- 1South African Environmental Observation Network (SAEON), National Research Foundation, Pretoria, South Africa
- 2School of Animal, Plant & Environmental Sciences, University of the Witwatersrand, Johannesburg, South Africa
- 3Institute for Developmental Learning & Environmental Sustainability (IDLES), Hoedspruit, South Africa
- 4Independent Researcher, Hoedspruit, South Africa
Grasslands are one of the most threatened terrestrial ecosystem types, and montane grasslands of particular conservation concern. Despite high rates of transformation in recent decades, croplands and plantations are being abandoned in parts of many countries, creating an opportunity for conservation of montane grasslands through restoration. We report on the changes in the cover of major vegetation types (indigenous forest, grassland, and plantations) between 1935 and 2022, in an area that was intensively afforested from 1930 to 1960 and abandoned in 2000. Montane grassland at the site declined from over 50% of all landcover to below 15%, but subsequently recovered to 30% within 20 years. Many former plantations developed into secondary grassland with estimated gamma plant species richness of 231 for herbaceous species and 45 for savanna species. These are high values considering the size of the study area (4000 ha), and comparable to estimates from primary grassland sites in the broader region. However, at the scale of 1 m2 sampling quadrats, richness in the secondary grasslands was below that recorded in the last remaining patches of primary grassland at the site (means of 2.6 versus 4.7 for graminoid species, and 1.9 versus 2.9 for forbs). Some of the former Eucalyptus plantations had transformed into novel savannas dominated by fire-tolerant, resprouting trees, and may require more active restoration. Secondary grasslands such as those reported on here could potentially make a significant contribution to the conservation of montane biodiversity over the coming decades, warranting further research (both socio-economic and ecological) on the factors that lead to abandonment and promote the emerge of secondary grasslands of high diversity.
1 Introduction
Grasslands and savannas are the most threatened biomes globally (Hoekstra et al., 2005). Within these biomes, undisturbed montane grasslands are one of the most diverse and threatened ecosystem types (Bond and Parr, 2010; Veldman, 2016). While most montane grasslands did not experience the massive degree of transformation to agriculture that occurred in most of the lower-lying grasslands in the early 20th century, many have been transformed more recently. These losses have serious implications for conservation, as montane grasslands typically have high biodiversity, including many species with small ranges and endemic distributions which are vulnerable to transformation (Srinivasan et al., 2015; Clark et al., 2022). These ecosystems are also important for a range of ecosystem services, such as the provision of medicinal plants (Davenport and Ndangalasi, 2003), production of forage for livestock (Boval and Dixon, 2012), carbon sequestration (Bai and Cotrufo, 2022) and regulation of freshwater supplies (Carbutt and Thompson, 2021).
Most montane grassland ecosystem types have low levels of formal protection. For example, of the 39 highland and montane grassland vegetation types in South Africa, 27 have less than 10% of their area within a formal protected area (Skowno et al., 2019). Outside of these protected areas, transformation to cropland and afforestation has been widespread and much of what remains is highly fragmented (Mucina et al., 2006; Niemandt and Greve, 2016). Similar losses over the past few decades have been reported for montane grasslands around the world, including the shola grasslands of India (Arasumani et al., 2018), highland grasslands in East Africa (Ndang’ang’a et al., 2002) and montane grasslands in Brazil (Hermann et al., 2016) and Ecuador (Farley Wolf, 2007). Agricultural conversion, opencast mining, anthropogenic increases in or suppression of fires, mismanaged livestock, wood extraction, invasive species and global climate change present additional risks to these grasslands in the foreseeable future (Veldman et al., 2015).
While the primary focus for the conservation of montane grasslands should remain the protection of remaining, intact, primary ecosystems, this may not be sufficient. Many of these undisturbed systems are small and fragmented, and may not be capable of sustaining viable populations in the long-term, particularly as global climate change intensifies. Even within well managed protected areas, climate change, altered fire regimes and invasive alien plants may degrade or further fragment these ecosystems. The restoration of grasslands between the remaining fragments may prove to be a valuable and even necessary conservation effort for the long-term persistence of our remaining montane biodiversity (Buisson et al., 2021). Despite the recent, widespread conversion of grassland to agriculture, and predictions of increases in afforestation over the coming decades (e.g. McEwan et al., 2020), there are some montane areas where the reverse is currently occurring. This may provide an opportunity for expanding the area of montane grassland under protection, and reconnecting fragments of primary grasslands. In some countries the area of montane grasslands has actually increased in recent decades following abandonment of croplands (Zarzycki et al., 2022). While the total area of plantations continues to increase globally, the rate of increase has declined, and reductions have occurred in some countries over the past decade (FAO, 2020). For European countries combined, the total plantation area decreased from 2000-2010 to 2010-2020. In Australia, the area of plantations has steadily declined since 2012, not all of which has been transformed to agriculture (ABARES, 2023). In South Africa, substantial areas of state forestry were decommissioned in the early 2000s, following concerns about the impact of plantations on freshwater supplies (Jackson et al., 2005; Scott and Prinsloo, 2008; Van Wilgen, 2015; Cano et al., 2023).
Secondary grasslands in montane environments have rarely been studied (Bond and Parr, 2010). A few studies show a reduced diversity of herbaceous plants, particularly forbs, even decades after plantations are removed (Zaloumis and Bond, 2011; Zaloumis and Bond, 2016), consistent with secondary grasslands in general (Nerlekar and Veldman, 2020). Less is known about the diversity of other taxa, although Eckert et al. (2019) found a rapid return of species richness of a range of invertebrate taxa in montane grassland following the removal of a plantation. While secondary montane grasslands may not develop the same degree of plant diversity as original primary grasslands over decadal time scales - at least not without massive and costly restoration efforts (Buisson et al., 2022) - they could still make substantial contributions to conservation. Firstly, by creating corridors between fragments of primary grassland. Secondly, by providing habitats for mobile species which are not dependent on high plant diversity and have home ranges larger than fragments of remaining primary grassland within a region. Secondary grasslands may also provide similar, if not equal, levels of ecosystem services provided by former primary grasslands (such as the recovery of river flows following removal of plantations). However, abandoned areas can be rapidly colonized by invasive alien plant species which may negate these potential benefits, and may create source populations that invade further into areas of primary grasslands (Nuñez et al., 2017). Abandonment of croplands and plantations can thus create a threat rather than an opportunity for conservation. Determining rates of cropland and forestry abandonment, factors which increase biodiversity within secondary grasslands, and factors that limit colonization by alien species, are therefore key research questions for the future conservation of montane grasslands.
In this study, the vegetation of a large area of decommissioned plantations was studied in a sub-tropical montane region of South Africa. The aims were to determine the current extent of grasslands that have developed in these areas, identify factors which promote their restoration, and estimate their plant diversity.
2 Methods
2.1 Site
The study area was the upper catchment of the Klaserie River, an area of approximately 4000 ha on the eastern slopes of the Mariepskop and Hebron mountains of the North Eastern Great Escarpment, South Africa (Figure 1). Vegetation of the area in pre-industrial times is considered to have been Legogote Sour Bushveld on the lower slopes (Mucina et al., 2006), a savanna type classified as an Endangered Ecosystem in South Africa (Skowno and Monyeki, 2021). Aerial photography from 1938 indicates that the upper part of the site was a mosaic of indigenous forest, grasslands and wetlands. The forest falls within the Northern Mistbelt Forest type of Mucina et al. (2006), and was not directly affected by afforestation. Detailed phytosociological studies of these forests indicate that 5 forest subtypes are likely to occur within the study area: Mariepskop Mistbelt Forest, Foothills Mistbelt Forest, Blyde Scarp Forest, Mapulaneng Scarp Forest and Escarpment Riverine Forest (Lötter et al., 2014). Many of the grasslands are likely to have fitted within the Northern Escarpment Quartzite Sourveld of Mucina et al. (2006), although a broader diversity of communities was probably present given the fine-scale variation in soils, aspect, slope and precipitation in the upper part of the site.
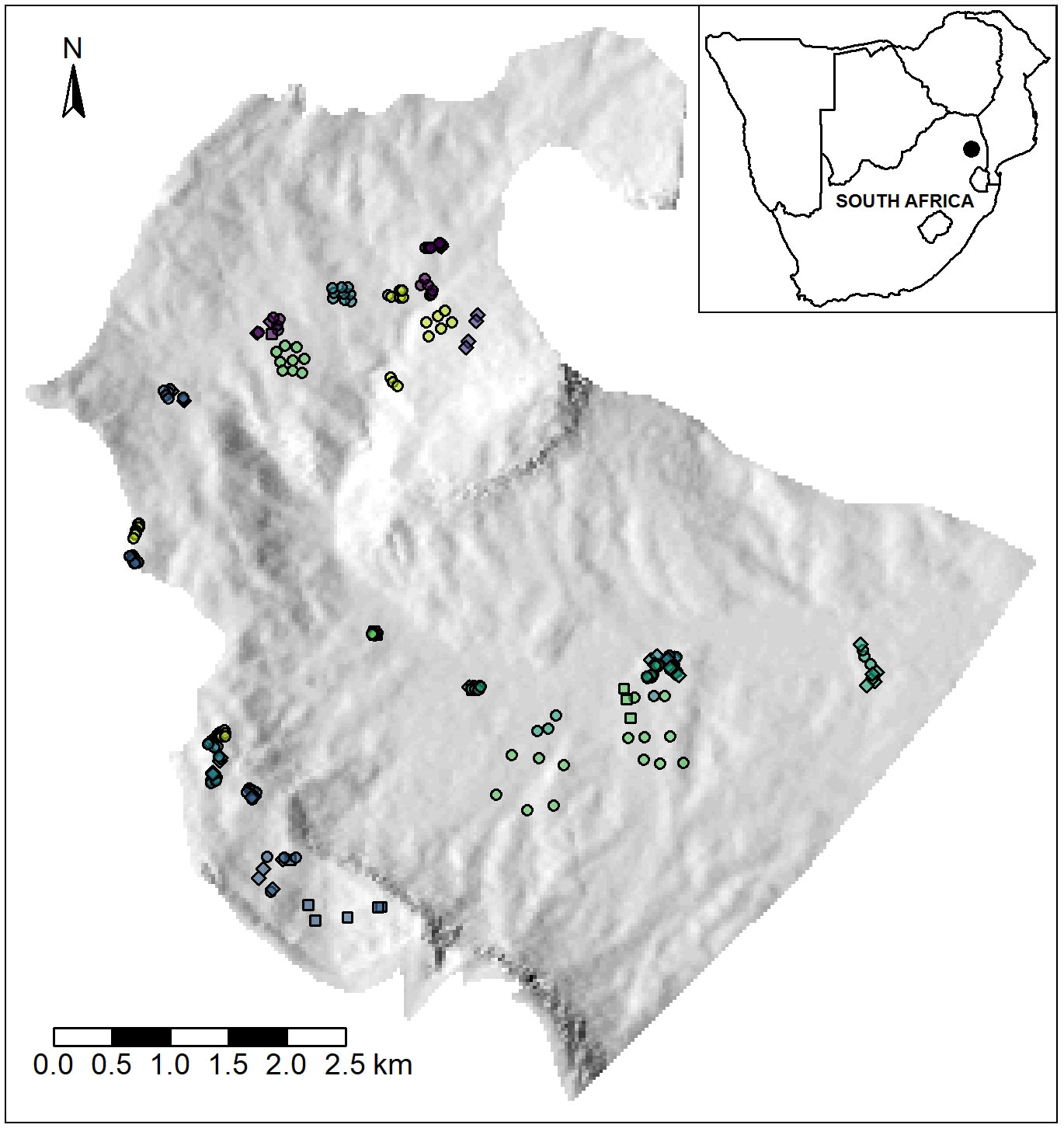
Figure 1 Map of the study area, showing the location of the transects and quadrats sampled in the upper catchment of the Klaserie River. Circles are quadrats, squares are starting points of transects, and diamonds show both a transect start/end and a quadrat. Points are coloured according to each of the 23 sites.
The geology of the lower part of the study area is granite, with mostly quartzite in the middle and upper parts and quarzitic cliffs around the top (Matthews et al., 1993). Soils vary from shallow sandy loams on ridges to deeper soils with higher organic content in depressions and valleys. Wetlands are common, including peat wetlands.
Strong rainfall gradients exist across the study area, ranging from approximately 700 mm to 2000 mm per annum. Daily rainfall data recorded at two forestry stations within the study area indicate a mean annual rainfall of approximately 1250mm for much of the middle altitudes of the study area: 1218 mm (85 years between 1935 and 2021) at the Salique forestry station at 890 m.a.s.l and 1266 mm (90 years between 1930 and 2022) at the Mariepskop forestry station at an altitude of 1314 m. a. s. l. As no long-term rainfall data were available for other parts of the site, automated rain gauges were installed in 2019. One higher in the catchment at 1485 m. a. s. l., where rainfall is assumed to be greatest, indicates an annual rainfall of approximately 2000 mm. Another on the north-facing slopes at an altitude of 1340 m.a.s.l indicates that the southern-western part of the catchment is considerably drier, with annual rainfall of approximately 750 mm. The lowest end of the study area, where the altitude falls below 700 m. a. s. l., is likely to have an annual total of about 700mm, based on data from other gauges in the region with similar altitudes. Rainfall is strongly seasonal, with the majority falling in the summer, between October and April. Mist is common at the higher altitudes and the duration of rainy season is longer.
The study area was afforested between the early 1930s and 1970s, as part of a national government forestry programme. This extended across the study area and further south, ultimately transforming 1100 ha of grassland and savanna to a variety of alien Eucalyptus and Pinus plantations. Forestry operations were decommissioned in 2001 and the majority of the study area eventually gazetted as a state nature reserve in 2021. From 2000 to 2012 some plantation stands were felled and stumps treated with herbicide, and others felled but not treated. Most were simply abandoned due to the economic unviability of harvesting them. Unregulated, informal logging has also occurred, particularly over recent years, leading to the partial felling of many of the abandoned stands. Concurrent with the above felling, a state agency (Working-for-Water) and later a non-governmental organization (Kruger-to-Canyons Biosphere Reserve NPO) implemented removal of all alien plants, including plantation trees in some of the old plantation stands. This involved ring-barking of adult Pinus trees, ring-barking and the application of herbicide for adult Eucalyptus trees and cutting and herbicide application to cut stumps for juveniles of these species as well as any other alien plants. No restoration planting activities or any other active management have been implemented to date.
2.2 Land cover change
Long-term changes in the cover of the major vegetation types of the study area were quantified using aerial photography from 1938, 1954, 1965, 1970, 1971, 1995 and 2012, and Google Earth imagery for 2007, 2012 and 2017 – 2022. One hundred random points were virtually placed within the study site using GIS, and overlaid on the 1938, 1954, 1970/71, 2017 and 2022 imagery. For each year, each point was visually classified as either indigenous forest, plantation, grassland (primary or secondary grassland with no or sparse shrubs or trees) or transitional (areas with partial tree cover, usually of juvenile trees – it was often not possible to distinguish if these areas where young plantations or previously felled plantations that were subsequently colonized by alien trees and/or indigenous pioneer tree species). The proportion of each were summed to create a relative percentage cover of each type per year.
2.3 Experimental design
The diversity of altitude, precipitation, soil type and aspect across the study area, together with the ad hoc felling of plantation trees and unplanned fires, created the opportunity to conduct a natural experiment to investigate the development of secondary grassland in abandoned plantations. Based on field observations, Google Earth imagery and local knowledge, the following classification was used to categorize the major ecosystem types present in each abiotic group in 2019 (Figure 2):
1. Primary grasslands, which were limited to two small patches of 1 and 2.5 ha. These were the only sites that could be found that were never afforested or invaded by tree species, although may have been significantly affected by past grazing by domestic livestock, fire exclusion and fragmentation.
2. Secondary grassland that had developed from plantation stands and were largely free of plantation species by 2019. The term grassland is used broadly here, to include open savannas with a low cover of shrubs and trees (sensu Buisson et al., 2021). In sites that were previously Eucalypts stands, it is likely that cut stumps in these sites were treated with herbicide, but no records were found to confirm where and when such treatments were applied. In the old Pinus stands, it is unlikely that herbicides were applied as Pinus trees usually die after being cut. Aerial photography indicates that the clearing of all these sites took place between 2003 and 2007.
3. Plantations that were not cut before abandonment and remained dominated by plantation species. These included stands of adult Eucalyptus or Pinus, and stands of mixed species and mixed size classes, which had been cut at some stage but had resprouted and/or were colonized by trees through seed recruitment. The mixed stands were typically a mix of Eucalyptus, Pinus and indigenous forest species, and had a range of tree size classes. This type was sub-divided according to the dominant plantation species (Eucalyptus or Pinus).
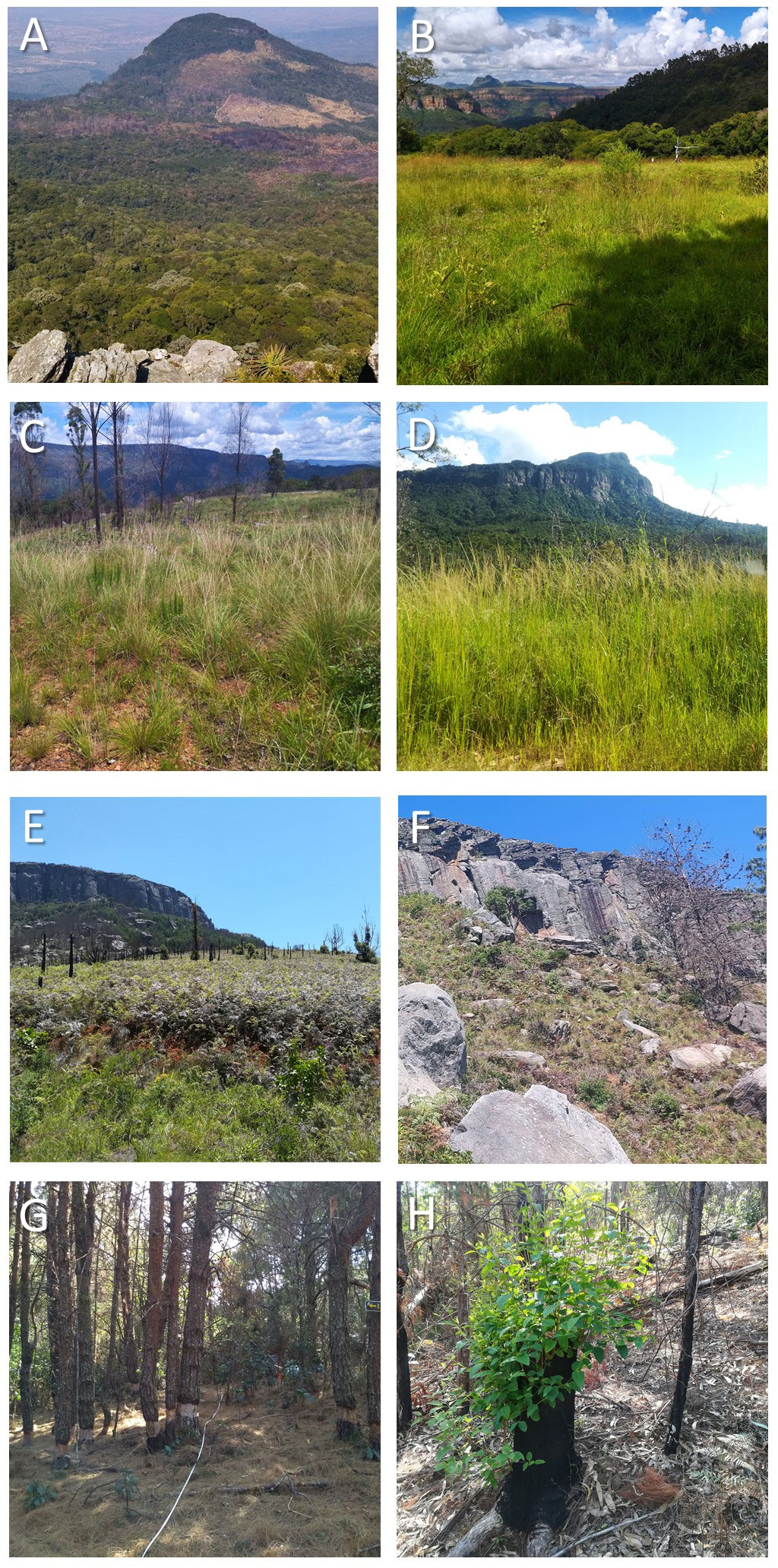
Figure 2 Examples of vegetation in the four categories of ecosystem type used for analysis. (A) a view of sites on the higher altitude, north-facing slopes of the study area, with indigenous forest in the foreground, and patches of burnt and unburnt plantations and secondary grassland in the background; (B) one of the patches of primary grassland; (C) secondary grassland on shallow soils on a relatively wet, south-facing slope; (D) secondary grassland in one of the valley sites, with a mix of indigenous forest and old plantations on the slopes behind; (E) secondary grassland on a drier, north-facing slope with a high cover of Bracken fern; (F) secondary grassland on a drier, north-facing slope with relatively high altitude and steep slope; (G) abandoned Pinus plantation with recently debarked adult Pinus trees and a thick cover of pine litter; (H) abandoned Eucalyptus plantation showing a Eucalyptus individual resprouting following felling and fire.
The sites ranged in size from 1 to 38 ha. Natural forest was excluded, and none of the plantation units were likely to have been natural forest prior to afforestation.
2.3.1 Vegetation sampling
Vegetation structure and composition was sampled at points semi-randomly allocated in Google Earth within the 2 unplanted grassland sites, 6 secondary grassland sites, 3 Pinus plantations and 12 Eucalyptus plantations. The sampling regime was originally designed for a decadal-scale monitoring of vegetation change, using a staggered, four-year rotational sampling schedule. The first round of samples were used for this analysis, with the majority (71) being sampled in 2019 to 2021, and an additional 26 sampled in 2022 and 2023.
Vegetation structure was sampled in belt transects of 20 m length and 2 m width. Within these all individual woody plants (trees and shrubs) were identified and recorded, the height of the highest leaf measured for juvenile trees and shrubs, and diameter at breast height for adult trees (which were all Eucalyptus or Pinus trees). Any individuals less than 30 cm tall were categorized as seedlings and counted but not measured. At each 1 m point along the transect the cover of herbaceous vegetation was sampled in twenty 50 cm × 50 cm quadrats. In each of these, the percentage aerial cover of grasses, sedges, forbs, Bracken fern (Pteridium aquilinium (L.) Kuhn subsp. aquilinum), other ferns, litter and bare ground was visually estimated, and litter depth measured near each corner.
Herbaceous plant diversity was sampled by identifying and estimating the aerial cover of each herbaceous species in 1 m × 1 m quadrats. These were located at the start point and end points of most transects, with some additional quadrats randomly located within each site to increase sample sizes.
Initially 3 transects and 9 quadrats were located in each site. However, following examination of the historical aerial photography, some of the boundaries of the sites were adjusted to correct initial assumptions regarding the original vegetation type and afforestation history. Others had to be discarded due to severe fires or unregulated clearing immediately before sampling. These adjustments resulted in unbalanced replication, with between 2 - 7 transects and 4 - 13 herbaceous quadrats per site. Supplementary Table S1 provides the altitude, afforestation and fire history for each site.
2.3.2 Abiotic variables
To try to account for major abiotic differences across the study area, sites were assigned to one of three geographical groups that varied according to precipitation, soils and mostly likely micro-climate:
1. The south-facing slopes, located in the northern part and at higher altitude (approximately 1300 m. a. s. l) with high rainfall.
2. The north-facing slopes, located in the south-western part, and at similar altitude to the south-facing slopes, but warmer and with approximately half the average rainfall, and generally on steeper slopes.
3. The valley, located in the eastern part, and at lower altitude but with rainfall intermediate between the other two groups, and mostly on gentle slopes and with deeper soils.
Secondary grasslands, Eucalypt and Pinus vegetation sites occurred in each of these geographical groups, but primary grassland was absent from the ‘valley’ group.
2.3.3 Fire history
While fire would have been suppressed and largely absent while forestry was active at the site, wildfires have occurred regularly over the past decade. To estimate recent fire frequencies for each site, the ΔNBR (differenced Normalised Burn Ratio index; Ch and Benson, 2006) was computed for the study area for 2014 to 2022 using Google Earth Engine and ArcGIS 3.0.0, and the raster (Hijmans et al., 2015) and tmap (Tennekes, 2018) packages in R statistical software. This index is one of the most widely-used for burn severity mapping (Pfoch et al., 2023). It employs NIR (near infrared) and SWIR (shortwave infrared) bands of multispectral sensors that are useful for detecting the combustion of vegetation (García and Caselles, 1991). Typically, analysis involves identification and contrasting the spectral signatures of pre- and post-fire periods. For each year, pre-fire satellite imagery were constructed using a date range spanning 1 December to 30 April. This period is the second half of the wet season, when vegetation is green with high moisture contents and fires are rare. Post-fire imagery was constructed for 1 May to 30 November of the focal year, a period of low rainfall when vegetation senesces and fires occur. Only images containing zero clouds, snow and cloud shadows {band: pixel_qa; bits 3 to 5 (cloud shadow, snow and cloud)} were selected. This resulted in excluding the year 2015 and 2022. The near- and short-wave infrared bands from the Landsat 8 OLI Surface Reflectance Tier 1 product were used to derive the index values following a workflow developed by the United States Geological Survey and the United Nations, where resultant pixel values are divided into 8 classes (Keeley, 2009; Vermote et al., 2016). Upon inspection of the index values and comparison with large resolution (sub-meter) aerial photography available for the study area for late post-fire period (October) in 2021 and 2022, it was decided to only include values of >440 (moderate to high burn severity) for burnt areas. ΔNBR values were calculated for each year and each sampling point. This allowed for the determination of whether a point experienced a moderate to high burn severity for the respective year which was treated as a definitively burnt area. The number of fires between 2014 and 2022 was then calculated for each site, based on whether sampling points within the site were categorized as burnt each year. If a site contained points indicating both burnt and not burnt, in a year, the entire site was assumed to have burnt that year.
2.3.4 Data analysis
To quantify vegetation structure, the density of trees and shrubs was calculated per transect, as well as the mean cover of Bracken fern and herbaceous species in the 20 quadrats per transect. Mean litter depth was calculated for each quadrat and the mean of these used for a single transect value. The mean of the transect values was then calculated to create a mean site value for each of these variables (Supplementary Tables S2, S3). For herbaceous plant diversity, the replication of herbaceous quadrats was considered too low and unbalanced to estimate the species pool for each site, or compare composition between sites. Instead, diversity was only estimated as the mean number of species per 1m2 quadrat, a simple measure of alpha diversity for each site. This was calculated separately for graminoid (grasses and sedges) and forb species. Differences in these means were tested with a mixed linear model in R using the ‘nlme’ package (Pinheiro et al., 2023), with ecosystem type as a main effect and site as a random effect. Separate tests were performed for graminoid and forb species, and in both cases the effect of ecosystem type was significant at p = 0.05. Differences between the ecosystem type means were tested with a post doc Tukey test using the emmeans package (Lenth and Lenth, 2018). To estimate the total species pool for the study area, species accumulation curves were calculated separately for forest and savanna woody species and herbaceous species, using transects and quadrats as the sampling units, respectively. The exact method was used, and the Chao estimate for extrapolation.
The relationship between the vegetation of the sites, their abiotic group and fire was explored using Non-metric Multidimensional Scaling (NMDS) ordination with euclidean distances, strong ties and 50 random restarts. To characterize the vegetation of each site, the mean values for variables describing the abundance of woody vegetation (the density of adult and juvenile trees categorized as either indigenous forest, indigenous savanna, Pinus or Eucalyptus), the percentage cover of the most common ground cover groups (herbaceous vegetation excluding Bracken fern, litter and Bracken fern), and the species richness of the herbaceous layer (the mean number of species per 1 m2 quadrat for graminoid or forb species) were used. An ordination with 2 dimensions provided a good fit, with a stress of 0.097. Adding dimensions resulted in marginal reductions in stress (Supplementary Figure S1). The above analyses were performed in R using the ‘vegan’ package (Oksanen et al., 2022).
3 Results
Analysis of historical aerial photography indicated that by 1938, plantations covered over 20% of the study area although grassland was still the dominant vegetation type (Figure 3). By 1970, the area under plantation had greatly increased, with grassland declining to a combined total of around 15%. Plantation cover was unlikely to have increased further after 1970 and declined rapidly in the 2000s, with a concurrent increase in grassland and transitional vegetation.
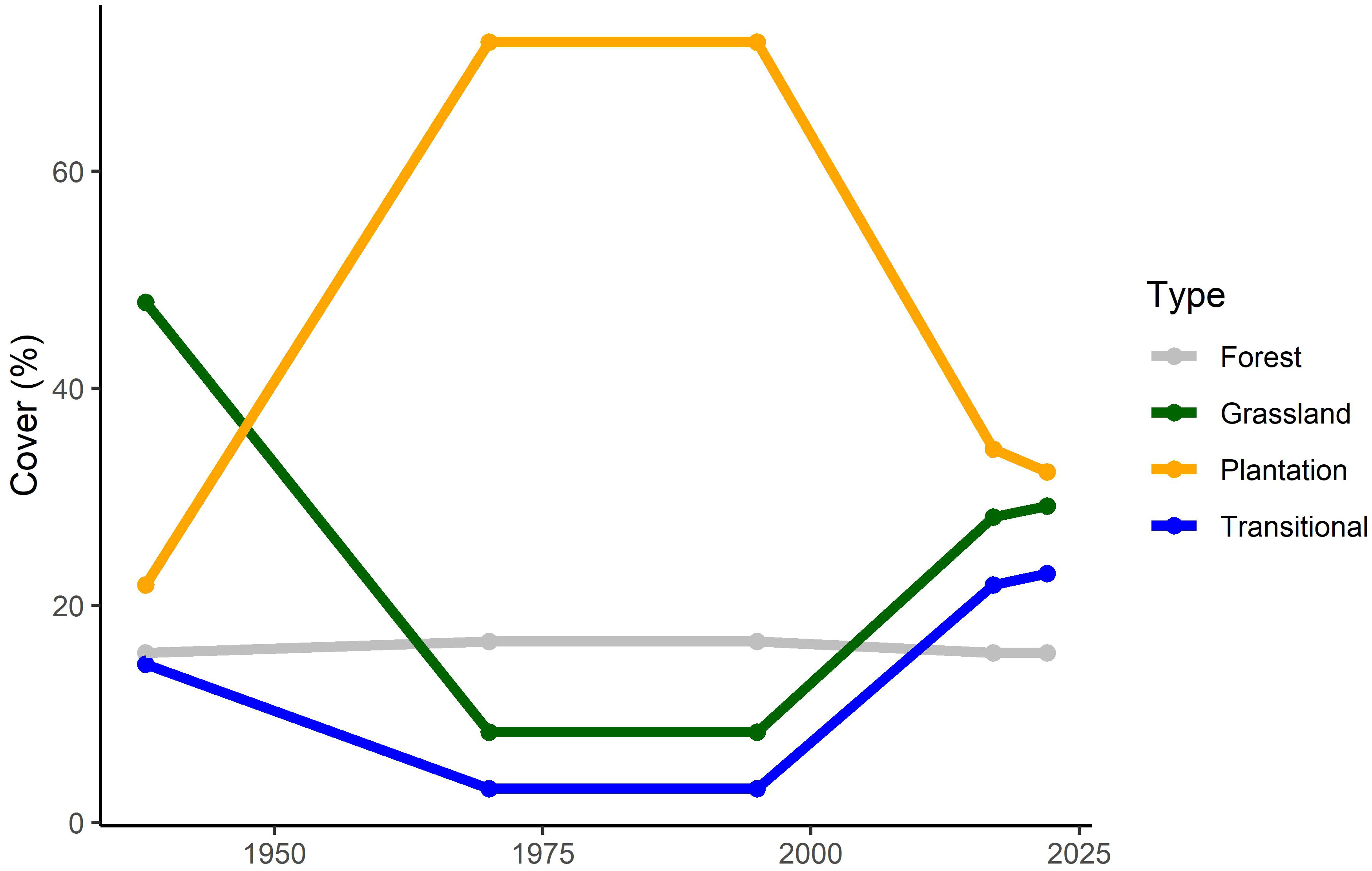
Figure 3 The relative cover of the four ecosystem types of the study area, based on 100 random points placed in historical aerial photography (1938, 1970/71 and 1995) and Google Earth imagery (2017 and 2022). Each point was visually classified as either indigenous forest, plantation, grassland (primary or secondary grassland with no or sparse shrubs or trees) or transitional (areas with partial tree cover, usually of both alien and indigenous juvenile trees).
Ordination indicated that sites of primary grassland and secondary grassland were similar in terms of vegetation structure (Figure 4A). Remaining Pinus and Eucalyptus plantations were distinct, with the former having the highest densities of juvenile indigenous forest species, but no juvenile Pinus. Remaining Eucalyptus plantations had a wide range of structure, which was expected as this category included old, uncut plantations as well as previously cut areas which had resprouted and some which had also burnt and resprouted. A subset with higher densities of adult Eucalyptus also had many juveniles and juvenile Pinus, deeper litter and low cover and species richness of herbaceous plants. These were apparently sites where the plantation species have been able to persist and recruit. Another subset was more similar to the secondary grasslands in terms of herbaceous vegetation, and had fewer plantation trees but more juveniles of other alien species and the most indigenous savanna woody species. These were perhaps sites in transition to secondary grassland. The secondary grassland sites, as well as the two unplanted grassland sites, were separated from all the plantation sites by a high cover of herbaceous species, higher numbers of forb and graminoid species, and lower densities of all woody species groups. The cover of Bracken fern was associated with the variation between these sites.
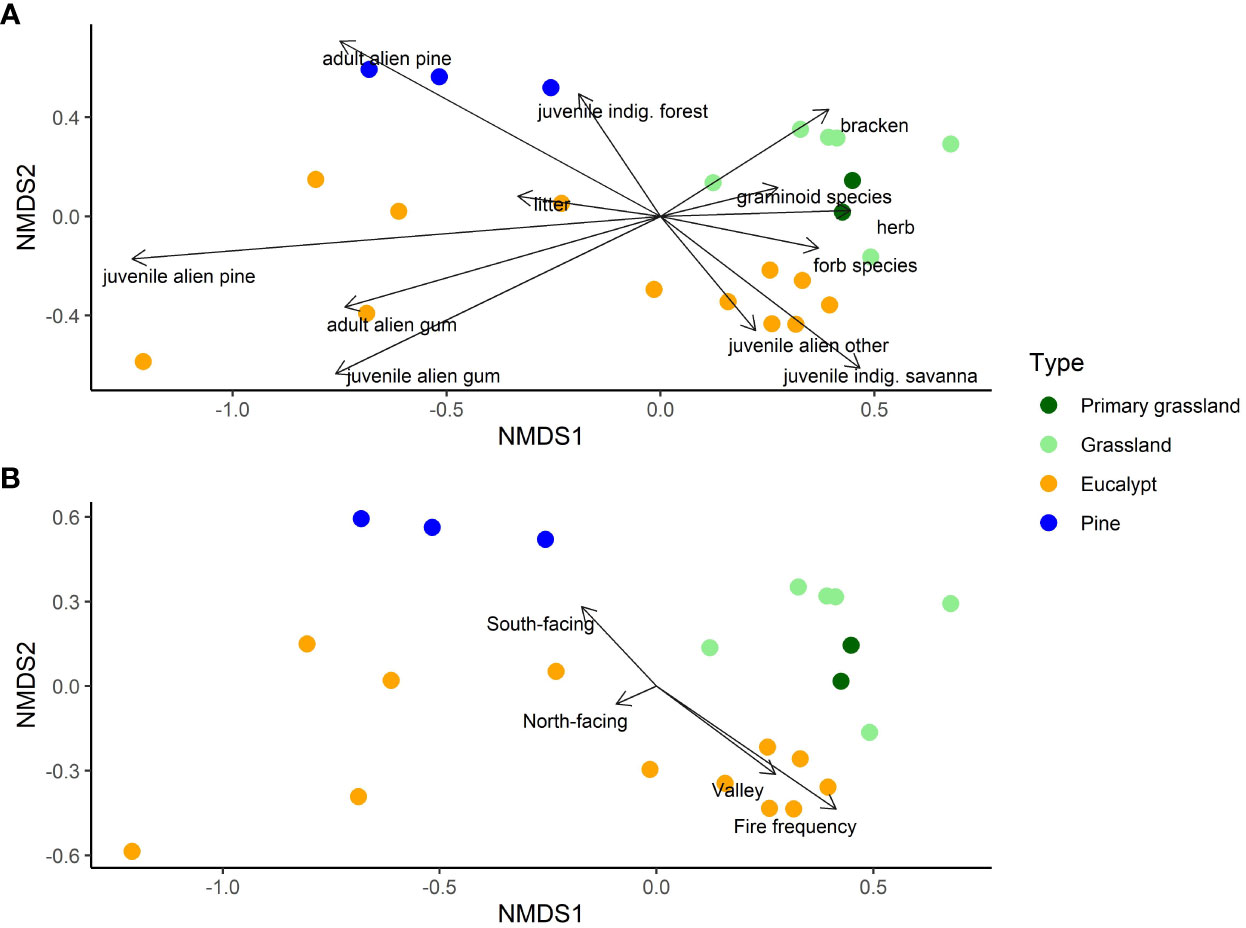
Figure 4 Ordination plots of the NMDS showing the relationship of the four ecosystem types to the vegetation variables (A) and selected environmental determinants (B) for the 23 sites. In (A), ‘adult.alien.gum’ and ‘pine’ refers to Eucalyptus and Pinus trees with stems greater than 10cm diameter at breast height, and ‘juvenile…’ to ones smaller that this but taller than 30cm; ‘juvenile.alien.other’ refers to other alien tree species less than 5m in height; ‘juvenile indig. forest’ to indigenous woody species that occur in forests and ‘juvenile indig. savanna’ to those that do not occur in the local forests; ‘herb’ is the aerial cover of herbaceous species, and ‘bracken’ the aerial cover of Bracken fern; ‘forb species’ and ‘graminoid species’ refer to species richness. In (B), ‘North-facing’ and ‘South-facing’ indicates sites at higher altitude of different aspect and ‘Valley’ sites at lower altitude. “Fire frequency” is the number of years each site burnt in 7 years between 2014 and 2021.
When the abiotic and fire history variables were overlain on the ordination, fire frequency was most strongly correlated with the NMDS axes (Figure 4B). None of the Pinus and some of the Eucalyptus sites burnt, between 2014 and 2021, while other Eucalyptus sites and grassland sites burned 3 or 4 times. These latter were mostly in the valley group, while the Pinus sites and some unburnt Eucalyptus sites were on the cooler, wetter south-facing slopes. Only one secondary grassland site occurred in the valley, and it experienced the maximum fire frequency, while the other grassland sites at higher altitude experienced the full range. The confounding of the abiotic group with fire - with more frequent fire in the valley - is clear from Figure 5A.
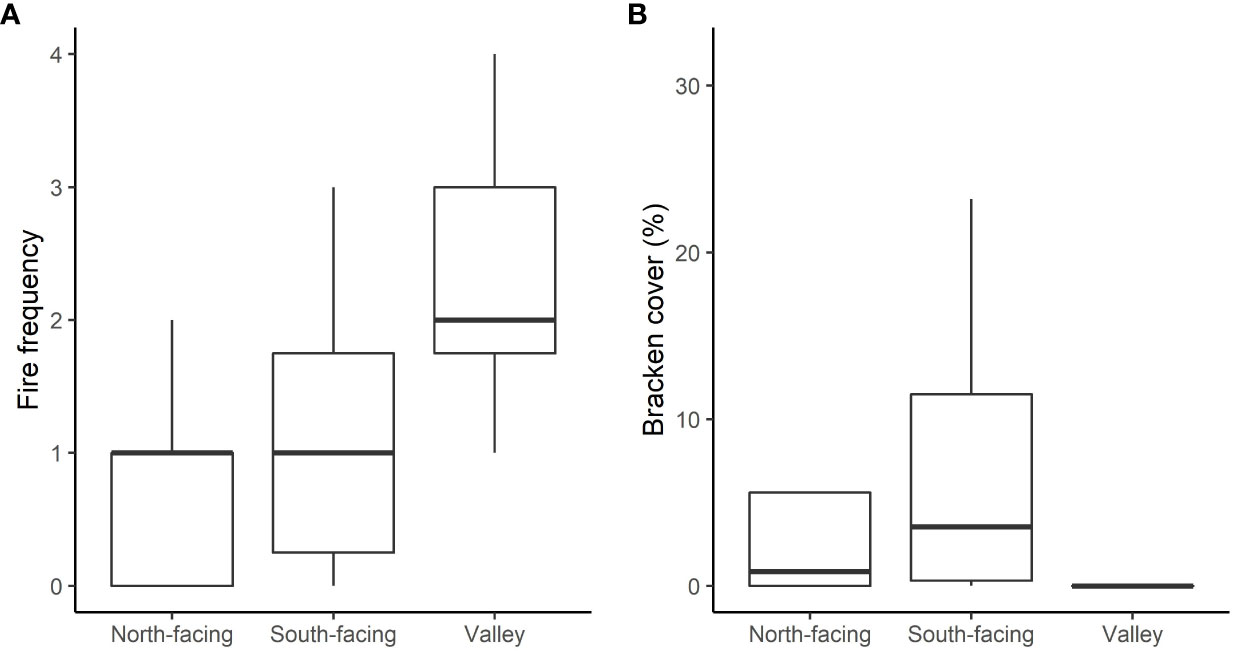
Figure 5 Boxplots showing the variation of fire frequency (A) and the aerial cover of Bracken fern (B) with the three abiotic groups. These group sites according to similar aspect and precipitation. Lines are medians, boxes show the inter-quartile range and whiskers are 1.5 × the inter-quartile range.
A total of 23 forest and 38 savanna woody species, and 166 herbaceous species were recorded across all the sites, with extrapolated estimates of total pool sizes (± standard error) of 26 ± 3.6, 45 ± 5.4 and 231 ± 23.7 respectively (Supplementary Figure S2). The mean number of species recorded per quadrat was substantially greater in the primary grassland for graminoids, but not forbs (Figure 6). Graminoid richness was higher in the secondary grasslands than the plantations, but below that of the primary grassland (Figure 6). This pattern was not evident for forbs, for which richness was low in the Pinus sites but similar for all the other ecosystem types.
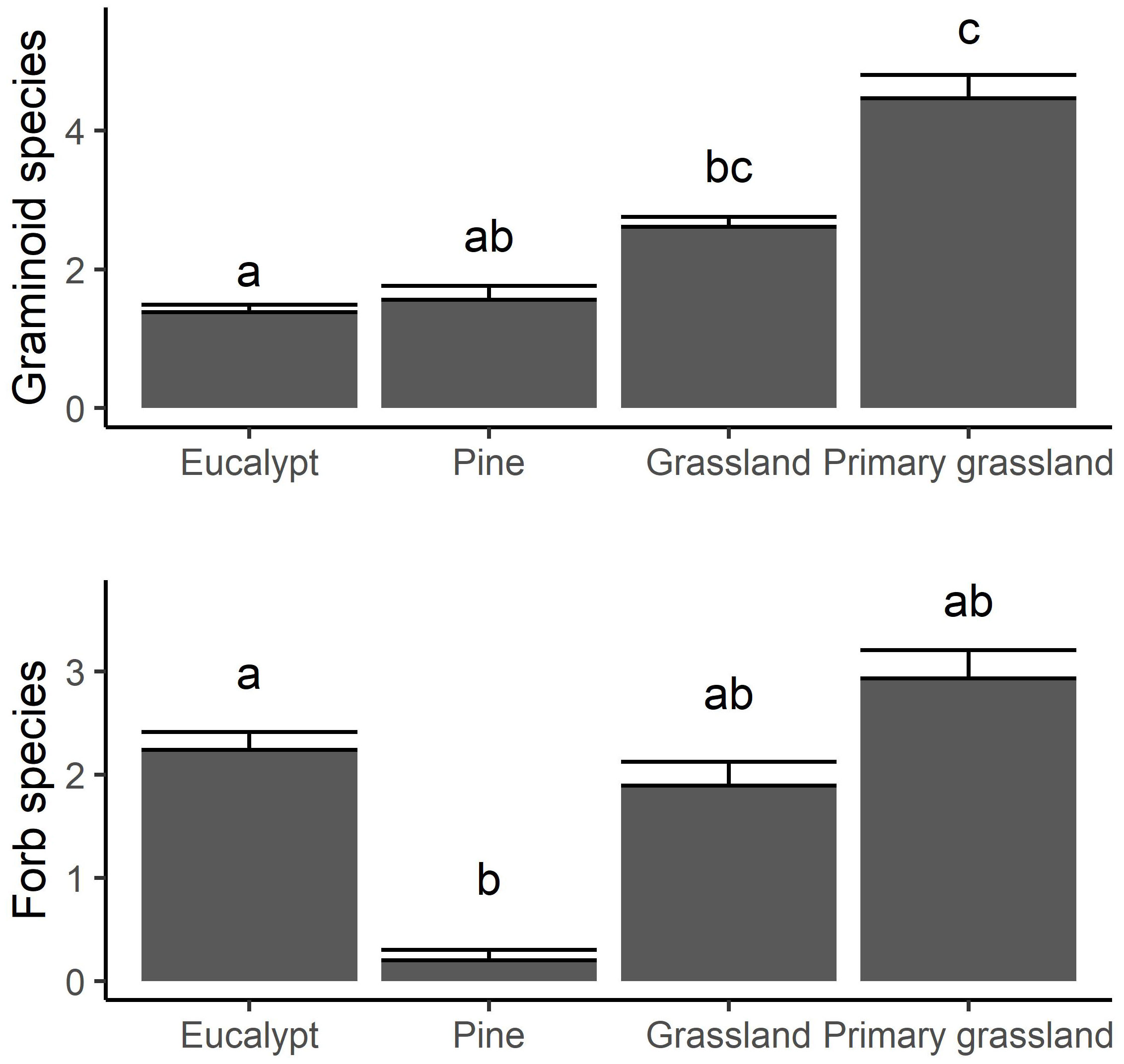
Figure 6 The mean number of graminoid (top) and forb (bottom) species recorded in the 1 m2 quadrats in the four ecosystem types. Error bars show 1 standard error, and subscripts indicate statistically distinct groups at p = 0.05 according to a Tukey post hoc test applied to a linear mixed model.
Variation in herbaceous species richness was correlated with both forb species richness and graminoid species richness (r = 0.82, p < 0.01 in both cases) but forb and graminoid richness were not related to each other (r = 0.42, p > 0.05) and high numbers of both were present at sites with the highest herbaceous richness. At many sites, forb richness was no greater than graminoid richness. Fire frequency was the best univariate predictor of forb richness, and more frequent fire appeared to result in greater forb species richness even amongst the valley sites (Figure 7). In contrast, there was little effect of fire on graminoid richness (r2 = 0.08, P > 0.1).
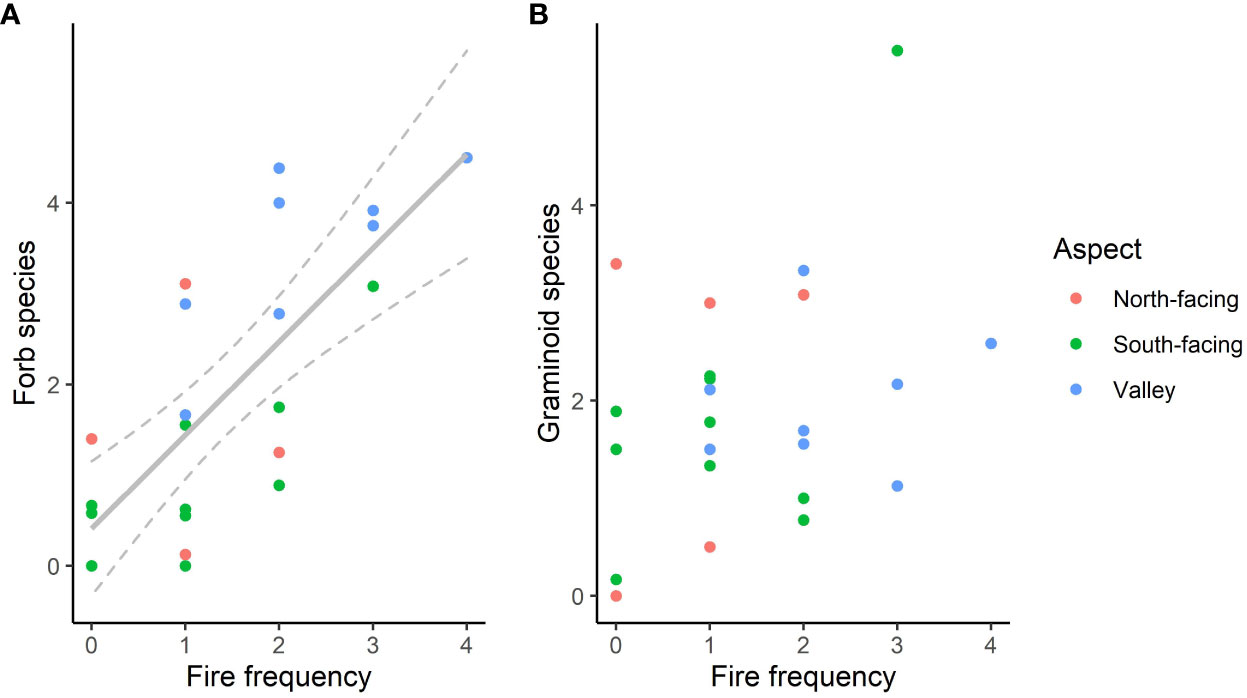
Figure 7 Forb species richness (A) and graminoid species richness (B) versus the number of years each site burnt in 7 years from 2014 and 2021. In (A), the grey line shows a linear regression with 95% confidence intervals (r2 = 0.54, p < 0.01).
Bracken cover, another potential determinant of herbaceous diversity, did not have any significant linear effects on forb or graminoid richness. However, an envelope effect was evident, with low richness at sites where Bracken cover was above 10%, particularly for forb richness. Any effects of Bracken cover were also confounded with the abiotic group and fire, with Bracken being almost entirely absent from the valley sites and most common on the south-facing slopes (Figure 5B), and having low cover at the sites which burned the most. While an absence of Bracken did not necessarily result in high herbaceous richness and other factors where controlling richness at the valley sites, a high cover may still have been the direct cause of lower richness at the higher altitude sites, with low fire frequency being the cause of high Bracken cover. However, Bracken cover did not have a significant co-efficient (at p = 0.05) in multiple linear regression of forb richness versus fire frequency and Bracken cover, nor did this model produce a lower AIC than the univariate regression with fire frequency alone.
4 Discussion
A combination of felling plantation trees and regular fire appears to have been the main driver of transitions from plantation to secondary grasslands over the past 20 years at our study site. Due to the limited and ad hoc removal of plantation stands, the area consisted of a complicated mosaic of undisturbed indigenous forest, a few small remnants of primary grassland, secondary grasslands, and extensive novel ecosystems derived from plantations of a variety of size, shape and plant composition. For many Eucalyptus plantations, transitions to secondary grassland had either not occurred, or are occurring very slowly. Extensive areas of Eucalyptus that had been felled and burnt regularly still existed as novel savanna ecosystems, with low herbaceous species richness and dominated by resprouting Eucalyptus and various other woody species. It is likely that herbicide application is needed to complete the transition of these areas to grassland, as presumably occurred in other Eucalyptus plantations that are now secondary grasslands.
Despite an absence of planned burning or extensive clearing of invasive aliens, the secondary grasslands which have recently developed were largely free of alien species. The climate of the study area would allow forest or very dense savanna to develop in any of these sites, but indigenous tree species were also found at low densities. This presumably indicates that the fire frequency that has emerged following the exit of formal forestry - largely as a result of unplanned wildfires and perhaps lightning ignition – is adequate to allow for the maintenance and even expansion of the secondary grasslands.
The secondary grasslands generally had high herbaceous cover, and species richness was reasonably high albeit well below that of the few remaining patches of pristine grassland. While it is difficult to make statistical comparisons with the few studies from primary grasslands within the broader region (due to differences in sampling methods), a crude comparison suggests that species richness in the secondary grasslands at our site was similar to that found in protected areas nearby. The 91 species recorded in the 75 quadrats in secondary grasslands in our study equates to 1.2 species per m2 sampled, compared to less than 0.5 for grasslands in protected areas at other sites along same mountain range: Uys et al. (2004) recorded 141 species in 27 plots of 1000m2 each (~ 0.5 species per m2) at mesic savanna site and 89 species in 27 plots of 1000m2 each (~ 0.3 species per m2) at a montane grassland site; Zaloumis and Bond (2016) recorded 164 species in 16 plots of 78m2 each (~ 0.1 species per m2) at dry grassland site and 174 species in 12 plots of 78m2 each (~ 0.2 species per m2) at wet montane grassland site; Matjie (2019) recorded 47 species in 40 plots of 1m2 each (~ 1.2 species per m2) at a nearby grassland site. While this crude comparison shows an impressive richness of herbaceous species, it must be noted that it does not consider the type of species present, and it is likely that functional diversity is lower in the secondary grasslands. Furthermore, forbs comprised only 60% of the species recorded in the secondary grasslands in this study, a lower percentage than in all the studies cited above. Finally, while the montane grasslands of this region are known for high levels of endemism (Clark et al., 2022), only two endemic species were recorded in this study.
When considering the combined richness of herbaceous and woody species, the diversity of the site is also impressive. Prior to plantations, the site was a mosaic of grassland (often containing savanna tree species) and forest. Our estimate of total plant species pool for the study area was 231 herbaceous and 45 savanna woody species. While forest was not sampled, a phytosociological study of forests of the region reported 260 species for the forest types within our site (Lötter et al., 2014). That equates to a total plant species pool of 536 species in an area of 4000 ha. Together with the unknown diversity of forest and grassland fauna, this makes the area potentially very valuable for conservation within the region, even if the plant diversity of the secondary grassland does not increase any further. However, regular fires will be needed to maintain a stable co-existence of forest and grassland and prevent encroachment of forest into grassland (Valencia et al., 2023), and further research is need to determine the appropriate frequency and seasonality of fire for this.
We found that the secondary grasslands in the lower altitudes of the area had higher herbaceous species richness, with some evidence that low fire frequency was responsible for the relatively low richness at higher altitudes. More frequent fires in the valley may be a result of long dry season at lower altitude, where vegetation is likely to be in a senescent and more flammable state for a longer time at the end of each dry season. The south-facing slopes are also protected from fires spreading up from below by surrounding tracts of forest. The valley may also experience far more ignitions as cattle graze the lower slopes and valley and cattle owners are known to light fires in the area in the late dry season, to open the vegetation and promote a flush of green grass. Fire frequency appears to be increasing on the wetter south-facing slopes, with a few fires deliberately set by managers in recent years, in an attempt to promote restoration. The planned repeated sampling of the sites used in this study should reveal if that leads to the expected increase in forb species richness. Fire frequency has been found to promote forb diversity at a pristine montane grassland site in the region, with maximum richness in annual burning treatments (Uys et al., 2004). More focused research is needed to confirm this role of fire in secondary grasslands in this regard, as well as the ecological mechanisms that lead to increased forb establishment with frequent burning. Research on other interventions to increase forb diversity, such as seeding or transplanting (Carbutt and Kirkman, 2022) would also be valuable, even if such interventions are not feasible for the entire area.
An additional factor that may have had a negative effect on herbaceous diversity was a high cover of Bracken fern, an indigenous invasive species that can dominate stands of grasslands and exclude other herbaceous species, even in undisturbed montane grasslands (Singh et al., 2013). The absence of Bracken from the valley sites may be an important reason for the higher forb diversity there. Determining the reason(s) for its absence (ostensibly the warmer climate) would be insightful and valuable for the management of other secondary grasslands where Bracken occurs or may invade.
Further monitoring is needed before any conclusions can be made with confidence regarding the long-term, stable state of the emerging ecosystems in the abandoned plantations of this area. As is more focused research regarding the levels of beta diversity and rarity that have developed thus far, and some estimation of the ecosystem services they are now providing. However, our results already indicate that even with modest interventions, secondary grasslands supporting reasonable plant diversity can establish and persist in montane areas subjected to plantation forestry for up to 75 years.
As global climate change intensifies and water scarcity becomes more severe in many parts of the world, more plantations may be abandoned to secure adequate river flows during dry seasons and droughts. Private forestry companies may choose to convert plantations to crops or orchards which, in the case of South Africa at least, requires a reduction in the area under use in order to comply with water use legislation. They may also choose to decommission sections of their plantations to meet environmental and social governance targets. Increases in temperature and evapotranspiration, reductions in rainfall, and associated risks of increased wildfires, pathogens and pests could make commercial forestry economically unviable in some montane areas over the coming decades (Booth, 2013; Pinkard et al., 2015; Watt et al., 2019; Teshome et al., 2020).
Socio-economic research to identify montane regions where future abandonment of plantations might occur is needed. Finally, an additional emerging barrier to such land use change must be considered - afforestation for the purpose of selling carbon credits. Any decision-maker considering the fate of abandoned cropland or plantation now days would be tempted to consider reforestation for the purpose of earning carbon credits, rather than conservation. The recent drive to plant trees for carbon sequestration actually pose a risk to both primary and secondary grasslands (Bond, 2016; Dudley et al., 2020), and brings potential social costs (Lyons and Westoby, 2014). Rates of carbon sequestration in the secondary ecosystems studied here are unstudied, and quantifying this could be valuable as a potential counter-argument to any future reforestation threat, and informative for restoration initiatives in montane grasslands elsewhere. In addition, outreach initiatives are needed to dispel misconceptions that grasslands are degraded forests, which is common in our study region as well as many other parts of the world (Bond et al., 2008; Lahiri et al., 2023)
5 Conclusion
The abandoned plantations of the Mariepskop area have provided a natural experiment on large-scale, passive restoration of montane grassland. Our initial research shows natural regeneration of grassland structure and substantial plant diversity within a few decades, highlighting an opportunity for increasing the conservation of montane grasslands in similar areas elsewhere in the world. Future research on the diversity of other taxa, the role of regular fire, and the ecosystem services provided by these secondary ecosystems, including carbon sequestration, are needed to further elucidate the potential value of restoring grasslands and promote them as an alternative land use of montane environments.
Data availability statement
The datasets analyzed for this study can be found in the SAEON Data Portal https://catalogue.saeon.ac.za/).
Author contributions
AS: Conceptualization, Formal analysis, Funding acquisition, Investigation, Methodology, Project administration, Resources, Writing – original draft. RL: Formal analysis, Methodology, Writing – review & editing. DT: Writing – review & editing. JG: Conceptualization, Methodology, Writing – review & editing. LS: Methodology, Writing – review & editing. MM: Methodology, Writing – review & editing.
Funding
The author(s) declare financial support was received for the research, authorship, and/or publication of this article. This research was funded with internal funding of the South African Environmental Observation Network, a division of the National Research Foundation and funded by the South African Department of Science and Innovation, and a research contract from the Natural Resources Management division of the South African Department of Forestry, Fisheries and Environment (DFFE).
Acknowledgments
The South African Department of Forestry, Fisheries and Environment (DFFE) provided access to the site and the Mariepskop Forest Manager, Mr Winners Mashego, is thanked for facilitating this access and providing information on the recent history of the site. Staff of the Kruger-to-Canyons Biosphere Reserve NPO assisted with some of the vegetation sampling and facilitated initial engagements with DFFE.
Conflict of interest
The authors declare that the research was conducted in the absence of any commercial or financial relationships that could be construed as a potential conflict of interest.
Publisher’s note
All claims expressed in this article are solely those of the authors and do not necessarily represent those of their affiliated organizations, or those of the publisher, the editors and the reviewers. Any product that may be evaluated in this article, or claim that may be made by its manufacturer, is not guaranteed or endorsed by the publisher.
Supplementary material
The Supplementary Material for this article can be found online at: https://www.frontiersin.org/articles/10.3389/fcosc.2024.1297148/full#supplementary-material
References
ABARES (2023). Australian Plantation Statistics. 2023 Update. Australian Bureau of Agricultural and Resource Economics and Sciences (ABARES). doi: 10.25814/hhk8-4x26
Arasumani M., Khan D., Das A., Lockwood I., Stewart R., Kiran R. A., et al. (2018). Not seeing the grass for the trees: Timber plantations and agriculture shrink tropical montane grassland by two-thirds over four decades in the Palani Hills, a Western Ghats Sky Island. PloS One 13, e0190003. doi: 10.1371/journal.pone.0190003
Bai Y., Cotrufo M. F. (2022). Grassland soil carbon sequestration: Current understanding, challenges, and solutions. Science 377, 603–608. doi: 10.1126/science.abo2380
Bond W. J., Parr C. L. (2010). Beyond the forest edge: Ecology, diversity and conservation of the grassy biomes. Biol. Conserv. 143, 2395–2404. doi: 10.1016/j.biocon.2009.12.012
Bond W. J., Silander J. A. Jr., Ranaivonasy J., Ratsirarson J. (2008). The antiquity of Madagascar’s grasslands and the rise of C4 grassy biomes. J. Biogeogr. 35, 1743–1758. doi: 10.1111/j.1365-2699.2008.01923.x
Booth T. H. (2013). Eucalypt plantations and climate change. For. Ecol. Manage. 301, 28–34. doi: 10.1016/j.foreco.2012.04.004
Boval M., Dixon R. M. (2012). The importance of grasslands for animal production and other functions: a review on management and methodological progress in the tropics. animal 6, 748–762. doi: 10.1017/S1751731112000304
Buisson E., Archibald S., Fidelis A., Suding K. N. (2022). Ancient grasslands guide ambitious goals in grassland restoration. Science 377, 594–598. doi: 10.1126/science.abo4605
Buisson E., Fidelis A., Overbeck G. E., Schmidt I. B., Durigan G., Young T. P., et al. (2021). A research agenda for the restoration of tropical and subtropical grasslands and savannas. Restor. Ecol. 29, 1–18. doi: 10.1111/rec.13292
Cano D., Cacciuttolo C., Custodio M., Nosetto M. (2023). Effects of grassland afforestation on water yield in basins of Uruguay: A spatio-temporal analysis of historical trends using remote sensing and field measurements. Land 12, 185. doi: 10.3390/land12010185
Carbutt C., Kirkman K. (2022). Ecological grassland restoration—A South African perspective. Land 11, 575. doi: 10.3390/land11040575
Carbutt C., Thompson D. I. (2021). Mountain watch: how LT(S)ER is safeguarding southern Africa’s people and biodiversity for a sustainable mountain future. land 10, 1–27. doi: 10.3390/land10101024
Ch K., Benson N. (2006) Landscape assessment: ground measure of severity, the Composite Burn Index; and remote sensing of severity, the Normalized Burn Ratio (USDA Forest Service, Rocky Mountain Research Station, Ogden, UT: USDA Forest Service, Rocky Mountain Research Station). Available at: https://cir.nii.ac.jp/crid/1572543024897547776 (Accessed December 12, 2023).
Clark V. R., Burrows J. E., Turpin B. C., Balkwill K., Lötter M., Siebert S. J. (2022). The limpopo–mpumalanga–Eswatini escarpment—Extra-ordinary endemic plant richness and extinction risk in a summer rainfall montane region of southern Africa. Front. Ecol. Evol. 10. doi: 10.3389/fevo.2022.765854
Davenport T. R. B., Ndangalasi H. J. (2003). An escalating trade in orchid tubers across Tanzania’s Southern Highlands: assessment, dynamics and conservation implications. Oryx 37, 55–61. doi: 10.1017/S0030605303000127
Dudley N., Eufemia L., Fleckenstein M., Periago M. E., Petersen I., Timmers J. F. (2020). Grasslands and savannahs in the UN decade on ecosystem restoration. Restor. Ecol. 28, 1313–1317. doi: 10.1111/rec.13272
Eckert M., Gaigher R., Pryke J. S., Samways M. J. (2019). Rapid recovery of soil arthropod assemblages after exotic plantation tree removal from hydromorphic soils in a grassland-timber production mosaic. Restor. Ecol. 27, 1357–1368. doi: 10.1111/rec.12991
Farley Wolf K. (2007). Grasslands to tree plantations: forest transition in the Andes of Ecuador. Ann. Assoc. Am. Geographers Ann. ASSN AMER Geogr. 97, 755–771. doi: 10.1111/j.1467-8306.2007.00581.x
García M. J. L., Caselles V. (1991). Mapping burns and natural reforestation using thematic Mapper data. Geocarto Int. 6, 31–37. doi: 10.1080/10106049109354290
Hermann J., Lang M., Gonçalves J., Hasenack H. (2016). Forest–grassland biodiversity hotspot under siege: land conversion counteracts nature conservation. Ecosys. Health Sustain. 2, e01224. doi: 10.1002/ehs2.1224
Hijmans R. J., Van Etten J., Cheng J., Mattiuzzi M., Sumner M., Greenberg J. A., et al. (2015). Package ‘raster.’. R Package 734, 473.
Hoekstra J. M., Boucher T. M., Ricketts T. H., Roberts C. (2005). Confronting a biome crisis: global disparities of habitat loss and protection. Ecol. Lett. 8, 23–29. doi: 10.1111/j.1461-0248.2004.00686.x
Jackson R. B., Jobbágy E. G., Avissar R., Roy S. B., Barrett D. J., Cook C. W., et al. (2005). Trading water for carbon with biological carbon sequestration. Science 310, 1944–1947. doi: 10.1126/science.1119282
Keeley J. E. (2009). Fire intensity, fire severity and burn severity: a brief review and suggested usage. Int. J. Wildland Fire 18, 116–126. doi: 10.1071/WF07049
Lahiri S., Roy A., Fleischman F. (2023). Grassland conservation and restoration in India: a governance crisis. Restor. Ecol. 31, e13858. doi: 10.1111/rec.13858
Lenth R., Lenth M. R. (2018). Package ‘lsmeans.’. Am. Statistician 34, 216–221. doi: 10.1080/00031305.1980.10483031
Lötter M. C., Mucina L., Witkowski E. T. F. (2014). Classification of the indigenous forests of Mpumalanga Province, South Africa. South Afr. J. Bot. 90, 37–51. doi: 10.1016/j.sajb.2013.09.010
Lyons K., Westoby P. (2014). Carbon colonialism and the new land grab: Plantation forestry in Uganda and its livelihood impacts. J. Rural Stud. 36, 13–21. doi: 10.1016/j.jrurstud.2014.06.002
Matjie M. R. (2019) A vegetation survey and mapping of the woodbush granite grassland in the Limpopo Province, South Africa. PhD dissertation (University of Limpopo). Available at: http://ulspace.ul.ac.za/bitstream/handle/10386/3176/matjie_mr_2019.pdf?sequence=1 (Accessed December 12, 2023).
Matthews W. S., van Wyk A. E., Bredenkamp G. J. (1993). Endemic flora of the north-eastern Transvaal Escarpment, South Africa. Biol. Conserv. 63, 83–94. doi: 10.1016/0006-3207(93)90077-E
McEwan A., Marchi E., Spinelli R., Brink M. (2020). Past, present and future of industrial plantation forestry and implication on future timber harvesting technology. J. For. Res. 31, 339–351. doi: 10.1007/s11676-019-01019-3
Mucina L., Rutherford M. C., Powrie L. W. (2006). Vegetation of South Africa, Lesotho and Swaziland. Online, Version 2018. South African National Biodiversity Institute. Available at: http://bgis.sanbi.org/SpatialDataset/Detail/18.
Ndang’ang’a P. K., du Plessis M. A., Ryan P. G., Bennun L. A. (2002). Grassland decline in Kinangop Plateau, Kenya: implications for conservation of Sharpe’s longclaw (Macronyx sharpei). Biol. Conserv. 107, 341–350. doi: 10.1016/S0006-3207(02)00072-1
Nerlekar A. N., Veldman J. W. (2020). High plant diversity and slow assembly of old-growth grasslands. Proc. Natl. Acad. Sci. 117, 18550–18556. doi: 10.1073/pnas.1922266117
Niemandt C., Greve M. (2016). Fragmentation metric proxies provide insights into historical biodiversity loss in critically endangered grassland. Agric. Ecosyst. Environ. 235, 172–181. doi: 10.1016/j.agee.2016.10.018
Nuñez M. A., Chiuffo M. C., Torres A., Paul T., Dimarco R. D., Raal P., et al. (2017). Ecology and management of invasive Pinaceae around the world: progress and challenges. Biol. Invasions 19, 3099–3120. doi: 10.1007/s10530-017-1483-4
Oksanen J., Blanchet F. G., Friendly M., Kindt R., Legendre P., McGlinn D., et al. (2022). vegan: community ecology package. R Package version 2.5-7, 2020.
Pfoch K., Pflugmacher D., Okujeni A., Hostert P. (2023). Mapping forest fire severity using bi-temporal unmixing of Sentinel-2 data - Towards a quantitative understanding of fire impacts. Sci. Remote Sens. 8. doi: 10.1016/j.srs.2023.100097
Pinheiro J., Bates D., DebRoy S., Sarkar D. (2023). Package 'nlme'. Nonlinear mixed-effects models, version 3, 1–164.
Pinkard E., Battaglia M., Bruce J., Matthews S., Callister A. N., Hetherington S., et al. (2015). A history of forestry management responses to climatic variability and their current relevance for developing climate change adaptation strategies. Forestry: Int. J. For. Res. 88, 155–171. doi: 10.1093/forestry/cpu040
Scott D. F., Prinsloo F. W. (2008). Longer-term effects of pine and eucalypt plantations on streamflow. Water Resour. Res. 45, 1–8. doi: 10.1029/2007WR006781
Singh K., Forbes A., Akombelwa M. (2013). The evaluation of high resolution aerial imagery for monitoring of bracken fern. South Afr. J. Geomatics 2, 296–208. doi: 10.4314/sajg.v2i4
Skowno A. L., Monyeki M. S. (2021). South Africa’s red list of terrestrial ecosystems (RLEs). Land 10, 1048. doi: 10.3390/land10101048
Skowno A., Poole C., Raimondo D., Sink K., Van Deventer H., Van Niekerk L., et al. (2019) National Biodiversity Assessment 2018: The status of South Africa’s ecosystems and biodiversity. Synthesis Report (Pretoria, South Africa: South African National Biodiversity Institute). Available at: http://hdl.handle.net/20.500.12143/6362 (Accessed August 29, 2023).
Srinivasan M., Bhatia S., Shenoy K. (2015). Vegetation-environment relationships in a South Asian tropical montane grassland ecosystem: Restoration implications. Trop. Ecol. 56, 201.
Tennekes M. (2018). tmap: Thematic maps in R. J. Stat. Softw. 84 (6), 1–39. doi: 10.18637/jss.v084.i06
Teshome D. T., Zharare G. E., Naidoo S. (2020). The threat of the combined effect of biotic and abiotic stress factors in forestry under a changing climate. Front. Plant Sci. 11. doi: 10.3389/fpls.2020.601009
Uys R. G., Bond W. J., Everson T. M. (2004). The effect of different fire regimes on plant diversity in southern African grasslands. Biol. Conserv. 118, 489–499. doi: 10.1016/j.biocon.2003.09.024
Valencia S., Salazar J. F., Hoyos N., Armenteras D., Villegas J. C. (2023). Current forest–savanna transition in Northern South America departs from typical climatic thresholds. Ecosystems 1–16. doi: 10.1007/s10021-023-00872-y
Van Wilgen B. W. (2015). Plantation forestry and invasive pines in the Cape Floristic Region: Towards conflict resolution. South Afr. J. Sci. 111, 1–2. doi: 10.17159/SAJS.2015/A0114
Veldman J. W. (2016). Clarifying the confusion: old-growth savannahs and tropical ecosystem degradation. Philos. Trans. R. Soc. B: Biol. Sci. 371, 20150306. doi: 10.1098/rstb.2015.0306
Veldman J. W., Buisson E., Durigan G., Fernandes G. W., Le Stradic S., Mahy G., et al. (2015). Toward an old-growth concept for grasslands, savannas, and woodlands. Front. Ecol. Environ. 13, 154–162. doi: 10.1890/140270
Vermote E., Justice C., Claverie M., Franch B. (2016). Preliminary analysis of the performance of the Landsat 8/OLI land surface reflectance product. Remote Sens. Environ. 185, 46–56. doi: 10.1016/j.rse.2016.04.008
Watt M. S., Kirschbaum M. U. F., Moore J. R., Pearce H. G., Bulman L. S., Brockerhoff E. G., et al. (2019). Assessment of multiple climate change effects on plantation forests in New Zealand. Forestry: Int. J. For. Res. 92, 1–15. doi: 10.1093/forestry/cpy024
Zaloumis N. P., Bond W. J. (2011). Grassland restoration after afforestation: No direction home? Austral Ecol. 36, 357–366. doi: 10.1111/j.1442-9993.2010.02158.x
Zaloumis N. P., Bond W. J. (2016). Reforestation or conservation? The attributes of old growth grasslands in South Africa. Philos. Trans. R. Soc. B: Biol. Sci. 371. doi: 10.1098/rstb.2015.0310
Keywords: restoration, fire, species richness, forestry, land cover
Citation: Swemmer AM, Lerm RE, Thompson DI, Graf JA, Stone L and Mashele M (2024) Plant diversity in secondary, montane grasslands – a case study of the abandoned plantations of Mariepskop Mountain, South Africa. Front. Conserv. Sci. 5:1297148. doi: 10.3389/fcosc.2024.1297148
Received: 19 September 2023; Accepted: 05 January 2024;
Published: 25 January 2024.
Edited by:
Peter Taylor, University of the Free State, South AfricaReviewed by:
Thomas Edward Marler, University of Guam, GuamMatthew E. Aiello-Lammens, Pace University, United States
Copyright © 2024 Swemmer, Lerm, Thompson, Graf, Stone and Mashele. This is an open-access article distributed under the terms of the Creative Commons Attribution License (CC BY). The use, distribution or reproduction in other forums is permitted, provided the original author(s) and the copyright owner(s) are credited and that the original publication in this journal is cited, in accordance with accepted academic practice. No use, distribution or reproduction is permitted which does not comply with these terms.
*Correspondence: Anthony M. Swemmer, YW0uc3dlbW1lckBzYWVvbi5ucmYuYWMuemE=