulo.
Cláudio V.-P. (1993). The ecology, behavior and conservation of the black lion tamarins (Gainesville: Ph.D, University of Florida).
Coimbra-Filho A., Mittermeier R. (1977). “Conservation of the Brazilian lion tamarins (Leonthopithecus rosalia),” in Primate Conservation. (Academic Press), 59–94.
Colombo A. F., Joly C. (2010). Brazilian Atlantic Forest lato sensu: the most ancient Brazilian forest, and a biodiversity hotspot, is highly threatened by climate change. Braz. J. Biol. 70, 697–708. doi: 10.1590/S1519-69842010000400002
Culot L., Griese J., Knogge C., Tonini M, Mulato dos Santos M, Estevam C, et al. (2015). New records, reconfirmed sites and proposals for the conservation of black lion tamarin (Leontopithecus chrysopygus) in the middle and upper Paranapanema. Neotropical Primates 22, 32–39. doi: 10.62015/np.2015.v22.150
Culot L., Pereira L., Agostini I., Almeida M. A., Alves R., et al. (2018). ATLANTIC-PRIMATES: a dataset of communities and occurrences of primates in the Atlantic Forests of South America. Ecology. 100, e02525. doi: 10.1002/ecy.2525
Dietz J., Baker A. (1993). Polygyny and female reproductive success in golden lion tamarins Leontopithecus rosalia. Anim. Behav. 46, 1067–1078. doi: 10.1006/anbe.1993.1297
Dietz J., Peres C., Pinder L. (1997). Foraging ecology and use of space in wild golden lion tamarins (Leontopithecus rosalia). Am. J. Primatology 41, 289–305. doi: 10.1002/(SICI)1098-2345(1997)41:4<289::AID-AJP2>3.0.CO;2-T
Frankham R., Ballou J., Briscoe D. (2004). A primer of conservation genetics (Cambridge, UK; New York: Cambridge University Press).
Garbino G., Rezende G., Valladares-Padua C. (2016). Pelage variation and distribution of the black lion tamarin, leontopithecus chrysopygus. Folia Primatologica 87, 244–261. doi: 10.1159/000450998
Garcia F., Prado B., Souza E., MaChado V., Albino C., Rocha V. (2021). Nest box use and polygyny in an endangered primate species: the black lion tamarin (Leontopithecus chrysopygus). Oecologia Australis. 25, 166–173. doi: 10.4257/oeco.2021.2501.16
Gilpin M., Soulé M. (1986). “Minimum viable populations: processes of species extinction,” in Conservation biology: the science of scarcity and diversity. Ed. Soulé M. E. (Sinauer Associates Inc., Sunderland, MA), 19–34.
Holst B., Medici P., Marino-Filho O., Kleiman D., Leus K., Pissinatti A., et al. (2006). Lion Tamarin Population and Habitat Viability Assessment Workshop 2005, final report (Apple Valley, MN, US: IUCN/SSC Conservation Breeding Specialist Group). Available at: https://www.cpsg.org/sites/default/files/2024-05/Lion%2520Tamarin%2520PHVA%25203.pdf (Accessed March 15, 2020).
Instituto Nacional De Pesquisas Espaciais (INPE) (2019). Relatório do Método do Cálculo do Risco de Fogo do Programa INPE – versão 11, junho 2019. Available online at: http://mtc-m21c.sid.inpe.br/col/sid.inpe.br/mtc-m21c/2019/11.21.11.03/doc/publicacao.pdf (Accessed April 6, 2020).
Instituto Nacional De Pesquisas Espaciais (INPE) (2020). Banco de Dados de queimadas. Available online at: http://www.inpe.br/queimadas/bdqueimadas (Accessed April 6, 2020).
Korstjens A. H., Hillyer A. P. (2016). “Primates and climate change: A review of current knowledge,” in An Introduction to Primate Conservation. Eds. Wich S. A., Marshall A. J. (Oxford University Press, Oxford), 175–192.
Lacy R. (1993). VORTEX: A computer simulation model for Population Viability Analysis. In Wildlife Research (Australia: CSIRO Publishing).
Lacy R. (2000a). Considering threats to the viability of small populations. Ecol. Bulletins 48, 39–51.
Lacy R. (2000b). Structure of the VORTEX simulation model for population viability analysis. Ecol. Bulletins 48, 191–203.
Lacy R. (2019). Lessons from 30 years of population viability analysis of wildlife populations. Zoo Biol. 38, 67–77. doi: 10.1002/zoo.21468
Lacy R., Miller P., Traylor-Holzer K. (2019). Vortex 10 User’s Manual (Wiley: IUCN SSC Conservation Breeding Group & Chicago Zoological Society).
Lacy R., Pollak J. P. (2020). Vortex: A Stochastic Simulation of the Extinction Process (Brookfield, Illinois, USA: Chicago Zoological Society).
Laurence C., Lucas P., Ilaria A., Antônio A. M., Rafael A., Izar A., et al. (2018). ATLANTIC-PRIMATES: a dataset of communities and occurrences of primates in the atlantic forests of south america. Ecology. 100, e02525. doi: 10.1002/ecy.2525
Lima F., da Silva I., Martins C., Padua C. (2003). On the occurrence of the black-lion-tamarin (Leontopithecus chrysopygus) in Buri, São Paulo, Brazil. Neotropical Primates 11, 144–145. doi: 10.62015/np.2003.v11.536
Mamede-Costa A. C. (1997). Ecologia de um grupo de micos-leões-pretos (Leontopithecus chrysopygus, Mikan 1823) na mata ciliar da Fazenda Rio Claro, Lençóis Paulista, SP. Universidade Estadual Paulista (UNESP, Rio Claro-SP.
Mamede-Costa A. C., Gobbi N. (1998). The black lion tamarin Leontopithecus chrysopygus – its conservation and management. Oryx 32, 295–300. doi: 10.1046/j.1365-3008.1998.d01-59.x
McCall T., Brown R., Bender L. (1997). Comparison of techniques for determining the nutritional carrying capacity for white-tailed deer. J. Range Manage 50, 33–38. doi: 10.2307/4002702
Meyer A., Pie M., Passos F. (2014). Assessing the exposure of lion tamarins (Leontopithecus spp.) to future climate change: Exposure of Lion Tamarins to Climate Change. Am. J. Primatology 76, 551–562. doi: 10.1002/ajp.22247
Morton N. E., Crow J. F., Muller H. J. (1956). An estimate of the mutational damage in man from data on consanguineous marriages. Proc. Natl. Acad. Sciences U.S.A. 42, 855–863. doi: 10.1073/pnas.42.11.855
O’Grady J., Brook B., Reed D., Ballou J., Tonkyn D., Frankham R. (2006). Realistic levels of inbreeding depression strongly affect extinction risk in wild populations. Biol. Conserv. 133, 42–51. doi: 10.1016/j.biocon.2006.05.016
Paranhos K. (2006). Estimativas populacionais para espécies raras: o Mico-leão-preto Leontopithecus chrysopygus (Mikan 1823) como modelo (Curitiba: Universidade Federal do Paraná).
Passos F. (1999). Dieta de um grupo de mico-leão-preto, Leontopithecus chrysopygus (Mikan) (Mammalia, Callitrichidae), na Estação Ecológica dos Caetetus, São Paulo. Rev. Bras. Zoologia 16, 269–278. doi: 10.1590/S0101-81751999000500019
Peloggia C. L. T.. Censo populacional e avaliação da variabilidade genética das populações de mico-leão-preto (Leontopithecus chrysopygus mika) na floresta nacional de capão bonito- SP. 2014. 65 f (São Carlos: Dissertação (Mestrado em Ciências Biológicas) - Universidade Federal de São Carlos).
Perez-Sweeney B., Valladares-Padua C., Martins C., Morales J., Melnick D. (2008). Examination of the taxonomy and diversification of leontopithecus using the mitochondrial control region. Int. J. Primatology 29, 245–263. doi: 10.1007/s10764-007-9224-7
Pinto B. (2017). Influência da paisagem e das características locais na ocorrência do mico-leão-preto (Leontopithecus chrysopygus, Callitrichidae). Mestre em Ciências Biológicas (Zoologia) (Rio Claro -São Paulo: Universidade Estadual Paulista).
Reed D., O’Grady J., Brook B., Ballou J., Frankham R. (2003). Estimates of minimum viable population sizes for vertebrates and factors influencing those estimates. Biol. Conserv. 113, 23–34. doi: 10.1016/S0006-3207(02)00346-4
Rezende G. C., Knogge C., Passos F., Ludwig G., Oliveira L., Jerusalinsky L., et al. (2020). The IUCN Red List of Threatened Species 2020: Leontopithecus chrysopygus (International Union for Conservation of Nature and Natural Resources).
Rodrigues S., Gagetti B. L., Piratelli A. (2016). First record of Leontopithecus chrysopygus (Primates: Callitrichidae) in Carlos Botelho State Park, São Miguel Arcanjo, São Paulo, Brazil. Mammalia. 2014, 121–124. doi: 10.1515/mammalia-2014-0104
Röhe F., Antunes A., Tófoli C. (2003). The discovery of a new population of black lion tamarins (Leontopithecus chrysopygus) in the Serra de Paranapiacaba, Sao Paulo, Brazil. Neotropical Primates 11, 75–76.
Ruiz-Miranda C., De Morais M., Dietz L. A., Alexandre B., Martins A., Ferraz L., et al. (2019). Estimating population sizes to evaluate progress in conservation of endangered golden lion tamarins (Leontopithecus rosalia). PloS One 14, e0216664. doi: 10.1371/journal.pone.0216664
Santos M. (2016). O uso da matriz pelo mico-leão-preto, Leontopithecus chrysopygus, no município de Guareí, São Paulo. Trabalho de conclusão de curso (licenciatura e bacharelado - Ciências biológicas) (Rio Claro - São Paulo: Universidade Estadual Paulista).
Smith A. T., Peacock M. M. (1990). Conspecific attraction and the determination of metapopulation colonization rates. Conserv. Biol. 4, 320–323. doi: 10.1111/j.1523-1739.1990.tb00294.x
Souza C., Shimbo J. Z., Rosa M., Parente L., Alencar A., Rudorff B., et al. (2020). Reconstructing three decades of land use and land cover changes in Brazilian biomes with landsat archive and earth engine. Remote Sens. 12, 2735. doi: 10.3390/rs12172735
Suelen R., Bruna L.G., Augusto P. (2016). First record of leontopithecus chrysopygus (Primates: Callitrichidae) in carlos botelho state park, são miguel arcanjo, são paulo, brazil. Mammalia. Mammalia. doi: 10.1515/mammalia-2014-0104
Valladares-Pádua C. (1993). The ecology, behavior and conservation of the black lion tamarins (Gainesville: University of Florida).
Keywords: carrying capacity, climate change, fire risk, vortex, metapopulation, conservation planning
Citation: Forero-Sánchez F, Rezende GC, Valladares-Pádua C, Vannucchi FS, Jerusalinsky L, Pacca L and Traylor-Holzer K (2025) Securing black lion tamarin populations: improving habitat-based inputs and risks for population viability analysis to inform management decisions. Front. Conserv. Sci. 5:1423321. doi: 10.3389/fcosc.2024.1423321
Received: 25 April 2024; Accepted: 30 December 2024;
Published: 30 January 2025.
Edited by:
David R. Breininger, University of Central Florida, United StatesReviewed by:
Aurelio F. Malo, University of Alcalá, SpainCopyright © 2025 Forero-Sánchez, Rezende, Valladares-Pádua, Vannucchi, Jerusalinsky, Pacca and Traylor-Holzer. This is an open-access article distributed under the terms of the Creative Commons Attribution License (CC BY). The use, distribution or reproduction in other forums is permitted, provided the original author(s) and the copyright owner(s) are credited and that the original publication in this journal is cited, in accordance with accepted academic practice. No use, distribution or reproduction is permitted which does not comply with these terms.
*Correspondence: Francy Forero-Sánchez, ZmZvcmVyb3NhbmNoZXpAZ21haWwuY29t
†These authors have contributed equally to this work and share first authorship
Disclaimer: All claims expressed in this article are solely those of the authors and do not necessarily represent those of their affiliated organizations, or those of the publisher, the editors and the reviewers. Any product that may be evaluated in this article or claim that may be made by its manufacturer is not guaranteed or endorsed by the publisher.
Research integrity at Frontiers
Learn more about the work of our research integrity team to safeguard the quality of each article we publish.