- 1Department of Natural Resources, Cornell University, Ithaca, NY, United States
- 2Wildlife Conservation Research Unit, Department of Biology, The Recanati-Kaplan Centre, Oxford University, Oxford, United Kingdom
- 3Department of Botany, University of Wisconsin — Madison, Madison, WI, United States
Forests in the United States continue to lose biodiversity and many fail to regenerate due to high deer (family Cervidae) abundance. Declines in biodiversity and overall ecosystem health due to high deer populations increases prevalence of wildlife and human diseases associated with increasing tick abundances and decreases forest resilience and the ability to deliver benefits provided by healthy ecosystems. In the eastern and midwestern United States, white-tailed deer (Odocoileus virginianus) are the main stressor, while in the western U.S. elk (Cervus elaphus) and black-tailed and mule deer (Odocoileus hemionus) can become equally problematic. Federal and State Wildlife Agencies are responsible for environmental stewardship and management of deer, migratory and endangered species, yet they lack authority to address human health concerns or commercial interests (we acknowledge tribal authority to manage wildlife as an important contributor to conservation). Furthermore, State Wildlife Agencies have retained their traditional focus to manage wildlife for recreational hunters while neglecting their obligations to manage wildlife in the interest of all citizens rather than special interest groups. Fragmented institutional arrangements and widely scattered responsibilities for human health, environmental conservation and management, agriculture, and commerce among tribal, federal, and state agencies have allowed deer impacts to grow into nationwide conservation and human health crises. Given that local, regional, and state-level initiatives have failed to provide appropriate remedies, federal leadership is now essential to integrate concerns among disciplines, policy domains, regions, habitats, and biota. We recommend developing a National Strategy to build strong collaborative efforts and diverse and inclusive relationships across environmental, human health and economic interests. These should reach beyond state boundaries to comprehensively address interrelated deer, human health, forest, and conservation crises. A well-coordinated and collaborative approach has the potential to overcome traditional turf battles between tribal, state, and federal interests by recognizing joint responsibilities and obligations to manage wildlife as a public trust resource. This collective approach can protect species before they become endangered, avoiding further declines in environmental and human health.
Introduction
Older residents of Cayuga Heights and Lansing, small villages in Central New York adjacent to Cornell University, remember gorges full of wildflowers. Graduate students from Cornell’s Botany, Ecology, and Entomology departments used their bicycles to get to local study sites in the 1950’s. These times are long gone — today these gorges are mostly devoid of native wildflowers, shrubs and saplings, and often entirely barren (Figure 1), except for the slowly dying old trees that are not being replaced. Memories of abundant wildflowers in local gorges disappear as human residents age, move, or die. New residents consider current conditions ‘normal’ and often have difficulties imagining what local gorges looked like only a few decades ago. Pauly (1995) described this loss of generational knowledge of previous ecological conditions as a ‘shifting baseline syndrome’. While Pauly (1995) was referencing memories of fisheries scientists and fishermen, his concept gained prominence as a basis for recognizing how sustained environmental degradation is limiting today’s expectations and perspectives for a different future (Alleway et al., 2023).
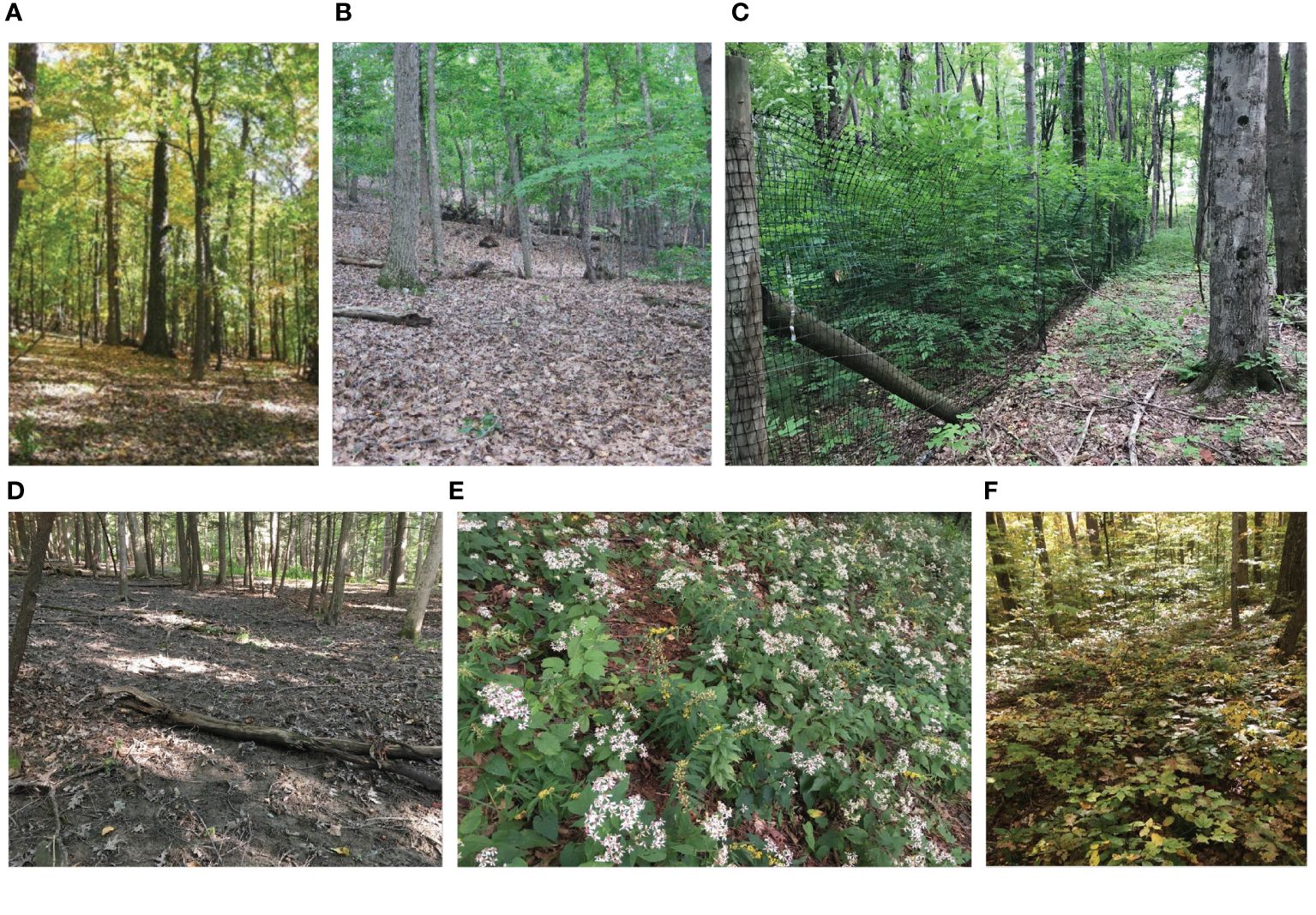
Figure 1 (A, B, D) Barren forest floor devoid of seedlings, saplings or wildflowers adjacent to gorges in the Village of Lansing, New York. Note lack of leaf litter and abundant earthworm middens (D). (C) Recruitment of tulip tree (Liriodendron tulipifera) in a local deer exclosure. (E) Display of white wood aster (Eurybia divaricata) and blue stem goldenrod (Solidago caesia). (F) Examples of seedling and sapling recruitment in an area with intensive deer management. Photo credits: (C) by Andy Zepp, all others by Bernd Blossey.
Archeologists and historians who rely on evidence preserved in sediments and pollen records have pieced together the regional waxing and waning of species and ecological communities (Gill et al., 2009; Oswald et al., 2020). Such information exists for trees, some grasses, and even megaherbivores (Gill et al., 2009), but we lack information how wildflower abundance has changed over generations and centuries. Evidence collected by botanists over the last 150 years indicate that across the eastern U.S., forest understory carpets of trilliums, lilies, orchids, and other charismatic wildflowers, have been replaced by near monocultures of sedges, ferns and, increasingly, introduced plants (Horsley et al., 2003; McGraw and Furedi, 2005; Knapp and Wiegand, 2014; Gorchov et al., 2021). Canada yew (Taxus canadensis) — once so common that its Ojibway name, ‘ne’bagandag’, is ‘the shrub that grows everywhere’ — has vanished from much of its range. In most instances, like in the gorges around Cornell, the species have not been entirely lost but are now confined to refugia on cliff edges, in the steepest parts of gorges, or on boulders (Comisky et al., 2005). These remaining individuals represent the potential for a return and a better and healthier future.
Protecting and restoring local and regional biodiversity requires recognizing the important stressors that lead to species declines. White-tailed deer (Odocoileus virginianus), whose populations have increased beyond their ecological carrying capacities, have been implicated as the main reason for widespread forest devastation (Côté et al., 2004; Miller et al., 2023). This is not only a problem in the Northeast, nor is it only wildflowers that have vanished. Entire cohorts of tree seedlings in forest understories — representing future forests — face poor survival odds and forests in the Great Lakes, Mid-Atlantic and Northeast (including National Parks and Monuments) are in imminent danger of recruitment failure affecting landscapes for decades to come (Patton et al., 2018; Rogers and McAvoy, 2018; Alverson et al., 2019; Miller and McGill, 2019; Miller et al., 2023). In western North America, black-tailed or mule deer (Odocoileus hemionus) and elk (Cervus elaphus) create similar problems (Porter and Underwood, 1999; Binkley et al., 2006; Martin et al., 2011; Rogers and McAvoy, 2018; Chollet et al., 2021) in western forests. The oldest National Park in the U.S., Yellowstone, has for decades been under severe elk browse pressure eliminating aspen (Populus tremuloides) and willow (Salix spp.) growth and recruitment (McNulty et al., 2020). Only recently has there been some relief associated with declines of the elk population (Brice et al., 2022). Some of these problems have been recognized for nearly a century (Leopold et al., 1947; Flader, 1974; Binkley et al., 2006), while others have been coming into focus recently due to purposeful deer introductions or range expansions (Martin et al., 2011; Sterba, 2012). However, beyond evidence often associated with research investigations, we do not fully comprehend the full extent of deer impacts (family Cervidae, including elk, white-tailed, black-tailed, and mule deer) at local or regional scales because we lack appropriate long-term monitoring (Quirion and Blossey, 2023).
Impacts of high deer populations
Environmental health
When Europeans arrived in North America, eastern forests had a diverse large herbivore assemblage including bison (Bison bison bison), woodland caribou (Rangifer tarandus caribou), moose (Alces alces), and elk (Foster et al., 2002), but we know little about how these large herbivores shaped abundances of trees, grasses, ferns and wildflowers. As European settlements expanded, persecution of large carnivores and unregulated market hunting drove deer, other large herbivores, and their predators to near extinction in the late 1800’s (Halls, 1984). Conservation efforts, enforcements of hunting laws, and abundant nutrition in the regrowing forests in the East, and subsidies provided by forestry operations, farming and gardening allowed deer (but not their predators) to quickly return to their traditional ranges and increase in abundance (Leopold et al., 1947).
Deer are selective browsers (although elk consume a higher proportion of grasses) and at low abundance limit recruitment of species they prefer to consume; at high abundance, deer can eliminate herbaceous and woody recruitment entirely (Figure 1), a process that plays out over decades. Island and exclosure studies implicate deer as the main driver of differences in plant community composition regardless of climate or previous land-use (Mudrak et al., 2009; Goetsch et al., 2011; Martin et al., 2011; Frerker et al., 2014; Alverson et al., 2019; Kelly, 2019; Dobson et al., 2024). Wildflowers and many shrubs never escape deer browsing and so tend to disappear first. Mature trees beyond the reach of deer, however, continue to produce many seeds and seedlings. These often grow to 10–20cm but then recurrent deer browsing prevents further heigh growth, often resulting in death (Kelly, 2019). Continued browse pressure leads to eventual seedling death creating a permanent regeneration debt (Russell et al., 2017; Vickers et al., 2019; Miller et al., 2023). These effects can last for decades (Knauer et al., 2023).
Impacts of high deer populations extend beyond the individual plants and species they consume. Insects, birds (including neotropical migrants), small mammals, nutrient cycling and forest carbon storage capabilities can all be negatively affected (Côté et al., 2004; Martin et al., 2011; Nuttle et al., 2011, 2014; Schweitzer et al., 2014; Chips et al., 2015; Mo et al., 2023; Phillips and Cristol, 2024). Introduced plants and earthworms thrive where deer are abundant (Dávalos et al., 2015a, b; Gorchov et al., 2021). High earthworm populations eliminate leaf litter (see Figure 1D), which decreases litter invertebrate abundance, which, in turn, leads to collapse of salamander and ground nesting bird populations (Maerz et al., 2009; Loss and Blair, 2011).
Climate change
Climates have always fluctuated, and species respond in three general ways: move, tolerate in place, and evolve. Deer can affect all three, interfering with inherent climate resiliency of other species. Mobile animals can move and actively search for new residences — wildflowers, shrubs, and trees disperse passively via seeds, fruits and vegetative fragments. Effective dispersal requires plants to produce large numbers of seeds that can find suitable safe sites to germinate and grow. By reducing local abundance, flowering, and seed output (Figure 2), deer limit reproductive efforts of wildflowers leading to shrinking populations (Mooney and McGraw, 2007; Knight et al., 2009; Flaherty et al., 2018) and reduced dispersal capabilities. Reduced population size and reproductive effort also curtails the ability of species to respond to climate change with rapid evolutionary adaptations (facilitated by large gene pools) and increases risks of inbreeding depression (Lankau, 2011; Williams et al., 2016). Propagules that disperse will find few safe places to germinate and grow because deer and their high browse pressure are ubiquitous. Even for species able to tolerate climate change in place, old individuals will need to be replaced by new recruits, but deer curtail or prevent this option for palatable species. In summary, deer appear to be a larger threat to wildlflowers, shrubs, and trees than climate change, curtailing their ability to respond via dispersal, phenotypic plasticity or rapid evolution.
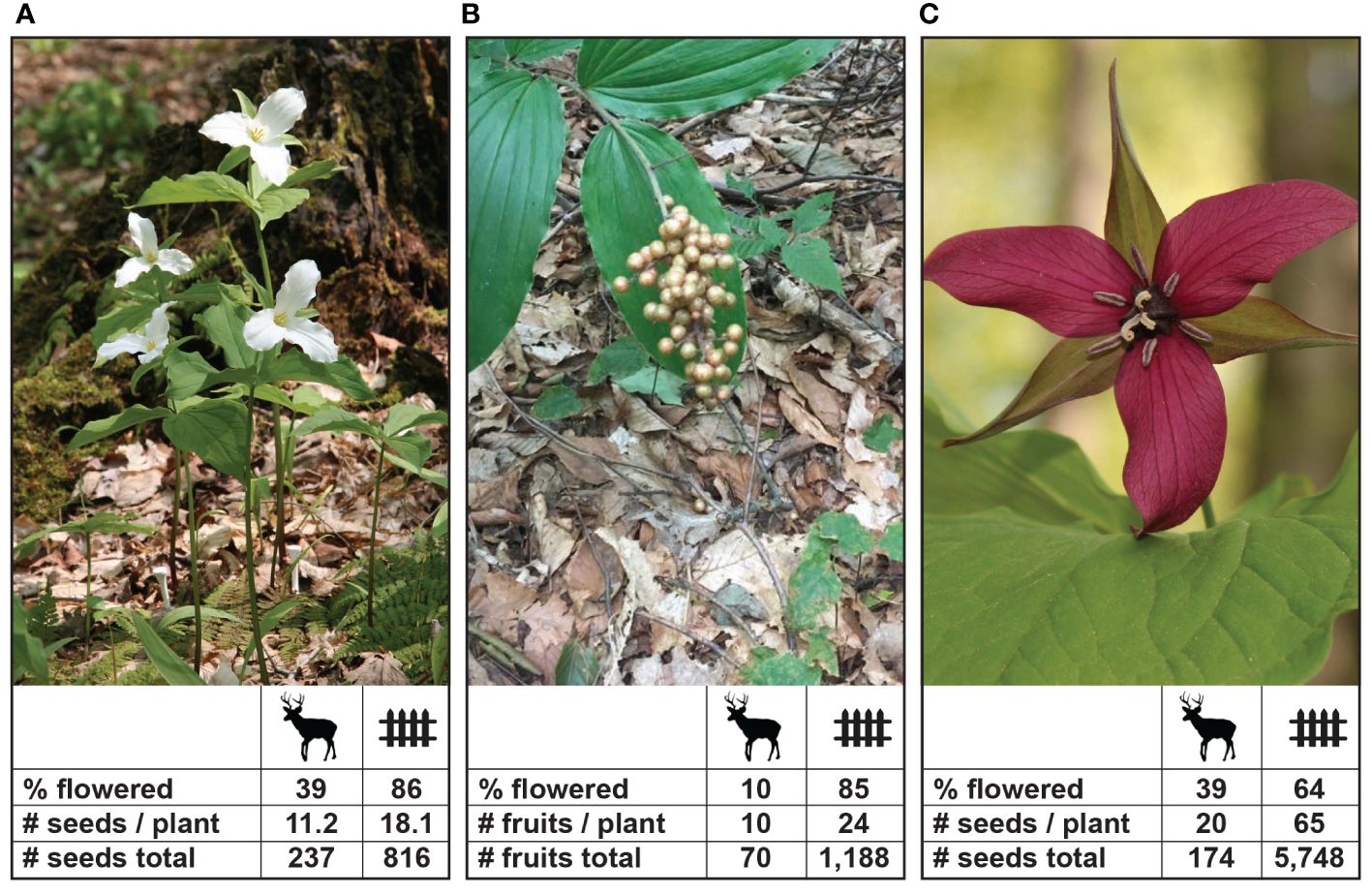
Figure 2 Examples of charismatic understory species that are preferred by deer and their reproductive effort and output (N = 100 individuals) with and without deer access in 2015 at Bobolink Hill, Richford, New York. Depicted are (A) Trillium grandiflorum; (B) Maianthemum racemosum; and (C) Trillium erectum. For M. racemosum these are fruits/plant containing 1–3 seeds/fruit. Photo credits: Bernd Blossey. Deer icon by Tracy Heath (phylopics.org); fence icon from vectorstock.com.
Human health
When forest biodiversity declines, infection rates of diseases affecting wildlife and humans increase (Civitello et al., 2015; Keesing and Ostfeld, 2015; Ostfeld and Keesing, 2017). At high deer abundance, plant and animal diversity decreases while the abundance of black-legged (deer) ticks (Ixodes scapularis) and the incidence of Lyme and other tick borne diseases increase (Raizman et al., 2013; Werden et al., 2014). Deer are the main host for adult ticks and risks of tick-borne diseases (e.g. Lyme, Ehrlichiosis, Babesiosis, Anaplasmosis, Rocky Mountain spotted fever, Powassan virus) are steadily increasing across the U.S. (Telford, 2017). But where deer abundance is low, for example in urban parks, I. scapularis is absent or occurs only in extremely low abundance (VanAcker et al., 2019). When deer reduce plant and animal diversity overall, the opposite response is observed in generalist species. White footed mice (Peromyscus leucopus), eastern chipmunks (Tamias striatus) and American robins (Turdus migratorius) thrive in altered systems and are all competent hosts of Borellia burgdorferi, the spirochaete causing Lyme disease. While deer-tick-disease dynamics are complex (Kilpatrick et al., 2017), the focus on white-footed mice as the main driver of tick and Lyme disease dynamics overlooks many other competent bird and mammalian hosts (Zikeli and Zohdy, 2020; Goethert and Telford, 2022). Experiments attempting to lower tick abundance by targeting ticks directly using the biopesticide Met52 (F52 strain of the fungus Metarhizium brunneum) and bait boxes attracting small rodents and applying tick-killing fipronil, failed to reduce tick abundance or the number of tick-borne diseases reported by humans (Ostfeld et al., 2023). In contrast, experimental deer reductions dramatically decreased tick abundance and Lyme disease incidence (Stafford et al., 2003; Kilpatrick et al., 2014).
High deer populations facilitate the emergence and spread of other diseases with serious consequences for human, wildlife and livestock health. One example is chronic wasting disease (CWD) a debilitating disease of the nervous system (ataxia), facilitated by high deer populations, that results in slow agonizing deaths in affected ungulates. Shipments of infected animals from captive deer herds led to its rapid spread (Escobar et al., 2020; Maloney et al., 2020). CWD receives enormous attention as a potential threat to human health, because it is broadly similar to Creutzfed-Jacob (or BSE) disease, although there are no reported cases of deer-human transmission (Williams et al., 2002). Management agencies spend significant resources on public awareness campaigns and hunter education but these efforts have failed to reduce deer populations or CWD spread and infection rates (Jennelle et al., 2014). Modelling exercises indicate that abundant large predators might reduce CWD prevalence in wild herds by selectively removing weak individuals (Wild et al., 2011), but these predators remain absent over much of the U.S. White-tailed deer in North America are also a reservoir for SARS-CoV-2 with potential negative consequences for livestock and human health, but the virus has not been detected in European deer (Mallapaty, 2022).
Economic interests
High deer populations also threaten economic productivity and livelihoods. Two decades ago, Conover (2002) estimated that annual crop losses due to deer in the U.S. exceeded $900 million and wildlife damage compensations were estimated at >$4 billion. More recent and comprehensive data on economic costs of deer have not been published, but a recent study in New Jersey estimated that just 27 small farms incurred $1.4 million in financial losses due to deer impacts in 2019 (Paulin et al., 2022). This included direct losses in marketable crops, but also costs to install and maintain physical protections (fences) and the inability to grow preferred crops. There is no national approach or methodology to help quantify economic losses due to deer impacts in forestry, gardening, agriculture, tourism or conservation. Losses are likely greatly underestimated due to lack of reliable assessment methods (Kovacs et al., 2020). Substantial health and economic losses also occur on U.S. highways where 2.1 million deer are killed annually, often in gruesome ways, injuring nearly 60,000 people with >400 human fatalities at a cost of >$10 billion (Cunningham et al., 2022).
Depending on the region, 2–4% of state residents participate in hunting with economic benefits estimated at $26 billion in 2016; 60% of this is associated with deer and other big game hunting (U.S. Fish and Wildlife Service, 2018). In contrast, 26–35% of state residents participate in wildlife watching activities contributing $75 billion to the U.S. Economy in 2016 (U.S. Fish and Wildlife Service, 2018). The Bureau of Economic Analysis estimates that outdoor recreation contributed $454 billion to the U.S. GDP in 2021 (https://www.bea.gov/data/special-topics/outdoor-recreation) and non-consumptive outdoor activities by far outperform hunting as an economic driver. This overview, even without comprehensive accounting, suggests that economic costs of high deer populations are likely to greatly outweigh their benefits.
Humans, wolves, bears, cougars, and coyotes as deer population regulators
When Europeans arrived in eastern North America, large herbivores existed with large carnivores, specifically wolves (Canis lupus) and cougars (Puma concolor). Grizzly or brown bears (Ursus arctos) roamed from Alaska to Mexico but were restricted to areas west of the Mississippi (Hall, 1981). Coyotes (Canis latrans) were western species occupying the grasslands but expanded into eastern forested landscapes more recently (Hody and Kays, 2018). Black bears (Ursus americanus) occurred through much of North America, as did bobcats (Lynx rufus). Deer were also hunted for food, clothing and tools by Native Americans but effects of human and other predators, together with climate and disease on North American deer populations before European arrival are unclear as are estimates of historic deer abundance (McCabe and McCabe, 1984).
As Europeans pushed westward, unregulated market hunting decimated deer and other wildlife. Conservation efforts then allowed deer to make a remarkable comeback. Populations of their predaotr than also stsarted to rebound slowly. By the 1930’s and 40’s, deer reached high local abundances with overbrowsing evident on preferred species, resulting in poor nutrition, starvation and winter mortality (Leopold et al., 1947; Severinghouse and Brown, 1956). Ecosystems started to decline with accumulating negative impacts soon reaching crisis levels.. These negative impacts have now reached crises level. The question then is, what factors can achieve deer population control that recreational hunting, as presently practiced and regulated, has been unable to achieve?
Many conservationists believe that more successful deer management requires the return of large predators, which might initiate a trophic cascade (Estes et al., 2011; Ripple et al., 2014). Wolf reintroduction to Yellowstone National Park in 1995 to reverse impacts of enormous elk populations are often referenced for such effects. Yellowstone’s ecosystems may be recovering, but it is uncertain whether wolves actually played a key role as selective use of evidence may have overemphasized role (Ford and Goheen, 2015; Fleming, 2019; Brice et al., 2022). Although wolves and other large predators in Yellowstone (and elsewhere) kill large herbivores, particularly the very young and the very old, mortality due to human hunting during winters spent outside the park was likely more significant in initiating the elk population decline before wolves were released (Smith et al., 2020; Brice et al., 2022). Reports of coyotes reducing deer populations in the eastern U.S. are disputed (Robinson et al., 2014; Bragina et al., 2019; Kilgo et al., 2019). Even in the absence of predators, early fawn mortality may reach 50% without causing deer populations to decline (Dion et al., 2020).
Can wolves and other predators keep deer populations at low abundance? We cannot reject this possibility, but evidence for such effects is limited (Blossey and Hare, 2022). Instead, wolf abundance itself may be a function of available prey biomass (mostly ungulates, but also beavers, rodents and fruit) and their social structure (Pimlott, 1967; Fuller, 1989; Neufeld et al., 2021). Half a century ago, Pimlott (1967) argued that wolves would be unable to control (i.e. reduce) deer populations where deer abundance exceeds 7–8/km2, a density exceeded over much of the U.S. today (Williams et al., 2013). For example, New York’s deer population is about 1.2 million; annually hunters kill about 220,000 and cars 70,000. Managers typically consider removing about 40% of adult does sufficient to stabilize a herd but up to 60% may need to be removed annually to cause population declines (Matschke et al., 1984; Merrill et al., 2003). Unfortunately, the vast majority of hunters continue to prefer to shoot antlered males — in 2022 only 12% of New York hunters shot an antlerless deer (this includes male fawns) and only 4% shot more than two antlerless deer antlerless deer despite antlerless tags being available, (https://content.govdelivery.com/accounts/NYSDEC/bulletins/3766de2). Even if we assume that half of the car and hunter killed deer in New York are females, this annual mortality removes only 20% of the doe population in the state, half of what would be required for herd stabilization and much less than required to induce a population decline. Nationwide, hunter success varies widely by state and region but typically <50% of license holders actually shoot a deer (https://deerassociation.com/2024-deer-report/). Individual adult wolves consume about 20 deer/year (Fuller, 1989). For wolves to aid in stabilizing or help begin herd reductions in New York, they would need to kill at least as many deer as hunters and cars combined, should they return. This would require >20,000 wolves, more than currently occur in the entire U.S. (including Alaska). This is neither biologically feasible, nor socially acceptable, and not unique to New York.
Hope for enabling predator dispersal, or reintroduction efforts, to help manage local deer herds faces insurmountable ecological and social problems. Wolves or other large predators might help maintain deer populations once they decline to lower levels, but this is, at present, a distant possibility. It is our opinion that wolves should be allowed to return to their traditional range in the eastern U.S. because they are keystone species, but not because we expect them to manage deer populations for us.
If natural and human hunters do not limit deer populations, what does? The role of quantity and quality of nutrition is critical but often overlooked. Large winter mortalities in the 1930’s–1950’s were linked to starvation with deer having exhausted their food supplies (Matschke et al., 1984). This becomes particularly important in the northern portions of the deer range with longer, colder winters and deep snow (Van Deelen et al., 2010; Olson et al., 2021). Climate change, however, is rapidly reducing winter severity and large winter kill events across North America. Furthermore, females under nutrient stress have lower fecundity, abort fetuses, and low birthweights increase early fawn mortality (Verme and Ullrey, 1984; Dion et al., 2020). This central role of nutrition in determining population dynamics occurs in other cervids including woodland caribou (Schaefer et al., 2016), moose (Jesmerr et al., 2021; Oates et al., 2021), mule deer (Bishop et al., 2009), and elk (Coughenour and Singer, 1996; Lukacs et al., 2018), but is rarely considered of importance in deer management. Before European settlement, deer populations were likely food limited in the uncut contiguous forests of the eastern U.S. Populations were only as large as could be sustained during the most nutritionally limiting season. Fall masting events of American chestnut (Castanea dentata), American beech (Fagus grandifolia) and oaks (Quercus spp.) likely provided nutritional supplies for deer to survive winters — deer were mostly absent in the conifer forests around the Great Lakes, for example, until forests were cut and hardwoods regrew (Rogers et al., 1981). Spring wildflowers, now scarce in many eastern woodlands, could probably persist previously at high abundances and tolerate the lower browse pressure (like mast fruiting events in trees that overwhelm seed predators). Agriculture, forestry operations, gardens, and supplemental feeding now subsidize deer nutrition through much of the year, elevating deer populations and browse pressure that may have led to the demise of the wildflowers we no longer see today.
Current deer management responsibilities in the United States
Responsibilities for conservation and stewardship of species and their habitats in the U.S. are scattered depending on ownership (federal, state, tribal, and private), taxon (plants, invertebrates, vertebrates), and status (i.e., migratory or not; endangered, threatened or not; wildlife with a hunting, fishing, or trapping season or not, etc.). The federal government owns nearly 30% of the terrestrial surface area of the U.S. (Vincent et al., 2020) overseen and managed by the National Park Service (NPS; National Parks and Monuments), the U.S. Fish and Wildlife Service (USFWS; National Wildlife Refuges), the Bureau of Land Management (BLM; grazing lands, wilderness areas, National Monuments, and National Conservation Lands including Wild and Scenic Rivers and National Scenic and Historic Trails), the U.S. Forest Service (USFS; national grasslands, national forests and wilderness areas), and the Department of Defense (DoD; military installations). Federal lands provide major habitats for many species listed under the Endangered Species Act (ESA) and the vast majority of elk, mountain goat (Oreamnos americanus) and bighorn sheep (Ovis canadensis) habitat (Williamson, 2011). High populations of native and introduced ungulates can create major ecological and management problems in many National Parks and an updated policy indicates that abundance reductions of 50–90% in native elk but particularly for white-tailed deer are necessary to protect park resources (Plumb et al., 2014). The National Park Service is actively engaged in ungulate management using contractors (hunting or trapping is not allowed in U.S. National Parks, but fishing is, unless specifically outlawed in individual parks), for example in Old Forge (https://parkplanning.nps.gov/document.cfm?documentID=29135) or Gettysburg (https://www.nps.gov/gett/learn/news/deer-management-22-23.htm) The authority to manage wildlife on federal lands has repeatedly been challenged by states, claiming their constitutional and legal authority includes federal lands within their jurisdiction. We do not have the room to explore these arguments in detail here but refer readers to a comprehensive analysis of constitutional and legal arrangements for land and wildlife management. Nie et al. (2017) review how courts have repeatedly interpreted the legal and constitutional arrangements rejecting state supremacy claims for federal lands. They summarize their findings as follows:
‘Federal land management agencies have an obligation, and not just the discretion, to manage and conserve fish and wildlife on federal lands. We debunk the myth that “the states manage wildlife and federal land agencies only manage wildlife habitat.” The myth is not only wrong from a legal standpoint, but it leads to fragmented approaches to wildlife conservation, unproductive battles over agency turf, and an abdication of federal responsibility over wildlife. Another problem exposed is how the states assert wildlife ownership to challenge the constitutional powers, federal land laws, and supremacy of the United States. While the states do have a responsibility to manage wildlife as a sovereign trust for the benefit of their citizens, most states have not addressed the conservation obligations inherent in trust management; rather, states wish to use the notion of sovereign ownership as a one way ratchet — a source of unilateral power but not of public responsibility. Furthermore, the states’ trust responsibilities for wildlife are subordinate to the federal government’s statutory and trust obligations over federal lands and their integral resources.’
States and State Wildlife Agencies (SWAs) have well established historical responsibilities over wildlife management within their borders and federal agencies have traditionally deferred to the states regarding hunting, fishing, or trapping seasons and bag limits. But managing wildlife (typically defined as mammals, birds and fish) as a public trust resources for all citizens is an obligation that goes well beyond establishing hunting seasons or methods (Nie et al., 2017). When SWAs were established in the early 20th century, their main focus was to help recover deer populations (Halls, 1984) as well as populations of other huntable species that had declined due commercial and recreational overexploitation. Funding for these efforts was largely derived from hunting and fishing licenses and SWAs have maintained a focus on consumptive uses and sustaining high deer populations for recreational hunting (Jacobson et al., 2007; Freyfogle and Goble, 2009). This historic association of SWAs with consumptive users of wildlife led to the articulation of the North American Model of Wildlife Conservation, NAMWC (Mahoney and Geist, 2019), leading to claims that hunting is conservation. This claim continues to be perpetuated by hunting advocates, their organizations and SWAs (https://www.rmef.org/hunting-is-conservation/).
However, only a small proportion of U.S. residents (typically <10%) hunts, and participation is declining while participation in other non-consumptive outdoor activities is increasing (U.S. Fish and Wildlife Service, 2018). Hunters, their advocacy, and funding was important in advancing conservation of many species and these contribution are widely recognized, but the continued promotion, breeding and release for example of non-native species such as European brown trout (Salmo trutta) and ring necked pheasant (Phasianus colchicus) constitute threats to many native species and cannot be considered to advance conservation (Bixby, 2020). Furthermore, the narrow focus on consumptive uses as the cornerstone of conservation explicit in the NAMWC has come under strong criticism as marginalizing the more widespread public interest in non-consumptive uses and conservation of species without hunting or fishing seasons (Feldpausch-Parker et al., 2017; Bixby, 2020). The policies, practices, and values of SWAs and their mostly white and male employees thus have failed to adjust to changing social values (Sullivan et al., 2022), prohibiting bridge building to other environmental conservation groups (Feldpausch-Parker et al., 2017). Consequently, SWAs fail to deliver outcomes that satisfy societal expectations for healthy and diverse environments (Hare et al., 2017; Manfredo et al., 2021), leading to calls for agency reform (Jacobson et al., 2010; Decker et al., 2016).
Although most SWAs do not have authority to manage plants or invertebrates such as molluscs, these are among the taxa with the most significant declines and risk of extinction. Of the >1,300 species listed under the Endangered Species Act in the U.S. 942 are plants and 287 are invertebrates (ESA; https://www.epa.gov/endangered-species/endangered-species-species-information-factsheets). Furthermore, how can SWAs achieve better conservation and human health outcomes when their core responsibilities remain restricted to wildlife? Public trust obligations expand SWAs responsibilities beyond wildlife, but their legal authority is limited, even if SWAs were willing to embrace fundamental change. SWAs have no legal authority in health care, agriculture, transportation, or across state boundaries. Even within SWAs, responsibilities for wildlife, forestry, or conservation often rest in different units.
When SWAs were established, it made sense to give them authority to develop appropriate management of wildlife according to differences in habitats and wildlife species across the nation. Today deer populations that exceed ecological carrying capacities are widespread across different regions and habitats, although the severity may differ. The currently fragmented ‘landscape’ of deer management has failed to deliver a healthy future for wildlife and humans alike. Federal leadership to develop an improved and collaborative approach to deer management while recognizing local, state, tribal, and regional stakeholders appears essential to fulfill federal and state public trust obligations. This will need to include development of clearly articulated responsibilities across sectors, metrics to assess goals and outcome of management, and increased funding to overcome current inertia and constraints to address the breadth and severity of deer impacts across the nation.
The need for a national deer management strategy
Without substantial changes to deer management, the U.S. will be unable to fulfill its obligations under international treaties on climate change, invasive and endangered species, and national priorities to protect the nation’s biodiversity and forest resources. The >1,300 species listed under the ESA are already a federal responsibility, although they occur on federal, state, tribal, and private lands. Only collaborative efforts (led by federal authority) stand a chance to safeguard their future existence. Of the >700 bird species that breed in North America, >50% are Neotropical migrants and thus also fall under federal management authority. Deer strongly influence songbird community structure by thinning ground cover and low shrubs and saplings, reducing foraging habitat quality and insect abundance (Nuttle et al., 2014; Chips et al., 2015; Rushing et al., 2020; Phillips and Cristol, 2024). Dramatic declines in bird and insect abundance have raised alarm (Rosenberg et al., 2019; van Klink et al., 2023). While many factors play a role, the available evidence points to a strong contributing factor of high deer abundance, particularly in forest habitats.
Without changes in deer management that can successfully reduce deer populations where needed, the ability of agencies to find solutions in nature to restore and conserve the nation’s forests to provide habitat and sequester carbon, as outlined in Executive Orders 13990 and 14072 (The White House, 2021, 2022), will be severely curtailed. Thriving forests require that trees that are cut or die be replaced by a diversity of new recruits. Tree diversity increases forest carbon sequestration potential, but deer limit, or even prevent, diverse forest regeneration across the nation (Reed et al., 2022; Miller et al., 2023). At current densities, deer are making this climate change adaptation strategy in the U.S. difficult, if not impossible. Detrimental deer impacts will come into sharper focus with the development of environmental-economic statistics to quantify and enhance the value of natural assets (The White House, 2023). Maintaining or enhancing the values of the nation’s natural assets will require effective deer management as the staus quo continues to erode asset values. These national problems require a National Strategy.
Our call for federal leadership in deer management is not unique — federal leadership for issues or problems of national or regional scope are commonplace. Federal leadership, coordination (and funding) has tackled many agricultural pests and human diseases (Wyss, 2006). For example, screwworms, the flesh-eating larvae of the fly Cochliomyia hominivorax, a species native to the southern U.S., once caused widespread problems in cattle, wild animals, and the occasional human from Florida to California. Rather than leaving this as a problem for states or individual ranchers to solve, federal leadership with state and international partners mass reared and released sterile males and eradicated this fly from North and Central America (Zhang, 2020). New accidental introductions, such as recently in Florida, are quickly and effectively dealt with. The program requires continued releases to maintain flies south of an invisible border in Panama (Zhang, 2020). Similar federal, state, and local partnerships eradicated malaria and yellow fever from North America. Unfortunately, such leadership has not yet materialized for tick-borne diseases, and is cited as a major problem preventing an effective response (Rochlin et al., 2019; Eisen, 2020).
The North American Waterfowl Management Plan (https://www.fws.gov/birds/management/project-assessment-tools-and-guidance.php) provides a precedent for wildlife management decisions across ecological and political boundaries and collaborative efforts involving federal, tribal and state cooperation. The U.S. Fish and Wildlife Service partners with tribal governments, Mexico and Canada to conduct annual aerial monitoring of waterfowl breeding grounds to collect information on population sizes, recruitment, and to calculate acceptable harvest recommendations. States are assigned quotas and implement their own regulations and seasons. Waterfowl hunters are required to purchase Duck Stamps annually to fund these monitoring efforts. This has worked well for most species with waterfowl one of the few bird groups not showing dramatic recent declines (Rosenberg et al., 2019). Similar ambitious National Strategies are developed in the National Feral Camel Action Plan in Australia (Woolnough et al., 2016), New Zealand’s Predator Free 2050 Strategy (New Zealand Department of Conservation, 2023), and are proposed to help address deleterious kangaroo impacts in Australia (Read et al., 2021).
A National Strategy should be developed collaboratively with the aim to reduce adverse human and wildlife health impacts associated with high deer abundance. Conservation and climate change adaptation does not happen on a state-by-state basis, and neither can conservation and health inspired deer management — federal leadership appears to be essential given the current impasse. Congress or the White House could initiate the development of a National Strategy by establishing a commission or council. Developing a National Strategy needs to be an iterative, inclusive and participatory process sensitive to local socio-cultural and ecological conditions. Historically underserved and marginalized residents such as Black, Indigenous, and People of Color, and diverse non-consumptive advocacy groups will need representation in addition to experts integrating technical and policy expertise from conservation, wildlife, forestry, agriculture, economic development, and public health. Hunters, ranchers and animal welfare groups need to be part of the deliberations but their currently outsized influence on wildlife management decisions (Pomeranz and Decker, 2017) needs to be curtailed.
Reducing deer populations and deer impact at regional scales will likely require lethal control as other means (sterilization, fertility control) have failed to deliver long-term benefits even when paired with recreational hunting (Blossey et al., 2019). Many examples of successful lethal control reducing local deer populations exist including in Rock Creek National Park (https://www.nps.gov/rocr/learn/management/white-tailed-deer-management.htm) in the U.S. capitol, and in the small village of Cayuga Heights in central New York (https://cayuga-heights.ny.us/projects-2/deer-management/). Good political leadership, funding and endurance can overcome well-funded and organized opposition (Sterba, 2012), but isolated local efforts cannot reduce deer populations at regional scales. Public support for such efforts may be strong. For example, over 70% of respondents in a New York State survey supported lethal deer management when justified to protect human health, plants, or animals (Blossey et al., 2024). This support was equally strong among different racial and ethnic groups, rural and urban residents, and regardless of whether respondents self-identified as hunters, animal protectionists, or conservationists. This widespread consensus among the general public provides a solid foundation to develop and implement a National Strategy.
Implementing a National Strategy will benefit all members of society and will likely require additional resources. Neither implementation, nor financing of this strategy, should be the responsibility of recreational hunters. SWAs have experimented with many different approaches to increase participation in hunting and encourage removal of additional females including Earn-a-Buck (where hunters must take a female before males can be shot), nearly unlimited antlerless deer tags, special tags to reduce deer damage, extending seasons, and approving new weapons. None of these have made much difference in improving forest health (Blossey et al., 2019; Nagy et al., 2022). Many municipalities use external federal or private contractors and the USDA’s Wildlife Services program may offer important financial and technical resources. However, we still lack an effective ‘blueprint’ to reduce deer impacts at the landscape scale. The development of a bounty system, or regulated market hunting (Vercauteren et al., 2011), plus other potential alternatives, need to be evaluated for their ability to deliver the desired improvements in human and environmental health. This will require additional research and experimentation.
The importance of evidence and assessment of outcomes
The Evidence-Based Policymaking Act of 2018 recognizes that federal decision makers need evidence about whether federal programs achieve intended results (Government Accountability Office, 2021). Unfortunately, methodologies to track how policy decisions impact outcomes are poorly developed and agency capacity (staff, expertise) is often limited (Government Accountability Office, 2021). The development of a National Strategy to reduce deer impacts will face similar issues. Despite a long history of research documenting deer impacts, we still lack scientifically validated and standardized methods to track deer impacts on human and environmental health at appropriate spatial and temporal scales (Quirion and Blossey, 2023). Deer management is further entangled in value systems, financial interests, and emotions of different interest groups (Sterba, 2012). To overcome these obstacles, a National Strategy should develop goals and metrics that articulate environmental and human health outcomes, and not only focus on local deer abundance to reduce conflicts.
Assessing whether current deer management is delivering beneficial outcomes for people and the environment requires development and validation of both rapid response and long-term monitoring metrics (social, human health, ecological and financial). Important advancements assessing ecological impacts have been made recently (Waller et al., 2017; Quirion and Blossey, 2023). Metrics able to gauge immediate monthly or annual impacts, for example of large winter kills, regulated market hunting, culling operations, or diseases on human and environmental health are essential. Management agencies require, and residents and interest groups will demand, this rapid-response capability to maintain accountability and the ability to quickly adjust management means. Exclosure studies, plant monitoring, and the Forest Inventory and Analysis (FIA) program can all deliver information on long-term changes over decades and centuries (Patton et al., 2018; Miller et al., 2023), but are unable to deliver rapid annual or monthly response metrics (Nuzzo et al., 2017; Knauer et al., 2023).
A National Strategy could specify metrics, benchmarks, and deliverable goals for states to meet to remain eligible for federal funding. Federal funding derived from Pittmann-Robertson (excise tax on guns, ammunition, and archery equipment) and Dingell-Johnson Acts (excise taxes fishing tackle, boats and small engine fuel) already provides important funding for SWAs (approx. 25% of annual budgets). The currently pending bipartisan Recovering America’s Wildlife Act (RAWA) would dedicate >$1 billion to conservation efforts led by states, tribes, and territories (Branch et al., 2022) and aims to use these resources towards proactive conservation, and keeping species from being listed under the Endangered Species Act (ESA). Reducing deer herds will be essential to safeguard many species whose populations are currently declining, and thus aligns well with the ambitions outlined in RAWA. This ‘power of the purse’ linking performance of state deer management and agreed upon metrics, to federal funding eligibility is a cornerstone of other management programs. Those appreciating non-consumptive uses of the nation’s fields and forests and the beauty of wildflowers, pollinators and birds should also be asked for additional contribution to fund deer management to enhance conservation efforts, even beyond RAWA. Potential funding streams include surcharges on recreational and commercial use of wildlife and habitats by bikers, bird watchers, boaters, climbers, hikers, hunters, motorized vehicle users, photographers, and skiers, as well as the tourism, film, and TV industries. This diversified funding model should replace and enhance the outdated ‘user-pay, user-benefit’ funding model that has privileged consumptive users and their interests, while ignoring conservation efforts targeting the vast majority of biodiversity. It may also help uncouple SWAs from their emphases on consumptive users while providing increased and more stable resources without privileging particular interests.
Conclusion: a moral imperative to address the deer crisis
We propose federal leadership to develop a National Strategy to improve human and environmental health that will likely require significant reductions in deer populations over large areas. How these reductions are achieved and how to structure and fund collaborative efforts among federal, state, tribal, and other interests need careful thought and development. A commission or task force could develop and test how best to reduce deer populations and measure impacts. They could also recommend how best to assign responsibilities among federal, state, tribal, and private land owners, agencies, and managers and what additional legislation might be necessary. Current institutional arrangements are fragmented among state and federal agencies, preventing them from being able to develop forward-looking solutions to limit and reduce deer impacts. Current institutional arrangements are fragmented among state and federal agencies, preventing them from being able to develop forward-looking solutions to limit and reduce deer impacts. Coordinated killing could be emotionally distressing and morally difficult for some individuals, but we also find widespread public support for such efforts, as long as this is done to improve human and environmental health outcomes (Hare et al., 2021; Blossey et al., 2024). We emphasize our moral responsibilities to humans and other species reliant on vibrant and diverse ecosystems. Understory plants, tree seedlings, songbirds, amphibians, insects, and many mammals are all negatively impacted by high deer densities. Our emotional connections to these organisms — as well as our scientific understanding — drive our recommendations. We do not make our proposals for lethal means lightly — but we are not willing to leave the killing to cars, disease, and starvation. The return of large predators, where feasible, will not absolve us of our responsibilities of trying to correct problems we created in the first place. Doing nothing to address the deer crisis will condemn many organisms to continued suffering, death and sometimes local or global extinction (Figures 1, 2).
Author contributions
BB: Conceptualization, Visualization, Writing – original draft, Writing – review & editing. DH: Conceptualization, Writing – review & editing. DW: Conceptualization, Writing – review & editing.
Funding
The author(s) declare that no financial support was received for the research, authorship, and/or publication of this article.
Acknowledgments
Comments from the Blossey lab group, Victoria Nuzzo, Eli Arnow, Avalon Bunge and two reviewers improved earlier versions of this manuscript. Sarah Markes assisted with figure designs.
Conflict of interest
The authors declare that the research was conducted in the absence of any commercial or financial relationships that could be construed as a potential conflict of interest.
Publisher’s note
All claims expressed in this article are solely those of the authors and do not necessarily represent those of their affiliated organizations, or those of the publisher, the editors and the reviewers. Any product that may be evaluated in this article, or claim that may be made by its manufacturer, is not guaranteed or endorsed by the publisher.
References
Alleway H. K., Klein E. S., Cameron L., Douglass K., Govia I., Guell C., et al. (2023). The shifting baseline syndrome as a connective concept for more informed and just responses to global environmental change. People Nat. 5, 885–896. doi: 10.1002/pan3.10473
Alverson W. S., Lea M. V., Waller D. M. (2019). A 20-year experiment on the effects of deer and hare on eastern hemlock regeneration. Can. J. For. Res. 49, 1329–1338. doi: 10.1139/cjfr-2019-0071
Binkley D., Moore M. M., Romme W. H., Brown P. M. (2006). Was Aldo Leopold right about the Kaibab deer herd? Ecosystems 9, 227–241. doi: 10.1007/s10021-005-0100-z
Bishop C. J., White G. C., Freddy D. J., Watkins B. E., Stephenson T. R. (2009). Effect of enhanced nutrition on mule deer population rate of change. Wildl. Monogr. 172, 1–28. doi: 10.2193/2008-107
Bixby K. (2020) Why hunting isn’t conservation, and why it matters. Available online at: https://rewilding.org/hunting-isnt-conservation/.
Blossey B., Brice E., Dalaba J., Hare D. (2024). When dogma meets reality: perspectives of New York State residents to deer management, hunting, and predator reintroductions. Sci. Rep.
Blossey B., Curtis P., Boulanger J., Dávalos A. (2019). Red oak seedlings as bioindicators to assess browsing pressure and efficacy of white-tailed deer management. Ecol. Evol. 9, 13085–13103. doi: 10.1002/ece3.5729
Blossey B., Hare D. (2022). Myths, wishful thinking and accountability in predator conservation and management in the United States. Front. Conserv. Sci. 3. doi: 10.3389/fcosc.2022.881483
Bragina E. V., Kays R., Hody A., Moorman C. E., Deperno C. S., Mills L. S. (2019). Effects on white-tailed deer following eastern coyote colonization. J. Wildl. Manage. 83, 916–924. doi: 10.1002/jwmg.21651
Branch J. V., Karlen J., Organ J., Bishop C., Mitchell M., Regan R., et al. (2022). Echoes of 1937: Recovering America’s Wildlife Act would bring wildlife conservation funding full circle. Conserv. Lett. e12890. doi: 10.1111/conl.12890
Brice E. M., Larsen E. J., MacNulty D. R. (2022). Nonrandom sampling and untested assumptions exaggerate the strength of a classic trophic cascade. Ecol. Lett. 25, 177–188. doi: 10.1111/ele.13915
Chips M. J., Yerger E. H., Hervanek A., Nuttle T., Royo A. A., Pruitt J. N., et al. (2015). The indirect impact of long-term overbrowsing on insects in the Allegheny National Forest region of Pennsylvania. Northeast. Nat. 22, 782–797. doi: 10.1656/045.022.0412
Chollet S., Baltzinger C., Maillard M., Martin J. L. (2021). Deer exclusion unveils abiotic filtering in forest understorey plant assemblages. Ann. Bot. 128, 371–381. doi: 10.1093/aob/mcab079
Civitello D. J., Cohen J., Fatima H., Halstead N. T., Liriano J., McMahon T. A., et al. (2015). Biodiversity inhibits parasites: broad evidence for the dilution effect. Proc. Natl. Acad. Sci. 112, 8667–8671. doi: 10.1073/pnas.1506279112
Comisky L., Royo A. A., Carson W. P. (2005). Deer browsing creates rock refugia gardens on large boulders in the Allegheny National Forest, Pennsylvania. Am. Midland Nat. 154, 201–206. doi: 10.1674/0003-0031(2005)154[0201:DBCRRG]2.0.CO;2
Conover M. R. (2002). Resolving Human-wildlife Conflicts: The Science of Wildlife Damage Management (Boca Raton, Florida, US: Lewis Publishers).
Côté S. D., Rooney T. P., Tremblay J.-P., Dussault C., Waller D. M. (2004). Ecological impacts of deer overabundance. Annu. Rev. Ecol. System. 35, 113–147. doi: 10.1146/annurev.ecolsys.35.021103.105725
Coughenour M. B., Singer F. J. (1996). Elk population processes in Yellowstone National Park under the policy of natural regulation. Ecol. Appl. 6, 573–593. doi: 10.2307/2269393
Cunningham C. X., Nuñez T. A., Hentati Y., Sullender B., Breen C., Ganz T. R., et al. (2022). Permanent daylight saving time would reduce deer-vehicle collisions. Curr. Biol. 32, 4982–4988. doi: 10.1016/j.cub.2022.10.007
Dávalos A., Nuzzo V., Blossey B. (2015a). Single and interactive effects of deer and earthworms on non-native plants. For. Ecol. Manage. 351, 28–35. doi: 10.1016/j.foreco.2015.04.026
Dávalos A., Simpson E., Nuzzo V., Blossey B. (2015b). Non-consumptive effects of native deer on introduced earthworm abundance. Ecosystems 18, 1029–1042. doi: 10.1007/s10021-015-9881-x
Decker D., Smith C., Forstchen A., Hare D., Pomeranz E., Doyle-Capitman C., et al. (2016). Governance principles for wildlife conservation in the 21st century. Conserv. Lett. 9, 290–295. doi: 10.1111/conl.2016.9.issue-4
Dion J. R., Haus J. M., Rogerson J. E., Bowman J. L. (2020). White-tailed deer neonate survival in the absence of predators. Ecosphere 1, e03122. doi: 10.1002/ecs2.3122
Dobson A., Bowe A., Nuzzo V., Dávalos A., Fahey T., Blossey B. (2024). Individual and combined effects of invasive earthworms and native white-tailed deer on understorey plant survival, growth and reproduction. J. Ecol. 00, 1–19. doi: 10.1111/1365-2745.14285
Eisen L. (2020). Stemming the rising tide of human-biting ticks and tickborne diseases, United States. Emerg. Infect. Dis. 26, 641–647. doi: 10.3201/eid2604.191629
Escobar L. E., Pritzkow S., Winter S. N., Grear D. A., Kirchgessner M. S., Dominguez-Villegas E., et al. (2020). The ecology of chronic wasting disease in wildlife. Biol. Rev. 95, 393–408. doi: 10.1111/brv.12568
Estes J. A., Terborgh J., Brashares J. S., Power M. E., Berger J., Bond W. J., et al. (2011). Trophic downgrading of planet earth. Science 333, 301–306. doi: 10.1126/science.1205106
Feldpausch-Parker A. M., Parker I. D., Vidon E. S. (2017). Privileging consumptive use: A critique of ideology, power, and discourse in the North American model of wildlife conservation. Conserv. Soc. 15, 33–40. doi: 10.4103/0972-4923.201395
Flader S. (1974). Thinking like a mountain. Aldo Leopold and the evolution of an ecological attitude toward deer, wolves and forests (Madison, Wisconsin, USA: University of Wisconsin Press).
Flaherty K. L., Grafton W. N., Anderson J. T. (2018). White-tailed deer florivory influences the population demography of Polemonium vanbruntiae. Plant Biosyst. 152, 453–463. doi: 10.1080/11263504.2017.1305999
Fleming P. J. S. (2019). They might be right, but Beschta et al. (2018) give no strong evidence that ‘trophic cascades shape recovery of young aspen in Yellowstone National Park’: A fundamental critique of methods. For. Ecol. Manage. 454. doi: 10.1016/j.foreco.2019.04.011
Ford A. T., Goheen J. R. (2015). Trophic cascades by large carnivores: A case for strong inference and mechanism. Trends Ecol. Evol. 30, 725–735. doi: 10.1016/j.tree.2015.09.012
Foster D. R., Motzkin G., Bernardos D., Cardoza J. (2002). Wildlife dynamics in the changing New England landscape. J. Biogeogr. 29, 1337–1357. doi: 10.1046/j.1365-2699.2002.00759.x
Frerker K., Sabo A., Waller D. (2014). Long-term regional shifts in plant community composition are largely explained by local deer impact experiments. PloS One 9, e115843. doi: 10.1371/journal.pone.0115843
Fuller T. K. (1989). Population dynamics of wolves in north-central Minnesota. Wildl. Monogr. 105), 1–41. Available at: https://www.jstor.org/stable/3830614
Gill J. L., Williams J. W., Jackson S. T., Lininger K. B., Robinson G. S. (2009). Pleistocene megafaunal collapse, novel plant communities, and enhanced fire regimes in North America. Science 326, 1100–1103. doi: 10.1126/science.1179504
Goethert H. K., Telford S. R. (2022). Host contributions to the force of Borrelia burgdorferi and Babesia microti transmission differ at edges of and within a small habitat patch. Appl. Environ. Microbiol. 8 (6), e02391–21. doi: 10.1128/aem.02391-21
Goetsch C., Wigg J., Royo A. A., Ristau T. E., Carson W. P. (2011). Chronic over browsing and biodiversity collapse in a forest under- story in Pennsylvania: results from a 60 year-old deer exclusion plot. J. Torrey Bot. Soc. 138, 220–224. doi: 10.3159/TORREY-D-11-00013.1
Gorchov D. L., Blossey B., Averill K. M., Dávalos A., Heberling J. M., Jenkins M. A., et al. (2021). Differential and interacting impacts of invasive plants and white-tailed deer in eastern U.S. forests. Biol. Invasions 23. doi: 10.1007/s10530-021-02551-2
Government Accountability Office (2021). Evidence-based policymaking (Washington, D.C: U.S.G.A. Office).
Halls L. K. (1984). White-tailed Deer: Ecology and Management (Harrisburg, Pennsylvania, USA: Stackpole Books).
Hare D., Daniels M., Blossey B. (2021). Public perceptions of deer management in Scotland. Front. Conserv. Sci. 2. doi: 10.3389/fcosc.2021.781546
Hare D., Decker D., Smith C. A., Forstchen A., Jacobson C. A. (2017). Applying public trust thinking to wildlife governance in the United States: challenges and potential solutions. Hum. Dimensions Wildl. 22, 506–523. doi: 10.1080/10871209.2017.1359864
Hody J. W., Kays R. (2018). Mapping the expansion of coyotes (Canis latrans) across North and Central America. ZooKeys 759, 81–97. doi: 10.3897/zookeys.759.15149
Horsley S. B., Stout S. L., DeCalesta D. S. (2003). White-tailed deer impact on the vegetation dynamics of a northern hardwood forest. Ecol. Appl. 13, 98–118. doi: 10.1890/1051-0761(2003)013[0098:WTDIOT]2.0.CO;2
Jacobson C. A., Decker D. J., Carpenter L. (2007). Securing alternative funding for wildlife management: insights from agency leaders. J. Wildl. Manage. 71, 2106–2113. doi: 10.2193/2006-442
Jacobson C. A., Organ J. F., Decker D. J., Batcheller G. R., Carpenter L. (2010). A conservation institution for the 21st century: implications for state wildlife agencies. J. Wildl. Manage. 74, 203–209. doi: 10.2193/2008-485
Jennelle C. S., Henaux V., Wasserberg G., Thiagarajan B., Rolley R. E., Samuel M. D. (2014). Transmission of chronic wasting disease in Wisconsin white-tailed deer: implications for disease spread and management. PloS One 9, e91043. doi: 10.1371/journal.pone.0091043
Jesmerr B. R., Kauffman M. J., Courtemanch A. B., Kilpatrick S., Thomas T., Yost J., et al. (2021). Life-history theory provides a framework for detecting resource limitation: a test of the nutritional buffer hypothesis. Ecol. Appl. 31, e02299. doi: 10.1002/eap.2299
Keesing F., Ostfeld R. S. (2015). Is biodiversity good for your health? Science 349, 235–236. doi: 10.1126/science.aac7892
Kelly J. F. (2019). Regional changes to forest understories since the mid-twentieth century: effects of overabundant deer and other factors in northern New Jersey. For. Ecol. Manage. 444, 151–162. doi: 10.1016/j.foreco.2019.04.050
Kilgo J. C., Cherry M. J., Ditchkoff S. S., Gulsby W. D., Miller K. V. (2019). Coyotes and white-tailed deer populations in the east: A comment on Bragina et al., (2019). J. Wildl. Manage. 83, 1636–1640. doi: 10.1002/jwmg.21735
Kilpatrick A. M., Dobson A. D. M., Levi T., Salkeld D. J., Swei A., Ginsberg H. S., et al. (2017). Lyme disease ecology in a changing world: consensus, uncertainty and critical gaps for improving control. Philos. Trans. R. Soc. B-Biol. Sci. 372. doi: 10.1098/rstb.2016.0117
Kilpatrick H. J., LaBonte A. M., Stafford K. C. (2014). The relationship between deer density, tick abundance, and human cases of Lyme disease in a residential community. J. Med. Entomol. 51, 777–784. doi: 10.1603/ME13232
Knapp W. M., Wiegand R. (2014). Orchid (Orchidaceae) decline in the Catoctin Mountains, Frederick County, Maryland as documented by a long-term dataset. Biodivers. Conserv. 23, 1965–1976. doi: 10.1007/s10531-014-0698-2
Knauer A., Betras T., Royo A. A., Diggins T. P., Carson W. P. (2023). Understory plant communities fail to recover species diversity after excluding deer for nearly 20 years. Can. J. For. Res. 53, 379–390. doi: 10.1139/cjfr-2022-0234
Knight T. M., Caswell H., Kalisz S. (2009). Population growth rate of a common understory herb decreases non-linearly across a gradient of deer herbivory. For. Ecol. Manage. 257, 1095–1103. doi: 10.1016/j.foreco.2008.11.018
Kovacs I., Toth M., Schally G., Csanyi S., Bleier N. (2020). The assessment of wildlife damage estimation methods in maize with simulation in GIS environment. Crop Prot. 127. doi: 10.1016/j.cropro.2019.104971
Lankau R. A. (2011). Rapid evolutionary change and the coexistence of species. Annu. Rev. Ecol. Evol. System. 42, 335–354. doi: 10.1146/annurev-ecolsys-102710-145100
Leopold A., Sowls L. K., Spencer D. L. (1947). A survey of over-populated deer ranges in the United States. J. Wildl. Manage. 11, 162–177. doi: 10.2307/3795561
Loss S. R., Blair R. B. (2011). Reduced density and nest survival of ground-nesting songbirds relative to earthworm invasions in northern hardwood forests. Conserv. Biol. 25, 983–992. doi: 10.1111/j.1523-1739.2011.01719.x
Lukacs P. M., Mitchell M. S., Hebblewhite M., Johnson B. K., Johnson H., Kauffman M., et al. (2018). Factors influencing elk recruitment across ecotypes in the Western United States. J. Wildl. Manage. 82, 698–710. doi: 10.1002/jwmg.21438
Maerz J. C., Nuzzo V. A., Blossey B. (2009). Declines in woodland salamander abundance associated with non-native earthworm and plant invasions. Conserv. Biol. 23, 975–981. doi: 10.1111/j.1523-1739.2009.01167.x
Mahoney S. P., Geist V. (2019). The North American Model of Wildlife Conservation (Baltimore, Maryland, USA: Johns Hopkins University Press).
Mallapaty S. (2022). Could deer become a natural Covid reservoir? Nature 604, 612–615. doi: 10.1038/d41586-022-01112-4
Maloney M., Merkle J. A., Aadland D., Peck D., Horan R. D., Monteith K. L., et al. (2020). Chronic wasting disease undermines efforts to control the spread of brucellosis in the Greater Yellowstone Ecosystem. Ecol. Appl. 30, e02129. doi: 10.1002/eap.2129
Manfredo M. J., Teel T. L., Berl R. E., Bruskotter J. T., Kitayama S. (2021). Social value shift in favour of biodiversity conservation in the United States. Nat. Sustainabil. 4, 323–330. doi: 10.1038/s41893-020-00655-6
Martin T. G., Arcese P., Scheerder N. (2011). Browsing down our natural heritage: Deer impacts on vegetation structure and songbird populations across an island archipelago. Biol. Conserv. 144, 459–469. doi: 10.1016/j.biocon.2010.09.033
Matschke G. H., Fagerstone K. A., Harlow R. F., Hayes F. A., Nettles V. F., Parker W., et al. (1984). “Population influences,” in White-Tailed Deer: Ecology and Management. Ed. Halls L. K. (Stackpole Books, Harrisburg, Pennsylvania, USA), 169–188.
McCabe R. E., McCabe T. R. (1984). “Of slings and arrows: an historical retrospection,” in White-Tailed Deer: Ecology and Management. Ed. Halls L. K. (Stackpole Books, Harrisburg, Pennsylvania, USA), 19–72.
McGraw J. B., Furedi M. A. (2005). Deer browsing and population viability of a forest understory plant. Science 307, 920–922. doi: 10.1126/science.1107036
McNulty D. R., Stahler D. R., Wyman T., Ruprecht J., Smith L. M., Kohl M. T., et al. (2020). “Population dynamics of Northern Yellowstone elk after wolf reintroduction,” in Yellowstone Wolves. Science and Discovery in the Wold’s First National Park. Eds. Smith D. W., Stahler D. R., MacNulty D. R. (University of Chicago Press, Chicago, US), 184–199.
Merrill J. A., Cooch E. G., Curtis P. D. (2003). Time to reduction: Factors influencing management efficacy in sterilizing overabundant white-tailed deer. J. Wildl. Manage. 67, 267–279. doi: 10.2307/3802768
Miller K. M., McGill B. J. (2019). Compounding human stressors cause major regeneration debt in over half of eastern US forests. J. Appl. Ecol. 56, 1355–1366. doi: 10.1111/1365-2664.13375
Miller K. M., Perles S. J., Schmit J. P., Matthews E. R., Weed A. S., Comiskey J. A., et al. (2023). Overabundant deer and invasive plants drive widespread regeneration debt in eastern United States national parks. Ecol. Appl. 33, e2837. doi: 10.1002/eap.283724
Mo L., Zohner C. M., Reich P. B., Liang J., de Miguel S., Nabuurs G.-J., et al. (2023). Integrated global assessment of the natural forest carbon potential. Nature 624, 7990, 101. doi: 10.5194/egusphere-egu24-11507
Mooney E. H., McGraw J. B. (2007). Alteration of selection regime resulting from harvest of American ginseng, Panax quinquefolius. Conserv. Genet. 8, 57–67. doi: 10.1007/s10592-006-9148-3
Mudrak E. L., Johnson S. E., Waller D. A. (2009). Forty-seven year changes in vegetation at the Apostle Islands: effects of deer on the forest understory. Natural Areas J. 29, 167–176. doi: 10.3375/043.029.0209
Nagy C., Ng C., Veverka N., Weckel M. (2022). Assessment of a 15-year white-tailed deer management program and woody recovery in a suburban forest preserve. For. Ecol. Manage. 503. doi: 10.1016/j.foreco.2021.119748
Neufeld B. T., Superbie C., Greuel R. J., Perry T., Tomchuk P. A., Fortin D., et al. (2021). Disturbance-mediated apparent competition decouples in a northern boreal caribou range. J. Wildl. Manage. 85, 254–270. doi: 10.1002/jwmg.21982
New Zealand Department of Conservation (2023). Predator Free 2050. Available online at: https://www.doc.govt.nz/nature/pests-and-threats/predator-free-2050/ (Accessed 25 July 2023).
Nie M., Barns C., Haber J., Joly J., Pitt K., Zellmer S. (2017). Fish and wildlife management on federal lands: debunking state supremacy. Environ. Law 47, 797–932. Available at: https://www.jstor.org/stable/44466736
Nuttle T., Ristau T. E., Royo A. A. (2014). Long-term biological legacies of herbivore density in a landscape-scale experiment: forest understoreys reflect past deer density treatments for at least 20 years. J. Ecol. 102, 221–228. doi: 10.1111/1365-2745.12175
Nuttle T., Yerger E. H., Stoleson S. H., Ristau T. E. (2011). Legacy of top-down herbivore pressure ricochets back up multiple trophic levles in forest canopies over 30 years. Ecosphere 2. doi: 10.1890/ES10-00108.1
Nuzzo V., Dávalos A., Blossey B. (2017). Assessing plant community composition fails to capture impacts of white-tailed deer on native and invasive plant species. AoB Plants 9, plx026. doi: 10.1093/aobpla/plx026
Oates B. A., Monteith K. L., Goheen J. R., Merkle J. A., Fralick G. L., Kauffman M. J. (2021). Detecting resource limitation in a large herbivore population is enhanced with measures of nutritional condition. Front. Ecol. Evol. 8. doi: 10.3389/fevo.2020.522174
Olson L. O., Deelen T. R. V., Storm D. J., Crimmins S. M. (2021). Understanding environmental patterns of canid predation on white-tailed deer (Odocoileus virginianus). Can. J. Zool. 99, 912–920. doi: 10.1139/cjz-2021-0024
Ostfeld R. S., Keesing F. (2017). Is biodiversity bad for your health? Ecosphere 8, e01676. doi: 10.1002/ecs2.1676
Ostfeld R. S., Mowry S., Bremer W., Duerr S., Evans R. S. Jr., Fischhoff I. R., et al. (2023). Impacts over time of neighborhood-scale interventions to control ticks and tick-borne disease incidence. Vector-Borne Zoonotic Dis. 23, 89–105. doi: 10.1089/vbz.2022.0094
Oswald W. W., Foster D. R., Shuman B. N., Chiton E. S., Doucette D.l., Duranleau D. L. (2020). Conservation implications of limited Native American impacts in pre-contact New England. Nat. Sustainabil. 3, 241–246. doi: 10.1038/s41893-019-0466-0
Patton S. R., Russell M. B., Windmuller-Campione M. A., Frelich L. E. (2018). Quantifying impacts of white-tailed deer (Odocoileus virginianus Zimmerman) browse using forest inventory and socioenvironmental datasets. PloS One 13, e0201334. doi: 10.1371/journal.pone.0201334
Paulin J. B., Arbab N. N., Schilling B. J. (2022). White-tailed deer and the hidden costs to farmers’ livelihoods: a case study of New Jersey stories (New Brundwick, New Jersey, USA: Rutgers University, School of Environmental and Biological Sciences, New Jersey Agricultural Experiment Station, Rutgers Cooperative Extension).
Pauly D. (1995). Anecdotes and the shifting base-line syndrome of fisheries. Trends Ecol. Evol. 10, 430–430. doi: 10.1016/S0169-5347(00)89171-5
Phillips G. E., Cristol D. A. (2024). Mechanisms of deer (Cervidae) impacts on birds: A comprehensive review. Biol. Conserv. 290, 110454. doi: 10.1016/j.biocon.2024.110454
Plumb G., Monello R., Resnik J., Kahn R., Leong K., Decker D. (2014). A comprehensive review of National Park Service ungulate management: second century challenges, opportunities, and coherence. Natural Resource Report NPS/NRSS/BRMD/NRR—2014/898. For Collins, CO: National Park Service.
Pimlott D. H. (1967). Wolf predation and ungulate populations. Am. Zool. 7, 267–278. doi: 10.1093/icb/7.2.267
Pomeranz E., Decker D. (2017). Designing regional-level stakeholder engagement processes: striving for good governance while meeting the challenges of scale. J. Environ. Policy Plann. 20, 403–418. doi: 10.1080/1523908X.2017.1417119
Porter W. F., Underwood H. B. (1999). Of elephants and blind men: deer management in the U.S. National Parks. Ecol. Appl. 9, 3–9. doi: 10.1890/1051-0761(1999)009[0003:OEABMD]2.0.CO;2
Quirion B., Blossey B. (2023). Experimental evaluation of four protocols for assessing white-tailed deer browse intensity. Ecol. Indic. 154, 110851. doi: 10.1016/j.ecolind.2023.110651
Raizman E. A., Holland J. D., Shukle J. T. (2013). White-tailed deer (Odocoileus virginianus) as a potential sentinel for human Lyme disease in Indiana. Zoonoses Public Health 60, 227–233. doi: 10.1111/j.1863-2378.2012.01518.x
Read J. L., Wilson G. R., Coulson G., Cooney R., Paton D. C., Moseby K. E., et al. (2021). Improving kangaroo management: A joint statement. Ecol. Manage. Restor. 22, 186–192. doi: 10.1111/emr.12467
Reed S. P., Royo A. A., Fotis A. T., Knight K. S., Flower C. E., Curtis P. S. (2022). The long-term impacts of deer herbivory in determining temperate forest stand and canopy structural complexity. J. Appl. Ecol. 59, 812–821. doi: 10.1111/1365-2664.14095
Ripple W. J., Estes J. A., Beschta R. L., Wilmers C. C., Ritchie E. G., Hebblewhite M., et al. (2014). Status and ecological effects of the World’s largest carnivores. Science 343, 1241484. doi: 10.1126/science.1241484
Robinson K. F., Diefenbach D. R., Fuller A. K., Hurst J., Rosenberry C. S. (2014). Can managers compensate for coyote predation of white-tailed deer? J. Wildl. Manage. 78, 571–579. doi: 10.1002/jwmg.693
Rochlin I., Ninivaggi D. V., Benach J. L. (2019). Malaria and Lyme disease - the largest vector-borne US epidemics in the last 100 years: success and failure of public health. BMC Public Health 19, 804. doi: 10.1186/s12889-019-7069-6
Rogers P. C., McAvoy D. J. (2018). Mule deer impede Pando’s recovery: implications for aspen resilience from a single-genotype forest. PloS One 13, e0203619. doi: 10.1371/journal.pone.0203619
Rogers L. L., Mooty J. J., Dawson D. (1981). “Foods of white-tailed deer in the Upper Great Lakes Region – a review,” in General Technical Report (U.S. Department of Agriculture, Forest Service, North Central Forest Experiment Station, St. Paul, Minnesota, USA).
Rosenberg K. V., Dokter A. M., Blancher P. J., Sauer J. R., Smith A. C., Smith P. A., et al. (2019). Decline of the North American avifauna. Science 366, 120–124. doi: 10.1126/science.aaw1313
Rushing C. S., Rohrbaugh R. W., Fiss C. J., Rosenberry C. S., Rodewalde A. D., Larkin J. L. (2020). Long-term variation in white-tailed deer abundance shapes landscape-scale population dynamics of forest-breeding birds. For. Ecol. Manage. 456, 117629. doi: 10.1016/j.foreco.2019.117629
Russell M. B., Woodall C. W., Potter K. M., Walters B. F., Domke G. M., Oswalt C. M. (2017). Interactions between white-tailed deer density and the composition of forest understories in the northern United States. For. Ecol. Manage. 384, 26–33. doi: 10.1016/j.foreco.2016.10.038
Schaefer J. A., Mahoney S. P., Weir J. N., Luther J. G., Soulliere C. E. (2016). Decades of habitat use reveal food limitation of Newfoundland caribou. J. Mammal. 97, 386–393. doi: 10.1093/jmammal/gyv184
Schweitzer D., Garris J. R., McBride A. E., Smith J. A. M. (2014). The current status of forest Macrolepidoptera in northern New Jersey: evidence for the decline of understory specialists. J. Insect Conserv. 18, 561–571. doi: 10.1007/s10841-014-9658-0
Severinghouse C. W., Brown C. P. (1956). History of the white-tailed deer in New York. New York Fish Game J. 3, 130–167. Available at: https://extapps.dec.ny.gov/docs/wildlife_pdf/histdeernewyork.pdf
Smith D. W., Stahler D. R., MacNulty D. R. (2020). Yellowstone Wolves. Science and Discovery in the Wold’s First National Park (Chicago, US: University of Chicago Press). doi: 10.7208/chicago/9780226728483.001.0001
Stafford K. C., Denicola A. J., Kilpatrick H. J. (2003). Reduced abundance of Ixodes scapularis (Acari: Ixodidae) and the tick parasitoid Ixodiphagus hookeri (Hymenoptera: Encyrtidae) with reduction of white-tailed deer. J. Med. Entomol. 40, 642–652. doi: 10.1603/0022-2585-40.5.642
Sterba J. (2012). Nature wars; the incredible story how wildlife comebacks turned backyards into battlegrounds (New York: Crown Publishers).
Sullivan L. M., Manfredo M. J., Teel T. L. (2022). Technocracy in a time of changing values: Wildlife conservation and the ‘relevancy’ of governance reform. Conserv. Sci. Pract. 4. doi: 10.1111/csp2.545
Telford S. (2017). Deer reduction is a cornerstone of integrated deer tick management. J. Integr. Pest Manage. 25, 21–25. doi: 10.1093/jipm/pmx024
The White House (2021). Executive Order 13990: Protecting public health and the environment and restoring science to tackle the climate crisis (Washington DC: Federal Register).
The White House (2022). Executive Order 14072: Strengthening the Nation’s forests, communities, and local economies (Washington DC: Federal Register).
The White House (2023). National strategy to develop statistics for environmental-economic decisions. A U.S. system of natural capital accounting and associated environmental-economic statistics (Washington, DC: Federal Register).
U.S. Fish and Wildlife Service (2018). 2016 National Survey of Fishing, Hunting, and Wildlife-Associated Recreation (Washington, DC: U.S. Fish and Wildlife Service).
VanAcker M. C., Little E. A. H., Molaei G., Bajwa W. I., Diuk-Wasser M. A. (2019). Enhancement of risk for Lyme disease by landscape connectivity, New York, New York, USA. Emerg. Infect. Dis. 25, 1136–1143. doi: 10.3201/eid2506.181741
Van Deelen T. R., Dhuey B. J., Jacques C. N., McCaffery K. R., Rolley R. E., Warnke K. (2010). Effects of earn-a-buck and special antlerless-only seasons on Wisconsin’s deer harvests. J. Wildl. Manage. 74, 1693–1700. doi: 10.2193/2009-551
van Klink R., Bowler D. E., Gongalsky K. B., Shen M., Swengel S. R., Chase J. M. (2023). Disproportionate declines of formerly abundant species underlie insect loss. Nature. doi: 10.1038/s41586-023-06861-4
Vercauteren K. C., Anderson C. W., Van Deelen T. R., Drake D., Walter W. D., Vantassel S. M., et al. (2011). Regulated commercial harvest to manage overabundant white-tailed deer: an idea to consider? Wildl. Soc. Bull. 35, 185–194. doi: 10.1002/wsb.36
Verme L. J., Ullrey D. E. (1984). “Physiology and nutrition,” in White-Tailed Deer: Ecology and Management. Ed. Halls L. K. (Stackpole Books, Harrisburg, Pennsylvania, USA), 91–118.
Vickers L. A., McWilliams W. H., Knapp B. O., D’Amato A. W., Dey D. C., Dickinson Y. L., et al. (2019). Are current seedling demographics poised to regenerate northern US forests? J. Forest. 117, 592–612. doi: 10.1093/jofore/fvz046
Vincent C. H., Hanson L. A., Bermejo L. F. (2020). Federal land ownership: overview and data. (Washington, DC: Congressional Research Service). Available at: https://sgp.fas.org/crs/misc/R42346.pdf.
Waller D. M., Johnson S. E., Witt J. C. (2017). A new rapid and efficient method to estimate browse impacts from twig age. For. Ecol. Manage. 404, 361–369. doi: 10.1016/j.foreco.2017.09.001
Werden L., Barker I. K., Bowman J., Gonzales E. K., Leighton P. A., Lindsay L. R., et al. (2014). Geography, deer, and host biodiversity shape the pattern of Lyme disease emergence in the Thousand Islands Archipelago of Ontario, Canada. PloS One 9, e85640. doi: 10.1371/journal.pone.0085640
Wild M. A., Hobbs N. T., Graham M. S., Miller M. W. (2011). The role of predation in disease control: a comparison of selective and nonselective removal on prion disease dynamics in deer. J. Wildl. Dis. 47, 78–93. doi: 10.7589/0090-3558-47.1.78
Williams S. C., DeNicola A. J., Almendinger T., Maddock J. (2013). Evaluation of organized hunting as a management technique for overabundant white-tailed deer in suburban landscapes. Wildl. Soc. Bull. 37, 137–145. doi: 10.1002/wsb.236
Williams J. L., Kendall B. E., Levine J. M. (2016). Rapid evolution accelerates plant population spread in fragmented experimental landscapes. Science 353, 482–485. doi: 10.1126/science.aaf6268
Williams E. S., Miller M. W., Kreeger T. J., Kahn R. H., Thorne E. T. (2002). Chronic wasting disease of deer and elk: a review with recommendations for management. J. Wildl. Manage. 66, 551–563. doi: 10.2307/3803123
Williamson A. (2011). Biological assessment of the United States Department of Agriculture National Forest System Land Management Planning Rule for federally listed endangered and threatened species, species proposed for federal listing, species that are candidates for federal listing on National Forest system lands (Washington, D C: F.S. USDA). Available at: https://www.fs.usda.gov/Internet/FSE_DOCUMENTS/stelprdb5359453.pdf.
Woolnough A. P., Edwards G., Hart Q. (2016). Feral camels: a nationally significant pest animal requiring a national management approach. Rangeland J. 38, 109–115. doi: 10.1071/RJ15098
Wyss J. A. (2006). Screwworm eradication in the Americas. Ann. New York Acad. Sci. 916, 186–193. doi: 10.1111/j.1749-6632.2000.tb05289.x
Zhang S. (2020). America’s never-ending battle against flesh-eating worms. Atlantic. Available at: https://www.theatlantic.com/science/archive/2020/05/flesh-eating-worms-disease-containment-america-panama/611026/
Keywords: biodiversity, conservation, wildlife management, forest regeneration, leadership, human health, deer management
Citation: Blossey B, Hare D and Waller DM (2024) Where have all the flowers gone? A call for federal leadership in deer management in the United States. Front. Conserv. Sci. 5:1382132. doi: 10.3389/fcosc.2024.1382132
Received: 05 February 2024; Accepted: 19 March 2024;
Published: 03 April 2024.
Edited by:
Hiroshi Tsunoda, Center for Environmental Science, JapanReviewed by:
Hiromasa Igota, Rakuno Gakuen University, JapanKoichi Kaji, Tokyo University of Agriculture and Technology, Japan
Copyright © 2024 Blossey, Hare and Waller. This is an open-access article distributed under the terms of the Creative Commons Attribution License (CC BY). The use, distribution or reproduction in other forums is permitted, provided the original author(s) and the copyright owner(s) are credited and that the original publication in this journal is cited, in accordance with accepted academic practice. No use, distribution or reproduction is permitted which does not comply with these terms.
*Correspondence: Bernd Blossey, YmIyMkBjb3JuZWxsLmVkdQ==
†ORCID: Bernd Blossey, orcid.org/0000-0001-7043-4435
Darragh Hare, orcid.org/0000-0003-4418-9637
Donald M. Waller, orcid.org/0000-0001-5377-3929