- 1ThINK – Thuringian Institute of Sustainability and Climate Protection GmbH, Jena, Germany
- 2Friedrich Schiller University Jena, Faculty of Biological Sciences, Institute of Ecology and Evolution, Jena, Germany
- 3GLU Gesellschaft für Geotechnik, Landschafts- und Umweltplanung mbH, Jena, Germany
Despite the sensitivity of ecosystems in extreme conditions, only 0.029% of the Antarctic continent is currently granted special protection as Antarctic Specially Protected Area (ASPA). Additionally, protected areas are unevenly distributed and unrepresentative of the biodiversity of Antarctica. The Antarctic Treaty Consultative Parties are thus striving to establish a more coherent network of protected areas. In this context, the Otto-von-Gruber-Gebirge was identified as an area highly worthy of protection due to its unique ecosystem including one of the largest snow petrel breeding sites, and relative pristine nature. However, in the process of designation, an update of knowledge, particularly of the population status of snow petrel in the area, was recognized to be needed. This study was aimed at estimating a population census of snow petrel breeding pairs in the Lake Untersee catchment, an important subarea of the potentially protected area. Investigations were severely limited by the remoteness and harsh conditions of the area, enabling only a short fieldwork period with limited resources. Thus, a combination of remote sensing and traditional methods was applied. We conducted a ground survey of a smaller reference area, including locating nest sites and assessing nest site characteristics. Snow petrels usually nest in the cavities of large boulders and we thus classified habitat suitability based on boulder presence. To do so, the study area was remotely surveyed by unpiloted aerial vehicles to gain orthophotomosaics in sufficient resolution to distinguish on-ground conditions for breeding. We then calculated nest site densities for the reference area. We also studied nest site data such as nest cavity depth, orientation, and attendance to gain basic knowledge of the characteristics of the breeding site. Finally, we measured a sample of four live snow petrels to determine which morph was present at the site. We were able to obtain nest site data and to extrapolate it, which produced population numbers of 11,765 breeding pairs for the whole study area. This underpins the size of the breeding site and thereby its importance for the species.
1 Introduction
Areas that are exposed to only minor human influence (Pertierra et al., 2017) provide unique opportunities for wilderness conservation, as they are refuges for rare ecosystems. However, these places are often difficult for humans to access and thus, little data is typically available to inform the management of such areas. An efficient way to collect data on such areas is through remote sensing (Turner, 2014). A variety of platforms and sensors is available to support the characterization of the biophysical environment of an area and fill data gaps in the spatial and temporal coverage of in situ observations (Skidmore et al., 2021; Cavender-Bares et al., 2022). However, remote sensing-based methods can be limited by spatial and temporal resolution and by the detectability of the feature or parameter of interest (cf. Joseph, 2017).
The Antarctic continent covers 14 million square kilometers (Kleinschmidt, 2021). At first glance, it may appear to be an empty and lifeless desert. On a closer look, however, this continent contains unique ecosystems that, in many cases, are still unknown. Due to the extreme environmental conditions, surprising life forms [e.g. endolithic algae or stromatolites (Friedmann and Ocampo, 1976; Andersen et al., 2011)] have often emerged there, which are also very sensitive due to these conditions. Threats to Antarctic ecosystems arise not only from the increasing impact of global processes such as climate change and the uptake of pollutant emissions, but also from the continuing advance of humans into previously untouched areas through tourism and scientific field work. Both research and protection of these systems are, therefore, very important.
The status of Antarctica under international law is determined by the Antarctic Treaty. This treaty was signed in 1959 and covers the Antarctic continent and its surrounding marine areas south of 60° south latitude. Under the Environmental Protocol to the Antarctic Treaty (ATS, 1991a), which entered into force in 1998, the Parties agreed on concrete regulations for the protection of the Antarctic environment and its associated ecosystems. Annex V of the Environmental Protocol contains the regulations on protected areas. According to these regulations, particularly valuable areas can be designated as Antarctic Specially Protected Areas (ASPAs). To date, 75 such ASPAs have been designated in the Antarctic. Thereby, 4,025 km² of the Antarctic is currently granted special protection (ATS, 1991b; Terauds, 2016) which is only 0.029% of the total. However, not only is the total area of protected sites small, but they are also spatially very unevenly distributed and considered unrepresentative of the biodiversity of the continent (Terauds et al., 2012; Shaw et al., 2014; Hughes et al., 2016; Terauds and Lee, 2016; Coetzee et al., 2017; Hughes and Grant, 2017; Wauchope et al., 2019; Phillips et al., 2022). This is mainly because logistics in Antarctica are complex and expensive. The activities of researchers and thus knowledge about the protected values are therefore often concentrated around scientific stations.
Due to these deficits, the Antarctic Treaty Consultative Parties are striving to establish and continuously expand a coherent network of protected areas in Antarctica. With this aim, an initiative of the Treaty Parties took place during the preparation of the XLII Antarctic Treaty Consultative Meeting (ATCM) 2019 and included the “Joint CEP/SCAR Workshop on Further Developing the Antarctic Protected Area System”. As a result, the Committee for Environmental Protection (CEP) as a permanent body of the ATCM encouraged Treaty Parties to continue efforts to expand the network of Antarctic protected areas based on scientifically collected data (ATCM, 2019).
In this context, after a consideration process of finding a suitable area integrating a large number of values to be protected, Germany elaborated a proposal for the designation of an ASPA in the mountains of Otto-von-Gruber-Gebirge. This high mountain range (see Figure 1) is a continental inland oasis at the north-eastern end of the Wohlthatmassiv, Queen Maud Land. It is about 90 km southeast of the Schirmacher Oasis. It has an area of about 300 km². In accordance with the regulations of the Antarctic Treaty, a prior assessment was carried out on the basis of existing data and presented by Germany and the USA at CEP XXIV 2022. CEP approved this proposal in principle although with the concern that some of the underlying data were out-of-date and in urgent need of updating. CEP expressed particular concern about the data on a large breeding site of snow petrels (Pagodroma nivea) in the area (ATCM, 2022).
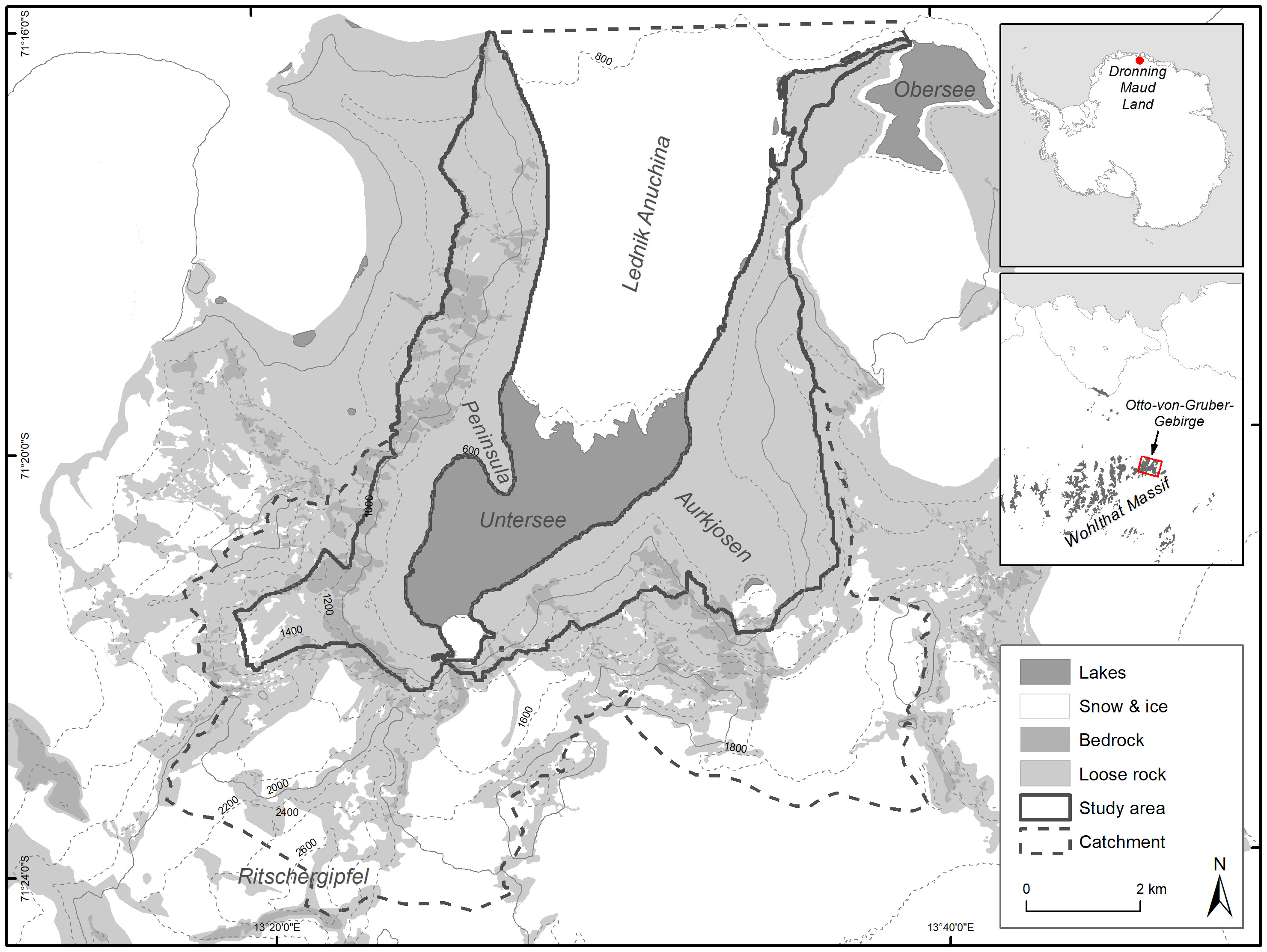
Figure 1 Overview of the study area, its position on the Antarctic continent and geographical features.
The snow petrel is an entirely white plumaged bird about 0.3-0.4 m long and weighing about 0.24-0.46 kg. The wingspan is 0.75-0.95 m. It has a circumpolar distribution, nesting on islands or at (mainly coastal) inland breeding sites, but with hunting grounds strongly associated with pack ice (Marchant and Higgins, 1990). However, occasional breeding sites occur more than 400 km inland (Goldsworthy and Thomson, 2000). Nest sites are in small caves on cliffs, steep slopes, in crevices and below boulders and occasionally in the open or under overhangs. They breed from late November to mid-March, with no variation between breeding sites (Marchant and Higgins, 1990). During breeding, both adults take shifts with one parent brooding and the other foraging. At end of November/beginning of December, a breeding shift lasts about a week, with change overs occurring at night (Marchant and Higgins, 1990). According to the IUCN, snow petrel populations are stable, exceeding 4,000,000 individuals, and the species is listed as of least concern (BirdLife International, 2018). Two subspecies of snow petrels (P.n. major/confusa or P.n. minor/nivea) have been recognized based on size differences (Bonaparte, 1856; Falla, 1937; Prevost, 1969). Nevertheless, most breeding sites seem to be mixed, with only few known to host only one subspecies (Marchant and Higgins, 1990). According to newer literature, including genetic investigations, it is at least questionable if speciation is complete and the term subspecies might not be appropriate (Jouventin and Bried, 2001). They propose instead to use the term “morph”.
The first records of snow petrel breeding sites in the western Wohlthatmassiv come from the late 1950s (Lovenskiold, 1960). The breeding site in the Otto-von-Gruber-Gebirge itself was noted on a map a few years later (Konovalov, 1962). This map gave an overview of the different bird species in Queen Maud Land. Neither this Russian publication nor its English version (Konovalov, 1964) provide quantitative data even though various authors (see below) have cited them for this purpose. The next refinement of this information occurred through reports of nests under rocks on the western shore of Lake Untersee (Kosenko and Kolobov, 1970). Lake Untersee is a dominant feature of the Otto-von-Gruber-Gebirge and the largest surface freshwater lake of this mountain range. The first estimate of snow petrel numbers of the slopes around Lake Untersee, was of 1,000 breeding pairs (Simonov et al., 1985). Hiller et al. (1988), on the basis of Kosenko and Kolobov, 1970 and Simonov et al., 1985, estimated the population at 10,000 breeding pairs. They also compiled the first map of the distribution of the birds around Lake Untersee and noted that 90% of the occupied nest sites are located below 850 m above sea level (a.s.l.) in snow- and ice-free moraines where the birds preferentially use cavities under or between large boulders. From then on, the number of 10,000 breeding pairs was cited various times (Croxall et al., 1995; Harris et al., 2015) and served as the basis for designating the locality as an Important Bird Area (Harris et al., 2015), but no new estimates were published. If the figure of 10,000 breeding pairs is correct, the locality is one of the most populous snow petrel sites in the world (Croxall et al., 1995). Great attention was paid to the remarkable deposits of stomach oil (mumiyo) which can be found in and around many active or inactive nest sites (Konovalov, 1962; Kosenko and Kolobov, 1970; Simonov et al., 1985; Hiller et al., 1988, 1995; McClymont et al., 2021).
There are two main causes for the very low availability of data on the snow petrel site in the Otto-von-Gruber-Gebirge. Firstly, the area is very rarely visited by scientists due to its distance from infrastructure and the difficult logistics. Secondly, the breeding site is very large and the nest sites difficult to detect. In cases of large or not completely accessible animal aggregations, where actual count data are only available for parts of the population, it is an established method to extrapolate densities from small parts to the whole area. At another snow petrel breeding site, extrapolation was used to estimate breeding pair numbers for a large area (Olivier et al., 2004). For other Antarctic species, this has also been done using remote sensing data at different scales (Fretwell et al., 2012; Naveen et al., 2012; Schwaller et al., 2018). It has also been shown that habitat structure was a suitable predictor for snow petrel nest presence (Olivier and Wotherspoon, 2008). In addition to the lack of data on the distribution of snow petrels within the area, there is also very limited knowledge of the breeding biology except for some comments on nest site choice (Hiller et al., 1988).
The scope of this study is to provide new information on the distribution of snow petrels within the proposed area under protection as ASPA. Due to the sheer size of the study area, the snow petrel population could only be surveyed with the help of remote sensing. As the species breeds hidden under rocks, the nests cannot be recognized directly by satellite or UAV remote sensing. The stomach oil deposits around the nests are also barely recognizable when viewed vertically. Therefore, we developed an approach to detect suitable breeding habitat from very-high resolution UAV imagery. With the usage of this classification, we aimed to refine an extrapolation of nest site densities from reference to study area by habitat suitability. Nest site data was recorded to gain knowledge of the species and its breeding biology in the area. This knowledge was the basis for the development of the extrapolation approach and can be used for better design of future studies.
2 Materials and methods
2.1 Study area
Lake Untersee is enclosed by an arc of mountains up to 2,810 m high (Peak Ritschergipfel) on the east, west and north. From the north, the glacier Lednik Anuchina flows southwards for about 6 km into the main valley and delimits Lake Untersee to the north (Schwab, 1998). There is a cirque with steep slopes at the southwestern end of Lake Untersee and the southeastern end of Aurkjosen valley (a side valley in the southeast). Here there are also small secondary and tertiary glaciers (Wand et al., 1996) below icefalls. Lake Untersee is about 6.5 km long and 2.5 km wide (Loopmann et al., 1986). The lake surface is at about 610 m a.s.l. and perennially covered with ice of average thickness 2.77 m (Faucher et al., 2019). The flanks of the valley are covered by moraines. Meteorological data from Lake Untersee (2008-2017) show a mean annual air temperature of -9.7°C and a mean relative humidity of 42%. There are strong south and east winds with an average speed of 5.4 m/s and frequent strong gusts. During the austral summer (December-February), the average air temperature is about -2.65° C, the maximum 6.78 and the minimum -12.66° C (Andersen et al., 2015; Faucher et al., 2019). Our study covers an area of 39 km² that represents the ice-free part of the catchment of Lake Untersee excluding high-elevated areas and rock faces which are no snow petrel breeding habitat (see Figure 1).
2.2 Field work
All field work was conducted by three people between November 27th and December 13th, 2022, in the catchment of Lake Untersee.
2.2.1 Ground surveys and nest site data
An area of 0.82 km², hereafter called the reference area, was surveyed on foot. To account for geographical and morphological differences, it was divided into three subareas (see Table 1; Supplementary Material I). The survey was conducted on 8 days between 30th of November and 11th of December.
We seamlessly searched the entire reference area once for snow petrels and snow petrel nests. We usually conducted searches from 10 AM to 6 PM UTC. Nest sites were identified by the presence of mumiyo and guano at the entrance or inside of suitable cavities. A nest site was classified as active if at least one bird was seen or heard inside the nest cavity. Examples of snow petrel nesting sites can be viewed in Supplementary Material I. If a nest was detected, its location was recorded using GNSS equipped tablets (Samsung Galaxy Tab Active3 Enterprise Edition SM-T575N) noting the following site data:
● Boulder Size (under which the nest is located) [<0.5 m; 0.5-1.0 m; 1.0-2.0 m; >2.0 m],
● Visibility [exposed, intermediate, concealed] (single nests only, see Supplementary Material I),
● Nest cavity depth [estimated (mostly horizontal) distance from entrance of the cavity to the nest, to within 0.05 m] (single nests only),
● Nest orientation [cardinal points] (single nests only),
● Active/inactive nests [active: at least one adult bird present],
● Attendance of adult birds (1 or 2, single nests only).
In some cases, several nests were found near to each other under a single boulder or under several neighboring boulders, so that recording them individually was impractical. In these cases, nest aggregations were recorded as polygons, noting the number of nests (whether active or inactive) and the size of the dominant boulders.
In addition to the intensive nest search in the reference area, we wanted to get an estimate of general distribution of snow petrels within the valley. These observations were used as a plausibility test of the distribution of nesting site densities found during habitat classification. Therefore, we screened an area within about 1.5 km from Lake Untersee, the western edge of the Lednik Anuchina, and the Aurkjosen valley for snow petrel presence. Using binoculars, all surface areas visible were each screened once for a few minutes. We identified areas of relatively high abundance as those where small groups (usually more than ten individuals) of snow petrels were almost constantly present, flying low over boulders and rocks, standing on them and interacting with neighboring birds. We identified as low abundance those areas where we usually saw only single or few birds showing these behaviors. Areas of no visible abundance were those where any petrels seen were clearly only in transit. We manually marked areas of high and low abundance on the map. These areas have been visually compared to the densities assigned during habitat suitability classification.
2.2.2 UAV surveys
To capture very high-resolution and current images of the study area, we used the Trinity F90+ UAV. This is an electrically powered fixed wing UAV with vertical take-off and landing (VTOL) capabilities. VTOL combines the advantages of the long flight range of fixed wing aircraft and the advantage of not needing a runway of rotary-wing aircraft like the common multicopter UAVs. The Trinity F90+ has a wingspan of 2.39 m and a maximum take-off weight of 5.5 kg. It has three rotors and is powered by a 12 Ah LiPo battery. The cruising speed is 17 m/s and the maximum flight time is 60-90 minutes, depending on the air temperature, air pressure and wind conditions. These conditions result in a flight range of approximately 60 - 90 km. Mission planning was conducted with the flight-planning software QBase 3D v.2.30.77 allowing custom DEM and base map images. As a basis for flight planning we used the REMA v1 DSM (8 m GSD) (Howat et al., 2019) and a mosaic of two high resolution Worldview-2 images (0.5 m GSD) from January 14th and February 21st 2021. Flights were planned at a flight height of 260 m AGL in a single grid pattern with a forward overlap of 85% and a side overlap of 65% of the inboard camera. To ensure a constant flight level, terrain following based on REMA DSM was used. The camera was a Sony UMC-10C digital RGB camera with a 20.1 MP APS-C sensor and a 16 mm Sony SEL16F28 lens. Images were triggered approximately every 2.5 seconds over the area of interest in JPG format and had a GSD of 70 mm. The exposure time and aperture were set to a fixed 1/1000 s and F8.0, the ISO rating was set to automatic. During surveys in the late evening (low light conditions), some flights were also flown with a fixed aperture of F2.8 and subsequently lower ISO rating but resulting image quality was worse than with higher ISO and F8.0. The flights were performed fully automatically based on the pre-planned flight route, the inboard GNSS receivers and Inertial Measurement Units (IMU). Starts and landings took place on the lake ice at 600 m above the geoid (EGM96). Some flights were performed beyond visual line of sight (BVLOS), the maximum distance to the start was 10 km and the maximum flight height was 1,720 m above the geoid. To increase the accuracy of the UAV positioning, Post-Processed Kinematic (PPK) (Žabota and Kobal, 2021) was carried out after the flights with QBase 3D. The GNSS signal used for the PPK correction was logged during the flight operations with a u-blox ANN-MB multi-band GNSS antenna, located close to the starting site. The air temperature near the ground during flights was around -5°C.
We were able to conduct 19 flights, using the methods described above, on four flight days (November 30th, December 1st, 8th & 9th 2022) during which a total of 8,500 individual aerial photographs were taken, covering an area of 50 km² of which 39 km² belong to the study area.
In post-processing, we corrected the position of the UAV during camera exposure by PPK and geotagged the single photos. We created one orthophotomosaic (GSD 70 mm) with the photogrammetry software Agisoft Metashape Pro v.1.8.4 (RRID : SCR_018119) using all photos of all flights (Over et al., 2021) (Supplementary Material II). As the flights took place on different days and at different times of day, the weather conditions varied. In particular, the different amounts of cloud cover caused different conditions of light and shadow in some parts of the final orthophotomosaic.
2.2.3 Morph differentiation
To identify the present morphs in the study area, it was necessary to measure the body size of adult snow petrels. For morph differentiation, we sampled four live birds for wing length, and also measured wing length of mummies and isolated wings to provide additional rare measurements from the field for potential comparisons to museum collections and for future reference. However, we did not use those measures for morph designation due to possible tissue shrinkage after the bird dies and the unavailability of data from the two morphs for comparison. The measures included, where possible:
● Wing length [mm] (flattened, all specimen)
● Bill length [mm] (living birds and most of the mummies)
● Bill width [mm] (see bill length)
● Cranium [mm] (living birds only)
● Tarsus [mm] (living birds and mummies)
● Weight [g] (living birds only).
2.3 Analyses
Boulder size was observed to be a key feature for occurrence of snow petrel nests during field work as well as in literature (Simonov et al., 1985). We thus applied a habitat suitability classification based on the density of large, suitable boulders. The aim of this classification was to be able to refine the extrapolation of nest site densities from the reference to the study area by habitat suitability. We used the nest data acquired during ground surveys within the reference area to determine the suitable boulder size (comp.). In addition to its size, a boulder was defined as suitable when its base was not filled with fine sediment. The whole study area was subdivided into grid cells of 25 x 25 m. Following (Braun, 2005), the cell size was defined by the home range of snow petrels (e.g. the area defended around the nest as observed during field work) and the landscape patchiness. Using the imagery from UAV surveys, each grid cell was manually assigned to one of three habitat suitability classes (see examples in Figure 2):
● Solitar: only a few (<10) suitable boulders of >1 m diameter.
● Intermediate: scattered suitable boulders over the whole grid, > 9 boulders.
● Dense: many (>9) suitable boulders, situated closely together, filling most of the grid cell.
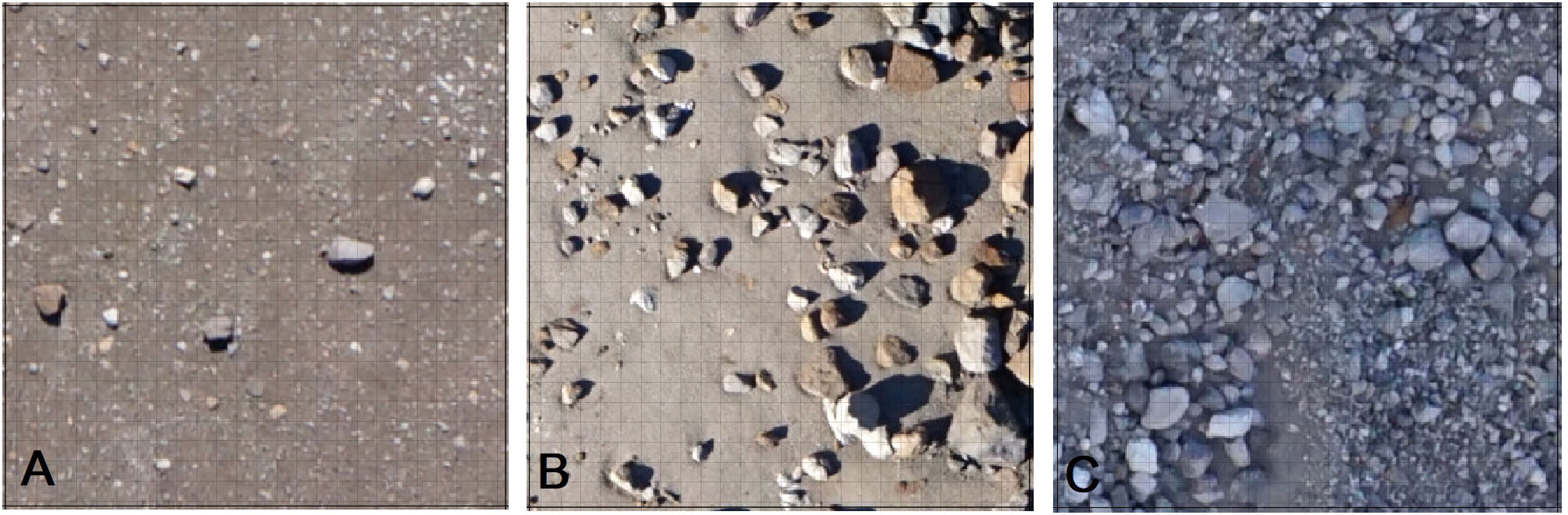
Figure 2 Example grid cells of 25 x 25 m showing the three habitat suitability classes used to classify the whole study area from mosaics acquired by UAV surveys. Small grid cells depict 1 m for identification of suitable boulders. (A) Solitary class: few suitable boulders. (B) Intermediate class: >9 suitable boulders, scattered. (C) Dense class: many suitable boulders close together.
Nest number (active nests) and nest site number (active and inactive nests) were calculated for each grid cell within the reference area using the ground survey data. The designation as active nest was based on the presence of at least one adult bird at the nest. Inactive nests were all nest sites with visible signs of usage (mumiyo, carcasses, guano) but without bird presence. It was not possible to distinguish inactive nests that were recently (e.g., this season) or historically active An ANOVA and subsequent pairwise t-test were used to test for differences between means of both values in the three habitat suitability classes. We then used the mean values of both variables for each habitat class to extrapolate the population data from reference area to the whole study area according to the assigned classification. To estimate the range of possible breeding pair numbers in the whole area based on differences in densities within the reference area, we calculated the confidence interval for our count data and extrapolated the upper and lower limits as well as means. We performed all statistical analyses and calculations in R (R Core Team, 2018; RRID : SCR_001905) using RStudio (RStudio Team, 2016; RRID : SCR_000432) and MS Excel (RRID : SCR_016137). Statistics were executed using base packages. Graphs were designed using ggplot (Wickham, 2016). GIS Analyses and cartography were carried out in QGIS (RRID : SCR_018507) and ArcGIS (RRID : SCR_011081).
3 Results
3.1 Population census
In the reference area, 1,036 nests were detected by ground survey either as single nests or nest aggregations (see Figure 3; Supplementary Material III). Aggregations (several nests at one or few boulders) were mainly found in subarea A (scree slopes) and only scattered within the other areas. In general, even in the flatter regions, nests were mostly detected on ridges.
After assignment of habitat classification to the 25 x 25 m grid cells within the reference area, we calculated the densities of nest sites and active nests for each class based on the location of nests found during ground surveys (Table 2). The dense class had the highest mean density, 23.8 nests per grid (0.038 nests per m²) and the solitary class the lowest mean density, 0.177 nests per grid (0.0003 nests per m²) (Figure 4). The density was highly significantly different between habitat suitability classes (ANOVA, df=2; F=209, p<0.0001), with significant differences between all three classes (pairwise t-test; p<0.0001 for each comparison).
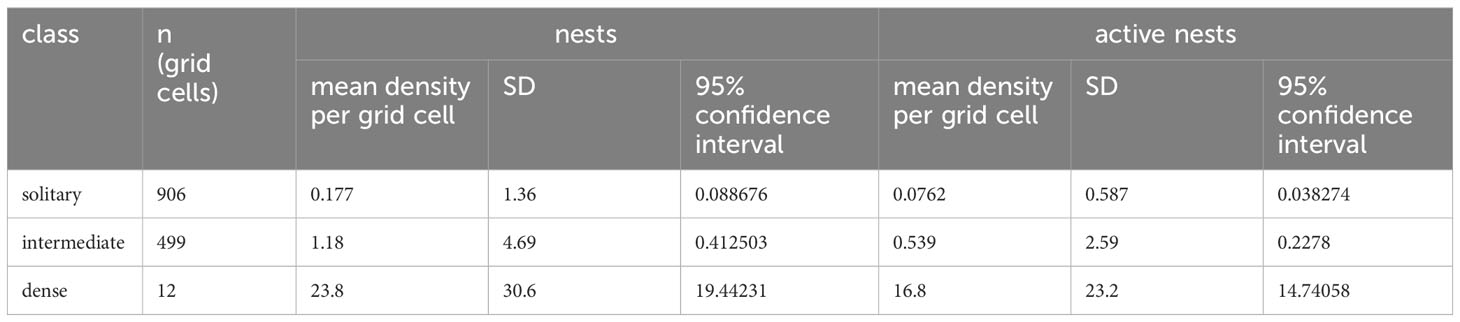
Table 2 Results of calculation of densities of snow petrel nests and aggregations in habitat classification grids within reference area.
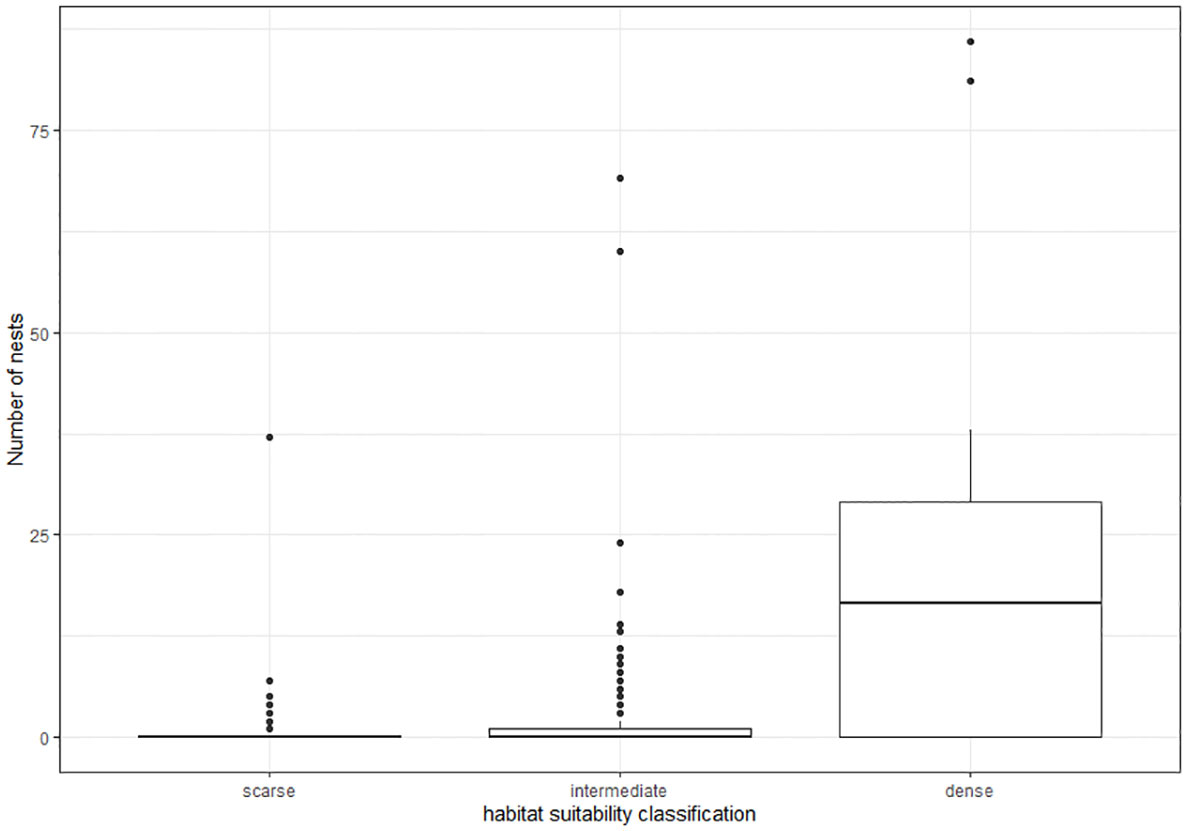
Figure 4 Boxplot showing the differences in total nest numbers (active and inactive) per grid cell between habitat suitability classes.
The habitat suitability classification was then assigned to the whole study area, resulting in the calculation of habitat areas for each class as shown in Table 3. The densities calculated within the reference area and the upper and lower limits of the 95% CI were then extrapolated for these areas (Table 3).
3.1.1 Abundance screening
During screening of the study area, we identified 56 areas of active snow petrel abundance in the air (23 of high abundance and 33 of low abundance, see Figure 5).
3.2 Nest site data
3.2.1 Boulder size and active nests
Out of 1,027 nests for which boulder size was noted, we found only ten associated with small boulders of less than 0.5 m diameter (<1%) (Figure 6). Under boulders of 0.5-1.0 m, we found 127 nests (12.4%). The majority of nests were found below boulders of more than one meter (900 nests; 87.6%), with 365 of them below boulders of more than two meters (35.5%). This general pattern was the same for all three subareas. However, in contrast to the other two areas, in area A (scree slopes) the highest proportion of nests were beneath boulders of 1.0-2.0 m. In the other areas, most nests were under boulders of more than two meters diameter.
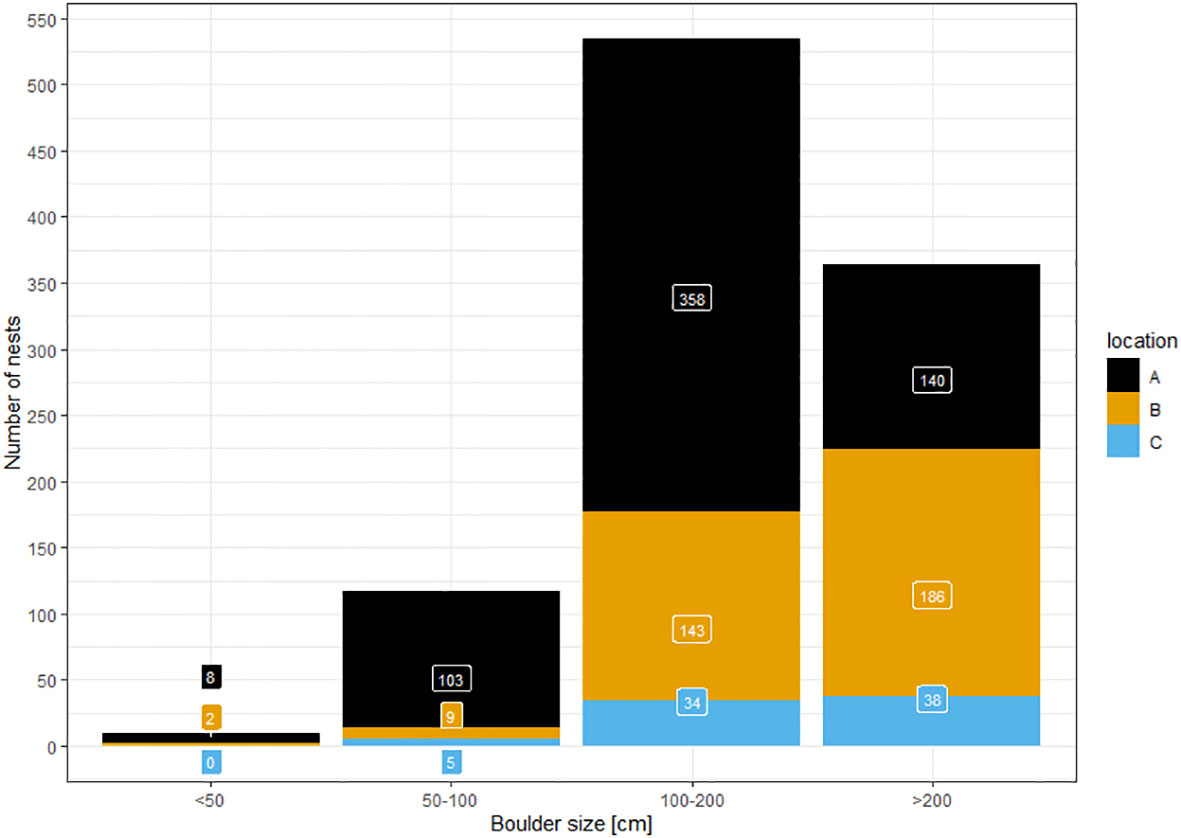
Figure 6 Number of nests found during ground surveys and the boulder size under which they were found in three reference areas (A…scree slopes; B…peninsula; C…Aurkjosen valley).
3.2.2 Visibility and nest cavity depth
During ground surveys, we recorded 129 concealed nests and 255 intermediate single nests. There were only three exposed (Figure 7). There were equal proportions of active nests in the categories concealed (42.6%) and intermediate (46.7%).
The depth of all the nest cavities was 0-2 m (mean=0.609 m, SD=0.352). Most nests were either about half a meter or about one meter deep. Concealed nests were slightly deeper (mean=0.699 m, SD=0.393) than intermediate nests (mean=0.575 m, SD=0.314) but both showed the full range of 0-2 m.
3.2.3 Nest orientation
The orientation of nests differed between the parts of the reference area. Each area had a different dominant orientation (Figure 8). On scree slopes this was eastern-southeastern, on the peninsula, southwestern-southeastern (but with the widest variation of the three), and in the Aurkjosen valley, northern-northeastern.
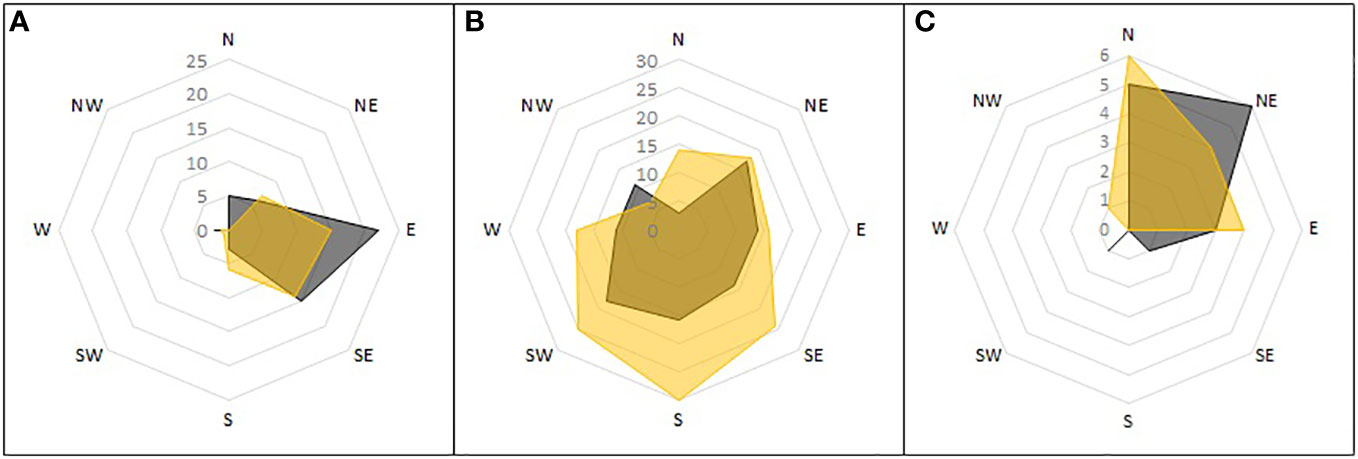
Figure 8 Orientation of single nests detected during ground surveys. Shown is the number of nests found in the subareas of the reference area and the direction towards which they opened. Active nests in grey, inactive nests in yellow. Subareas: (A) mountain slopes; (B) peninsula; (C) Aurkjosen valley.
3.2.4 Active nests and attendance
In all, 52.03% of nests detected during ground surveys were active. The proportion of active nests in aggregations (56.26%) was slightly higher than for single nests (44.99%). Active single nests were mostly occupied by one adult bird (161 of 175/92%), only in 14 cases were two adults observed at the nest. The proportion of active nests was highest in area A (scree slopes, 62.74%), lowest in area B (peninsula, 37.18%) and intermediate in area C (Aurkjosen valley, 41.77%).
3.3 Morph differentiation
The most important value differentiation between the larger and smaller morph is wing length (Bonaparte, 1856). None of the wings we measured were above the threshold of 280 mm. Live birds had wing lengths of 262-274 mm (mean=266.5 mm, SD=4.717, n=4). All measurements can be viewed in Supplementary Material IV.
4 Discussion
4.1 Population census
This study provided updated population estimates of snow petrels in the Otto-von-Gruber-Gebirge to inform the designation process of the region as Antarctic Specially Protected Area. Our extrapolated population estimates 22,493 individuals (range 10,451 – 34,534) based on nest sites and 11,765 (range 4,403 – 19,127) based on active nests or breeding pairs for the whole study area. The number of inactive nests is an estimate of maximum occupation, even though it is very unlikely that all available nests are ever used at the same time. These numbers underpin the size of the breeding site and thereby its importance for the species as one of the largest aggregations known (Croxall et al., 1995).
Ground surveys turned out to be extremely time consuming due to the concealed nesting biology of snow petrels. Nevertheless, through intensive search, we are confident to have found almost all nest sites within the reference area. This can also be backed up by literature results indicating a detection probability of about 85% for adult snow petrels (Southwell et al., 2011). Once incubation has started, one snow petrel adult is always present at the nest. Thus, time of day of detection did not influence attendance and thereby classification as active. Nevertheless, our survey started at the beginning of the incubation period (Marchant and Higgins, 1990), therefore some nests could have been not yet occupied. Those nests were therefore falsely classified as inactive. We cannot distinguish between inactive, but recently used and historical nests, since chemical and biological weathering is extremely slow and thus nests that were last occupied many years ago might still be well visible.
Since very little to nothing is known of the breeding phenology in this specific area, we also cannot comment on the maximum breeding pair number in this season. Our numbers only reflect a short time window during the breeding cycle. Nevertheless, according to data from other breeding sites, the beginning of December is the optimal time for monitoring. This period is after the pre-laying exodus, when many pairs leave the site for a short time. Before that time, breeding pair numbers might be over-estimated due to the presence of adults that do not, in fact, breed that season (Olivier et al., 2004). However, our counts were probably correctly timed for obtaining the most accurate estimate of population size because snow petrels do not replace clutches after loss and egg laying is supposedly highly synchronized in the period end of November to mid-December (Marchant and Higgins, 1990). It has been shown that weather conditions can have a severe impact on the population numbers and nest occupancy of Antarctic breeding birds, as for example extreme snow storms in the 2021/22 season (Descamps et al., 2023). This means that our results could be very specific to the season of investigation (2022/23), but long-term monitoring would be necessary to reveal such dynamics. We assume that due to the sheltered situation in Untersee valley and the observed differences between weather conditions in- and outside of the valley, this breeding aggregation could be relatively stable in comparison to others.
The breeding habitat of snow petrels have been analyzed before with the help of satellite remote sensing (Olivier and Wotherspoon, 2006) but this is the first study which used a UAV for this task. Though, there were many challenges to using UAVs in the study area. The weather conditions with high wind speeds allowed flying on few days only, which is a general problem in Antarctica (Dickens et al., 2021; Zmarz et al., 2023). The wind was also extremely gusty and had strong local maxima, especially below the mountain passes where cold air flows into the valley of Lake Untersee from the Antarctic Plateau. The low air temperatures reduced the performance of the UAV battery and required complex logistics to warm up the batteries in camp before flying. The area is characterized by peaks up to 2,700 m high and steep rock faces, which made flight planning extremely complicated and required accurate elevation models. Due to the southern latitude and the high mountains, GNSS reception is poor especially near the steep walls of the cirques.
Parameters other than the presence of boulders of more than one meter diameter are likely to influence nest availability, quality, and colonization. These factors include, for example, slope or topography, prolonged snow cover, wind situation, unfavorable thermal lifts that might impede take-off and many more. We focused the classification on one parameter (boulder size) for two main reasons: first, this parameter clearly directly influences breeding site choice, as indicated by own observations and literature (Simonov et al., 1985). Other parameters (like aspect, slope, micro topography) might also have an influence, but it is not clear if this is direct or indirect or at which scale. We assume that slope might not have a direct impact on nest site choice since snow petrels use areas of different slopes in different regions of the study area with seemingly no preference. Biologically, there is no need for steeper or shallower slopes because snow petrels have no problems taking off from flat ground or reaching escarpments. For predators in the area, it also makes no difference. On the other hand, slope influences the availability of large boulders which assemble particularly at the transition from steep to flat surfaces. But this effect is taken into account by using boulder size as parameter. For other parameters correlation is unclear at all. One such example is distance from the permanently frozen lake or the glacier. There seems to be no reason for snow petrels to avoid or stay close to these structures, but our reference area is too small to include different situations to test for this. For example, in the reference area there were almost no suitable boulders close to the lake, but in other areas of the valley there are. We are missing information completely if those habitats are used or not. Secondly, most data were not available either at all or in sufficient resolution. For example, wind direction is available on a regional scale, but even within the valley conditions differ vastly from the outsides. Even more diverse conditions exist within the complex high mountain topography, leading to spatially individual wind conditions.
Areas that are generally suitable might not be colonized just by coincidence. Our reference area is relatively small (because of the limited time available for ground surveys) and includes only a limited set of features. It is possible therefore that the numbers of birds measured in it are not typical of other areas, or that other parameters are more influential in other areas.
Our estimates align well with the sparse information in the literature. This information suggests about 10,000 breeding pairs (Hiller et al., 1988) but these numbers are not well validated. Nevertheless, our extrapolated results provide additional evidence for the large size of the population.
It is also possible to compare the distribution of habitat suitability classes within the study area with breeding sites recorded in the literature and with our own abundance screening. The dense areas (red grid cells) align relatively well with our high abundance screening areas (see Figure 9). Areas of high active snow petrel abundance all have red grid cells close by, or at least a large number of orange grid cells. It is important to note that this plausibility testing is uni-directional in a sense that we assume that areas of high abundance of birds in the air should be close to suitable habitat and host a higher density of nest sites. On the other hand, regions of low or no snow petrel abundances active in the air could still have suitable habitat. We cannot rule out that low abundances of flying snow petrels are caused by daily patterns, weather, or other factors not perceived. Both areas (high abundance, high habitat suitability) also correlate with the sites from literature (Hiller et al., 1988) or from personal communications (M. Andreev). Our classification method thus seems to represent well the known or assumed snow petrel breeding concentrations.
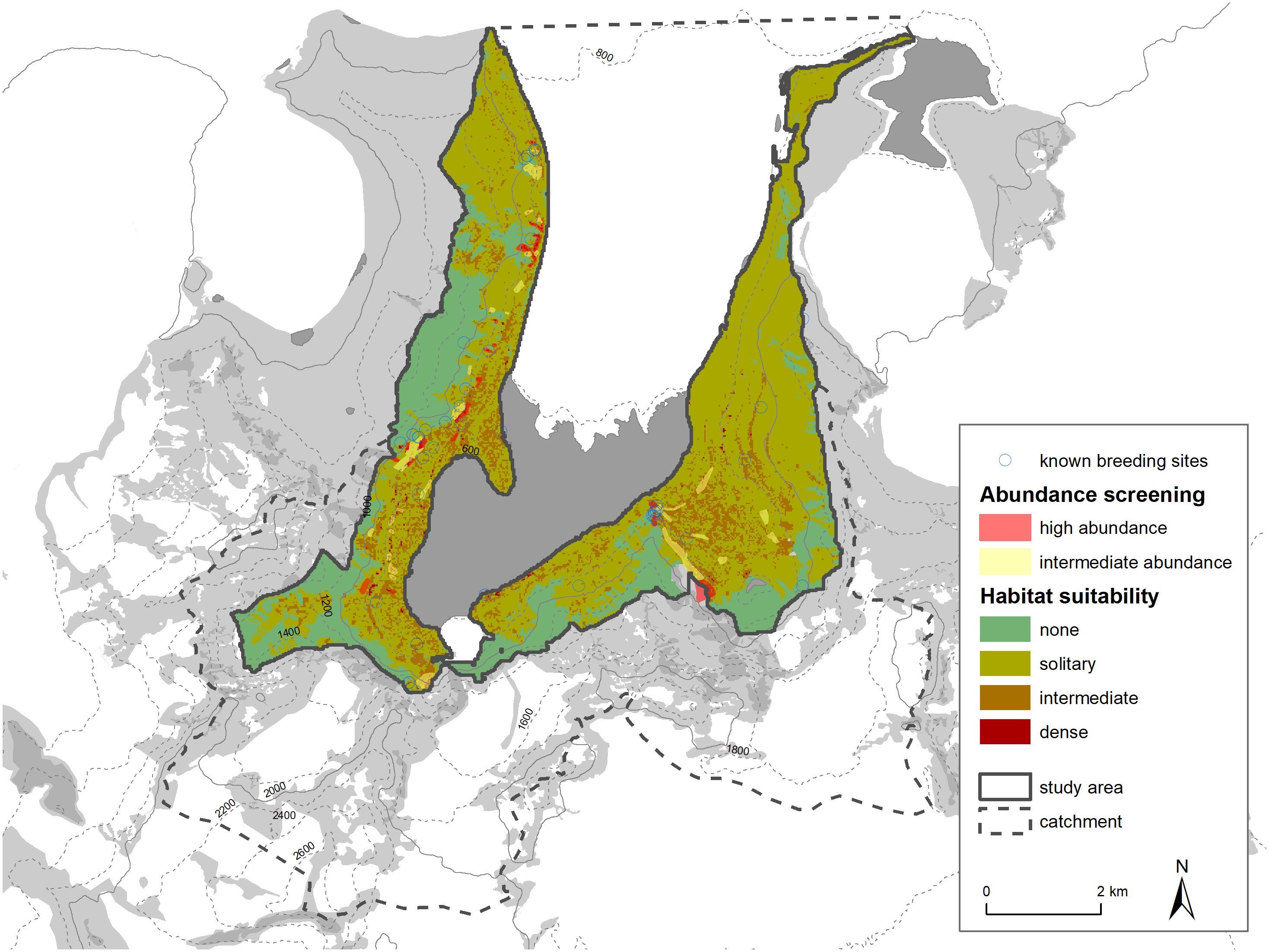
Figure 9 Comparison of habitat suitability classification with abundance screening and known snow petrel breeding places (Hiller et al., 1988 and pers. comm. M. Andreev).
4.2 Nest site data
We found the majority of nests below boulders of more than one meter in diameter. This accords well with existing information (Simonov et al., 1985) indicating that nests are preferentially constructed underneath stone blocks of more than one cubic meter in size or, very rarely, in cracks or niches of crags and cliffs. This result is used as a basis for our habitat suitability classification. We found that most nests on mountain slopes were underneath smaller boulders than in other areas. We assume that this is a bias simply due to boulders more than two meters in diameter being unlikely to remain on steep slopes.
We found that most nests were intermediately concealed, which means they were not directly visible and protected from weather in almost all directions, but not within a small tunnel or split. Only very few nests were out in the open. It is possible that, in contrast to other regions (Ryan and Watkins, 1989; Olivier and Wotherspoon, 2006), snow petrels in the study area don’t need very concealed nests since the valley is relatively protected from weather, particularly snow storms. Concealed nests were also slightly deeper, but it was possible to detect both types (concealed and intermediate) down to depths of two meters.
Nest orientation is associated with the dominant wind direction, mainly facing towards the wind to keep entries free from snow blockage (Olivier and Wotherspoon, 2006, 2008). In the Untersee valley, the prevailing wind direction was from the south-southeast. The nests, however, mainly faced east (scree slopes), south (peninsula) or northeast (Aurkjosen valley). Thus, an association with prevailing wind direction is only present on the peninsula. Other factors must therefore play a role. We propose that nest orientation is also greatly influenced by availability of suitable caves. For example, most nests on the scree slopes face east due to the local topography. The peninsula region has the fewest limitations by topography due to its flatness, which explains why the nest orientation varies most here. Because winds in the Aurkjosen valley are particularly strong, there is almost no snow accumulating there. In consequence, the risk of snow blockage of cavities is minimal and unassociated with wind direction. Under these conditions, snow petrels probably prefer nest orientations protected from direct wind (Olivier and Wotherspoon, 2008). It is also possible that orientation plays no role in the study area since it is relatively protected from weather events. There are also other breeding areas where orientation had no role on occupation (Ryan and Watkins, 1989).
The reason that we encountered two birds at a nest only in a few cases is very likely caused by the breeding shifts of snow petrel pairs, as change overs mostly occur at night (Marchant and Higgins, 1990).
The proportion of active nests was highest on the mountain slopes and lowest on the peninsula. This difference is possibly because of differences in nest quality consequent on several factors such as boulder availability, sedimentation, predation risk, wind exposition and so on. Another possibility is that it is a consequence of colonization history. Nests on the scree slopes are probably older and more established because these slopes became ice-free earlier than the peninsula. We found that about half of the nest sites were active (52.03%), which aligns with literature of 57% occupancy at Svarthamaren and 33% occupancy at Jutulsessen, two other breeding aggregations in Queen Maud Land (Descamps et al., 2023).
So far, there have been no systematic reports on nest site data in the Untersee valley. This knowledge can have important implications for protection measures, as for example areas of suitable habitat (e.g. high density of large boulders) should be protected from human influence. In addition, the comparison to breeding habits in other Antarctic regions provides information on general requirements of the species. Activity and adult bird presence data are crucial for designing future investigations, as for example on migration studies using tracking devices.
4.3 Morph differentiation
None of the wing measurements of live birds were above 280 mm. This fact strongly suggests that these snow petrels are all individuals of the small morph (P. n. minor). We also found that the wings of living birds are much longer than those of dead material (mummies and isolated wings). This difference could arise because tissues shrink after the bird dies, even though the conditions of Antarctica should preserve such materials. In some cases, the individual may not have been fully grown even though we only measured individuals with adult plumage. Nevertheless, because we measured relatively few wing lengths, we cannot rule out the large morph (P. n. major) also being present. Thus, the Lake Untersee breeding site is either mixed or consists solely of the small morph. Indeed, inland breeding sites, such Lake Untersee, tend to have populations that are either mixed or purely of the small morph (Jouventin and Bried, 2001).
4.4 Conclusion and further investigations
Using extrapolation methods, we quantified the snow petrel population in the catchment of Lake Untersee for the first time in at least 38 years, and the first of all with comparable and retraceable methods and information on potential spatial distribution. We estimated a population of 11,765 breeding pairs with 22,493 available nesting sites in an area of about 39 km². These figures indicate that this snow petrel breeding site contains one of the largest aggregations of the species known and is therefore of great importance for the global population. These findings underpin the importance of designating the Otto-von-Gruber-Gebirge as a protected area. Additionally, the information of the distribution of suitable habitat in the study area is currently used in the Draft Management Plan to design zones of special management and protection within the ASPA. The knowledge gained can also be used as a basis for designing future studies in the area, in particular regarding monitoring concepts for conservation.
We also show that it is possible to obtain large datasets using UAV-based remote sensing even in a remote area that poses major topographic, climatic and logistic challenges. This data is a crucial part of the designation and management of protected areas and thus for the conservation of species and ecosystems.
Further investigations need to focus on the refinement of the knowledge on the snow petrel population in the area. The information on general distribution of snow petrel nest sites in the whole area should be complemented by increased and spatially more differentiated sampling. The data gained by the UAV surveys can serve as a basis to design a representative sampling concept. Also, parameters influencing densities of nest sites apart from boulder size should be investigated, aiming at a better basis for extrapolations. It should also be verified if this extrapolation approach by habitat suitability can also be used for other snow petrel breeding sites with differing lithological and topographical conditions, or for other species. Furthermore, these results should be used to observe changes in the population to gain insights into regional population development and, therefore, protection needs. To do so, because ground monitoring of the whole area is likely to remain infeasible, it is necessary to define representative reference areas to use for future breeding pair comparisons.
Further investigation is also needed of snow petrel ecology and biology both at the study site as well as in general. This knowledge is needed in order to better identify threats and determine what protection is needed. More knowledge is particularly required on feeding trips and migration patterns, nest site behavior such as breeding shifts or territorial behavior, chick growth and survival rates and many more.
Data availability statement
The raw data supporting the conclusions of this article will be made available by the authors, without undue reservation.
Ethics statement
The animal study was approved by German Environment Agency (Umweltbundesamt). The study was conducted in accordance with the local legislation and institutional requirements.
Author contributions
M-CR: Conceptualization, Data curation, Formal analysis, Methodology, Resources, Validation, Visualization, Writing – original draft, Writing – review & editing. JE: Conceptualization, Data curation, Investigation, Methodology, Validation, Writing – review & editing. CP: Conceptualization, Data curation, Formal analysis, Investigation, Methodology, Resources, Validation, Visualization, Writing – original draft, Writing – review & editing. OM: Conceptualization, Data curation, Funding acquisition, Investigation, Methodology, Project administration, Resources, Supervision, Validation, Visualization, Writing – original draft, Writing – review & editing.
Funding
The author(s) declare financial support was received for the research, authorship, and/or publication of this article. This research was commissioned by the German Environment Agency (UBA), F&E-Vorhaben FKZ 3720182020 and funded by the Federal Ministry for the Environment, Nature Conservation and Nuclear Safety. We acknowledge support by the German Research Foundation Projekt-Nr. 512648189 and the Open Access Publication Fund of the Thueringer Universitaets- und Landesbibliothek Jena.
Acknowledgments
Fieldwork equipment was provided by the Alfred Wegener Institute. Logistics to and from the field site were conducted by White Desert Antarctica Ltd. We would like to thank Dale Anderson (SETI Institute, Mountain View, CA, USA; TAWANI Foundation) and his team for their great support before and during the expedition. We would also like to thank Mikhail Andreev (Komarov Botanical Institute, St. Petersburg, Russia) and Mirko Scheinert and Lutz Eberlein (Institute of Planetary Geodesy, TU Dresden, Germany) for their help in planning of the expedition. The text was edited during revision by Dr A. J. Davis (English Experience Language Services, Göttingen, Germany).
Conflict of interest
Authors M-CR, CP, and OM were employed by the company Thuringian Institute of Sustainability and Climate Protection GmbH.
The remaining author declares that the research was conducted in the absence of any commercial or financial relationships that could be construed as a potential conflict of interest.
Publisher’s note
All claims expressed in this article are solely those of the authors and do not necessarily represent those of their affiliated organizations, or those of the publisher, the editors and the reviewers. Any product that may be evaluated in this article, or claim that may be made by its manufacturer, is not guaranteed or endorsed by the publisher.
Supplementary material
The Supplementary Material for this article can be found online at: https://www.frontiersin.org/articles/10.3389/fcosc.2024.1298962/full#supplementary-material (Supplementary Material I, III, and IV) and https://figshare.com/s/898c0c1d673014613a8d (Supplementary Material II).
References
Andersen D. T., Mckay C. P., Lagun V. (2015). Climate conditions at perennially ice-covered lake untersee, east Antarctica. J. Appl. Meteorology Climatology 54, 20. doi: 10.1175/JAMC-D-14-0251.1
Andersen D. T., Sumner D. Y., Hawes I., Webster-Brown J., Mckay C. P. (2011). Discovery of large conical stromatolites in lake untersee, Antarctica: large conical stromatolites. Geobiology 9, 280–293. doi: 10.1111/j.1472-4669.2011.00279.x
ATCM. (2019). “Final report of the forty-second antarctic treaty consultative meeting.” Prague, Czech Republic (Prague: Secretariat of the Antarctic Treaty).
ATCM. (2022). Final report of the forty-fourth antarctic treaty consultative meeting, berlin, Germany, 23 may - 2 june 2022 (Buenos Aires: Secretariat of the Antarctic Treaty).
ATS. (1991a). Annex V to the protocol on environmental protection to the antarctic treaty (Madrid: ATS). Available at: https://documents.ats.aq/keydocs/vol_1/vol1_9_AT_Protocol_Annex_V_e.pdf.
ATS. (1991b). Protocol on environmental protection to the antarctic treaty. In: . Available online at: https://www.ats.aq/devph/en/apa-database/searchapa-results.
BirdLife International. (2018). Pagodroma nivea.” The IUCN red list of threatened species 2018:E.T22697885A132611127. Available online at: https://dx.doi.org/10.2305/IUCN.UK.2018-2.RLTS.T22697885A132611127.en. doi: 10.2305/IUCN.UK.2018-2.RLTS.T22697885A132611127.en
Bonaparte C. L. (1856). Espéces Nouvelles d’oiseaux d’Asie et d’Amérique et Tableaux Para Lléliques Des Pélagiens Ou Gavae Vol. 42 (Paris: Comptes Rendus Heb− Domadaires Des Séances de l’Académie Des Sciences), 764–776.
Braun C. E. (2005). Techniques for wildlife investigations and management. 6th ed (Bethesda, Md: Wildlife society).
Cavender-Bares J., Schneider F. D., Santos M. João, Armstrong A., Carnaval A., Dahlin K. M., et al. (2022). Integrating remote sensing with ecology and evolution to advance biodiversity conservation. Nat. Ecol. Evol. 6, 506–519. doi: 10.1038/s41559-022-01702-5
Coetzee B. W. T., Convey P., Chown S. L. (2017). Expanding the protected area network in Antarctica is urgent and readily achievable. Conserv. Lett. 10, 670–805. doi: 10.1111/conl.12342
Croxall J. P., Steele W. K., McInnes S. J., Prince P. A. (1995). Breeding distribution of the snow petrel pagodroma nivea. Mar. Ornithology 32, 69–100.
Descamps Sébastien, Hudson S., Sulich J., Wakefield E., Grémillet D., Carravieri A., et al. (2023). Extreme snowstorms lead to large-scale seabird breeding failures in Antarctica. Curr. Biol. 33, R176–R177. doi: 10.1016/j.cub.2022.12.055
Dickens J., Hollyman P. R., Hart T., Clucas G. V., Murphy E. J., Poncet S., et al. (2021). Developing UAV monitoring of south Georgia and the south sandwich islands’ Iconic land-based marine predators. Front. Mar. Sci. 8. doi: 10.3389/fmars.2021.654215
Faucher B., Lacelle D., Fisher D. A., Andersen D. T., McKay C. P. (2019). Energy and water mass balance of lake untersee and its perennial ice cover, east Antarctica. Antarctic Sci. 31, 271–855. doi: 10.1017/S0954102019000270
Fretwell P. T., Larue M. A., Morin P., Kooyman G. L., Wienecke B., Ratcliffe N., et al. (2012). An emperor penguin population estimate: the first global, synoptic survey of a species from space. PloS One 7, e337515. doi: 10.1371/annotation/32c246eb-3b73-4410-a44c-b41ddae11fc5
Friedmann E.I., Ocampo R. (1976). Endolithic blue-green algae in the dry valleys: primary producers in the antarctic desert ecosystem. Science 193, 1247–1249. doi: 10.1126/science.193.4259.1247
Goldsworthy P. M., Thomson P. G. (2000). An extreme inland breeding locality of snow petrels ( Pagodroma nivea ) in the southern prince charles mountains, Antarctica. Polar Biol. 23, 717–720. doi: 10.1007/s003000000146
Harris C., Lorenz K., Fishpool L. D. C., Lascelles B., Cooper J., Coria N. R., et al. (2015). Important bird areas in Antarctica 2015 (Cambridge: BirdLife International and Environmental Research & Assessment Ltd). Available at: http://www.era.gs/resources/iba/Important_Bird_Areas_in_Antarctica_2015_v5.pdf.
Hiller A., Hermichen W.-D., Wand U. (1995). Radiocarbon-dated subfossil stomach oil deposits from petrel nesting sites: novel paleoenvironmental records from continental Antarctica. Radiocarbon 37, 171–805. doi: 10.1017/S0033822200030617
Hiller A., Wand U., Kämpf H., Stackebrandt W. (1988). Occupation of the antarctic continent by petrels during the past 35 000 years: inferences from a 14C study of stomach oil deposits. Polar Biol. 9, 69–775. doi: 10.1007/BF00442032
Howat I. M., Porter C., Smith B. E., Noh M.-J., Morin P. (2019). The reference elevation model of Antarctica. Cryosphere 13, 665–745. doi: 10.5194/tc-13-665-2019
Hughes K. A., Grant S. M. (2017). The spatial distribution of Antarctica’s protected areas: A product of pragmatism, geopolitics or conservation need? Environ. Sci. Policy 72, 41–51. doi: 10.1016/j.envsci.2017.02.009
Hughes K. A., Ireland L. C., Convey P., Fleming A. H. (2016). Assessing the effectiveness of specially protected areas for conservation of Antarctica’s botanical diversity: effectiveness of plant conservation in Antarctica. Conserv. Biol. 30, 113–205. doi: 10.1111/cobi.12592
Jouventin P., Bried Joël (2001). The effect of mate choice on speciation in snow petrels. Anim. Behav. 62, 123–325. doi: 10.1006/anbe.2001.1713
Kleinschmidt G. (2021). “The geology of the antarctic continent,” in Beiträge zur regionalen geologie der erde, vol. 33. (Borntraeger Science Publishers, Stuttgart).
Konovalov G. V. (1962). Nablyudeniya za pticami na zemle korolevy mod (Observations of birds in queen maud land). Inf Byull Sov Antarkt Eksped 35, 45–48.
Konovalov G. V. (1964). Observations of birds in queen maud land. Inf. Bull. Soviet Antarctic Expedition 4196, 156–158.
Kosenko N. G., Kolobov D. D. (1970). Obsledovanje osera untersee [Survey of lake untersee. Byulleten Sovetskoi Antarkticheskoi Ekspeditsii Byulleten Sovetskoi Antarkticheskoi Ekspeditsii 79, 65–69.
Loopmann A., Kaup E., Haendel D., Simonov I. M., Klokov V. D. (1986). Zur bathymetrie einiger seen der schirmacher-und untersee-oase (Ostantarktika). Tallinn: Eesti NSV Teaduste Akadeemia.
Lovenskiold H. L. (1960). The snow petrel pagodroma nivea nesting in dronning maud land. Ibis 102, 132–134. doi: 10.1111/j.1474-919X.1960.tb05104.x
Marchant S., Higgins P. G. (1990). Handbook of Australian, New Zealand & Antarctic birds Vol. 1 (Melbourne, Victoria: Oxford University Press), 402–410. Available at: https://nzbirdsonline.org.nz/sites/all/files/068_Snow%20Petrel.pdf. Ratites to ducks.
McClymont E. L., Bentley M. J., Hodgson D. A., Spencer-Jones C. L., Wardley T., West M. D., et al. (2021). Summer sea-ice variability on the antarctic margin during the last glacial period reconstructed from snow petrel (Pagodroma nivea) stomach-oil deposits. Ocean Dynamics/Marine Archives/Pleistocene 18, 381–403. doi: 10.5194/cp-2021-134
Naveen R., Lynch H. J., Forrest S., Mueller T., Polito M. (2012). First direct, site-wide penguin survey at deception island, Antarctica, suggests significant declines in breeding chinstrap penguins. Polar Biol. 35, 1879–1885. doi: 10.1007/s00300-012-1230-3
Olivier F., Lee A., Woehler E. (2004). Distribution and abundance of snow petrels pagodroma nivea in the windmill islands, east Antarctica. Polar Biol. 27, 257–265. doi: 10.1007/s00300-004-0595-3
Olivier Frédérique, Wotherspoon S. J. (2006). Modelling habitat selection using presence-only data: case study of a colonial hollow nesting bird, the snow petrel. Ecol. Model. 195, 187–2045. doi: 10.1016/j.ecolmodel.2005.10.036
Olivier Frédérique, Wotherspoon S. J. (2008). Nest selection by snow petrels pagodroma nivea in east Antarctica: validating predictive habitat selection models at the continental scale. Ecol. Model. 210, 414–430. doi: 10.1016/j.ecolmodel.2007.08.006
Over J.-S. R., Ritchie A. C., Kranenburg C. J., Brown J. A., Buscombe D. D., Noble T., et al. (2021). “Processing coastal imagery with agisoft metashape professional edition, version 1.6—Structure from motion workflow documentation.” USGS numbered series 2021–1039,” in Processing coastal imagery with agisoft metashape professional edition, version 1.6—Structure from motion workflow documentation, vol. 2021–1039. (U.S. Geological Survey, Reston, VA). doi: 10.3133/ofr20211039
Pertierra L. R., Hughes K. A., Vega G. C., Olalla-Tárraga M. Á (2017). High resolution spatial mapping of human footprint across Antarctica and its implications for the strategic conservation of avifauna. PloS One 12, e01682805. doi: 10.1371/journal.pone.0168280
Phillips L. M., Leihy R. I., Chown S. L. (2022). Improving species-based area protection in Antarctica. Conserv. Biol. 36(4). doi: 10.1111/cobi.13885
Prevost J. (1969). A Propos Des Petrels Des Neiges de la Terre Adelie. Oiseau La Rev. Francaise d’Ornithologie 39, 33–49.
R Core Team. (2018). R: A language and environment for statistical computing (Vienna, Austria: R Foundation for Statistical Computing). Available at: https://www.R-project.org/.
RStudio Team. (2016). RStudio: integrated development for R (Boston, MA: RStudio, Inc). Available at: http://www.rstudio.com/.
Ryan P. G., Watkins B. P. (1989). Snow petrel breeding biology at an inland site in continental Antarctica. Evergreen, Colorado: Waterbird Society. 10. doi: 10.2307/1521338
Schwab M. (1998). Rekonstruktion der spätquartären Klima- und Umweltgeschichte der Schirmacher Oase und des Wohlthat Massivs (Ostantarktika) (Reconstruction of the Late Quaternary climatic and environmental history of the Schirmacher Oasis and the Wohlthat Massif (East Antarctica). Alfred-Wegener-Institut für Polar- und Meeresforschung. Application/pdf. Berichte zur Polarforschung = Reports on Polar Research. doi: 10.2312/BZP_0293_1998
Schwaller M. R., Lynch H. J., Tarroux A., Prehn B. (2018). A continent-wide search for antarctic petrel breeding sites with satellite remote sensing. Remote Sens. Environ. 210, 444–451. doi: 10.1016/j.rse.2018.02.071
Shaw J. D., Terauds A., Riddle M. J., Possingham H. P., Chown S. L. (2014). Antarctica’s protected areas are inadequate, unrepresentative, and at risk. PloS Biol. 12, e10018885. doi: 10.1371/journal.pbio.1001888
Simonov I. M., Stackebrandt W., Haendel D., Kaup E., Kampf H., Loopmann A. (1985). Komplexe Naturwissenschaftliche Untersuchungen Am Unter-Und Obersee, Zentrales Dronning-Maud-Land, Antarktika.(Etudes Scientifiques Intégrées Des Zones de Lacs de l’Untersee et Obersee, Dronning Maud Land Central, Antarctique). Petermanns Geographische Mitt. Gotha 129, 125–135.
Skidmore A. K., Coops N. C., Neinavaz E., Ali A., Schaepman M. E., Paganini M., et al. (2021). Priority list of biodiversity metrics to observe from space. Nat. Ecol. Evol. 5, 896–906. doi: 10.1038/s41559-021-01451-x
Southwell D. M., Einoder L. D., Emmerson L. M., Southwell C. J. (2011). Using the double-observer method to estimate detection probability of two cavity-nesting seabirds in Antarctica: the snow petrel (Pagodroma nivea) and the wilson’s storm petrel (Oceanites oceanicus). Polar Biol. 34, 1467–1474. doi: 10.1007/s00300-011-1004-3
Terauds A. (2016). An update to the antarctic specially protected areas (ASPAs) march 2016 (Kingston Tasmania: Australian Antarctic Data Centre). doi: 10.4225/15/572995579CD36
Terauds A., Chown S., Morgan F., Peat H., Watts D., Keys H., et al. (2012). Conservation biogeography of the Antarctic. Diversity Distributions 18, 726–741. doi: 10.2307/23258118
Terauds A., Lee J. R. (2016). Antarctic biogeography revisited: updating the antarctic conservation biogeographic regions. Diversity Distributions 22, 836–405. doi: 10.1111/ddi.12453
Wand U., Schwab M. J., Samarkin V. A., Schachtschneider D. (1996). Sedimentgeologische Arbeiten während der Expedition Schirmacheroase 1994/95 des AWI, Forschungsstelle Potsdam.
Wauchope H. S., Shaw J. D., Terauds A. (2019). A snapshot of biodiversity protection in Antarctica. Nat. Commun. 10, 9465. doi: 10.1038/s41467-019-08915-6
Wickham H. (2016). Ggplot2: elegant graphics for data analysis. 2nd ed (Cham, Switzerland: Springer International Publishing). doi: 10.1007/978-3-319-24277-4
Žabota B., Kobal M. (2021). Accuracy assessment of uav-photogrammetric-derived products using PPK and GCPs in challenging terrains: in search of optimized rockfall mapping. Remote Sens. 13, 3812. doi: 10.3390/rs13193812
Keywords: snow petrel, Pagodroma nivea, population survey, UAV, monitoring, ASPA, Dronning Maud Land, Otto-von-Gruber-Gebirge
Citation: Rümmler M-C, Esefeld J, Pfeifer C and Mustafa O (2024) Survey of a snow petrel nesting site in a remote high mountain region to inform designation of an Antarctic Specially Protected Area. Front. Conserv. Sci. 5:1298962. doi: 10.3389/fcosc.2024.1298962
Received: 22 September 2023; Accepted: 04 March 2024;
Published: 02 April 2024.
Edited by:
William J. McShea, Smithsonian Conservation Biology Institute (SI), United StatesReviewed by:
Lacey Hughey, Smithsonian Conservation Biology Institute (SI), United StatesAutumn-Lynn Harrison, Smithsonian's National Zoo & Conservation Biology Institute, United States
Copyright © 2024 Rümmler, Esefeld, Pfeifer and Mustafa. This is an open-access article distributed under the terms of the Creative Commons Attribution License (CC BY). The use, distribution or reproduction in other forums is permitted, provided the original author(s) and the copyright owner(s) are credited and that the original publication in this journal is cited, in accordance with accepted academic practice. No use, distribution or reproduction is permitted which does not comply with these terms.
*Correspondence: Marie-Charlott Rümmler, marie-charlott.ruemmler@think-jena.de