- 1Department of Landscape Architecture, University of Florida, Gainesville, FL, United States
- 2Center for Landscape Conservation Planning, Department of Landscape Architecture, University of Florida, Gainesville, FL, United States
- 3Institute of Science and Public Affairs, Florida State University, Tallahassee, FL, United States
- 4Conservation Science, Inc., Melrose, FL, United States
- 5Department of Wildlife Ecology and Conservation, University of Florida, Gainesville, FL, United States
- 6Independent Researcher, Fort Pierce, FL, United States
- 7Department of Environmental Science and Technology, University of Maryland, College Park, MD, United States
- 8Fish & Wildlife Research Institute, Florida Fish & Wildlife Conservation Commission, Tallahassee, FL, United States
Introduction: Given the different life histories and movement behaviors of diverse species, reconciling conservation measures to benefit all species is a critical concern for landscape conservation planning. Understanding land cover composition and finding multispecies movement routes across heterogeneous landscapes are crucial to maintaining many target species. The primary objectives of this study were to determine the optimal environment in Florida that promotes multispecies connectivity in landscapes increasingly threatened by rapid suburban development and to enhance methods for delineating the state’s ecological networks.
Methods: Potential functional connectivity of the focal species with statewide distributions and are considered priorities because of the historical and current threats to their population viability, such as the Florida black bear (Ursus americanus floridanus), Florida panther (Puma concolor coryi), eastern indigo snake (Drymarchon couperi), and southern fox squirrel (Sciurus niger niger), were modeled using Linkage Mapper, Omniscape, and resistant kernels. We combined quantiles from each method for a single species combination approach to leverage the results from the three models for planning purposes. Subsequently, we integrated the results into a novel multiple species, multi-model connectivity mapping approach. Following the corridor analysis, a comparison was made between multispecies connectivity maps, current managed conservation lands, and the main priority areas for the Florida Wildlife Corridor, a previously developed planning network of natural hubs and corridors. Finally, we used the spatial prioritization software Zonation to identify areas of conservation priority, while also illustrating the impacts of infrastructure (built infrastructure, roadways, mining, and future development 2040 and 2070 projections) and threats from human activity (landscape fragmentation, recreation, pollution, contamination, and clean-up sites).
Results: The study identified priority areas for all four species, with a particular focus on areas not currently protected. The connectivity models showed significant overlap with current managed conservation lands and the main priority areas for the Florida Wildlife Corridor. Pinchpoint areas or bottlenecks were identified as needing fine-scale incorporation into spatial planning. Using the spatial prioritization software Zonation, we identified areas of conservation priority and illustrated the impacts of infrastructure and threats.
Discussion: The results indicate that species-relevant connectivity models incorporating a group of focal species with both complementary and opposing habitat requirements can better inform biodiversity conservation and landscape design decisions. This multi-model approach provides a robust framework for identifying and prioritizing areas for conservation, particularly in landscapes facing rapid suburban development. Integrating multispecies connectivity models into conservation planning can enhance the effectiveness of ecological networks and contribute to the long-term viability of diverse species in Florida.
Introduction
In the context of rapid environmental change, the presence and condition of habitat in remaining conservation areas, their connectivity, and their ability to withstand disturbances and human impacts are all critical for preserving biodiversity and facilitating movement of multiple species (Haddad et al., 2015). In a mosaic of human-altered landscapes, complex impediments to species’ movement make protecting biological patterns (habitats, land cover) and processes (movement, gene flow) across large landscapes a priority for conservation action (Donald and Evans, 2006; Baldwin et al., 2012; Cushman and Landguth, 2012; Anderson et al., 2015; Cox et al., 2017; Carlier and Moran, 2019; Correa Ayram et al., 2019). Simulations of landscape connectivity for multiple species in large mixed-use landscapes, where fragmentation threatens movements and gene flow, have shown the need to establish protected corridors between suitable habitat sections as anthropogenic activities degrade and develop land in rapidly urbanizing areas (Brodie et al., 2015; Bauder et al., 2021; DeMatteo et al., 2023).
Due to differences in habitat suitability, dispersal distances and home range, and sensitivity to human activities, conservation plans to enhance connectivity for one species may not be effective for another (Brodie et al., 2015; Wang et al., 2018). Large, wide-ranging animals (commonly referred to as “flagships” or “umbrella species”) (Breckheimer et al., 2014; Wang et al., 2018; Shi et al., 2019; Shen et al., 2020) with large area requirements are extensively studied in connectivity research because it is believed that they provide a connectivity umbrella for other species. However, one wide-ranging animal may not provide a connectivity umbrella for other large-ranging species (Cushman and Landguth, 2012), and furthermore, habitat generalists may not serve as an umbrella for habitat specialists (Beier et al., 2011, 2008). If the principal objective were to maintain ideal corridors for all species, neglecting to consider these cross-taxon variations may undermine the efficacy of corridors and incur additional financial expenses (Dilkina et al., 2017).
To better understand the distribution and habitat connectivity of numerous species, researchers and conservation planners are examining connectivity, corridors, bottleneck and impedance zones that allow or hinder movement between diverse habitats using several spatial tools based on circuit theory including Omniscape (Tessier et al., 2020; Landau et al., 2021), Linkage Mapper (McRae and Kavanagh, 2011), and resistant kernels (Compton et al., 2007; Zeller et al., 2018). Connectivity model results can vary for different modeling methods, with outputs for designing corridors varying due to the underlying methodologies. In order to maintain population stability and mobility in dynamic rapidly urbanizing environments, it is possible to map multi-species connectivity by overlapping mapping results from a single model such as least-cost pathways or maximum current flow (Marrotte et al., 2017; Sahraoui et al., 2017; Liu et al., 2018; Ashrafzadeh et al., 2020; Lines et al., 2021; Riggio et al., 2022; Spontak and Hoctor, 2017; Santini et al., 2016). Few attempts have been made to incorporate multiple models into a single, comprehensive framework that can leverage the strengths of different methods (Gallo et al., 2020; Jennings et al., 2020a, 2020b).
Besides modeling habitat suitability and connectivity, environmental stressors should be considered when prioritizing areas for conservation planning (Moilanen and Arponen, 2011). Existing connectivity corridor design methods often fail to prioritize spatial conservation and often fail to consider non-ecological data, such as crucial infrastructure or threat elements that influence land-based acquisitions (state/federal/local government and private conservation actors) to procure lands for corridor conservation, such as nearby pollution features or other human pressures. The creation of operational models and decision support tools, or simplified conceptualizations of processes for implementing conservation action in priority conservation areas, is indispensable for directing conservation planning efforts when allocating limited conservation funds (Knight et al., 2006; Knight et al., 2009; Vizek and Nielsen-Pincus, 2017; Offer, 2020; Sparks, 2021).
Functional landscapes are a global issue, and are especially important in Florida, a rapidly developing state within a region designated as a biodiversity hotspot (Noss et al., 2015). Due to rapid urban development and increasing human population, the natural habitat of many species has been compromised (Zhu et al., 2015; Zhu et al., 2015; Rodgers and Pienaar, 2018; Bauder et al., 2021; Davis et al., 2021). Florida has experienced considerable landscape transformation due to urbanization and changes in land use, the latter of which is primarily attributable to the rapid immigration of new residents (Kautz et al., 2007; Reece et al., 2013; Carr and Zwick, 2016; Volk et al., 2017). Previous land use/land cover change research has shown that Florida’s natural and semi-natural vegetation base declined between 73,063 and 93,938 ha annually between 1985–1989 and 2003, suggesting a true intensification of the central portion of the Florida peninsula (Kautz et al., 2007). The Florida Ecological Greenways Network (FEGN) and Florida Wildlife Corridor (FLWC) (The FEGN is the modeling process and corridor planning tool that serves as the foundation for the legally defined FLWC) use ecological geodesign and spatial prioritization to create a network of natural areas, working lands, and habitat corridors for priority species to protect and preserve the state’s biodiversity. The FEGN modeling process includes Florida panther and Florida black bear priority areas and connectivity, other fragmentation sensitive or landscape dependent species habitat models, habitat for additional rare or focal species, landscape matrix and underrepresented natural communities, priority wetlands and floodplains, priority natural areas, the existing habitat network, biodiversity, critical lands and waters, and multiple landscape connectivity models (major river, coastal-to-inland, xeric) (Hoctor and Volk, 2021a).
The establishment of an extensive network of conservation lands can be attributed to conservation programs administered at federal, state, and local levels that now encompasses 31% of the state’s total area (Boughton et al., 2019). In 2021, the FLWC corridor design was officially recognized by Florida state law, and beginning in 2024, there would be recurrent financing for the purchase of conserved property (Brodeur, 2021; Hutson, 2024). In reports detailing the FLWC’s strategic prioritization for incorporation with the Department of Environmental Protection’s Division of State Lands (DSL), Hoctor and Volk (2021a) show that corridor design is constantly evolving through iterative processes, including the latest methodological data layers and science, as informed by a network of statewide governance and environmental management stakeholders.
Improving decision support tool science and techniques is crucial for communicating with land protection staff, other conservation agencies and NGOs, and other governmental officials. We wanted to investigate new approaches and tactics for boosting priority species integration into the wildlife corridor beyond the current FLWC’s wildlife and environmental connectivity layers and show methods for further incorporating threats and infrastructure into the modeling framework. The species chosen for these analyses were selected through a collaborative stakeholder process based on available data on distribution, occupied patches, and previously developed habitat suitability models, as well as priority for conservation due to perceived losses in population in the past decade and urgency for conservation and recovery. Statewide significance constituted one of the factors associated with inclusion in the study, whereas species with limited ranges are better analyzed at a county or subregional scale. Four representative vulnerable species with different landscape mobility, yet with a statewide distribution, were chosen: Florida black bear (Ursus americanus floridanus), Florida panther (Puma concolor coryi), eastern indigo snake (Drymarchon couperi) and southern fox squirrel (Sciurus niger niger) (see Supplemental Information 1 for background on these species including home ranges and any information on dispersal). Our goals were to: a) assess and compare connectivity patterns among species, b) merge different connectivity modeling results to identify areas of commonality between modeling approaches for each species, and c) examine integrated connectivity models of the four species combined. These approaches merge a multi-model connectivity procedure into map results for different species to integrate new methodologies for assessing strategic connectivity priorities across the state.
After conducting the corridor analysis, multispecies connectivity maps were compared to the currently managed conservation lands and the principal priority areas of the FLWC to illustrate any areas of overlapping connectivity. Finally, we further illustrated potential threats and infrastructure development using the spatial prioritization software Zonation, while also illustrating the effects of overall landscape integrity, human activities (recreation, pollution, contamination, pesticides, and clean-up sites) and infrastructure (built infrastructure, roadways, mining, and future development projections for 2040 and 2070) on ecological connectivity planning. While various iterations of the FLWC do remove infrastructure and development effects from the strategic prioritization, this study investigates potential ways to include rankings of infrastructure and future development threats into visualizations and potential for higher prioritization status and higher precision for strategic areas within multispecies corridor designs.
Methods
Study area and core habitat areas
The state of Florida comprises an extensive land area of 151,900 km2, which showcases a diverse array of 81 natural communities (Florida Natural Areas Inventory, 2010). Florida exhibits humid and subhumid subtropical and tropical climates as its primary climatic conditions. The state’s topography primarily consists of low terrain, with elevations not surpassing 100 meters (Boughton et al., 2019). Formally designated a biodiversity hotspot, the area is situated within the broader North American Coastal Plain, which extends throughout the southeastern region of the continent (Noss et al., 2015). There are 3038 vascular plants and 4,368 species of fauna in Florida, in addition to 269 endemic animal species (NatureServe, 2021). There are numerous species protected under state (44) and/or federal (89) law in Florida (Boughton et al., 2019). Many of Florida’s diverse range of species and habitats are threatened by climate change, sea level rise and land cover change (Hoctor et al., 2000; Reece et al., 2013; Romañach et al., 2020). This area is well-suited for testing corridors that support numerous species because of the patchwork of protected areas and the present plans for the Florida Wildlife Corridor with many vulnerable species still occupying the private land outside of the protected areas (Hoctor et al., 2007; Hoctor and Volk, 2021b, 2000).
Our study focused on large landscape connectivity across a large land mosaic comprised of conservation, agriculture, mining, rural, urban and suburban land uses. Natural vegetation has been fragmented and altered from urbanization (Lopez et al., 2004; Harveson et al., 2007; Carr and Zwick, 2016; Davis et al., 2021), large-scale agricultural uses like citrus and plantation forestry (Repenning and Labisky, 1985; Means et al., 1996; Fox et al., 2007; Andreu et al., 2008), cattle grazing (Sonnier et al., 2023), phosphate mining (Caple, 2017; Duan et al., 2021; Khare et al., 2021), as well as oil and gas operations (Baynard et al., 2014). Numerous factors negatively affect the health of the bioregion and its species, including long-term suppression of fire (Varner et al., 2005; Lindemann, 2009), deforestation (Enge and Marion, 1986), pesticides (Facemire et al., 1995; Marburger et al., 2002; Tavalieri et al., 2020; De María et al., 2021), air pollution (Edwards et al., 2019), invasions of non-native flora and fauna (Engeman et al., 2019; Assis et al., 2020; Conyers and Roy, 2021; Hardin, 2007; Julian et al., 2012; Hiatt et al., 2019; Sinclair et al., 2020), groundwater contamination (Outman, 2020; Heil and Muni-Morgan, 2021; Li et al., 2022; Vermeylen et al., 2022; Lapointe et al., 2023), sea level rise (Reece and Noss, 2014; Zhu et al., 2015; Zhu et al., 2015; Davis et al., 2021), and fluctuating climatic conditions (Catano et al., 2015; Montero et al., 2018; Abernathy et al., 2019; Zampieri et al., 2020).
Priority areas for focal species
Previously developed core habitat and species occurrences were used for each of the four species as our core priority areas (Supplementary Information 2 - Figure S1). Our goal was to simulate connectivity between core conservation areas that could support potential breeding populations using simulated movement thresholds previously identified in the literature (Table 1).
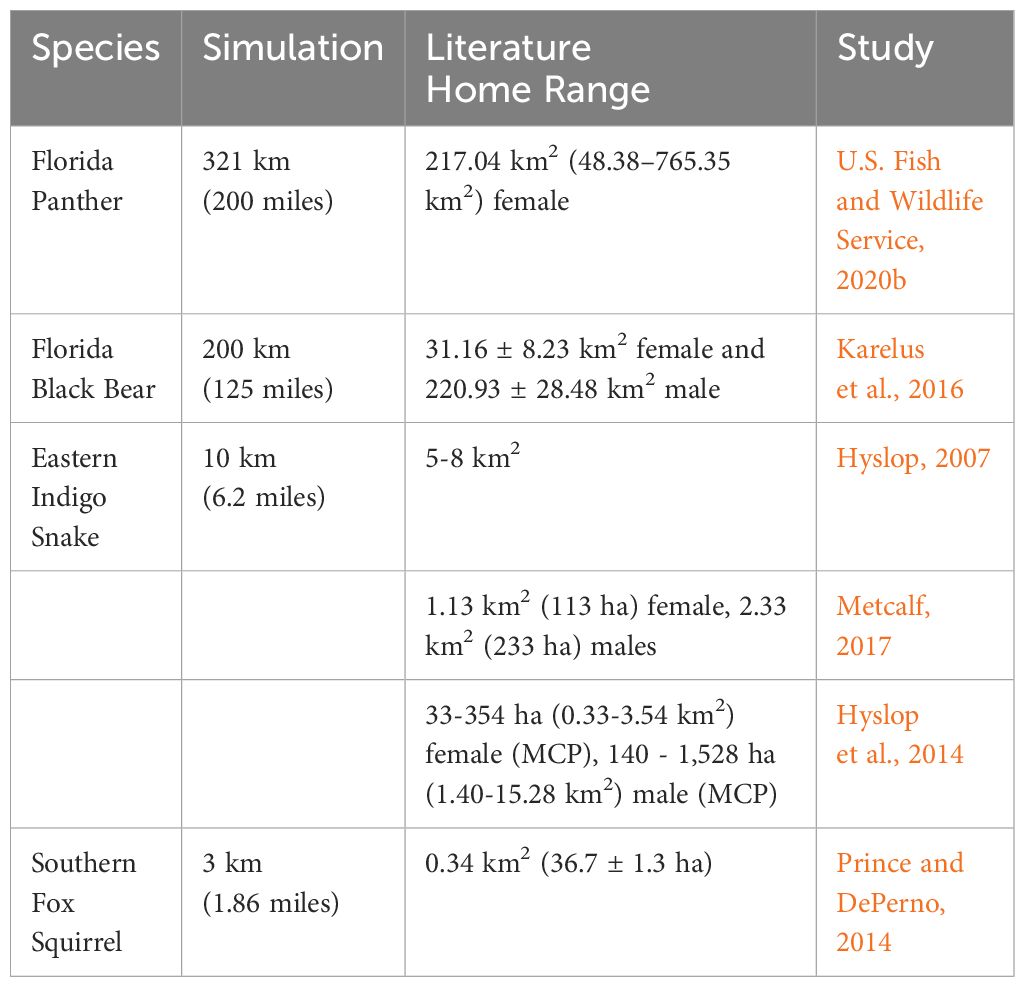
Table 1 Displays the simulated dispersal distance, reported home range from literature, and study reference for the focal species.
To create core habitat patches for Florida Panther, we identified core conservation areas that overlapped with the most suitable areas corresponding to previously developed habitat suitability models (Frakes et al., 2015; Frakes and Knight, 2021). The patches were rasterized using ESRI’s ArcGIS pro-3.3. The Florida Natural Areas Inventory (FNAI) Florida Managed and Conserved Lands (FMLA) layer was rasterized to remove any boundary layers and reduce it to continuous patches. The new FLMA contiguous polygons were clipped to the habitat patches and subset to any patch larger than 228 km2, which was decided based on known home range of females (Table 1). Due to the lack of realistic long-term connectivity and the foreseeable threat of development, two patches—the Withlacoochee State Forest and Goethe State Forest patches—were deleted. In addition, we incorporated expansive contiguous regions that are presently occupied by the Florida panther (Big Cypress, CREW, and OK Slough) but are not currently protected areas.
To create core patches for Florida Black Bear we subset the Florida Fish and Wildlife Conservation Commission’s (FWCC) defined occupied areas from the 2018 Florida black bear range map. We used a minimum area threshold of 202 km2 to subset the analysis to core breeding areas. We aimed to simulate connectivity (200 km) between larger breeding areas to identify the most vital pathways that maintain connectivity between sub-populations.
For indigo snakes, the core populations were provided by FNAI based on a collaboration with the USFWS species status assessment (U.S. Fish and Wildlife Service, 2018; Bauder, 2019).
The southern fox squirrel occurrences were provided by FWCC. The dataset includes all relevant confirmed locations for the fox squirrel, although may not adequately represent the full range of their habitat. We did not alter these layers prior to use in the modeling efforts.
Resistance surfaces
For Florida panthers, we used the previously developed random forest model (Frakes and Knight, 2021). For Florida black bear, an averaged, ensemble modeling strategy was used (Poor et al., 2020). They applied maximum entropy and Mahalanobis distance to model black bear habitat suitability in Florida. The eastern indigo snake (U.S. Fish and Wildlife Service, 2018; Bauder, 2019) and southern fox squirrel (FWC, 2017) habitat suitability models were both previously developed using MaxEnt.
When designing corridors for long-distance dispersal by mobile animals, previous research on resistance surfaces has suggested employing a negative exponential function to convert habitat suitability into resistance (Trainor et al., 2013; Mateo-Sánchez et al., 2015; Keeley et al., 2016). This has been presented as an alternative to the linear inverse of habitat suitability. We thought that the linear inverse of habitat suitability would be suitable for the fox squirrel whose range was simulated upwards of 3 km for dispersal, and therefore not long distance. For species with wider range and more general dispersal we chose negative exponential scaling. For Florida panther and Florida black bear we used a scaling value of -8, and for eastern indigo snake a scaled value of -2. The selection of these values was determined based on the most rational estimation of long-distance dispersal for the given species. Long-distance dispersal or pre-dispersal scouting may require animals to traverse moderately suitable settings, where resistance increases only modestly as suitability decreases from its maximum value but increases dramatically at lower suitability values. The exponential scaling was simulated with values between -2 and -8 to determine the realistic scenarios, using the following equation, where h represents the raster values and R represents the scaling value.
Prior to running the connectivity models the values were transformed linearly between 1–100.
Values closer to one hundred indicate the most resistant conditions, whereas values closer to one indicate the most suitable or least resistant conditions.
Least cost corridors
Linkage Mapper 3.1 was used to map least cost corridors using tools in the ESRI ArcGIS Desktop 10.8.2 toolbox (McRae and Kavanagh, 2011). The following parameters were applied based on literature-based home range or dispersal information (Table 1). Florida panther, a Euclidean distance of 321km to reflect dispersal, and a corridor truncation of 321km; Florida black bear, a Euclidean distance of 200km to reflect the potential dispersal on the landscape, and a corridor truncation of 200km; eastern indigo snake, we used core populations with a bounding circle and a 5m buffer, a Euclidean distance of 20km, and truncated corridors to 20km; southern fox squirrel, we used occurrences, a Euclidean distance of 3km, and a truncation of corridors to 8km. The Linkage Mapper outputs were reclassified using 1-10 quantiles to represent percentages.
Omniscape
Omniscape was implemented in Julia 1.8.5 (McRae et al., 2016; Landau et al., 2021). Within Omniscape we used a moving window radius of 100 pixels, and a block size of 21 pixels due to the statewide scale of our study, and yet need to capture finer scale features. For our analyses we used the following results from Omniscape: 1) cumulative current flow, which is a sum of the current maps from all iterations of the moving window analysis and 2) normalized flow which is derived by dividing the current flow by the potential flow, and gives out puts for impeded (< -0.5 SD from mean), diffuse (-0.5 to 1 SD from mean), channelized (1 to 2 SD from mean) and intensified flow (>2.0 SD from mean) (TNC, 2023). The cumulative current flow outputs were reclassified using 1-10 quantiles.
Resistant kernels
The resistance surface was modeled in UNICOR v2.0 (UNIversal CORridor and network simulation model) in conjunction with the least-cost resistant kernel parameterization (Landguth et al., 2012). Using Spatially Balanced Points, point arrays were constructed across the landscape for Florida panther (n=500), Florida black bear (n=3000), indigo snake (n=500), and fox squirrel (n=1000). The resistant kernel outputs were reclassified using 1-10 quantiles.
Comparing models
We computed correlations between the combined models to compare the effectiveness of individual species in predicting landscape connectivity and habitat use for the other species in this study. We used Band Collection Statistics in ESRI ArcGIS pro 3.3 to compute the correlation between layers, using the output values of the correlation coefficients, which represent the relationship between two datasets.
Overlapping priorities
Using the final integrated combined connectivity model for the four priority species, we evaluated the ability of the current protected area portfolio to preserve connectivity for these species as well as the Florida Wildlife Corridor’s ability to meet the specific needs of these species. To conduct a fine-scale case analysis, a specific subset that had been identified as a conservation void outside of the corridor plans but had high levels of prospective multi-species corridor connectivity was emphasized.
Assessing pinchpoints
A multi species corridor subset was in southern Alachua County and was evaluated for conservation. Our research used two ways to determine population bottlenecks or limited flow in the landscape or corridor. LinkageMapper uses Circuitscape to find pinch spots in the corridor. Similar to Omniscape, normalized current flow statistics may be partitioned to detect impeded, intensified, and channelized flow and visualize geographical conservation priorities. Our goal was to leverage both results for a visualization of each species-specific impeded and channelized flow according to the different outputs. To accomplish this objective, we reclassified the outcomes as quantiles. For LinkageMapper Pinch points the upper 20–50 percent was assigned a value of 1, and the upper 10–20 percent was assigned a value of 2. The Omniscape results were reclassified as follows: intensified as a 1 and channelized as a 2. Subsequently, the Linkage Mapper Pinchpoints and Omniscape intensified and channelized flow were combined using a scale of 1-4 to emphasize regions of overlap between the two outcomes. In our results, we also incorporated the Omniscape impeded category to emphasize regions that had already been impeded for each specie based on the underlying resistance surface.
Spatial conservation prioritization
In order to identify the priority areas for the conservation of corridor connectivity in Florida, we used Zonation v5 spatial conservation prioritization software (Moilanen et al., 2005; Moilanen, 2007; Lehtomäki et al., 2016). We compiled numerous geospatial data related to infrastructure (development, mines, transportation, utilities, recreation) and threats to environmental quality (pollution sites, cleanup sites and water pollution) as well as a landscape integrity layer from the Critical Lands and Waters Identification Project (Oetting et al., 2016). We had a panel of seven experts (3 University of Florida, 1 University of Maryland, 1 USFWS, 1 FWCC, and 1 private consultant) rank the infrastructure against the connectivity map for severity of threat to the corridor (see S3 for detailed information about data layers). Experts were advised to rank the infrastructure against the connectivity layer, which was weighted with a 1, on a scale of 0-2.
The output of Zonation is a landscape-wide conservation priority classification based on the complementarity of various input geospatial layers, determining optimal site selection for multiple variables (Moilanen et al., 2011, 2005). The priority ranking is determined by continuously eliminating the grid cell or planning unit with the smallest aggregate loss of conservation value, and accounts for the species distributions, assigned weights and connectivity of features. In the case of infrastructure, this permits the elimination of extremely poor landscape areas prior to further iterative cell removal (Moilanen, 2007) or a region threatened by future development could be negatively weighted to preferentially select other areas (Moilanen and Arponen, 2011). For all features, the exponent of the power function was set to z = 0.25. The allocation of weights to features in Zonation has a significant impact on the distribution of importance among the various features in the prioritization solution. Furthermore, negative values may be assigned to weights in certain cases, such as when numerous opportunity costs are incorporated into the analysis, such as for infrastructure or land costs, as shown by Di Minin et al. (2013) and Moilanen et al. (2011). To avoid unequal weights based on the corridor connectivity features, we assigned the same aggregate weight to each of the corridor models (Wj =1). In the infrastructure overlays, the pollution features were included as negatively weighted features (Wj = -1). The landscape integrity layer was included as a condition. These features enable the weighting and ranking of areas that are most vulnerable to development, as well as areas that are most important for maintaining biodiversity.
Prioritization tools are also used to identify areas based on their ecological value and can perform scenario-based analysis. We developed several scenarios: i) baseline corridor connectivity, a scenario where the corridors and current 2019 baseline infrastructure were included in the analysis, and ii) future development scenarios – Florida 2040 and Florida 2070 development including urban sprawl and conservation projection alternatives.
Results
Integrated combined models
The landscape connectivity models for Linkage Mapper (Supplementary Information 2 - Figure S2), Omniscape (Supplementary Information 2 - Figure S3), and resistant kernel (Supplementary Information 2 - Figure S4) were output for each species. Models for a combined three model species-specific connectivity output were also calculated (Supplementary Information 2 - Figure S5). Finally, we integrated the multi-model combinations for the species together for an integrated combined connectivity approach: Florida panther, Florida black bear, eastern indigo snake and southern fox squirrel (Figure 1).
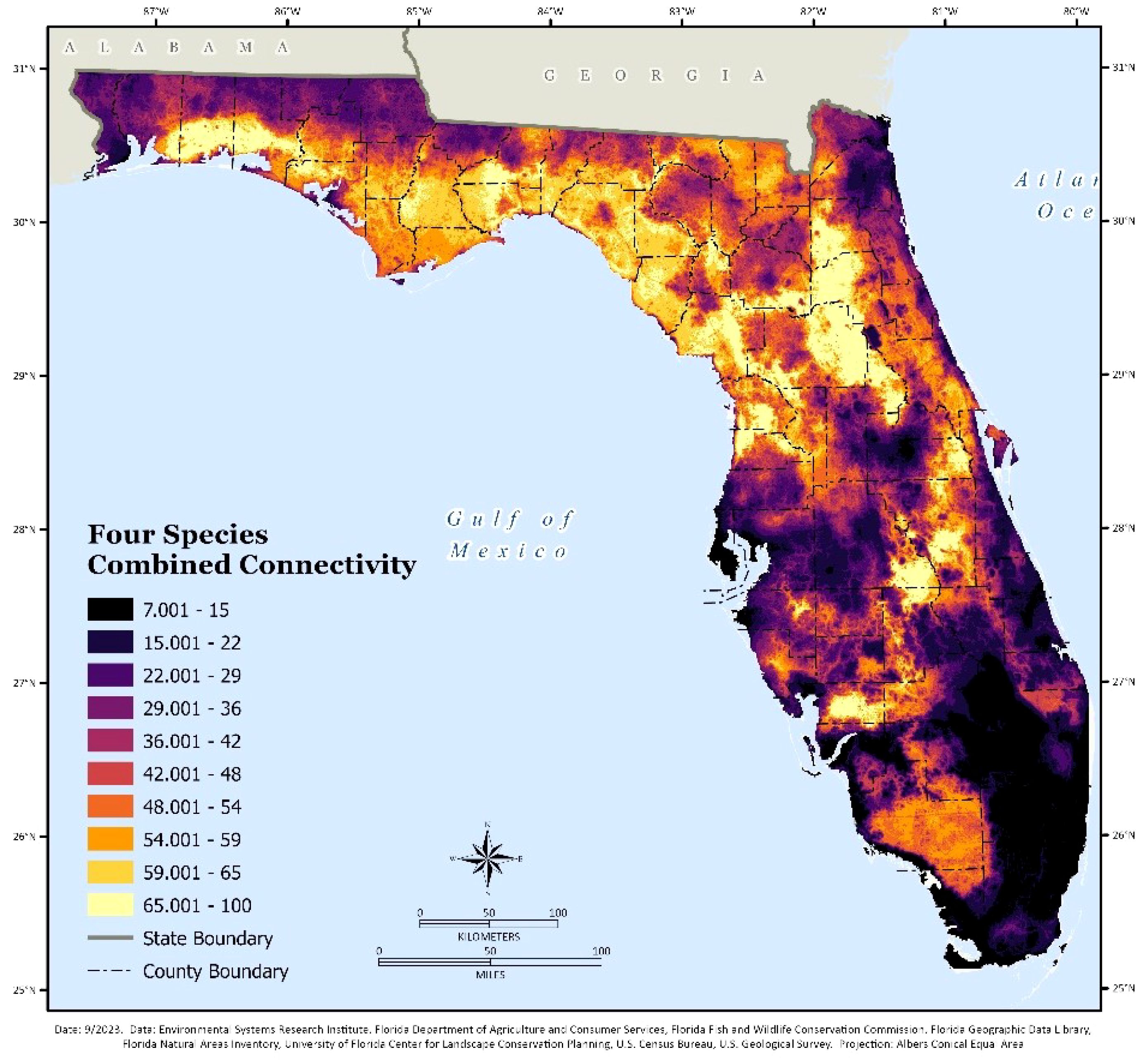
Figure 1 Integrated combined connectivity for the four focal species: Florida panther, Florida black bear, eastern indigo snake and southern fox squirrel.
Correlation coefficients
The Florida panther and Florida black bear showed significant levels of overlap (correlation 0.77) in their habitat preferences, with more broad levels of suitable connectivity throughout the landscape. We also found some correlation between eastern indigo snake and southern fox squirrel connectivity (correlation 0.375) (Table 2), Florida black bear and eastern indigo snake (correlation 0.312), Florida panther and southern Fox Squirrel (correlation 0.241). There was little overlap for connectivity models between Florida panther and eastern indigo snake (correlation 0.078) or Florida black bear and southern fox squirrel (correlation 0.11).
Conservation implications
Our results for the top 10 and 20 percent of corridor connectivity were overlaid with the Florida conservation lands and the Florida Wildlife corridor blueprint for priorities 1-3 (Figure 2).
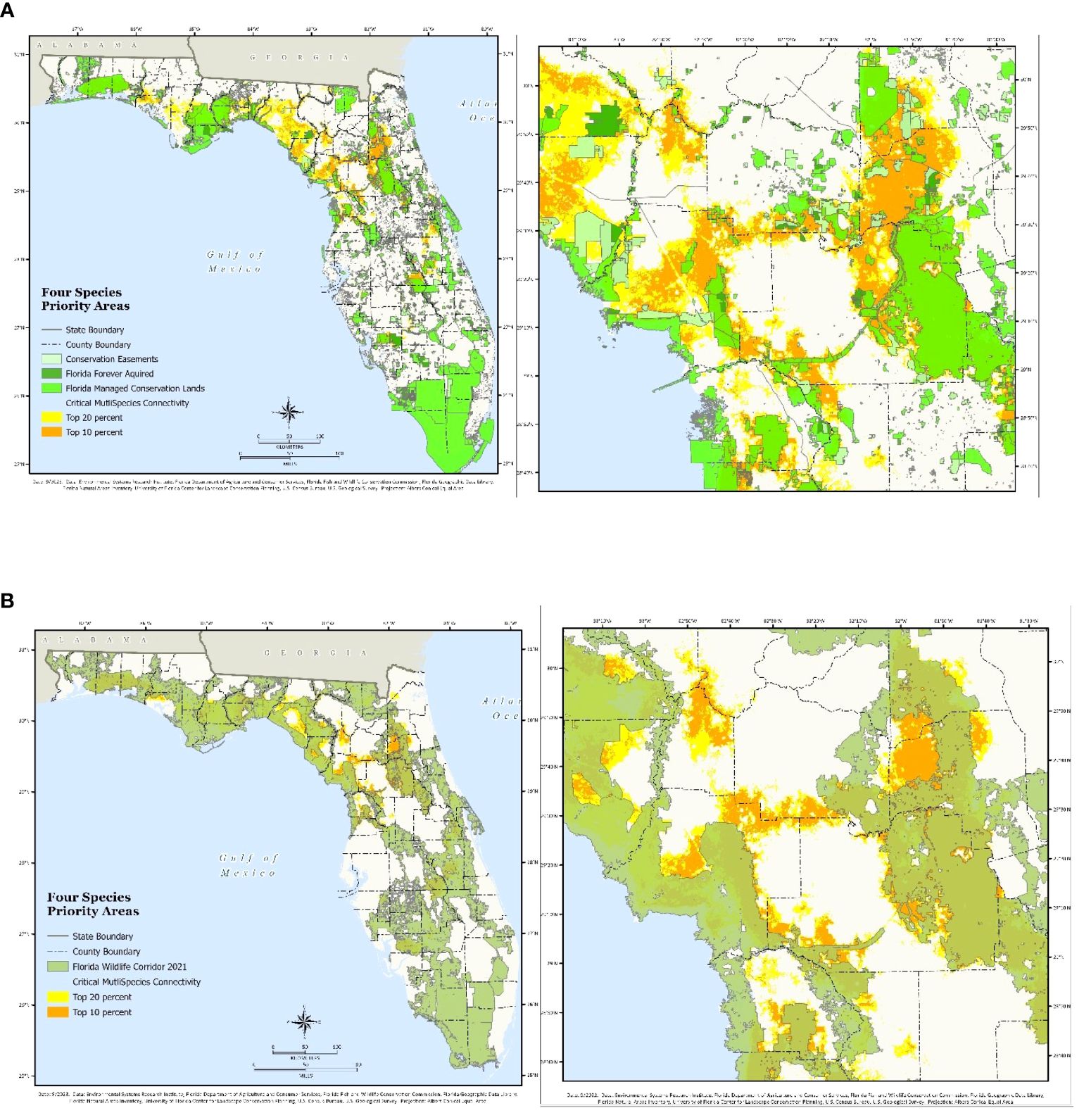
Figure 2 The top 10 and 20 percent of the four species connectivity modeling results with overlays for statewide (left) and southern Alachua County and O2O corridor (right) for (A) protected Florida conservation lands and (B) Florida Wildlife Corridor plans.
Bottleneck and pinchpoint mapping
Our goal was to illustrate the subset region characterized by a significant but unprotected prospective multispecies connection corridor and show each target species’ mobility bottlenecks or constricted movement (Figure 3). The Florida panther and Florida black bear had more constricted bottlenecks and flow obstruction in southern Alachua County, while the southern fox squirrel and eastern indigo snake had less, likely due to their scarce occurrence records and the pinchpoints being more defined between smaller suitable patch occurrences. Although with fewer corridors and potential for movement, the eastern indigo snake was modeled as having the most restricted movement across the landscape; therefore, bottleneck areas are located within those restricted areas.
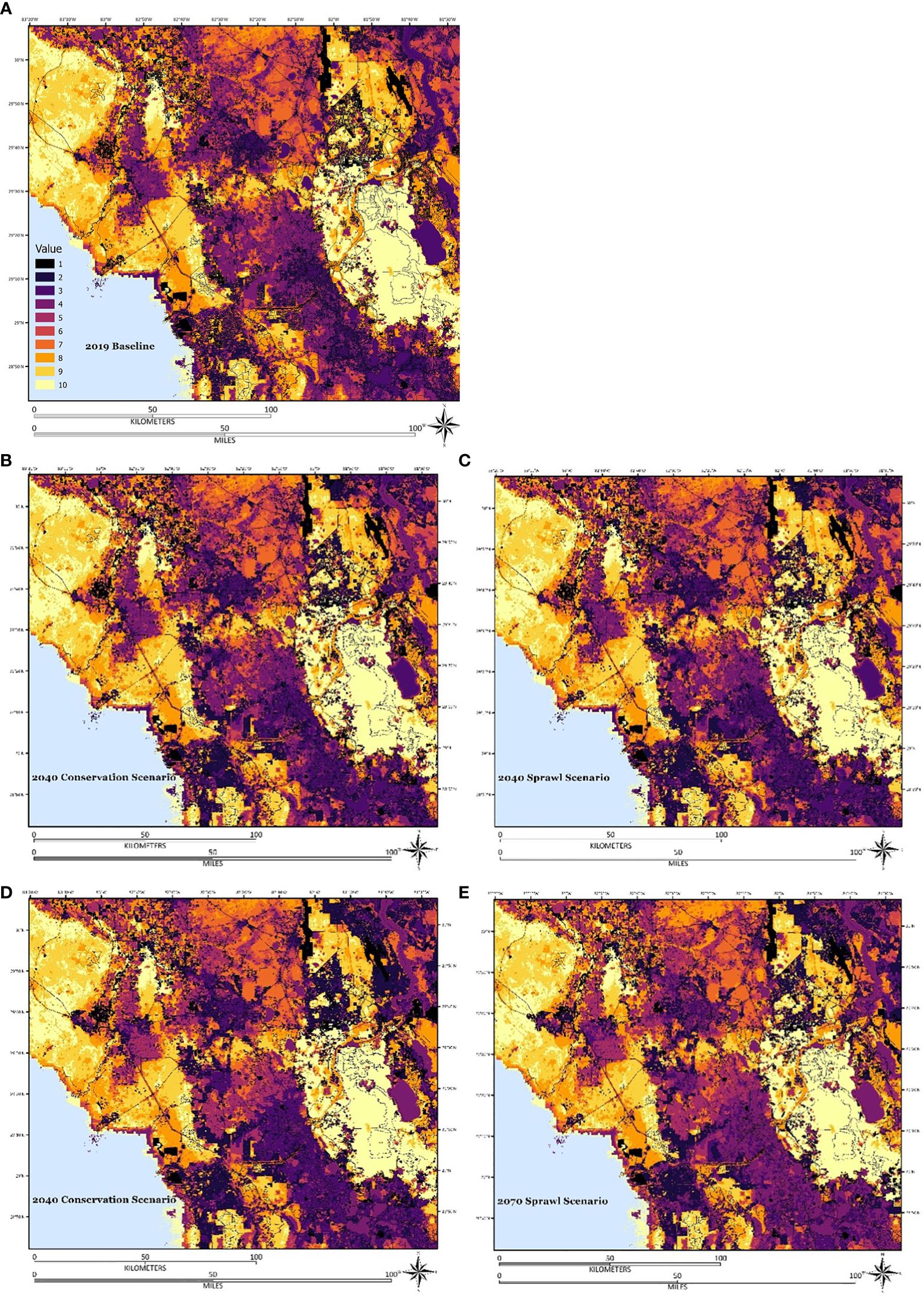
Figure 3 Spatial prioritization of future development, infrastructure, and threats for (A) 2019 development baseline, (B) 2040 conservation scenario, (C) 2040 sprawl scenario, (D) 2070 conservation scenario, and (E) 2070 sprawl scenario. Values represent areas of highest connectivity (10 yellow) and lowest connectivity (1 black) with the threats, pressures, and landscape integrity factored in.
Spatial conservation prioritization
Our results from including the Zonation rankings further emphasize the existing infrastructure and threats to the landscape (S3). Full statewide maps are available (Supplementary Information - Figure S6), in addition to the study area subset in north central Florida centering on Alachua county (Figure 3).
Discussion
A growing amount of research shows that wildlife corridors should incorporate multi-species connectivity to retain key ecological processes over wide geographic regions (Koen et al., 2014; Brodie et al., 2015; Dilkina et al., 2017; Riggio et al., 2022; DeMatteo et al., 2023). We have identified a common corridor map among multiple species using the most reliable statewide species occurrence information, habitat suitability data, and connectivity modeling. These methods may be used to supplement the existing geospatial data layers in FEGN planning and prioritization to include additional and more specific metrics of connectivity for species of conservation importance to the state.
Combining the results of these various models at the regional scale was a crucial component of our strategy to leverage the results. The various landscape connectivity models enabled us to incorporate the strengths of various methodologies. The Linkage Mapper analysis provided predictions of the most suitable corridors that could be constructed between the patches defined for each species. The resistant kernel analysis provided predictions of biologically meaningful dispersal distances from core habitats. The Omniscape analysis provided predictions across the landscape for a pixel-by-pixel circuit theory-based model for connectivity. Finally, we combined the map quantiles of these three approaches into one combination map for each species to leverage the strengths of the three models. Similar multi-model efforts have been made to apply ensemble modeling to machine learning algorithms (Fox et al., 2017; Dondina et al., 2020), and model averaging (Dormann et al., 2018) to the problem of determining habitat suitability for a particular species, but these techniques have not readily been transferred to the connectivity models currently in use. We sought to combine the models in a way that was simple and straightforward using map quantiles of the results for an equal weighting. The results revealed the most specific and optimal areas for conserving the four focal species. Since our study models connectivity specifically between the core habitat patches or occurrences using species-specific habitat suitability and by adding specific potential dispersal distances, more general landscape connectivity methodologies may reach a different result. The FEGN already uses a large collection of habitat suitability models, thus this research investigated the possibility to combine connectivity-related indicators using an integrated multi-model multi-species connectivity approach.
The modeling framework used in this study should improve upon previous modeling work to connect potential breeding habitats for Florida panthers and black bears to promote functional connectivity and gene flow. We sought to highlight the historical habitat fragmentation and patch isolation on the Florida panther population, including inbreeding and genetic drift (Johnson et al., 2010; Kerk et al., 2019; Saremi et al., 2019), along with the current limited range of the Florida panther population (Hostetler et al., 2013) and the need for further efforts to facilitate population growth and remove the species from the endangered species list (Hedrick and Fredrickson, 2010; Johnson et al., 2010; Gustafson et al., 2019; Kerk et al., 2019; Saremi et al., 2019). Natural dispersal of females may not be a viable strategy for population expansion and translocations of females to establish new populations in central and northern Florida has been suggested (Maehr et al., 2002; Thatcher et al., 2009; Frakes and Knight, 2021). Understanding the prospective interconnectivity among reintroduction regions can provide valuable insights for endeavors aimed at long-term, practical ecological connectivity planning.
Results illustrate the potential for restricted movement of certain species in a specific study area. We found that the Florida panther and Florida black bear had larger levels of restricted movement compared to the southern fox squirrel and eastern indigo snake, likely due to the habitat patch suitability for each species being very different in size. We highlight the need for future research to prioritize specific pinchpoints for priority species to keep small patches connected within large landscape conservation efforts (see Figure 4). The ability to conserve smaller species at a statewide scale would require a finer scale prioritization and may assist in prioritization for adaptive management at the county or multi-county level scale as well. Although few papers have discussed the implications of pinchpoints in the design and prioritization of corridor planning, here we have illustrated pinchpoints based on the combination of two modeling methods. Our results highlight the importance of the Ocala to Osceola (O2O) Wildlife Corridor, which is currently a regional partnership led by the North Florida Land Trust. (https://o2owildlifecorridor.org/), for important habitat and restricted flow for the southern fox squirrel, eastern indigo snake, as well as long term restricted flow for Florida panther and black bear. Movement across land designated for future protection should attempt to safeguard places that may offer diffuse flow for wildlife or areas becoming progressively confined to prevent such channels from being entirely obstructed (Carroll et al., 2012; McRae et al., 2012). The strategic prioritization designs of the current FLWC do not account for pinchpoints, even though research has identified the necessity of identifying and validating pinchpoints. Future research may seek to understand the major pinchpoints throughout the state for these species based on the methods presented in this study and seek to use landscape design principles for effective pinch point prioritization. In addition, future strategic prioritization of the FLWC is intended to better incorporate consideration of pinchpoints and similar bottlenecks to further refine the identification of top priorities for closing functional gaps in protected corridors.
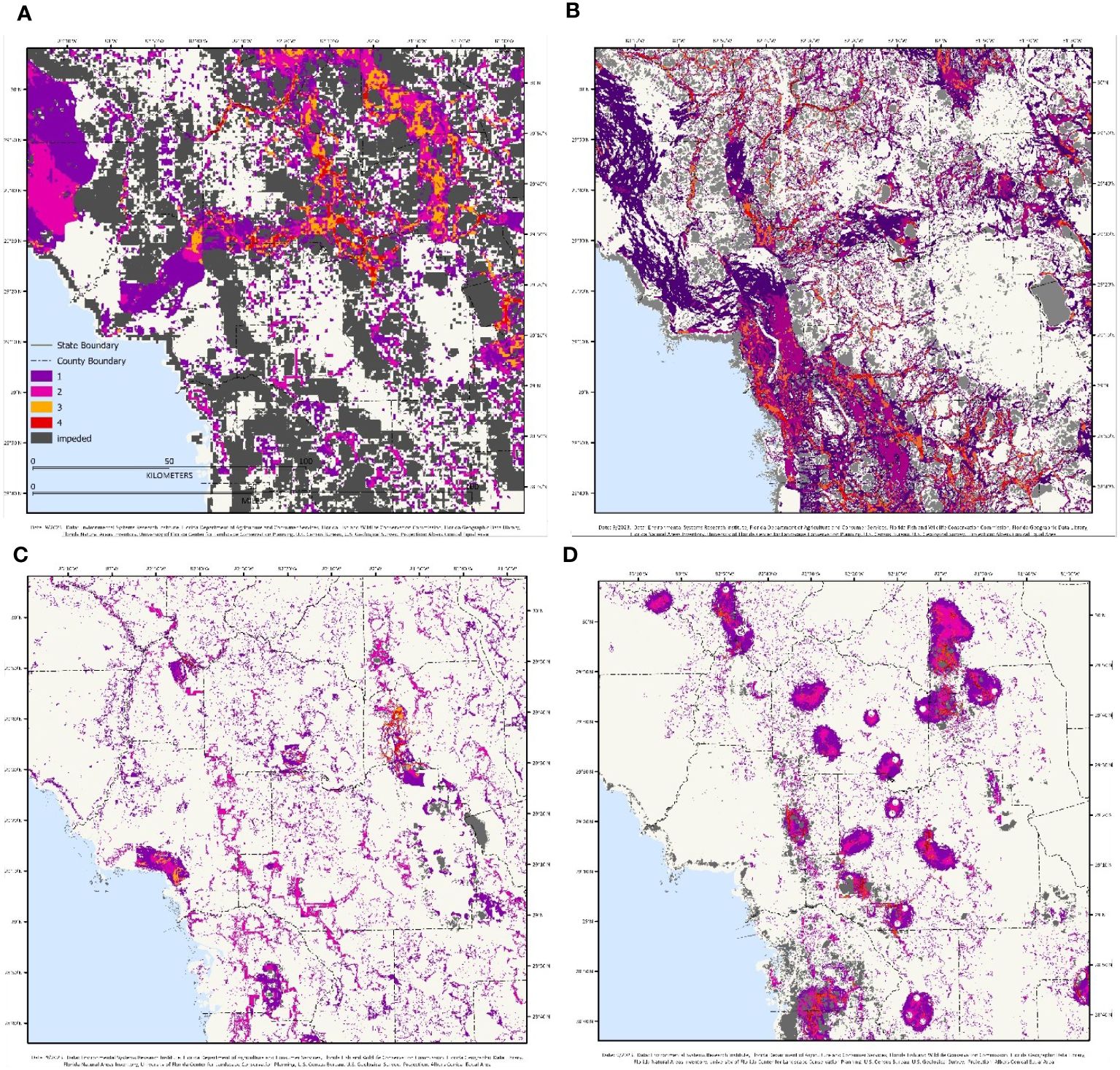
Figure 4 Pinchpoints and bottlenecks have been identified for the following species: (A) Florida panther, (B) Florida black bear, (C) eastern indigo snake, and (D) southern fox squirrel. Maps illustrate impeded flow (grey) as well as the pinchpoints ranked on a scale from 1-4 (with four being the most constricted).
One of our objectives was to assess the effectiveness of multi-species connectivity relative to the FLWC and the Florida conservation lands portfolio. We were able to identify areas shared by all four species that were not protected by the current protected area estate nor the FLWC priorities 1-3, illustrating additional potential priorities for land acquisition. There were large areas of northern Florida that remain unprotected. One of the main areas based on our analysis was Putnam, Marion, and Clay counties, in the O2O wildlife corridor (Figure 2). Other counties that have protection gaps included Alachua, Levy, Gilchrist, Dixie, Taylor, and Lafayette counties. We highlighted this with several maps further investigating this subset for protection with a Zonation approach (Figure 3). The corridor connectivity in our subset study area around southern Alachua County illustrated the potential for identifying and protecting connectivity for the four focal species, while also revealing underlying factors such as infrastructure, landscape integrity, and future development on the area that likely further degrade connectivity due to human pressure and loss of landscape integrity.
For the purposes of planning and prioritizing spatial conservation, additional considerations are made regarding land acquisitions, such as pollution sources and other potential threats. Future designs of the FLWC may be significantly influenced by the implications of large-scale infrastructure, which is why the FLWC is iteratively updated to incorporate threats as land use changes. To assess methods or increasing priority of areas with higher potential threats, additional research is required to ensure all elements of built infrastructure that might impede ecological connectivity are fully considered. Our work shows the potential impact of including more thorough built infrastructure in ecological connectivity planning but needs additional refinement to fully integrate existing and future threats in strategic prioritization. As the results of our Zonation model indicate, we attempted to depict interconnected regions that are presently less impacted by pressures or threats from human activity, highlighting refugia from multiple stressors of human activity.
Major transportation corridors and the built environment were listed by experts as among the greatest threats to the Florida Wildlife Corridor and multi-species habitat connectivity. At present, ten under-construction or proposed major expressways and toll roads of statewide to regional scope are part of the work programs in the Central Florida Expressway Master Plan 2045 and the Florida Transportation Plan 2045, and assessing, avoiding, and fully mitigating the impacts of future highway construction is essential for effective ecological connectivity planning. Road networks in south Florida have disrupted the panther’s habitat (Schwab, 2006), separating the sexes and reducing mobility of females more than males (Schwab and Zandbergen, 2011). Fragmented habitats also result in larger home ranges for animals, such as bears, as they avoid urban areas and major roads (Karelus et al., 2019, Karelus et al., 2016). Lack of conservation and connectivity among bear subpopulations can worsen habitat fragmentation and threaten population stability (Poor et al., 2020). Studies recommend building underpasses to protect Florida black bears from ever increasing road density and vehicle traffic that negatively affect adult survival and are the leading cause of mortality for bears and other large carnivore populations (Hostetler et al., 2009). Pressure and changes in land use patterns are also anticipated to occur during the horizon of our models, illustrating how conservation related scenarios of development will influence future land-use patterns in 2040 and 2070. This information could help safeguard statewide and regional connectivity of the populations of all four focal species and maintain genetically diverse populations through immigration and emigration if it is used to ensure and protect functional ecological connectivity before projected habitat loss to development occurs.
Limitations
Our study served as an example of a potential workflow to integrate multiple species and multiple models into landscape conservation planning. There are more species of importance that we have yet to include in this work that will likely influence future results. We chose two wide-ranging carnivores (Florida Black Bear, and Florida Panther), one large snake and one small mammal with regional landscape to landscape scale habitat needs and dispersal capabilities. Our goal moving forward is to integrate additional priority species into this workflow including wood stork (Mycteria americana), flatwoods salamander (Ambystoma cingulatum), swallow-tailed kite (Elanoides forficatus), gopher tortoise (Gopherus polyphemus), red-cockaded woodpecker (Leuconotopicus borealis), short tailed hawk (Buteo brachyurus), Florida scrub jay (Aphelocoma coerulescens) and a revised layer for xeric connectivity related species. Florida is home to myriad species that, despite an abundance of data, are not necessarily considered priorities by the state due to their conservation status as common or least concern species where maintaining connectivity would be considered a low priority compared to more urgent priorities. The challenge is to adequately rank species priorities in the final FEGN data products. The FEGN now includes 12 species’ habitat suitability maps, where the goal is to continue to integrate additional connectivity related data layers of more species to further improve identification of priority areas of habitat connectivity.
Future research
Using multiple models, we would hope to increase the potential for model validity, although without on-the-ground validation the benefits of multi-model efforts compared to single model efforts are currently unknown. Future work is needed to validate these models with on-the- ground data, such as a separate data collection effort, e.g., road mortality data (Iverson et al., 2024). Particularly for at-risk species and natural communities that are frequently data deficient due to the absence of formal data collection efforts, be it independently collected, or even through citizen science programs, validating multispecies models would be a challenging endeavor. Frequently, the data accessible via portals like the Global Biodiversity Information Facility (GBIF) are identical to the data accessible via NatureServe and the Florida Natural Areas Inventory programs; thus, they lacked independence from the underlying data and models used in our study. Furthermore, these datasets are frequently skewed toward common species within the state or comprise citizen science data that may not meet the criteria for research quality for priority at-risk species. Independent validation for single species models has been explored in separate research for Florida black bear using GPS collaring data, focusing on the use of various resistance surfaces making a large difference in effectiveness of the models (Poor et al., In Review)1. There are road mortality data available for Florida black bear and Florida panther, although for the Florida panther, the statewide scale of our models does not match the location of mortality data given that current movement for panthers is concentrated primarily in south Florida, with only occasional documented movements further north. Finer scale validation efforts would be necessary to produce the type of robust validation of multiple species crucial to corridor linkages identified in this study.
Future research may seek to integrate a dynamic connectivity approach that also includes future scenarios in the underlying habitat suitability models to see how connectivity shifts, including climate-wise connectivity for climate adaptation planning in addition to future development threats (Jennings et al., 2020a). A wide array of species and habitats are imperiled by climate change, sea level rise, and land cover transformation in Florida, where further research is needed to identify spatial priorities using long term datasets. Additionally, future extensions of this research may seek to integrate spatially explicit population modeling to better understand how the modeled connectivity planning would currently enable or hinder population growth for certain species within these new land mosaics of planned development.
Conclusions
Conservation techniques must adapt to Florida’s suburban development, which is negatively altering species ranges and ecological dynamics, and find ways to preserve functional connectivity in quickly changing landscapes. Our study simulated movement for four focal statewide species: the Florida panther, Florida black bear, eastern indigo snake, and southern fox squirrel. Our research aimed to use the results of different landscape connectivity models based on circuit theory and least-cost corridors to assess possible conservation areas for the protection of connectivity and to identify priorities for the FEGN/FLWC. The models utilized resistant kernels, least cost corridors, and Omniscape modeling to simulate the movement throughout Florida. Our research shows that conservation strategies that focus on limited, single species applications may prove inadequate for all species on account of variances in habitat suitability, dispersal distances, home ranges, and susceptibility to human activities, necessitating a multispecies approach. Our research also assessed the effectiveness of the existing protected area extent in maintaining connectivity for these species, as well as the capability of the FLWC to address their requirements and found a few significant gaps that should be considered in future protection strategies. Additionally, we investigated a subset of our study area in north central Florida, centered on Alachua County, of constricted species movement through the analysis of bottlenecks and impedance zones for a subset of the study area, finding that the larger mammals (Florida panther and Florida black bear) showed higher levels of constricted and impeded flow in larger areas, with smaller animals (eastern indigo snake and southern fox squirrel) having smaller pinchpoints between smaller occurrence patches, highlighting the need for a finer scale prioritization. Furthermore, we incorporated expert opinion rankings of infrastructure and threats using the spatial conservation prioritization software Zonation. This analysis developed a landscape integrity-based assessment of connectivity modeling using a baseline for infrastructure development in 2019, as well as alternative 2040/2070 future development scenarios. For prospective long-term protection of a subset of the southern Alachua County connection, the results of the analysis demonstrated loss of landscape integrity at the regional scale, as well as gaps in connectivity for both existing conservation status lands and the FLWC, while still highlighting the potential for more effective multi-species conservation in the region.
Data availability statement
The raw data supporting the conclusions of this article will be made available by the authors, without undue reservation.
Author contributions
EB: Conceptualization, Data curation, Formal analysis, Investigation, Methodology, Validation, Writing – original draft, Writing – review & editing. JO: Data curation, Investigation, Project administration, Writing – review & editing. RN: Conceptualization, Supervision, Writing – review & editing. MO’B: Data curation, Investigation, Writing – review & editing. RF: Data curation, Investigation, Writing – review & editing. DS: Investigation, Methodology, Writing – review & editing. SL: Writing – review & editing. JM: Data curation, Writing – review & editing. BS: Data curation, Writing – review & editing. EP: Data curation, Writing – review & editing. TH: Writing – review & editing.
Funding
The author(s) declare financial support was received for the research, authorship, and/or publication of this article. The funding for this work was provided by the Florida State Legislature and the Florida Department of Environmental Protection Division of State Lands Contract PL513, Task Assignment #205. UF project P0321753 subcontract R000003262.
Acknowledgments
We would like to thank the members of the Florida Ecological Greenways Network Technical Advisory Group as well at the Indigo Snake Advisory Subgroup for their review and recommendations of the species connectivity modeling used in this article.
Conflict of interest
Author RN was employed by Conservation Science, Inc.
The authors declare that the research was conducted in the absence of any commercial or financial relationships that could be construed as a potential conflict of interest.
Publisher’s note
All claims expressed in this article are solely those of the authors and do not necessarily represent those of their affiliated organizations, or those of the publisher, the editors and the reviewers. Any product that may be evaluated in this article, or claim that may be made by its manufacturer, is not guaranteed or endorsed by the publisher.
Supplementary material
The Supplementary Material for this article can be found online at: https://www.frontiersin.org/articles/10.3389/fcosc.2024.1406944/full#supplementary-material
Footnotes
- ^ Poor, E., Schieck, B., Cox, J., Guthrie, J., and Mullinax, J. (In Review). Towards robust corridors – a novel validation framework to more efficiently create corridors.
References
Abernathy H. N., Crawford D. A., Garrison E. P., Chandler R. B., Conner M. L., Miller K. V., et al. (2019). Deer movement and resource selection during Hurricane Irma: implications for extreme climatic events and wildlife. Proc. R. Soc B. 286, 20192230. doi: 10.1098/rspb.2019.2230
Anderson S. J., Kierepka E. M., Swihart R. K., Latch E. K., Rhodes O. E. (2015). Assessing the permeability of landscape features to animal movement: using genetic structure to infer functional connectivity. PloS One 10, e0117500. doi: 10.1371/journal.pone.0117500
Andreu M., Zobrist K., Hinckley T. (2008). Management practices to support increased biodiversity in managed loblolly pine plantations. EDIS. 2008 (3). doi: 10.32473/edis-fr236-2008
Ashrafzadeh M. R., Khosravi R., Adibi M. A., Taktehrani A., Wan H. Y., Cushman S. A. (2020). A multi-scale, multi-species approach for assessing effectiveness of habitat and connectivity conservation for endangered felids. Biol. Conserv. 245, 108523. doi: 10.1016/j.biocon.2020.108523
Assis V. R., Gardner S. T., Smith K. M., Gomes F. R., Mendonça M. T. (2020). Stress and immunity: Field comparisons among populations of invasive cane toads in Florida. J. Exp. Zool 333, 779–791. doi: 10.1002/jez.2389
Baldwin R. F., Reed S. E., McRae B. H., Theobald D. M., Sutherland R. W. (2012). Connectivity restoration in large landscapes: modeling landscape condition and ecological flows. Ecol. Restor. 30, 274–279. doi: 10.3368/er.30.4.274
Bauder J. M. (2019). Population viability and connectivity of the federally threatened eastern indigo snake in central peninsular florida. [Dissertation (PhD)]. (Massachusetts: University of Massachusetts Amherst). doi: 10.7275/XNH7-9S97
Bauder J. M., Peterman W. E., Spear S. F., Jenkins C. L., Whiteley A. R., McGarigal K. (2021). Multiscale assessment of functional connectivity: Landscape genetics of eastern indigo snakes in an anthropogenically fragmented landscape in central Florida. Mol. Ecol. 30, 3422–3438. doi: 10.1111/mec.15979
Baynard C. W., Schupp R. W., Zhang P., Fadil P. (2014). A geospatial approach to measuring surface disturbance related to oil and gas activities in west florida, USA. ARS 03, 77–93. doi: 10.4236/ars.2014.32007
Beier P., Majka D. R., Spencer W. D. (2008). Forks in the road: choices in procedures for designing wildland linkages: design of wildlife linkages. Conserv. Biol. 22, 836–851. doi: 10.1111/j.1523-1739.2008.00942.x
Beier P., Spencer W., Baldwin R. F., McRAE B. H. (2011). Toward best practices for developing regional connectivity maps: regional connectivity maps. Conserv. Biol. 25, 879–892. doi: 10.1111/j.1523-1739.2011.01716.x
Boughton R., Cooney S., Frohlich K., Gruver B., Mezich R., Rockwood S., et al. (2019). Florida’s state wildlife action plan. Florida Fish and Wildlife Conservation Commission (FWC).
Breckheimer I., Haddad N. M., Morris W. F., Trainor A. M., Fields W. R., Jobe R. T., et al. (2014). Defining and evaluating the umbrella species concept for conserving and restoring landscape connectivity: umbrellas species for connectivity. Conserv. Biol. 28, 1584–1593. doi: 10.1111/cobi.12362
Brodeur J. (2021). Florida wildlife corridor act (CS/CS/SB 976: protection of ecological systems). Environment and Natural Resources Committee (EN) Florida Senate.
Brodie J. F., Giordano A. J., Dickson B., Hebblewhite M., Bernard H., Mohd-Azlan J., et al. (2015). Evaluating multispecies landscape connectivity in a threatened tropical mammal community: Multispecies Habitat Corridors. Conserv. Biol. 29, 122–132. doi: 10.1111/cobi.12337
Caple Z. (2017). Holocene in fragments: a critical landscape ecology of phosphorus in Florida (Santa Cruz, Santa Cruz: University of California).
Carlier J., Moran J. (2019). Landscape typology and ecological connectivity assessment to inform Greenway design. Sci. Total Environ. 651, 3241–3252. doi: 10.1016/j.scitotenv.2018.10.077
Carr M., Zwick P. (2016). Florida 2070 mapping florida’s future – alternative patterns of development in 2070. Technical Report. 1000 Tallahassee, Fla.: 1000 Friends of Florida.
Carroll C., McRAE B. H., Brookes A. (2012). Use of linkage mapping and centrality analysis across habitat gradients to conserve connectivity of gray wolf populations in western north america. Conserv. Biol. 26 (1), 78–87. doi: 10.1111/j.1523-1739.2011.01753.x
Catano C. P., Romañach S. S., Beerens J. M., Pearlstine L. G., Brandt L. A., Hart K. M., et al. (2015). Using scenario planning to evaluate the impacts of climate change on wildlife populations and communities in the florida everglades. Environ. Manage. 55, 807–823. doi: 10.1007/s00267-014-0397-5
Compton B. W., MCGarigal K., Cushman S. A., Gamble L. R. (2007). A resistant-kernel model of connectivity for amphibians that breed in vernal pools. Conserv. Biol. 21, 788–799. doi: 10.1111/j.1523-1739.2007.00674.x
Conyers Z., Roy S. S. (2021). Modelling the role of environmental variables in determining the distribution of invasive Burmese Python in Florida. Spat. Inf. Res. 29, 749–760. doi: 10.1007/s41324-021-00394-6
Correa Ayram C. A., Mendoza M. E., Etter A., Pérez-Salicrup D. R. (2019). Effect of the landscape matrix condition for prioritizing multispecies connectivity conservation in a highly biodiverse landscape of Central Mexico. Reg. Environ. Change 19, 149–163. doi: 10.1007/s10113-018-1393-8
Cox K., Maes J., Van Calster H., Mergeay J. (2017). Effect of the landscape matrix on gene flow in a coastal amphibian metapopulation. Conserv. Genet. 18, 1359–1375. doi: 10.1007/s10592-017-0985-z
Cushman S. A., Landguth E. L. (2012). Multi-taxa population connectivity in the Northern Rocky Mountains. Ecol. Model. 231, 101–112. doi: 10.1016/j.ecolmodel.2012.02.011
Davis A. G., Cox J. J., Fei S. (2021). Alternative 2070: Mitigating the effects of projected sea level rise and urbanization on Florida black bear and Florida panther habitat. J. Nat. Conserv. 63, 126052. doi: 10.1016/j.jnc.2021.126052
De María M., Silva-Sanchez C., Kroll K. J., Walsh M. T., Nouri M.-Z., Hunter M. E., et al. (2021). Chronic exposure to glyphosate in Florida manatee. Environ. Int. 152, 106493. doi: 10.1016/j.envint.2021.106493
DeMatteo K. E., Escalante O. M., Ibañez Alegre D. M., Rinas M. A., Sotorres D., Argüelles C. F. (2023). A multispecies corridor in a fragmented landscape: Evaluating effectiveness and identifying high-priority target areas. PloS One 18, e0283258. doi: 10.1371/journal.pone.0283258
Dilkina B., Houtman R., Gomes C. P., Montgomery C. A., McKelvey K. S., Kendall K., et al. (2017). Trade-offs and efficiencies in optimal budget-constrained multispecies corridor networks. Conserv. Biol. 31, 192–202. doi: 10.1111/cobi.12814
Di Minin E., Macmillan D. C., Goodman P. S., Escott B., Slotow R., Moilanen A. (2013). Conservation businesses and conservation planning in a biological diversity hotspot: Conservation businesses and planning. Conserv. Biol. 27, 808–820. doi: 10.1111/cobi.12048
Donald P. F., Evans A. D. (2006). Habitat connectivity and matrix restoration: the wider implications of agri-environment schemes: Habitat connectivity and matrix restoration. J. Appl. Ecol. 43, 209–218. doi: 10.1111/j.1365-2664.2006.01146.x
Dondina O., Orioli V., Torretta E., Merli F., Bani L., Meriggi A. (2020). Combining ensemble models and connectivity analyses to predict wolf expected dispersal routes through a lowland corridor. PloS One 15, e0229261. doi: 10.1371/journal.pone.0229261
Dormann C. F., Calabrese J. M., Guillera-Arroita G., Matechou E., Bahn V., Bartoń K., et al. (2018). Model averaging in ecology: a review of Bayesian, information-theoretic, and tactical approaches for predictive inference. Ecol. Monogr. doi: 10.1002/ecm.1309
Duan S., Banger K., Toor G. (2021). Evidence of phosphate mining and agriculture influence on concentrations, forms, and ratios of nitrogen and phosphorus in a florida river. Water 13, 1064. doi: 10.3390/w13081064
Edwards A., Swall J., Jagoe C. (2019). Mercury concentrations in bat guano from caves and bat houses in florida and Georgia. Virginia J. Sci. 70, 1–13. Available at: https://digitalcommons.odu.edu/vjs/vol70/iss3/4/.
Enge K. M., Marion W. R. (1986). Effects of clearcutting and site preparation on herpetofauna of a North Florida flatwoods. For. Ecol. Manage. 14, 177–192. doi: 10.1016/0378-1127(86)90116-7
Engeman R. M., Kaiser B. W., Osorio K. J. (2019). Evaluating methods to detect and monitor populations of a large invasive lizard: the Argentine giant tegu. Environ. Sci. pollut. Res. 26, 31717–31729. doi: 10.1007/s11356-019-06324-2
Facemire C. F., Gross T. S., Guillette L. J. (1995). Reproductive impairment in the Florida panther: nature or nurture? Environ. Health Perspect. 103, 79–86. doi: 10.1289/ehp.103-1519283
Florida Natural Areas Inventory (2010). Natural communities (Natural Communities of Florida). Available online at: https://www.fnai.org/species-communities/natural-communities (Accessed 8.24.23).
Fox C., Huettmann F., Harvey G., Morgan K., Robinson J., Williams R., et al. (2017). Predictions from machine learning ensembles: marine bird distribution and density on Canada’s Pacific coast. Mar. Ecol. Prog. Ser. 566, 199–216. doi: 10.3354/meps12030
Fox T. R., Jokela E. J., Allen H. L. (2007). The development of pine plantation silviculture in the southern United States. J. Forestry. doi: 10.1093/jof/105.7.337
Frakes R. A., Belden R. C., Wood B. E., James F. E. (2015). Landscape analysis of adult florida panther habitat. PloS One 10, e0133044. doi: 10.1371/journal.pone.0133044
Frakes R. A., Knight M. L. (2021). Location and extent of unoccupied panther (Puma concolor coryi) habitat in Florida: Opportunities for recovery. Global Ecol. Conserv. 26, e01516. doi: 10.1016/j.gecco.2021.e01516
FWC (2017). Sherman’s fox squirrel biological status review report (Species status report) (Florida Fish and Wildlife Conservation Commission, Tallahassee).
Gallo J., Butts E., Miewald T., Foster K. (2020). Comparing and combining omniscape and linkage mapper connectivity analyses in western washington (figshare). doi: 10.6084/M9.FIGSHARE.8120924
Gustafson K. D., Gagne R. B., Vickers T. W., Riley S. P. D., Wilmers C. C., Bleich V. C., et al. (2019). Genetic source–sink dynamics among naturally structured and anthropogenically fragmented puma populations. Conserv. Genet. 20, 215–227. doi: 10.1007/s10592-018-1125-0
Haddad N. M., Brudvig L. A., Clobert J., Davies K. F., Gonzalez A., Holt R. D., et al. (2015). Habitat fragmentation and its lasting impact on Earth’s ecosystems. Sci. Adv. 1, e1500052–e1500052. doi: 10.1126/sciadv.1500052
Hardin S. (2007). “Managing non-native wildlife in florida: State perspective, policy and practice,” in Managing vertebrate invasive species: proceedings of an international symposium. Eds. Witmer G. W., Pitt W. C., Fagerstone K. A. (Fort Collins, Colorado, USA: USDA/APHIS Wildlife Services, National Wildlife Research Center). Available at: http://www.aphis.usda.gov/wildlife_damage/nwrc/symposia/invasive_symposium/nwrc_TOC_index.shtml.
Harveson P. M., Lopez R. R., Collier B. A., Silvy N. J. (2007). Impacts of urbanization on Florida Key deer behavior and population dynamics. Biol. Conserv. 134, 321–331. doi: 10.1016/j.biocon.2006.07.022
Hedrick P. W., Fredrickson R. (2010). Genetic rescue guidelines with examples from Mexican wolves and Florida panthers. Conserv. Genet. 11, 615–626. doi: 10.1007/s10592-009-9999-5
Heil C. A., Muni-Morgan A. L. (2021). Florida’s harmful algal bloom (HAB) problem: escalating risks to human, environmental and economic health with climate change. Front. Ecol. Evol. 9. doi: 10.3389/fevo.2021.646080
Hiatt D., Serbesoff-King K., Lieurance D., Gordon D. R., Flory S. L. (2019). Allocation of invasive plant management expenditures for conservation: Lessons from Florida, USA. Conserv. Sci. Prac. 1 (1), e51. doi: 10.1111/csp2.51
Hoctor T. S., Allen W. L., Carr M. H., Zwick P. D., Huntley E., Smith D. J., et al. (2007). Land corridors in the Southeast USA: connectivity to protect biodiversity and ecosystem services. Journal of Conservation Planning 4, 90122.
Hoctor T. S., Carr M. H., Zwick P. D. (2000). Identifying a linked reserve system using a regional landscape approach: the florida ecological network. Conserv. Biol. 14, 984–1000. doi: 10.1046/j.1523-1739.2000.99075.x
Hoctor T., Volk M. (2021a). Florida ecological greenways network (FEGN). Final Report. Florida Fish and Wildlife Conservation Commission, Tallahassee, FL.
Hoctor T., Volk M. (2021b). Florida ecological greenways network update project final report. Final Report. Florida Fish and Wildlife Conservation Commission, Tallahassee, FL.
Hostetler J. A., Onorato D. P., Jansen D., Oli M. K. (2013). A cat’s tale: the impact of genetic restoration on Florida panther population dynamics and persistence. J. Anim. Ecol. 82, 608–620. doi: 10.1111/1365-2656.12033
Hostetler J. A., Walter McCown J., Garrison E. P., Neils A. M., Barrett M. A., Sunquist M. E., et al. (2009). Demographic consequences of anthropogenic influences: Florida black bears in north-central Florida. Biol. Conserv. 142, 2456–2463. doi: 10.1016/j.biocon.2009.05.029
Hyslop N. L. (2007). Movements, habitat use, and survival of the threatened Eastern Indigo Snake (Drymarchon couperi) in Georgia. [Dissertation (PhD)]. University of Georgia. Available at: https://esploro.libs.uga.edu/esploro/outputs/doctoral/Movements-habitat-use-and-survival-of/9949334864702959.
Hyslop N. L., Meyers J. M., Cooper R. J., Stevenson D. J. (2014). Effects of body size and sex of Drymarchon couperi (eastern indigo snake) on habitat use, movements, and home range size in Georgia: Indigo Snake Home Range and Habitat Use. Jour. Wild. Mgmt. 78, 101–111. doi: 10.1002/jwmg.645
Iverson A. R., Waetjen D., Shilling F. (2024). Functional landscape connectivity for a select few: Linkages do not consistently predict wildlife movement or occupancy. Landscape Urban Plann. 243, 104953. doi: 10.1016/j.landurbplan.2023.104953
Jennings M., Haeuser E., Foote D., Lewison R., Conlisk E. (2020a). Planning for dynamic connectivity: operationalizing robust decision-making and prioritization across landscapes experiencing climate and land-use change. Land 9, 341. doi: 10.3390/land9100341
Jennings M., Zeller K. A., Lewison R. L. (2020b). Supporting adaptive connectivity in dynamic landscapes. Land 9, 295. doi: 10.3390/land9090295
Johnson W. E., Onorato D. P., Roelke M. E., Land E. D., Cunningham M., Belden R. C., et al. (2010). Genetic restoration of the florida panther. Science 329, 1641–1645. doi: 10.1126/science.1192891
Julian P., Everham E. M., Main M. B. (2012). Influence of a Large-scale Removal of an Invasive Plant ( Melaleuca quinquenervia ) on Home-range Size and Habitat Selection by Female Florida Panthers ( Puma concolor coryi ) within Big Cypress National Preserve, Florida. Southeastern Nat. 11, 337–348. doi: 10.1656/058.011.0215
Karelus D. L., McCown J. W., Scheick B. K., van de Kerk M., Oli M. K. (2016). Home ranges and habitat selection by black bears in a newly colonized population in Florida. Southeastern Naturalist 15, 346. doi: 10.1656/058.015.0215
Karelus D. L., McCown J. W., Scheick B. K., van de Kerk M., Bolker B. M., Oli M. K. (2019). Incorporating movement patterns to discern habitat selection: black bears as a case study. Wildl. Res. 46, 76. doi: 10.1071/WR17151
Kautz R., Stys B., Kawula R. (2007). Florida vegetation 2003 and land use change between 1985-1989 and 2003. Agricultural and Natural Resource Sciences. 70, 12–23. Available at: https://www.jstor.org/stable/24321563.
Keeley A. T. H., Beier P., Gagnon J. W. (2016). Estimating landscape resistance from habitat suitability: effects of data source and nonlinearities. Landscape Ecol. 31, 2151–2162. doi: 10.1007/s10980-016-0387-5
Kerk M., Onorato D. P., Hostetler J. A., Bolker B. M., Oli M. K. (2019). Dynamics, persistence, and genetic management of the endangered florida panther population. Wild. Mon. 203, 3–35. doi: 10.1002/wmon.1041
Khare Y. P., Paudel R., Wiederholt R., Abiy A. Z., Van Lent T., Davis S. E., et al. (2021). Watershed response to legacy phosphorus and best management practices in an impacted agricultural watershed in florida, U.S.A. Land 10, 977. doi: 10.3390/land10090977
Knight A. T., Cowling R. M., Campbell B. M. (2006). An operational model for implementing conservation action. Conserv. Biol. 20, 408–419. doi: 10.1111/j.1523-1739.2006.00305.x
Knight A. T., Cowling R. M., Possingham H. P., Wilson K. A. (2009). “From theory to practice: Designing and situating spatial prioritization approaches to better implement conservation action,” in Spatial conservation prioritization. Eds. Moilanen A., Wilson K. A., Possingham H. P. (Oxford: Oxford University Press), 249–259. doi: 10.1093/oso/9780199547760.003.0018
Koen E. L., Bowman J., Sadowski C., Walpole A. A. (2014). Landscape connectivity for wildlife: development and validation of multispecies linkage maps. Methods Ecol. Evol. 5, 626–633. doi: 10.1111/2041-210X.12197
Landau V., Shah V., Anantharaman R., Hall K. (2021). Omniscape.jl: Software to compute omnidirectional landscape connectivity. JournalofOpenSourceSoftware 6, 2829. doi: 10.21105/joss.02829
Landguth E. L., Hand B. K., Glassy J., Cushman S. A., Sawaya M. A. (2012). UNICOR: a species connectivity and corridor network simulator. Ecography 35, 9–14. doi: 10.1111/j.1600-0587.2011.07149.x
Lapointe B. E., Brewton R. A., Wilking L. E., Herren L. W. (2023). Fertilizer restrictions are not sufficient to mitigate nutrient pollution and harmful algal blooms in the Indian River Lagoon, Florida. Mar. pollut. Bull. 193, 115041. doi: 10.1016/j.marpolbul.2023.115041
Lehtomäki J., Moilanen A., Toivonen T., Leathwick J. (2016). Running a zonation planning project. Technical Report. The Biodiversity Informatics Conservation Group, University of Helsinki. doi: 10.13140/RG.2.1.4919.5289
Li X., Fatowe M., Lemos L., Quinete N. (2022). Spatial distribution of per- and polyfluoroalkyl substances (PFAS) in waters from Central and South Florida. Environ. Sci. pollut. Res. 29, 84383–84395. doi: 10.1007/s11356-022-21589-w
Lindemann D. (2009). History of south florida pine rocklands and how they have been negatively affected by fire suppression and fragmentation (Institute of Food and Agriculture, University of Florida).
Lines R., Bormpoudakis D., Xofis P., Tzanopoulos J. (2021). Modelling multi-species connectivity at the kafue-zambezi interface: implications for transboundary carnivore conservation. Sustainability 13, 12886. doi: 10.3390/su132212886
Liu C., Newell G., White M., Bennett A. F. (2018). Identifying wildlife corridors for the restoration of regional habitat connectivity: A multispecies approach and comparison of resistance surfaces. PloS One 13, e0206071. doi: 10.1371/journal.pone.0206071
Lopez R. R., Silvy N. J., Wilkins R. N., Frank P. A., Peterson M. J., Peterson M. N. (2004). Habitat-use patterns of florida key deer: implications of urban development. J. Wildlife Manage. 68, 900–908. doi: 10.2193/0022-541X(2004)068[0900:HPOFKD]2.0.CO;2
Maehr D. S., Land E. D., Shindle D. B., Bass O. L., Hoctor T. S. (2002). Florida panther dispersal and conservation. Biol. Conserv. 106, 187–197. doi: 10.1016/S0006-3207(01)00245-2
Marburger J. E., Johnson W. E., Gross T. S., Douglas D. R., Di J. (2002). Residual organochlorine pesticides in soils and fish from wetland restoration areas in central Florida, USA. Wetlands 22, 705–711. doi: 10.1672/0277-5212(2002)022[0705:ROPISA]2.0.CO;2
Marrotte R. R., Bowman J., Brown M. G. C., Cordes C., Morris K. Y., Prentice M. B., et al. (2017). Multi-species genetic connectivity in a terrestrial habitat network. Mov Ecol. 5, 21. doi: 10.1186/s40462-017-0112-2
Mateo-Sánchez M. C., Balkenhol N., Cushman S., Pérez T., Domínguez A., Saura S. (2015). A comparative framework to infer landscape effects on population genetic structure: are habitat suitability models effective in explaining gene flow? Landscape Ecol. 30, 1405–1420. doi: 10.1007/s10980-015-0194-4
McRae B. H., Hall S. A., Beier P., Theobald D. M. (2012). Where to restore ecological connectivity? Detecting barriers and quantifying restoration benefits. PloS One 7, e52604. doi: 10.1371/journal.pone.0052604
McRae B. H., Kavanagh D. (2011). Linkage mapper connectivity analysis software. The Nature Conservancy, Seattle, WA. Available at: https://linkagemapper.org.
McRae B., Popper K., Jones A., Schindel M., Buttrick S., Hall K., et al. (2016). Conserving nature’s stage: mapping omnidirectional connectivity for resilient terrestrial landscapes in the pacific northwest. Final Report to the Doris Duke Charitable Foundation. doi: 10.13140/RG.2.1.4158.6166
Means D. B., Palis J. G., Baggett M. (1996). Effects of slash pine silviculture on a florida population of flatwoods salamander. Conserv. Biol. 10, 426–437. doi: 10.1046/j.1523-1739.1996.10020426.x
Metcalf M. F. (2017). Spatial Ecology of the threatened eastern indigo snake (Drymarchon couperi) in a subtropical coastal landscape in the southern extent of its range. (Master’s Thesis). Florida Gulf Coast University.
Moilanen A. (2007). Landscape Zonation, benefit functions and target-based planning: Unifying reserve selection strategies. Biol. Conserv. 134, 571–579. doi: 10.1016/j.biocon.2006.09.008
Moilanen A., Anderson B. J., Eigenbrod F., Heinemeyer A., Roy D. B., Gillings S., et al. (2011). Balancing alternative land uses in conservation prioritization. Ecol. Appl. 21, 1419–1426. doi: 10.1890/10-1865.1
Moilanen A., Arponen A. (2011). Administrative regions in conservation: Balancing local priorities with regional to global preferences in spatial planning. Biol. Conserv. 144, 1719–1725. doi: 10.1016/j.biocon.2011.03.007
Moilanen A., Franco A. M. A., Early R. I., Fox R., Wintle B., Thomas C. D. (2005). Prioritizing multiple-use landscapes for conservation: methods for large multi-species planning problems. Proc. R. Soc B. 272, 1885–1891. doi: 10.1098/rspb.2005.3164
Montero N., Ceriani S. A., Graham K., Fuentes M. M. P. B. (2018). Influences of the local climate on loggerhead hatchling production in north florida: implications from climate change. Front. Mar. Sci. 5. doi: 10.3389/fmars.2018.00262
NatureServe (2021). Access data on species & Ecosystems. NatureServe. Available at: https://www.natureserve.org/access-data.
Noss R. F., Platt W. J., Sorrie B. A., Weakley A. S., Means D. B., Costanza J., et al. (2015). How global biodiversity hotspots may go unrecognized: lessons from the North American Coastal Plain. Diversity Distrib. 21, 236–244. doi: 10.1111/ddi.12278
Oetting J., Hoctor T., Volk M. (2016). Critical lands and waters identification project (CLIP): version 4.0. Florida Natural Areas Inventory. Florida State University, 140.
Offer J. H. (2020). Prioritizing parcels for conservation easements using least-cost path analyses of land ownership: case study within theorized grizzly bear migration corridors of western Montana. (Master of Science) (Montana: University of Montana).
Outman C. J. (2020). Florida’s red tide: the hidden costs of land development in the everglades (New York, NY United States: Fordham University).
Poor E. E., Scheick B. K., Mullinax J. M. (2020). Multiscale consensus habitat modeling for landscape level conservation prioritization. Scientific Reports 10, 17783. doi: 10.1038/s41598-020-74716-3
Prince A., DePerno C. S. (2014). Survival and home-range size of southeastern fox squirrels in north carolina. Southeastern Nat. 13, 456. doi: 10.1656/058.013.0305
Reece J. S., Noss R. F. (2014). Prioritizing species by conservation value and vulnerability: A new index applied to species threatened by sea-level rise and other risks in florida. Natural Areas J. 34, 31. doi: 10.3375/043.034.0105
Reece J. S., Noss R. F., Oetting J., Hoctor T., Volk M. (2013). A vulnerability assessment of 300 species in florida: threats from sea level rise, land use, and climate change. PloS One 8, e80658. doi: 10.1371/journal.pone.0080658
Repenning R. W., Labisky R. F. (1985). Effects of even-age timber management on bird communities of the longleaf pine forest in northern florida. J. Wildlife Manage. 49, 1088. doi: 10.2307/3801400
Riggio J., Foreman K., Freedman E., Gottlieb B., Hendler D., Radomille D., et al. (2022). Predicting wildlife corridors for multiple species in an East African ungulate community. PloS One 17, e0265136. doi: 10.1371/journal.pone.0265136
Rodgers P. D., Pienaar E. F. (2018). Tolerance for the Florida panther in exurban southwest Florida: Panther Tolerance in Exurban Communities. Jour. Wild. Mgmt. 82, 865–876. doi: 10.1002/jwmg.21431
Romañach S. S., Benscoter A. M., Haider S. M. (2020). Potential impacts of future urbanization and sea level rise on florida’s natural resources. J. Fish Wildlife Manage. 11, 174–184. doi: 10.3996/092019-JFWM-076
Sahraoui Y., Foltête J.-C., Clauzel C. (2017). A multi-species approach for assessing the impact of land-cover changes on landscape connectivity. Landscape Ecol. 32, 1819–1835. doi: 10.1007/s10980-017-0551-6
Santini L., Saura S., Rondinini C. (2016). A composite network approach for assessing multi-species connectivity: an application to road defragmentation prioritisation. PloS One 11, e0164794. doi: 10.1371/journal.pone.0164794
Saremi N. F., Supple M. A., Byrne A., Cahill J. A., Coutinho L. L., Dalén L., et al. (2019). Puma genomes from North and South America provide insights into the genomic consequences of inbreeding. Nat. Commun. 10, 4769. doi: 10.1038/s41467-019-12741-1
Schwab A. C. (2006). The influence of roads on the florida panther. USF Tampa Graduate Theses and Dissertations. Available at: https://digitalcommons.usf.edu/etd/3834.
Schwab A. C., Zandbergen P. A. (2011). Vehicle-related mortality and road crossing behavior of the Florida panther. Appl. Geogr. 31, 859–870. doi: 10.1016/j.apgeog.2010.10.015
Shen X., Li S., McShea W. J., Wang D., Yu J., Shi X., et al. (2020). Effectiveness of management zoning designed for flagship species in protecting sympatric species. Conserv. Biol. 34, 158–167. doi: 10.1111/cobi.13345
Shi X., Gong C., Zhang L., Hu J., Ouyang Z., Xiao Y. (2019). Which species should we focus on? Umbrella species assessment in southwest China. Biology 8, 42. doi: 10.3390/biology8020042
Sinclair J. S., Reisinger A. J., Bean E., Adams C. R., Reisinger L. S., Iannone B. V. (2020). Stormwater ponds: An overlooked but plentiful urban designer ecosystem provides invasive plant habitat in a subtropical region (Florida, USA). Sci. Total Environ. 711, 135133. doi: 10.1016/j.scitotenv.2019.135133
Sonnier G., Boughton E. H., Whittington R. (2023). Long-term response of wetland plant communities to management intensity, grazing abandonment, and prescribed fire. Ecol. Appl. 33, e2732. doi: 10.1002/eap.2732
Sparks G. M. (2021). Conserving working landscapes and open space: a socio-spatial analysis of private parcels suitable for conservation easements in Calveras County, California (Humboldt, Humboldt: California State Polytechnic University).
Spontak M., Hoctor T. (2017). A multi-faceted approach to corridor planning for the Florida black bear and Florida panther. Journal of Conservation Planning 13, 1–10.
Tavalieri Y. E., Galoppo G. H., Canesini G., Luque E. H., Muñoz-de-Toro M. M. (2020). Effects of agricultural pesticides on the reproductive system of aquatic wildlife species, with crocodilians as sentinel species. Mol. Cell. Endocrinol. 518, 110918. doi: 10.1016/j.mce.2020.110918
Tessier D. L., Maranger R., Poisot T. (2020). Omnidirectional and omnifunctional connectivity analyses with a diverse species pool (preprint). Ecology. doi: 10.1101/2020.02.03.932095
Thatcher C. A., van Manen F. T., Clark J. D. (2009). A habitat assessment for florida panther population expansion into central florida. J. Mammalogy 90, 918–925. doi: 10.1644/08-MAMM-A-219.1
Trainor A. M., Walters J. R., Morris W. F., Sexton J., Moody A. (2013). Empirical estimation of dispersal resistance surfaces: a case study with red-cockaded woodpeckers. Landscape Ecol. 28, 755–767. doi: 10.1007/s10980-013-9861-5
U.S. Fish and Wildlife Service (2018). Species status assessment (SSA) report for the eastern indigo snake. Version 1.0 (Drymarchon couperi). Available at: https://ecos.fws.gov/ecp/species/646.
Varner J. M., Gordon D. R., Putz F. E., Hiers J. K. (2005). Restoring fire to long-unburned pinus palustris ecosystems: novel fire effects and consequences for long-unburned ecosystems. Restor. Ecol. 13, 536–544. doi: 10.1111/j.1526-100X.2005.00067.x
Vermeylen M. K., Knowles T. G., Barron H. W. (2022). The influence of Lake Okeechobee discharges on Karenia brevis blooms and the effects on wildlife along the central west coast of Florida. Harmful Algae 115, 102237. doi: 10.1016/j.hal.2022.102237
Vizek A., Nielsen-Pincus M. (2017). Landowner attitudes toward conservation easements: balancing the private and public interest in land. Soc. Natural Resour. 30, 1080–1095. doi: 10.1080/08941920.2017.1331486
Volk M., Hoctor T., Nettles B., Hilsenbeck R., Putz F. (2017). “Florida land use and land cover change in the past 100 years,” in Florida’s climate: changes, variations, & Impacts (Florida Climate Institute). doi: 10.17125/fci2017.ch02
Wang F., McShea W. J., Li S., Wang D. (2018). Does one size fit all? A multispecies approach to regional landscape corridor planning. Divers. Distrib 24, 415–425. doi: 10.1111/ddi.12692
Zampieri N. E., Pau S., Okamoto D. K. (2020). The impact of Hurricane Michael on longleaf pine habitats in Florida. Sci. Rep. 10, 8483. doi: 10.1038/s41598-020-65436-9
Zeller K. A., Jennings M. K., Vickers T. W., Ernest H. B., Cushman S. A., Boyce W. M. (2018). Are all data types and connectivity models created equal? Validating common connectivity approaches with dispersal data. Diversity Distributions 24, 868–879. doi: 10.1111/ddi.12742
Zhu M.-J., Hoctor T. S., Volk M., Frank K. I., Zwick P. D., Carr M. H., et al. (2015). Spatial conservation prioritization to conserve biodiversity in response to sea level rise and land use change in the Matanzas River Basin, Northeast Florida. Landscape Urban Plann. 144, 103–118. doi: 10.1016/j.landurbplan.2015.08.018
Keywords: multispecies connectivity, spatial conservation prioritization, corridor design, infrastructure, landscape conservation planning, landscape architecture
Citation: Bohnett E, Oetting J, Noss R, O’Brien M, Frakes R, Smith D, Lockhart S, Mullinax J, Poor EE, Scheick B and Hoctor T (2024) Consolidating diverse modeling methods and spatial prioritization for multispecies connectivity planning. Front. Conserv. Sci. 5:1406944. doi: 10.3389/fcosc.2024.1406944
Received: 25 March 2024; Accepted: 03 July 2024;
Published: 26 July 2024.
Edited by:
David R. Breininger, University of Central Florida, United StatesCopyright © 2024 Bohnett, Oetting, Noss, O’Brien, Frakes, Smith, Lockhart, Mullinax, Poor, Scheick and Hoctor. This is an open-access article distributed under the terms of the Creative Commons Attribution License (CC BY). The use, distribution or reproduction in other forums is permitted, provided the original author(s) and the copyright owner(s) are credited and that the original publication in this journal is cited, in accordance with accepted academic practice. No use, distribution or reproduction is permitted which does not comply with these terms.
*Correspondence: Eve Bohnett, ZXZlYm9obmV0dEB1ZmwuZWR1