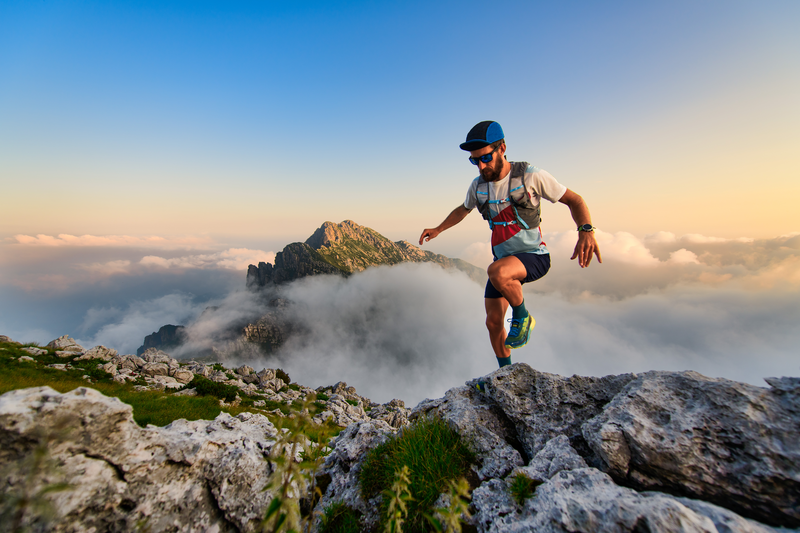
95% of researchers rate our articles as excellent or good
Learn more about the work of our research integrity team to safeguard the quality of each article we publish.
Find out more
ORIGINAL RESEARCH article
Front. Conserv. Sci. , 08 May 2024
Sec. Human-Wildlife Interactions
Volume 5 - 2024 | https://doi.org/10.3389/fcosc.2024.1351366
This article is part of the Research Topic Rewilding in Practice View all 10 articles
Rewilding landscapes through species or population restoration is an increasingly applied practice in biological conservation. There is expanding interest in wildlife release projects for apex predator population augmentation or reintroductions in historical ranges. Cheetahs (Acinonyx jubatus) are an IUCN Vulnerable-listed species with a declining global population facing major threats, which in southern Africa primarily include lethal persecution on livestock farms and bush encroachment transforming open habitats to woody areas. We used GPS radiocollars to monitor ten adult cheetahs from 2007 – 2018 in the Central Plateau of Namibia encompassing an area restored as an open savanna field (13.7 km2) located in a matrix of woodland savanna affected by bush encroachment. We generated a set of a priori hypotheses that tested the effects of various factors on cheetah movements indexed by step length. We compared cheetah movement metrics based on their history as wild, rehabilitated, and/or translocated individuals. Day/night activity, habitat type, and habitat edges were significant predictors of cheetah movement. Wild resident cheetahs displayed significantly longer steps than the other cheetah classes, possibly suggesting increased territorial behaviour in response to the presence of introduced cheetahs. Some cheetahs temporally segregated by moving extensively during daytime, but most individuals were primarily active during crepuscular periods. Small prey remained constant across time, whereas large prey declined over the study period. Cheetahs appeared to adjust behaviourally by increasing movements in years when large prey were scarce. Cheetahs appeared to be ecologically adaptable and behaviourally flexible in response to varying prey populations and when translocated to new environments, specifically at the interface between bush-encroached woodland and open savanna. Environmental settings and animal history need to be carefully considered in rewilding and ecosystem restoration, and monitoring of released and resident individuals, if present, is important to understand ecological dynamics at release sites.
Habitat loss, illegal killing, declining prey, and human-wildlife conflicts threaten the survival of apex predators globally (Woodroffe, 2006; Ripple et al., 2014; Lennox et al., 2022). Terrestrial apex predators confront challenges that extend beyond ecological realms, impacting economic and political dimensions of conservation (Jhala et al., 2020). In Africa, many large carnivores are classified by IUCN as endangered (e.g., the Ethiopian wolf [Canis simensis] and African wild dog [Lycaon pictus] or vulnerable (e.g., cheetahs [Acinonyx jubatus], lions [Panthera leo]), requiring conservation action for persistence or recovery (Durant et al., 2017; Marneweck et al., 2019; Seid et al., 2022).
Cheetahs face alarming population fragmentation and decline, with an approximate count of 7,100 adults and subadults remaining across Africa predominantly outside protected areas (Durant et al., 2017; Marker et al., 2018a). While we cumulatively know much about baseline cheetah ecology (Caro, 1994; Durant et al., 2007; Marker et al., 2018b), information on cheetah movement ecology is sparse. Insights from movement analysis of carnivores hold the potential to inform human-wildlife conflict mitigation and bolster critical ecosystem services by maintaining carnivores on the landscape (Odden et al., 2014; Van der Weyde et al., 2017; Loveridge et al., 2022; Teichman et al., 2023).
The world’s primary cheetah population stronghold is in southern Africa (Durant et al., 2017; Marker et al., 2018a), but cheetahs therein are subject to lethal persecution on livestock farms and in many areas must contend with bush encroachment, which can alter both predator and prey behaviours (Marker and Dickman, 2004; Nghikembua et al., 2021; Atkinson et al., 2022a; Nghikembua et al., 2023). Habitat change to woody vegetation potentially affects the cheetah’s hunting strategies and energy acquisition, given their preference for open landscapes (Caro, 1994; Atkinson et al., 2022b). While both human-wildlife conflict and habitat change can be tackled with management and education, in some areas the cheetah populations are low or extinct, and restoration requires translocations for population recovery. In such contexts, rewilding provides a pathway to restore populations that underwent extinction or significant declines (Fritts et al., 1997; Hayward et al., 2007; Jhala et al., 2021). Human interventions involving translocation, rehabilitation, and reintroduction are instrumental in this endeavour (Odden et al., 2014; Naha et al., 2021; Walker et al., 2022).
We assessed potential differences in movement patterns and ecological correlates associated with movement, comparing wild cheetahs and those subjected to human intervention through translocation and/or rehabilitation. Understanding movement characteristics and variability according to cheetah history and human-mediated management can assist conservation strategies and apex predator population management on African landscapes (Marker et al., 2018a; Fabiano et al., 2020). When linked to habitat conditions and prey information, apex predator movement data can allow insights into connectivity considerations and predator-prey dynamics respectively (Broekhuis et al., 2021; Loveridge et al., 2022).
Using GPS radiocollar data on cheetahs and prey information spanning 15 years, we studied movement rate (i.e., step length) (Turchin, 1998; Thurfjell et al., 2014), investigated relationships between multiple variables and movement, and examined associations with prey availability (Broekhuis et al., 2019, Broekhuis et al., 2021; Rodriguez-Recio et al., 2022) in a period with declining prey (Bandyopadhyay et al., submitted). Our cheetah data were categorised among four different classes (Wild Local, Rehabilitated Local, Rehabilitated Translocated, and Wild Rehabilitated Translocated; for full description, see Table 1). We tested the following hypotheses:
1. Cheetah movement patterns differ between individuals according to history and human intervention. Wild local individuals would move slower than translocated cheetahs due to greater familiarity with their surroundings requiring less energy expenditure. Translocated individuals are unlikely to possess the same ecological knowledge of the area, and we expected them to exhibit longer movements associated with exploratory behaviour (Walker et al., 2022).
2. Marking trees and waterholes significantly influence cheetah movement patterns as exhibited by shorter movements when in the vicinity of these landscape features. Cheetahs may claim these key resources with territorial exclusivity (Marker-Kraus and Kraus, 1997), wherein marking trees are used to advertise presence to territory contesters, and waterholes are prime hunting areas with predictable prey presence (Nghikembua et al., 2016; Broekhuis et al., 2021).
3. Cheetahs will move greater distances over time to encounter prey due to a steep decline in prey density (Bandyopadhyay et al., submitted). Although this process may also be influenced by interspecific competition and changes in habitat quality, this will not be tested in this study.
4. Cheetahs exhibit more diurnal activity than nocturnality, as a mechanism to temporal segregate from other, dominant large carnivores (leopard [Panthera pardus] in our system) and to maximise visibility during prey pursuits (Hayward and Slotow, 2009).
The study took place in the Central Plateau of Namibia on farmlands that are Cheetah Conservation Fund (CCF) property. The property covers 577 km2 and is managed as a wildlife reserve as well as mixed-use land for wildlife and livestock. The area has an average temperature of 19.2°C (+/- 2.4°C) and an annual rainfall of 400-450 mm (Marker et al., 2008). The predominant habitat type is semi-arid thornbush woodland savannah (Marker et al., 2008; Nghikembua et al., 2021), with dominant tree genera including: Boscia, Combretum, Dichrostachys, Grewia, Senegalia, Terminalia, and Vachellia (Nghikembua et al., 2021; Bandyopadhyay et al., submitted).
The focal study site for analysis centred around the ‘Big Field’ section of the broader property and is operated exclusively as a wildlife reserve (Lat -20.4839°, Long 17.0317°; Figure 1). ‘Big Field’ is managed as an open savanna area covering approximately 13.7 km2, while the remaining property is woodland savanna that is heavily bush-encroached, with bush cover >90% in some areas (Nghikembua et al., 2016). Big Field is of significant relevance as one of the largest open areas in north-central Namibia within a predominantly bush-encroached landscape.
Figure 1 Map showing the location of the focal study area (red) in the broader landscape of North-central Namibia. The area included a large field (Big Field) managed as open savanna in a matrix of bush encroached woodland savanna. Yellow points are the cheetah GPS radiocollar locations included in the analysis.
Cheetah data were collected via GPS radiocollars (Sirtrack – Havelock North, New Zealand, ATS – Minnesota, USA, or AWT – Pretoria, South Africa) on 17 adult cheetahs (11 females and 6 males) that were either captured on site by CCF for research and released at the capture location, or were captured on other properties by private farmers and translocated and released on the CCF property as part of human-wildlife conflict mitigation at the origin site, and cheetah population recovery at CCF. Cheetahs were captured by CCF or farmers through human-wildlife conflicts using double door (walk-through) cage traps, often at preferential cheetah marking sites. Cheetahs were anaesthetised with drug combinations including Telazol®, Ketamine-Medetomidine, or Ketamine-Midazolam (Marker et al., 2008). The capture and handling of animals adhered to approved procedures and were conducted in compliance with relevant regulations and permits (NCRST AN202101032). Additionally, some animals arrived at CCF as orphan cubs from human-wildlife conflict of livestock farms and were held in captivity in large enclosures on CCF property as part of a rehabilitation and release programme (Walker et al., 2022). All cheetahs were adult at the time of collaring and monitoring.
The available GPS location data covered the period September 2007 to May 2022, and included cheetah ID, individual history, sex, age class, reproductive class (solitary male, coalition male, solitary female, female with cubs), type of collar used, date and timestamp for each GPS location, latitude, longitude, release date, and release location.
Prey data were collected in the same timeframe as the cheetah collar data via road transects that were repeatedly driven to record ungulate observations visually. We classified prey into small (common duiker [Sylvicapra grimmia], dik-dik [Madoqua kirkii], springbok [Antidorcas marsupialis], steenbok [Raphicerus campestris]) and large categories (eland [Taurotragus oryx], greater kudu [Tragelaphus strepsiceros], oryx [Oryx gazella], red hartebeest [Alcelaphus buselaphus]).
Transects covered the entire CCF property and no habitat stratification was applied as the property can be classified as woodland savanna (Bandyopadhyay et al., submitted; Supplementary Table S1). Prey data were also collected along dedicated short transects in the Big Field section. Transects were driven at a speed of maximum 20 km/h and whenever an animal was detected, the vehicle was stopped and observers recorded the perpendicular distance from the transect to the animal for subsequent analysis in a distance sampling framework (Buckland et al., 2001). The total annual transect sampling effort was 2,382 km.
Prior to computing movement metrics, we refined the cheetah GPS radiocollar data to focus on Big Field and a buffer region around it. GPS fix acquisition rates for the deployed collars varied along the 15-year dataset. We inspected the data and found that 3-hour fix rates were used most extensively, therefore we constrained our analysis to this fix rate. While this refinement resulted in dropping seven individuals which either had different fix rates or sparse data, using variable fix rate would have affected our inferences on animal movements from collar data (Cristescu et al., 2015). Our final sample size was ten cheetahs (weight range at release – 25 to 57 kg) across four distinct classes: Wild Local, Rehabilitated Local, Rehabilitated Translocated, and Wild Rehabilitated Translocated, which contributed 4,965 data points for the analysis during 2007 – 2018 (Tables 1, 2). We calculated step length (movement rate) for all ten cheetahs and established the buffer size around Big Field as the 95th percentile of step length of the ten individuals pooled.
Table 2 Final data of 4,965 GPS points used to investigate factors associated with movement in a GLM analytical framework.
For each cheetah step we calculated distances to the closest marking tree (known from long-term monitoring to be used by cheetahs for marking), waterhole, and habitat edge of the open savanna (Big Field)-woodland savanna interface, using the distance matrix function in Q-GIS. We defined day/night cycles for each season (Cold-Dry [May-August], Hot-Dry [September-December] and Hot-Wet [January-April]; Nghikembua et al., 2021), assigning day, night or crepuscular period for each GPS radiocollar point from automated time zones (NST) using the ‘suncalc’ package in R (Core Team, 2021). We categorised steps as intersecting habitat edge (open savanna-woodland savanna interface), inside Big Field, or outside Big Field.
We estimated prey encounter likelihood separately for small and large prey, using the effective strip width model (ESW) and prey density estimates in a distance sampling framework (Bandyopadhyay et al., submitted). We further categorised prey by foraging strategy (browsers, grazers, and mixed feeders) when calculating prey encounter rate. Because the number of prey observations along the Big Field transects was too small to derive density estimates, we combined these monthly collected data with annual CCF property-wide transects to obtain density estimates. The property-wide estimates also had few observations of prey, therefore similarly to Bandyopadhyay et al. (submitted), we pooled the data into four-year intervals (2009-2012, 2013-2016, and 2017-2020) to relate to daily distance moved by cheetahs stratified by the same intervals. One cheetah (AJU1533) with data points in 2007 and 2008 was excluded from the predator-prey analysis due to a lack of yearly prey data for 2007-2008, attributed to staffing constraints.
Environmental influences on the cheetahs’ movements other than those tested in our analyses were minimised because GPS radiocollar locations occurred in the same land use type (wildlife reserve) and the same rainfall class as derived from the Atlas of Namibia (https://atlasofnamibia.online/).
Step length and turning angles for the collared cheetahs were calculated using the ‘move’ package in R (v4.2.4) (Turchin, 1998; Thurfjell et al., 2014; Core Team, 2021). We used ANOVA, t-tests and post-hoc Tukey tests to check for significant differences in step length among class, sex, and season.
We used a Generalised Linear Model (GLM) approach with covariate combinations to determine associations of ecological factors and cheetah step length. We ran a correlation analysis to identify covariates that might induce multicollinearity, and covariate combinations with |r| > 0.6 were excluded from the same model structure (Supplementary Table S2). For each of the four cheetah classes, we used the same candidate model set to identify fixed-effects covariates associated with step length as the dependent variable (Table 3). We excluded prey density from the GLMs because prey density was a non-spatial estimate. For each cheetah class, we ranked models in the candidate set using the Akaike Information Criterion (AIC) corrected for small sample sizes (AICc), and considered models to be supported if their ΔAICc <2. Additionally, we ran two sets of mixed-effects models with the same fixed-effects covariates, using cheetah class and individual cheetah ID respectively as random intercepts. We ranked models in these two sets using AIC (Supplementary Tables S11 – S16).
Table 3 Table of multiple hypotheses tested with GLM models to investigate factors associated with cheetah movements.
We analysed the variability in prey density over time using Wilcoxon-tests separately for large and small prey. We contrasted the empirical prey estimates with symmetrical distributions to independently test if prey density fluctuated significantly from 2009 to 2020, using the 4-year intervals mentioned above. Finally, we determined the prey encounter rates by dividing the encounter rate per day per prey species by the total number of independent observations made during the effort. Results were split into prey foraging categories (browsers, grazers, and mixed feeders). We then contrasted them with the daily distance travelled by each cheetah class.
We used Q-GIS (v3.2.8), ArcGIS (v10.8.2), R Studio (v4.2.1) and R packages dplyr, lubridate, suncalc, move, lme4, and ggplot2 for analyses.
Wild Local cheetahs had the highest average step length (1,164 m), followed by Rehabilitated Local (601 m), with both local groups displaying longer movements than Rehabilitated Translocated and Wild Rehabilitated Translocated (400 m and 278 m respectively) (Figure 2). The difference was statistically significant for Wild Local compared to all other classes (p <0.05) (Supplementary Table S3).
Figure 2 Histograms for step lengths of the four classes of cheetah. Average frequencies of step length represented as percentages, with error bars included for each class.
Cheetahs moved the shortest distances in the Cold-Dry season (405 m) compared to Hot-Dry (675 m) and Hot-Wet (632 m) periods (p <0.05) (Supplementary Table S4). Males (903 m) exhibited longer step lengths on average than females (364 m) (p <0.001) (Supplementary Table S5).
Cheetahs mostly moved in the buffer outside of the Big Field (proportion of steps 0.80) but when therein they took shorter steps (488 m). While spending less time inside Big Field, they moved faster in the open area (799 m). In contrast, steps intersecting the open savanna-woodland savanna habitat edge produced long-range movements (1618 m), but these steps were proportionally lowest compared to steps within and outside Big Field (Supplementary Table S6).
All analyses found the day, night, and crepuscular covariates to be significant, but for the mixed-effects models using pooled data, no other fixed-effects covariates were significant (Supplementary Tables S11 – S16). Cheetahs did not show a specific preference for directionality, with turning angles appearing relatively evenly distributed (Supplementary Figure S1).
Only the Global Model (no marking tree) was supported for Wild Local individuals (Table 4; Supplementary Table S7). Wild Local cheetahs moved shorter distances during the day and night compared to crepuscular periods, and steps that intersected habitat edge were longer compared to steps inside Big Field.
Table 4 Covariates associated with cheetah movements based on supported models ranked in an AICc framework (ΔAICc <2).
Four models were supported (ΔAICc <2): Global Model 2 (Resource Movement), Habitat Use model, Hunting Habitat model, and Global Model (No marking tree), but the Resource Movement model received the most support (Table 4; Supplementary Table S8). Rehabilitated Local cheetahs moved longer distances during the day and shorter distances during the night compared to crepuscular periods. Cheetahs moved shorter distances when far from waterholes.
Only the Global Model (no marking tree) was supported for Rehabilitated Translocated individuals (Table 4; Supplementary Table S9). Results for steps intersecting habitat edge, day and night showed the same trend as Wild Local cheetahs. In addition, distance to edge and quadratic distance to edge were associated with step length, indicating that step length varied non-linearly with edge habitat.
Only the Global Model 2 (Resource Movement) was supported (Table 4; Supplementary Table S10). These cheetahs moved longer distances during the day compared to crepuscular periods. Additionally, the cheetahs moved shorter distances when far from marking trees. Steps intersecting habitat edges were longer compared to those confined by the Big Field boundaries.
Small prey did not vary significantly over time, whereas large prey did (W=36, p=0.029*) (Figure 3). In years of higher prey availability, including both grazer and browser ungulate species, cheetahs moved shorter distances (2013-2016) (Figure 4).
Figure 3 Prey density (per km2) for small and large ungulate prey, calculated over time. The blue line represents large prey, and the red line represents small prey. Giraffe was excluded due to low likelihood of cheetah hunting this species, and Leporids, ostrich and secretary birds excluded due to few observations precluding calculation of the estimated strip width from distance sampling. .
Figure 4 Daily distance (km) moved by cheetahs in four-year increments: 2009-2012 (n=2), 2013-2016 (n=5), 2017-2020 (n=2). The prey encounter rate (prey per km2) is displayed on the secondary y-axis, with results represented by lines split into three prey categories. Ungulate prey according to foraging strategy included mixed feeders (n=2), grazers (n=5), and browsers (n=3). The blue line represents grazers, the red line represents browsers and the orange line represents mixed feeders.
The releases of cheetahs in our study system were carried out to augment a local population that persisted at low densities thought to be below carrying capacity, while simultaneously mitigating human-wildlife conflict at the sites where the released animals originated from. The releases of translocated individuals took place within existing cheetah range on CCF property which functioned as wildlife reserve, and contributed to the enhancement of the local population and presumably to ecosystem services therein (Fabiano et al., 2020). Some of the cheetahs released had initially been brought to CCF as orphaned cubs when their mothers were killed as part of human-wildlife conflicts, therefore post-release monitoring provided an ideal opportunity to compare the movement ecology of rehabilitated individuals to wild ones. We justify our categorisation of cheetahs through ecological rationale and variables included in our methodology, but acknowledge that large carnivores exhibit individual behavioural variability (Cristescu and Boyce, 2013; Shaw, 2020). Our sample size was insufficient to build individual models and to directly assess individual differences as well as influence of age within a given reproductive class. However, we did account for individual and reproductive class variability using a mixed-effects modelling approach (Supplementary Tables S14 – S16).
Wild Local cheetahs exhibited significantly longer steps than other classes, which is contrary to our expectation that they would have shortest movements because of familiarity with the area and absence of exploratory movements. The extensive movement rates of Wild Local individuals might be attributed to enhanced territorial behaviour with the release of conspecifics in the same system. Cheetah releases occurred throughout the study duration, including releases when the wild local cheetahs were monitored (2007-2009). However, some of the releases involved VHF radiocollars and therefore could not be included in this study due to lack of GPS data for movement analysis. The large movement rates of Wild Local individuals could also reflect a hunting strategy that focuses on large prey which are present at low density and require extensive searches for movements.
Also contrary to expectations, released cheetahs that were unfamiliar with the area having been translocated displayed shorter movements, which might be suggestive of cautious exploratory behaviour. The difference in movement rates among cheetah classes suggest possible behavioural changes that may be triggered by environmental factors but also possibly by conspecific interactions, pointing to the need to understand the effects of releases on resident and introduced individuals in rewilding and reintroduction efforts. Differences in movement rates were recorded among sexes also, with male cheetahs having significantly longer step lengths than females, despite females maintaining larger home ranges in this species (Marker et al., 2007). Extensive movements by males may be a strategy for territorial defence (Weise et al., 2015).
Although classically considered to be diurnal hunters, with bouts of crepuscular activity (Hayward and Slotow, 2009; Nghikembua et al., 2016), cheetahs have been demonstrated to be flexible in activity patterns and can have nocturnal movements where dominant large carnivores are absent or where they are heavily persecuted by humans. Cheetahs can be particularly active during lunar cycles with increased moonlight, a strategy thought to increase nocturnal hunting efficiency (Broekhuis et al., 2014; Searle et al., 2021). Our findings on cheetah movements show a predominance of long movements during the crepuscular period, which might place them at risk of encountering a dominant competitor, the leopard (Verschueren et al., 2021).
Rehabilitated Local individuals were the only ones for which movements were associated with waterhole proximity, but in the opposite pattern than we had anticipated. When close to waterholes, this class of cheetahs moved greater distances than when far from waterholes, possibly indicating that proximity to waterhole may not be desirable. Although waterholes are key resources for many large carnivores (Jhala et al., 2021) for hydration and hunting opportunities, they may especially be sought after by ambush predators (Crosmary et al., 2012). The cheetahs in this category were solitary and familiar to the area, thereby presumably aware that waterholes increase the likelihood of encountering leopards (Krag et al., 2023). Leopards are the dominant predator and are highly abundant in this system (Cheetah Conservation Fund, unpublished data). Wild local cheetah individuals may not have shown the same pattern due to being part of male coalitions, which are at lower risk from leopards due to group living. Nonetheless, ungulates in our study system appear to adjust their waterhole visitation patterns with cheetah presence (Ruble et al., 2022), suggesting a potential response to perceived predation risk. For rehabilitated local individuals, the movements here may be determined by prey distribution as certain species of prey are more water-dependent than others (Kihwele et al., 2020), but for this study we lacked the information to confirm spatial prey availability by cheetahs.
Distance to confirmed marking trees was associated with step length of the one Wild Rehabilitated Translocated cheetah included in the study, which moved longer distances when close to the marking tree. As a translocated cheetah, this female was unfamiliar with the area and minimising the time spent around marking trees might have been part of a strategy of risky conspecific avoidance involving resident male(s). Cheetahs, particularly males use marking trees repeatedly for territorial and reproductive advertising (Marker-Kraus and Kraus, 1997), whereas in leopards both sexes often scent mark (Cornhill and Kerley, 2020). Cheetahs and leopards often choose the same marking trees but exhibit temporal segregation to avoid conflict (Rafiq et al., 2020; Verschueren et al., 2021).
The movements of Rehabilitated Translocated cheetahs in relation to the hard edge between human-managed open savanna and bush encroached woodland savanna revealed a non-linear relationship. Habitat edges are often favoured by prey and also provide concealment, which can assist in hunting (Bissett and Bernard, 2007; Atkinson et al., 2022b).
Our study system experienced a decline in large prey over time and we were able to detect an adjustment in movement rates by cheetahs particularly in relation to the variability in browser and grazer ungulates. As resource availability fluctuates, cheetahs must adapt their ecological and behavioural processes (Broekhuis et al., 2019, Broekhuis et al., 2021; Rodriguez-Recio et al., 2022). Our data support the resource dispersion hypothesis, which predicts that large carnivores will expand their ranging patterns as resource availability becomes dispersed, resulting in increased movements (Macdonald, 1983; Farhadinia et al., 2016; Rodriguez-Recio et al., 2022).
Post-release monitoring of the behaviour of apex predators released as part of rewilding or reintroductions remains challenging and has rarely been compared with behaviours of locally resident animals. Based on a dataset of released and resident GPS radiocollared cheetahs and prey populations monitored over >10 years, we were able to draw inferences on factors that affect apex predator movements and began to explore the complexity of predator-prey relationships. We found extensive differences among cheetahs regarding their movement rates in relation to their familiarity with the area, rehabilitation, and ecological factors. We recommend that rewilding and reintroduction programmes monitor individuals closely using GPS tracking technology to understand movements and, for population augmentation projects, the potential effects on the resident population.
High movement rates in males suggest that it would be beneficial to rewild female cheetahs initially as they have shorter movements, and those females may encourage residency in future releases of males. Less expansive movements can be beneficial for cheetah survival in unprotected areas (Sievert et al., 2022; Cristescu et al., submitted). For example, roads pose a dynamic threat to individuals that cover extensive distances, and road density and type become paramount during the selection of individuals for release. This is due to the fact that roads contribute significantly to the mortality of wild individuals (Mohammadi et al, 2018; Mohammadi and Kaboli, 2016). Cheetahs that move longer distances, particularly through extensive exploratory behaviour, are most vulnerable to encountering risky situations through human-wildlife conflict. Our study highlighted that males in coalitions undertake longest movements, and territorial males will repeatedly use specific areas to advertise their presence and mark their territory in communication hubs (Caro, 1994; Melzheimer et al., 2020). We recommend farmers remove their livestock from these known areas, and that livestock should not be free-ranging during crepuscular or night-time periods, to avoid the most frequent activity periods of large carnivores such as cheetahs, leopards, and hyenas (Puls et al., 2021; Vissia et al., 2021, Vissia et al., 2022). Removing livestock late afternoon and releasing them again in the morning would be a good strategy for conflict mitigation. Because the success of release projects for apex predators relies heavily on prey abundance and accessibility, research could benefit from incorporating observations of diet composition and prey preference, as well as kill frequencies and kill intervals by predator age and sex class (Cristescu et al., 2022).
Although focal studies such as ours are informative behaviourally by incorporating detailed parameters such as location of marking sites and waterholes and detailed knowledge of habitats, conducting movement analyses on a broader landscape scale has its own set of advantages. A great opportunity is the availability of remote sensing data, which can be used to relate animal movement trajectories to landscape characteristics over extensive regions.
Rewilding landscapes through the restoration of species that either went locally extinct or experienced significant declines is an increasingly applied practice in biological conservation. We encourage researchers to continue to investigate the post-release movement ecology of apex predators and the effects of released animals on the food webs and ecosystems at the release sites, while not neglecting resident individuals that might be present at the sites.
The raw data supporting the conclusions of this article will be made available by the authors, without undue reservation.
The animal study was approved by National Commission on Research, Science and Technology, Government of Namibia. The study was conducted in accordance with the local legislation and institutional requirements.
JD: Conceptualization, Formal analysis, Investigation, Methodology, Writing – original draft. BC: Conceptualization, Methodology, Resources, Supervision, Writing – review & editing. KB: Formal analysis, Methodology, Writing – review & editing. NR: Conceptualization, Supervision, Writing – review & editing. LM: Resources, Supervision, Writing – review & editing.
The author(s) declare financial support was received for the research, authorship, and/or publication of this article. We thank the Cheetah Conservation Fund, its sponsors and anonymous donors for supporting this research along the entirety of the data collection period for the monitored cheetahs and the herbivore population monitoring.
This research was supported by the Cheetah Conservation Fund (CCF), Namibia and the University of Bristol, UK. The long-term dataset for this project was provided by CCF, with countless staff and interns working on the data collection since 2007. We thank Utarera Katjavivi and Matti Nghikembua for discussions related to earlier versions of the manuscript.
The authors declare that the research was conducted in the absence of any commercial or financial relationships that could be construed as a potential conflict of interest.
All claims expressed in this article are solely those of the authors and do not necessarily represent those of their affiliated organizations, or those of the publisher, the editors and the reviewers. Any product that may be evaluated in this article, or claim that may be made by its manufacturer, is not guaranteed or endorsed by the publisher.
The Supplementary Material for this article can be found online at: https://www.frontiersin.org/articles/10.3389/fcosc.2024.1351366/full#supplementary-material
Atkinson H., Cristescu B., Marker L., Rooney N. (2022a). Habitat thresholds for successful predation under landscape change. Landscape Ecol. 37, 2847–2860. doi: 10.1007/s10980-022-01512-x
Atkinson H., Cristescu B., Marker L., Rooney N. (2022b). Bush encroachment and large carnivore predation success in African landscapes: A review. Earth. 3, 1010–1026. doi: 10.3390/earth3030058
Bandyopadhyay K., Cristescu B., Koprowski J. L., Alfeus M., Beck J. L., Nghikembua M. T., et al. Declining trends in density and biomass, and incipient population halving of African herbivores in a woodland savanna.
Bissett C., Bernard R. T. F. (2007). Habitat selection and feeding ecology of the cheetah (Acinonyx jubatus) in thicket vegetation: is the cheetah a savanna specialist. J. Zool. 271, 310–317. doi: 10.1111/j.1469-7998.2006.00217.x
Broekhuis F., Elliot N. B., Keiwua K., Koinet K., Macdonald D. W., Mogensen N., et al. (2021). Resource pulses influence the spatio-temporal dynamics of a large carnivore population. Ecography. 44, 358–369. doi: 10.1111/ecog.05154
Broekhuis F., Grunewalder S., McNutt J. W., Macdonald D. W. (2014). Optimal hunting conditions drive circalunar behaviour of a diurnal carnivore. Behav. Ecol. 25, 1268–1275. doi: 10.1093/beheco/aru122
Broekhuis F., Madsen E. K., Klaasen B. (2019). Predators and pastoralists: how anthropogenic pressures inside wildlife areas influence carnivore space use and movement behaviour. Anim. Conserv. 22, 404–416. doi: 10.1111/acv.12483
Buckland S. T., Anderson D. R., Burnham K. P., Laake J. L., Borchers D. L., Thomas L. (2001). Introduction to distance sampling: Estimating abundance of biological populations (Oxford: Oxford University Press). doi: 10.1093/oso/9780198506492.001.0001
Caro T. (1994). Cheetahs of the Serengeti Plains: Group living in an asocial species (Chicago: University of Chicago Press).
Core Team R. (2021). R: A language and environment for statistical computing (Vienna: R Foundation for Statistical Computing).
Cornhill K. L., Kerley G. I. (2020). Cheetah behaviour at scent-marking sites indicates differential use by sex and social rank. Ethology. 126, 976–986. doi: 10.1111/eth.13071
Cristescu B., Boyce M. S. (2013). Focusing ecological research for conservation. Ambio. 42, 805–815. doi: 10.1007/s13280-013-0410-x
Cristescu B., Elbroch L. M., Dellinger J. A., Binder W., Wilmers C. C., Wittmer H. U. (2022). Kill rates and associated ecological factors for an apex predator. Mamm. Biol. 102, 291–305. doi: 10.1007/s42991-022-00240-8
Cristescu B., Jhala Y. V., Balli B., Qureshi Q., Schmidt-Küntzel A., Tordiffe A. S. W., et al. Spatial ecology of cheetahs in India: complexities beyond extrapolation from Africa.
Cristescu B., Stenhouse G. B., Boyce M. S. (2015). Predicting multiple behaviours from GPS radiocollar cluster data. Behav. Ecol. 26, 452–464. doi: 10.1093/beheco/aru214
Crosmary W. G., Valeix M., Fritz H., Madzikanda H., Cote S. D. (2012). African ungulates and their drinking problems: hunting behaviour and predation risks constrain access to water. Anim. Behav. 83, 145–153. doi: 10.1016/j.anbehav.2011.10.019
Durant S. M., Bashir S., Maddox T., Laurenson M. K. (2007). Relating long-term studies to conservation practice: the case of the Serengeti Cheetah Project. Conserv. Biol. 21, 602–611. doi: 10.1111/j.1523-1739.2007.00702.x
Durant S. M., Mitchell N., Groom R., Pettorelli N., Ipavec A., Jacobson A. P., et al. (2017). The global decline of cheetah Acinonyx jubatus and what it means for conservation. P. Natl. Acad. Sci. U.S.A. 114, 528–533. doi: 10.1073/pnas.1611122114
Fabiano E. C., Sutherland C., Fuller A. K., Nghikembua M., Eizirik E., Marker L. (2020). Trends in cheetah Acinonyx jubatus density in north-central Namibia. Popul. Ecol. 62, 233–243. doi: 10.1002/1438-390X.12045
Farhadinia M. S., Gholikhani N., Behnoud P., Hobeali K., Taktehrani A., Hosseini-Zavarei F., et al. (2016). Wandering the barren deserts of Iran: illuminating high mobility of the Asiatic cheetah with sparse data. J. Arid Environ. 134, 145–149. doi: 10.1016/j.jaridenv.2016.06.011
Fritts S. H., Bangs E. E., Fontaine J. A., Johnson M. R., Phillips M. K., Koch E. D., et al. (1997). Planning and implementing a reintroduction of wolves to Yellowstone National Park and central Idaho. Restor. Ecol. 5, 7–27. doi: 10.1046/j.1526-100X.1997.09702.x
Hayward M. W., Kerley G. I., Adendorff J., Moolman L. C., O’Brien J., Sholto-Douglas A., et al. (2007). The reintroduction of large carnivores to the Eastern Cape, South Africa: an assessment. Oryx. 41, 205–214. doi: 10.1017/S0030605307001767
Hayward M. W., Slotow R. (2009). Temporal partitioning of activity in large African carnivores: tests of multiple hypotheses. S. Afr. J. Wildl. Res. 39, 109–125. doi: 10.3957/056.039.0207
Jhala Y. V., Qureshi Q., Nayak A. K. (2020). Status of tigers, copredators and prey in Indi (New Delhi: National Tiger Conservation Authority, Government of India, and Wildlife Institute of India).
Jhala Y. V., Ranjitsinh M. K., Bipin C. M., Yadav S. P., Kumar A., Mallick A., et al. (2021). Action plan for introduction of cheetah in India (Wildlife Institute of India, National Tiger Conservation Authority and Madhya Pradesh Forest Department).
Kihwele E. S., Mchomvu V., Owen-Smith N., Hetem R. S., Hutchinson M. C., Potter A. B., et al. (2020). Quantifying water requirements of African ungulates through a combination of functional traits. Ecol. Monogr. 90, e01404. doi: 10.1002/ecm.1404
Krag C., Havmøller L. W., Swanepoel L., Van Zyl G., Møller P. R., Havmøller R. W. (2023). Impact of artificial waterholes on temporal partitioning in a carnivore guild: a comparison of activity patterns at artificial waterholes to roads and trails. PeerJ 11, e15253. doi: 10.7717/peerj.15253
Lennox R. J., Brownscombe J. W., Darimont C., Horodysky A., Levi T., Raby G. D., et al. (2022). The roles of humans and apex predators in sustaining ecosystem structure and function: Contrast, complementarity and coexistence. People Nature. 4, 1071–1082. doi: 10.1002/pan3.10385
Loveridge A. J., Sousa L. L., Cushman S., Kaszta Z., Macdonald D. W. (2022). Where have all the lions gone? Establishing realistic baselines to assess decline and recovery of African lions. Ecography. 28, 2388–2402. doi: 10.1111/ddi.13637
Macdonald D. W. (1983). The ecology of carnivore social behaviour. Nature. 301, 379–384. doi: 10.1038/301379a0
Marker L. L., Cristescu B., Dickman A., Nghikembua M. T., Boast L., Morrison T., et al. (2018b). “Ecology of free-ranging cheetahs,” in Cheetahs: biology and conservation. Eds. Nyhus P. J., Marker L., Boast L., Schmidt-Kuntzel A. (Elsevier, London), 107–119. doi: 10.1016/B978-0-12-804088-1.00008-3
Marker L. L., Cristescu B., Morrison T., Flyman M. V., Horgan J., Sogbohossou E. A., et al. (2018a). “Cheetah rangewide status and distribution,” in Cheetahs: biology and conservation. Eds. Nyhus P. J., Marker L., Boast L., Schmidt-Kuntzel A. (Elsevier, London), 33–54. doi: 10.1016/B978-0-12-804088-1.00004-6
Marker L. L., Dickman A. (2004). Human aspects of cheetah conservation: lessons learned from the Namibian farmlands. Hum. Dimens. Wildl. 9, 297–305. doi: 10.1080/10871200490505729
Marker L. L., Dickman A. J., Mills M. G. L., Jeo R. M., Macdonald D. W. (2008). Spatial ecology of cheetahs on north-central Namibian farmlands. J. Zool. 274, 226–238. doi: 10.1111/j.1469-7998.2007.00375.x
Marker L., Dickman A., Wilkinson C., Schumann B., Fabiano E. (2007). The Namibian cheetah: status report. Cat News. 2, 4–13.
Marker-Kraus L., Kraus D. (1997). “Conservation strategies for the long-term survival of the Cheetah,” in International zoo yearbook, vol. 35. , 59–66.
Marneweck C., Becker P. A., Beverley G., Davies-Mostert H. T., Du Plessis C., Forssman K., et al. (2019). Factors affecting the success of artificial pack formation in an endangered, social carnivore: the African wild dog. Anim. Conserv. 22, 493–502. doi: 10.1111/acv.12490
Melzheimer J., Heinrich S. K., Wasiolka B., Mueller R., Thalwitzer S., Palmegiani I., et al. (2020). Communication hubs of an asocial cat are the source of a human–carnivore conflict and key to its solution. Proc. Natl. Acad. Sci. 117, 33325–33333. doi: 10.1073/pnas.2002487117
Mohammadi A., Almasieh K., Clevenger A. P., Fatemizadeh F., Rezaei A., Jowkar H., et al. (2018). Road expansion: A challenge to conservation of mammals, with particular emphasis on the endangered Asiatic cheetah in Iran. J. Nat. Conserv. 43, 8–18. doi: 10.1016/j.jnc.2018.02.011
Mohammadi A., Kaboli M. (2016). Evaluating wildlife-vehicle collision hotspots using kernel-based estimation: a focus on the endangered Asiatic cheetah in Central Iran. Hum. Wildl. Interact. 10, 1–13. doi: 10.26077/0xjd-az08
Naha D., Dash S. K., Kupferman C., Beasley J. C., Sathyakumar S. (2021). Movement behaviour of a solitary large carnivore within a hotspot of human-wildlife conflicts in India. Sci. Rep. 11, 1–14. doi: 10.1038/s41598-021-83262-5
Nghikembua M., Harris J., Trengenza T., Marker L. (2016). Spatial and temporal habitat use by GPS collared male cheetahs in modified bushland habitat. Open J. Forest. 6, 269–280. doi: 10.4236/ojf.2016.64022
Nghikembua M. T., Marker L. L., Brewer B., Leinonen A., Mehtatalo L., Appiah M., et al. (2023). Response of woody vegetation to bush thinning on freehold farmlands in north-central Namibia. Sci. Rep. 13, 1–14. doi: 10.1038/s41598-022-26639-4
Nghikembua M. T., Marker L. L., Brewer B., Leinonen A., Mehtatalo L., Appiah M., et al. (2021). Restoration thinning reduces bush encroachment on freehold farmlands in north-central Namibia. Forestry: Int. J. For. Res. 94, 551–564. doi: 10.1093/forestry/cpab009
Odden M., Athreya V., Rattan S., Linnell J. D. (2014). Adaptabl neighbours: movement patterns of GPS-collared leopards in human dominated landscapes in India. PloS One 9, e112044. doi: 10.1371/journal.pone.0112044
Puls S., Teichman K. J., Janse C., O’Riain M. J., Cristescu B. (2021). Activity patterns of leopards (Panthera pardus) and temporal overlap with their prey in an arid depredation hotspot of southern Africa. J. Arid Environ. 187, 104430. doi: 10.1016/j.jaridenv.2020.104430
Rafiq K., Jordan N. R., Meloro C., Wilson A. M., Hayward M. W., Wich S. A., et al. (2020). Scent-marking strategies of a solitary carnivore: boundary and road scent marking in the leopard. Anim. Behav. 161, 115–126. doi: 10.1016/j.anbehav.2019.12.016
Ripple W. J., Estes J. A., Beschta R. L., Wilmers C. C., Ritchie E. G., Hebblewhite M., et al. (2014). Status and ecological effects of the world’s largest carnivores. Science. 343, e1241484. doi: 10.1126/science.1241484
Rodriguez-Recio M., Burgos T., Krofel M., Lozano J., Moleon M., Virgos E. (2022). Estimating global determinants of leopard home range size in a changing world. Anim. Conserv. 25, 748–758. doi: 10.1111/acv.12777
Ruble D. B., Verschueren S., Cristescu B., Marker L. L. (2022). Rewilding apex predators has effects on lower trophic levels: Cheetahs and ungulates in a woodland savanna. Animals. 12, 3532. doi: 10.3390/ani12243532
Searle C. E., Smite J. B., Cusack J. J., Strampelli P., Grau A., Mkuburo L., et al. (2021). Temporal partitioning and spatiotemporal avoidance among large carnivores in a human-impacted African landscape. PloS One 16, e0256876. doi: 10.1371/journal.pone.0256876
Seid A., Adugna C., Atickem A., Yihune M., Bekele A. (2022). Habitat suitability and distribution of endangered Ethiopian wolf (Canis simensis) and the potential effects of climate change on its habitat in the Ethiopian highlands. Ethiopian J. Biol. Sci. 21, 131–155. doi: 10.21203/rs.3.rs-1618917/v1
Shaw A. K. (2020). Causes and consequences of individual variation in animal movement. Move. Ecol. 8, 1–12. doi: 10.1186/s40462-020-0197-x
Sievert O., Fattebert J., Marnewick K., Leslie A. (2022). Assessing the success of the first cheetah reintroduction in Malawi. Oryx. 56, 505–513. doi: 10.1017/S0030605321000788
Teichman K. J., Cristescu B., Crevier L., O’Riain M. J., Hodges K. E. (2023). Movement choices of persecuted caracals on farmlands in South Africa. Rangeland Ecol. Manage. 88, 77–84. doi: 10.1016/j.rama.2023.02.004
Thurfjell H., Ciuti S., Boyce M. S. (2014). Applications of step-selection functions in ecology and conservation. Movement Ecol. 2, 1–12. doi: 10.1186/2051-3933-2-4
Turchin P. (1998). Quantitative analysis of movement: Measuring and modelling population redistribution of plants and animals (Sunderland: Sinauer Associates).
Van der Weyde L. K., Hubel T. Y., Horgan J., Shotton J., McKenna R., Wilson A. M. (2017). Movement patterns of cheetahs (Acinonyx jubatus) in farmlands in Botswana. Biol. Open 6, 118–124. doi: 10.1242/bio.021055
Verschueren S., Briers-Louw W. D., Cristescu B., Fabiano E., Nghikembua M., Torres-Uribe C., et al. (2021). Spatiotemporal sharing and partitioning of scent-marking sites by cheetahs and leopards in north-central Namibia. Afr. J. Ecol. 59, 605–613. doi: 10.1111/aje.12878
Vissia S., Fattebert J., van Langevelde F. (2022). Leopard density and interspecific spatiotemporal interactions in a hyena-dominated landscape. Ecol. Evol. 12, e9365. doi: 10.1002/ece3.9365
Vissia S., Wadhwa R., van Langevelde F. (2021). Co-occurrence of high densities of brown hyena and spotted hyena in central Tuli, Botswana. J. Zool. 314, 143–150. doi: 10.1111/jzo.12873
Walker E., Verschueren S., Schmidt-Kuntzel A., Marker L. (2022). Recommendations for the rehabilitation and release of wild-born, captive-raised cheetahs: the importance of pre- and post-release management for optimizing survival. Oryx. 56, 495–504. doi: 10.1017/S0030605321000235
Weise F. J., Lemeris J. R. Jr., Munro S. J., Bowden A., Venter C., van Vuuren M., et al. (2015). Cheetahs (Acinonyx jubatus) running the gauntlet: An evaluation of translocations into free-range environments in Namibia. PeerJ. 3, e1346. doi: 10.7717/peerj.1346
Keywords: behavioural adjustments, bush encroachment, ecosystem restoration, large carnivore, movement ecology, restoration ecology, translocation, wildlife rehabilitation
Citation: Dimbleby J, Cristescu B, Bandyopadhyay K, Rooney NJ and Marker L (2024) Rewilding landscapes with apex predators: cheetah (Acinonyx jubatus) movements reveal the importance of environmental and individual contexts. Front. Conserv. Sci. 5:1351366. doi: 10.3389/fcosc.2024.1351366
Received: 06 December 2023; Accepted: 15 April 2024;
Published: 08 May 2024.
Edited by:
Rene Beyers, University of British Columbia, CanadaReviewed by:
Muhammad Ali Nawaz, Qatar University, QatarCopyright © 2024 Dimbleby, Cristescu, Bandyopadhyay, Rooney and Marker. This is an open-access article distributed under the terms of the Creative Commons Attribution License (CC BY). The use, distribution or reproduction in other forums is permitted, provided the original author(s) and the copyright owner(s) are credited and that the original publication in this journal is cited, in accordance with accepted academic practice. No use, distribution or reproduction is permitted which does not comply with these terms.
*Correspondence: Nicola Jane Rooney, bmljb2xhLnJvb25leUBicmlzdG9sLmFjLnVr
†ORCID: Bogdan Cristescu, orcid.org/0000-0003-2964-5040
Kathan Bandyopadhyay, orcid.org/0000-0002-8342-4049
Nicola Jane Rooney, orcid.org/0000-0001-9629-1700
Laurie Marker, orcid.org/0000-0002-1636-2191
Disclaimer: All claims expressed in this article are solely those of the authors and do not necessarily represent those of their affiliated organizations, or those of the publisher, the editors and the reviewers. Any product that may be evaluated in this article or claim that may be made by its manufacturer is not guaranteed or endorsed by the publisher.
Research integrity at Frontiers
Learn more about the work of our research integrity team to safeguard the quality of each article we publish.