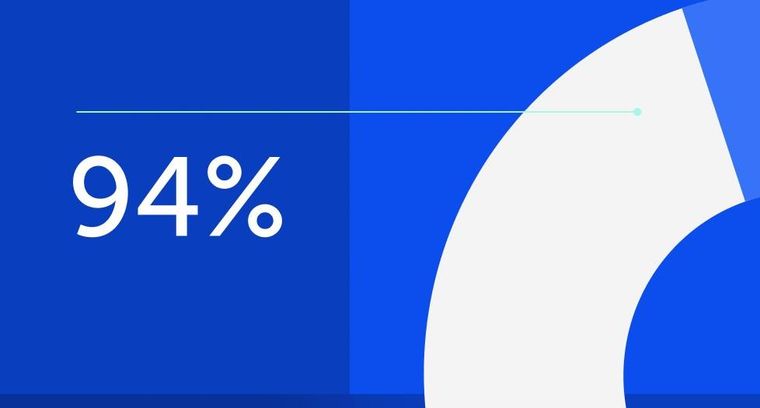
94% of researchers rate our articles as excellent or good
Learn more about the work of our research integrity team to safeguard the quality of each article we publish.
Find out more
ORIGINAL RESEARCH article
Front. Conserv. Sci., 25 July 2023
Sec. Human-Wildlife Interactions
Volume 4 - 2023 | https://doi.org/10.3389/fcosc.2023.981424
Land use, habitat suitability, and seasonality can fundamentally shape small-mammal abundance, species richness, diversity, evenness, and composition. However, how these characteristics of small mammals are determined by land use, habitat type, and rainfall seasonality is still poorly understood for most ecosystems. We analyze how land use (protection in a national park, pastoralism, and crop agriculture), habitat type, and rainfall seasonality influence small-mammal relative abundance, species richness, and diversity in the Tanzania Serengeti Ecosystem. We used 141 live traps to capture 612 small mammals in the wet and dry seasons of 2017 and 2018. Relative abundance was higher in the pastoral land than in the park or agricultural land and in the dry season in all the three land use types. Species richness and diversity were highest in the park, middling in the agricultural land, and lowest in the pastoral land. The high relative abundance in the pastoral land was primarily due to the numerical dominance of two generalist species in the shrubland (grass rat Arvicanthis niloticus) and cropland (multimammate rat Mastomys natalensis), resulting in low species richness and diversity. High species richness and diversity in the park indicate high habitat heterogeneity, whereas high species diversity in the agricultural land during the dry season reflects high food availability during and soon after harvests. Thus, human activities apparently exert deleterious effects on some specialist small mammals as a result of reduced habitat heterogeneity while promoting the abundance of some generalist species in African savanna ecosystems. However, increased abundance of generalist species reduces small mammal species diversity while increasing the risk of human–small mammal conflicts. We offer several testable hypotheses motivated by our results.
Human influence on ecosystems is intensifying worldwide due to rapid human population growth and increasingly resource-consuming lifestyles (Macchi, 2010; Barnosky et al., 2017). This influence has become so important and pervasive that mankind is now, and will likely remain for years to come, the main global driver of ecological change (IPBES, 2019). As a result, human-altered ecosystems made of settlements, agro-pastoral and protected areas, dominate the terrestrial biosphere, covering more than three quarters of the total ice-free land areas (Ellis and Ramankutty, 2008). These alterations to ecosystems have resulted in a global biodiversity crisis that threatens the world’s species and ecosystems alike (Butchart et al., 2010). To counter these alterations, protected areas have been expanding significantly worldwide since 2010. This has resulted in a noticeable reduction in biodiversity loss and increase in species dependent on protected areas (Lehikoinen et al., 2018; Maxwell et al., 2019). Even so, these areas are still unable to halt or reverse these alterations because of intensifying anthropogenic activities and persistent resource constraints (Cazalis et al., 2020; Maxwell et al., 2019). Today, most protected areas, set aside to safeguard the remaining global biodiversity, are surrounded by different human activities, making them isolated “islands” (Estes et al., 2012). The pace, scale, and magnitude of these changes raise fundamental questions concerning whether the existing protected areas will last into the far future and whether they may effectively conserve wildlife species and associated ecosystem functions and services given the prevailing and projected rates of human population growth, activities, and consumption of goods and services (Ogutu et al., 2016, Wilting et al., 2017).
Land use changes and human activities cause and perpetuate biodiversity loss and degrade the associated ecosystem services (Kija et al., 2020; Anley et al., 2022). Notably, about three quarters of the Earth’s land surface has been altered by humans within the 20th century alone (Winkler et al., 2021). The alterations by human activities such as agriculture and livestock production have caused dramatic and unprecedented declines in global species diversity and richness (Newbold et al., 2020). Agriculture is the dominant land use activity on the planet and is responsible for altering and endangering wildlife communities on a massive scale (Hurst et al., 2014). It transforms native vegetation into monocultures, thereby decreasing biodiversity by destroying and homogenizing habitats (Shilereyo et al., 2021). Although agricultural activities can provide food to some wildlife species, a leading conservation concern is that agricultural lands alter wildlife communities, favoring generalists at the expense of specialists (Caro, 2001; Byrom et al., 2015; Chidodo et al., 2020). On the other hand, livestock grazing at low to moderate densities is often more compatible with wildlife conservation (Du Toit et al., 2012; Keesing et al., 2018). However, increasing demand for livestock and livestock products and adoption of improved and less disease-tolerant breeds as a result of growing economic incentives, affluence, and human populations are driving intensification of livestock production systems and threatening wildlife and biodiversity (Cao et al., 2016 Gbogbo et al., 2017). The threats occur through multiple pathways including mechanical disturbance, reduced plant biomass, and changing vegetation composition and structure (Schmidt et al., 2005). The changes in vegetation structure can have several knock-on effects on critical ecosystem functions, such as provision of shelter and food for wild animals, and on their species composition and richness (Cao et al., 2016). The threats also occur through major land transformations and fragmentation, such as through fencing that excludes wildlife from pastures and water points and impedes their free movements (Ogutu et al., 2013; Said et al., 2016).
Understanding how land use affects their abundance, species diversity, and composition is important for understanding and minimizing the deleterious influences of human activities on small mammals, their habitats and ecosystem services. Such understanding should factor in potentially differentiated influences of contrasting land uses and their consequences on small-mammal community characteristics. This is because contrasting land uses, such as grazing and farming activities, can differentially influence small-mammal community characteristics, such as abundance, diversity, and composition (Cao et al., 2016; Magige, 2016; Keesing et al., 2018; Shilereyo et al., 2021) with adverse consequences for ecosystem services, including provisioning and supporting services and buffering disease-causing pathogens (Ralaizafisoloarivony et al., 2014; Young et al., 2017). For example, some small-mammal species (e.g., Mastomys natalensis and Arvicanthis niloticus) tend to be more common in human-dominated habitats and are potential crop pests and hosts for, or reservoirs of, vectors that transmit zoonotic diseases including the plague (Bergstrom, 2013; Mulungu, 2017, Hieronimo et al., 2014). Consequently, it is important to quantify how different land uses affect particular characteristics of small-mammal communities and assess the likely consequences of these impacts on small mammals and their habitats and ecosystem services. This knowledge can then form a sound basis for developing adaptive strategies for effectively conserving small-mammal communities and their habitats. Yet, despite land use change, including agriculture and pastoralism, posing significant widespread and mounting threats to biodiversity, often leading to marked declines in wildlife abundance, diversity, and natural habitats (Haines-Young, 2009, Wilting et al., 2017, Trisurat et al., 2019), relatively few empirical studies have investigated its impacts on small-mammal community characteristics for many ecosystems, including the premier Greater Serengeti Ecosystem.
The few studies undertaken in the Tanzania Serengeti ecosystem have thus far focused on small-mammal species diversity and abundance in contrasting habitats and along elevational gradients (Magige and Senzota, 2006; Magige, 2013), conflicts with humans (Magige, 2012), and influences on their predator abundances (Byrom et al., 2014). A few other studies have also compared protected areas with their adjacent human-dominated habitats to infer the influence of anthropogenic activities on small-mammal abundance and species diversity (Byrom et al., 2015; Magige, 2016). These studies and other earlier assessments of influences of human activities on small-mammal abundance, species richness, and diversity have produced mixed results, ranging from positive, negative, to neutral effects (Cao et al., 2016). Although this is unsurprising given the complex and dynamic interactions among geographical, ecological, historical, and evolutionary processes shaping small-mammal abundance, species richness, and diversity, it highlights the need for further empirical studies that quantitatively assess the nature and consequences of small mammal–human interactions in contrasting contexts to establish the generality of the patterns. We thus compared trapped small-mammal species and abundance in different habitats in areas with different land uses in and around the Serengeti National Park in wet and dry seasons. This combination of land use and habitat as separate factors represents a vast improvement upon designs used by most earlier studies that have considered either only one of the two factors or have even confounded them (Osbourne et al., 2005; Magige and Senzota, 2006; Panzacchi et al., 2009). Such studies would also help assess the generality of the findings of the few earlier studies that have comparatively analyzed small-mammal abundance, species richness, diversity, and composition between protected areas and their adjoining human-inhabited areas across seasons in African savannas, including in the Serengeti ecosystem (Magige and Senzota, 2006; Byrom et al., 2015). Most crucially, even though it is fundamental to improving our understanding of small mammal–human interactions, the effects on small mammals of land use and habitat as separate factors and how seasonality modifies the effects of both factors have rarely been analyzed.
To fill this important gap in our understanding, we asked: how do small-mammal abundance, species richness, diversity, and composition differ seasonally between the protected areas and their adjoining human-inhabited areas and among the different habitats within the different land uses in the Serengeti ecosystem? To answer this question, we analyzed how small-mammal abundance, species diversity, richness, and evenness vary with anthropogenic land use, habitat type, and rainfall seasonality in the Serengeti ecosystem. This analysis expands upon and extends our earlier analysis of small-mammal habitat selection in the Serengeti ecosystem (Shilereyo et al., 2021) to encompass patterns and correlates of variation in small-mammal community characteristics across three land uses, five habitat types, and two seasons. The objectives of our analyses are 2-fold. First, we quantify small-mammal abundance, species richness, diversity, and evenness in five habitat types distributed across the three land uses. Second, we characterize seasonal variation in small-mammal abundance, species richness, diversity, and evenness across the five habitat types and three land uses. We do this by testing predictions of four hypotheses. We anticipate that small-mammal relative abundance, species richness, and diversity should be highest inside the protected park, intermediate in the pastoral land, and lowest in the most disturbed agricultural land (Hypothesis, H1). This prediction contradicts findings of some earlier studies that have reported higher small-mammal species abundance outside and higher species richness inside protected areas and pastoralism to be more compatible with wildlife conservation than agriculture (Salvatori et al., 2001; Niamir-Fuller et al., 2012; Magige, 2016). However, it accords with findings of several other studies of the influence of land use on the abundance and diversity of small mammals that have produced mixed results, ranging from negative, positive, to neutral (Magige and Senzota, 2006; Shilereyo et al., 2021). Because protection, such as in parks, reduces human footprints and maintains high habitat heterogeneity in protected areas, protection should sustain greater diversity of food and refuges and result in higher small-mammal relative abundance and diversity, most especially within protected areas. Moreover, the absence of a hard boundary (e.g., fences) between the protected and human-dominated areas can promote habitat heterogeneity across the human–wildlife interface (Magige and Senzota, 2006) with a potentially higher small-mammal relative abundance and diversity.
Furthermore, because small mammals breed seasonally (Catzeflis et al., 2019; Shilereyo et al., 2020; Welegerima et al., 2020), with birth peaks in the wet season when food quality and quantity also peak, the abundance of most species should be higher in the dry than the wet season due to juvenile recruitment (H2). For the same reason, small-mammal species richness and diversity should be higher in the dry than the wet season (H3). Nevertheless, small-mammal species should respond contrastingly to human disturbance, such that habitat generalist species should be more abundant than habitat specialist species in the more disturbed areas (Ralaizafisoloarivony et al., 2014). Thus, habitat generalists should be more abundant than specialists in the disturbed pastoral and agricultural lands but not in the protected park (H4).
Data were collected from the Greater Serengeti ecosystem in northern Tanzania, East Africa. We focused on the northeastern Serengeti ecosystem, including the Serengeti National Park (2°20′ S, 34°50′ E) and two adjacent administrative districts, namely, the Serengeti (2°15′ S, 34°68′ E) and Ngorongoro (3°24′ S, 35°48′ E). The park protects 14,750 km2 of tropical savanna ecosystem (Sinclair, 2008) and encompasses woodlands and open grasslands besides other more restricted habitat types (Byrom et al., 2014), with farming and livestock herding practiced around the ecosystem. The study covered three main land uses (the protected park, pastoral land, and agricultural land) and five habitat types (riverine forest, woodland, shrubland, grassland, and cropland) in the northern part of the Serengeti ecosystem located along the Mto Wa Mbu-Musoma road. This area was selected because it contains contrasting land use types, including agricultural lands (southwest), pastoral and limited agricultural lands in the southeast and the Serengeti National Park situated between these two land use types (Figure 1).
In the agricultural land in the southwest of the Serengeti ecosystem, the residents are mostly subsistence and cash crop farmers (Kavana et al., 2017) but some also engage in limited livestock keeping. In the pastoral land, the residents engage mostly in pastoral activities and limited crop production. In the cropland habitats, the land is prepared and planted during the wet season and thus is mostly covered with immature crops and bare understory due to the local weeding practices. But in the dry season, the land is covered by mature crops before harvest or maize cobs and stalks after harvest. However, the cropping system in the pastoral land and agricultural land differs because farmers mainly use oxen to prepare the land for cropping in the agricultural land but the hand hoe in the pastoral land (Monica Shilereyo, Pers. Observation, 2018). Also, the fallow period in the pastoral land is longer than that in the agricultural land (for more details, see Shilereyo et al., 2020). To enable a comparison between cropland habitats in the pastoral land and agricultural land, therefore, all trappings in the croplands in the dry season were done postharvest. Crop fields with maize (Zea mays) and beans (Phaseolus spp.), sometimes mixed with sweet potatoes (Ipomea batatas), were selected for trapping because both crops are commonly cultivated in both pastoral and agricultural land uses.
The climate in the Serengeti ecosystem is warm and semiarid, with mean monthly temperatures varying between 15°C and 25°C (Magige, 2016). The rainy season is bimodal with the short rains spanning November–December and the long rains covering March–May (Norton-Griffiths et al., 1975). January and February often tend to be dry. Rainfall increases from east to west toward Lake Victoria, south to north, and southeast to northwest. Rainfall increases along a gradient from 700 mm/year on the dry southeastern plains to 1,050 mm/year on the wet northwestern region of the ecosystem (Norton-Griffiths et al., 1975; Mukhopadhyay et al., 2019).
The data collection methods are described in greater detail by Shilereyo et al. (2020); Shilereyo et al. (2021), so we provide only a brief synopsis here. To establish how land use and habitat influence small-mammal abundance, species diversity, richness, evenness, and composition and rainfall seasonality modify these influences, we trapped small mammals in different habitats in areas with different land uses in and around the Serengeti National Park in the wet and dry seasons. This provided a rare opportunity to evaluate the separate effects of land use and habitat on small-mammal abundance, species diversity, and composition, an evaluation that greatly improves upon earlier studies that have sometimes confounded the two factors or merely considered only one of them.
Initially, we had planned to sample all of the 15 land use (n = 3) and habitat (n = 5) combinations, but several factors made this impractical, rendering the study design, unfortunately, incomplete (n = 10 land use and habitat combinations). Thus, there was no cropland in the park because farming is prohibited there. Moreover, the riverine forest was difficult to find in the agricultural land and pastoral lands. Also, we were denied access to Ololosokwan, a pastoralist village with historical land use conflict with the Tanzania National Parks, and so could not sample the two most typical habitats for pastoralism, the wooded grassland and grassland habitats, in the pastoral land. Replication of the factorial design for the different land uses and habitats was also not feasible because of the huge effort involved in the fieldwork, the extensive spatial coverage, and time, logistical, and budgetary constraints. The incomplete factorial design and lack of replication, unfortunately, complicated statistical analyses and inference on the effects of land use and habitat. Consequently, we explicitly take these caveats and drawbacks into account in discussing the results. Future work should endeavor to surmount these limitations by ensuring completeness and adequate spatial and temporal replication of the factorial study design.
We initially selected four habitats from each land use type for a total of 12 habitats. However, only the park and agricultural land each had four habitats, whereas the pastoral land had only two habitats, resulting in a total of 10 habitats for all of the three land uses. The 10 habitats belonged to five habitat types. A trapping plot measuring 100 × 100 m (1 ha) was selected in each of the 10 habitats. The four habitat types in the park were wooded grassland, shrubland, grassland, and riverine forest, whereas the four habitat types in the agricultural land were wooded grassland, shrubland, grassland, and cropland. The two habitat types in the pastoral land comprised shrubland and cropland. We used a total of 141 live traps (100 Sherman traps, 30 wire mesh traps, and 11 bucket pitfall traps). Trapping was done twice a year, in the wet (April–May) and dry (August–September) seasons, for two consecutive years (2017 and 2018). In 2017, trapping was carried out from 18 April to 30 May in the wet season and from 5 August to 25 September in the dry season. But in 2018, we trapped from 10 April to 28 May in the wet season and 5 August to 20 September in the dry season. Trapping started in the pastoral land (eastern part of the ecosystem) followed by the park and the agricultural land (western part of the ecosystem) because the eastern part of the ecosystem receives relatively lower rainfall than the western part and so dries earlier. The same sequence was followed throughout except in the wet season of 2018 when logistical difficulties forced us to set traps in the park after the agricultural land.
Pitfall lines were set to capture mostly shrews and trap lines to capture rodents. A pitfall line consisting of 11 buckets spaced 5 m apart was placed in each plot. Pitfall traps were buried into the ground so that the top of the bucket was at the ground level. Each of the 11 buckets per plot was 26 cm deep and had a 20-L capacity and upper and lower diameters of 30 cm and 26 cm, respectively. The bottom of each bucket was pierced with small holes to allow water drainage. Each pitfall line had a 50-cm-high black plastic drift fence running over the center of each bucket. These passive and non-baited traps capture animals moving on the habitat floor that encounter the drift fence and follow it until they fall into a bucket, mainly Crocidura and Mus species. The pitfall lines were generally set along straight trails; however, rocks and logs occasionally forced deviations. This technique has been used with success in other small mammal surveys (Stanley et al., 2011). Sherman traps (23 × 9.5 × 8 cm) were arranged along the lines, with a total of 100 traps. They were arranged on a grid of 10 × 10 traps and spaced 10 m apart. To maximize capture and the variety of small mammals caught, 30 wire mesh traps “Mgono” were placed in-between the Sherman trap lines. Five wire mesh traps were placed 20 m apart from each other. The precise layout of all of the 141 traps on a trapping grid in each plot is depicted diagrammatically in Figure 2 in Shilereyo et al. (2021). These wire mesh traps are widely used in Tanzania by local hunters and are funnel-shaped multi-capture traps made of thin wire.
Traps stayed in each of the 10 habitats for five consecutive days before being taken to the next habitat for the same duration. Bait for both the Sherman and “Mgono” traps consisted of freshly fried coconut coated with peanut butter and mixed with Lake Victoria sardines (Rastrineobola argentea). Traps were checked and rebaited twice daily, early in the morning and evening. We identified trapped animals to the genus or species using the recorded morphometric (external shape and dimensions) measurements (body length, tail, ear, and feet) and field guides (Kingdon, 2015). In addition, we recorded distinguishing features such as sex, age, and reproductive status to facilitate individual identification. We marked trapped animals by toe-clipping and released them at the points of capture to avoid duplicate counts of individuals on subsequent sampling days.
Because of variation in the total number of habitat types across the three land uses, we use “trap nights,” one trap in operation for a 24-h period (one full day and night), to quantify sampling effort. We refer to the success rate of capture as trap success, calculated by dividing the total number of all first-time captured individuals by the total number of trap nights and multiplying by 100. Trap success is a good measure of spatial and temporal variation in relative abundance (Cavia et al., 2012). We therefore use trap success as a measure of relative abundance. Relative abundance does not account for possibly different detection probabilities across sampling grids or different small-mammal species. So, we tried to minimize any potential differences in detection probabilities by using a fixed sampling season, number and type of traps, number of visits per day, and time of visit in a day (morning and evening).
Statistical analyses aimed to determine how small-mammal relative abundance, species richness, diversity, and evenness vary among the three land uses, five habitat types, and two seasons and their interactions. We used a Poisson regression model with a log link and the logarithm of the total capture effort per land use as the offset to adjust for varying effort among land uses and to model variation in small-mammal relative abundance and species richness between land use, habitat type, and season and all of their two-way interactions. We used an exponential regression model with a log link and the logarithm of the total capture effort per land use as the offset to model similar variations in species diversity (hill numbers) calculated using the iNEXT R Package (Hsieh et al., 2016). Hill numbers were chosen because they have several advantages over other diversity indices and were calculated according to Chao et al. (2014) and Hsieh et al. (2016). To ascertain whether sampling effort was sufficient to represent species richness within each land use, we used sample coverage graphs. A sample coverage curve was used because it takes into consideration standardization of samples in comparing species diversity across areas with different samples or sampling efforts (Chai and Wang, 2016). Finally, we used a gamma regression model with a reciprocal link function and the logarithm of the total capture effort per land use as the offset to model variation in species evenness across the three land uses and five habitat types without considering their interaction. Statistical significance was assessed at α = 0.05 unless otherwise stated. To determine the similarity in species composition between pairs of land use types, we used the Bray–Curtis similarity index. This index quantifies the compositional similarity between communities at two sites based on count data. The index ranges from 1 for two communities that share all their species to 0 for those that share none (Bray and Curtis, 1957). The Bray–Curtis index uses abundance data but is a modified version of the Sørensen index that uses presence/absence data (Chao et al., 2005).
The aggregate sampling effort for all of the three land uses was 28,200 trap nights, corresponding to a total effort of 2,820 trap nights for each of the 10 habitats. A total of 612 individuals (excluding 117 recaptures) belonging to 19 species and two orders (Rodentia and Eulipotyphla) of small mammals were captured. Of these, 86% (n = 528) were rodents, whereas 14% (n = 84) were shrews. Of the 117 recaptured individuals, 81.2% belonged to only one of the small-mammal species, i.e., Arvicathis niloticus. The full trapping data set is provided in S1 Data in the Supplementary Materials.
Relative abundance (captures/100 trap nights) varied with land use and was highest for the pastoral land (4.91, = 277 individual small mammals), intermediate for the park (2.1, = 237), and lowest for the agricultural land (0.86, n = 98). Both A. niloticus and M. natalensis had the highest trap success most especially in the pastoral land. By contrast, Dendromus murinus and Graphiurus murinus had higher trap success in the park. Also, most of the species with the lowest trap success were trapped in the park (Table 1).
Table 1 Relative abundance (100 × number of first-time captures/numbers of trap nights) for each small-mammal species recorded in each of the three land uses in the Serengeti ecosystem during the wet and dry seasons of 2017 and 2018.
The mean relative abundance differed between the land uses. The relative abundance was higher in the pastoral land than in the park or in the agricultural land. Specifically, relative abundance in the pastoral land was approximately six times higher than that in the agricultural land, two times higher in the park than that in the agricultural land, and two times higher in the pastoral land than that in the park (Table 2, Figure 2), contrary to the prediction that abundance should be highest in the park (H1). Even though it had the highest relative abundance, the pastoral land was species-poor and dominated by a few most common species. The relative abundances for these most common species were highest for the pastoral land and lowest for the agricultural land, with minor exceptions. Notably, relative abundances of M. natalensis and A. niloticus were highest for both the pastoral and agricultural lands, whereas those for Crocidura spp., Dendromus melanotis, and G. murinus were highest for the park. In addition, a few species, each represented by less than 10 first-time captured individuals, were mostly restricted to within the park confines, resulting in greater diversity there. These rare species were Grammomys spp., Lemniscomys striatus, Praomys jacksoni, Saccostomus spp., and Pelomys spp. (Table 1, Supplementary Table S1).
Table 2 Tests of fixed effects of land use, habitat type, and season and their two-way interactions on variation in the relative abundance of small mammals in the Serengeti ecosystem during 2017 and 2018 based on the Poisson regression model.
Figure 2 Relative abundance of small mammals in each of the three land uses in the Serengeti ecosystem during 2017 and 2018.
Relative abundance was comparable between the five habitat types, but several differences were apparent (Table 2). Grassland had the lowest relative abundance across all of the three land uses. Although not significant, the relative abundance in shrubland appeared higher in the pastoral land than that in either the park or the agricultural land (Figure 3). For cropland, relative abundance was apparently higher in the pastoral than in the agricultural land. For the wooded grassland, however, relative abundance tripled in the park relative to that in the agricultural land. Also, the riverine forest in the park had relatively high relative abundance. Although shrubland and cropland in the pastoral land had the highest relative abundances in the ecosystem, they had relatively fewer species, with only two dominating abundance, such that A. niloticus contributed 78% to relative abundance in the shrubland whereas M. natalensis contributed 46% in the cropland, partially supporting H3. By contrast, shrubland in the park had a variety of species (Supplementary Table S1).
Figure 3 Relative abundance of small mammals in each of five habitat types in the three land uses in the Serengeti ecosystem during 2017 and 2018.
Relative abundance also varied seasonally and interannually (Figure 4, Supplementary Table S2). The mean relative abundance was higher in the dry than in the wet season in the pastoral land (97.5 for the dry vs. 41.0 for the wet season) and agricultural land (15.5 for the dry vs. 9.0 for the wet season) but was higher in the wet season in the park (132.0 for the wet vs. 105.0 for the dry season), partially supporting H2 (Table 2, Supplementary Table S2). In addition, across all species, relative abundance was lower in 2017 than 2018. The seasonal variation in relative abundance persisted even when each of the 2 years was considered separately such that it was higher in the dry season in 2017 and 2018. As well, the relative abundance of individual species also varied seasonally, but with different species showing contrasting patterns. Specifically, A. niloticus was more abundant in the dry season, while Crocidura spp. and G. murinus were more abundant in the wet season. All of the other species (Saccostomus spp., O. angoniensis, S. parvus, Pelomys sp., Zelotomys sp., and P. jacksoni) had low relative abundances and were mostly captured in the dry season (Figure 4).
Figure 4 Relative abundance (percentage) of all of the small mammals captured in each season during 2017–2018 in the Serengeti ecosystem. Percentages are used because both seasons had the same total trap nights of effort.
Species richness varied across the three land uses but was comparable between habitat types and seasons, supporting H1 but not H3 (Supplementary Tables S3A, B). Species richness was higher in the park than that in either the pastoral or agricultural land, both of which had comparable richness (Tables 3, 4; Supplementary Table S4). Although the overall mean species richness did not vary seasonally, it apparently varied seasonally in the grassland, which had nine species in the dry season but only one species in the wet season (Supplementary Table S3A).
Table 3 The variation in species richness with land use and habitat type in the Serengeti ecosystem during 2017 and 2018.
Table 4 Tests of fixed effects of land use, habitat type, and season on variation in small-mammal species richness in the Serengeti ecosystem during 2017 and 2018 based on the Poisson regression model.
Although not statistically significant, species richness apparently varied between habitat types. Notably, it was higher and comparable in the wooded grassland and shrubland than that in the grassland, forest, and cropland, the latter three of which had similar richness. Furthermore, richness varied between habitat types in the same land use (Figure 5). Most notably, it was lower in the shrubland than that in the wooded grassland, riverine forest, or grassland habitat in the park. For the agricultural land, grassland had seemingly lower richness than all of the other three habitat types.
Figure 5 Small-mammal species richness in the five habitat types in the three land uses in the Serengeti ecosystem during 2017 and 2018.
Species diversity (Hill number) varied with land use, habitat type, and season and with the interactions between land use and season as well as between habitat type and season (Table 5, Figures 6–8). The overall species diversity in the ecosystem was 2.25. Species diversity was almost twice as high in both the park and agricultural land as in the pastoral land. After adjusting for effort, diversity was higher in the park (1.45) than that in the pastoral land (1.13) or agricultural land (1.14), both of which had comparable diversities, supporting H1. Sample coverage curves show that sampling effort was virtually complete for all of the three land use types. This is because the trend from the base coverage to 1.0 indicates that any further increase in the sampling effort would not yield any significant further improvement in the sample coverages (Figure 6). However, the final slope of the curve for the agricultural land was seemingly lower than those for the other two land use types, suggesting that increasing the sampling effort in the agricultural land use may potentially yield a few more species. The 95% bootstrap confidence bands suggest that, generally, species diversity was highest in the park, middling in the agricultural land, and the lowest in the pastoral land. More precisely, species diversity was similar between the park and the agricultural land at lower coverages but became significantly higher in the park than that in the agricultural land at coverages exceeding 0.8 (Figure 6).
Table 5 Tests of fixed effects of land use, habitat type, and season and their interactions on variation in small-mammal species diversity in the Serengeti ecosystem during 2017 and 2018 based on the exponential regression model.
Figure 6 Sample coverage-based rarefaction (solid curves) and interpolation (dashed lines) curves for the three land use types. Shaded bands are the 95% bootstrap confidence bands based on 1,000 bootstrap replications.
Figure 7 Species diversity index (Hill number ± 1 standard error) for small mammals in the three land uses in the Serengeti ecosystem during 2017 and 2018.
Figure 8 Small-mammal species diversity in each of the five habitat types across the three land use types in the Serengeti ecosystem in the wet and dry seasons of 2017 and 2018.
Across all of the five habitat types, and after adjusting for effort, mean species diversity was highest for the forest followed, in decreasing order, by cropland, wooded grassland, shrubland, and grassland (Supplementary Table S5). Diversity also varied seasonally and was higher in the dry season (Table 5, Supplementary Table S5), consistent with H3. Across land uses, diversity was higher in the dry season in both the park and the agricultural land but in the wet season in the pastoral land. Lastly, across habitat types, diversity was mostly higher in the dry season, but in the grassland and forest habitat types, it was higher in the wet season. Diversity was even higher when land use interacted with habitat type (Table 5, Supplementary Table S5).
Moreover, species evenness varied among the three land use types but was comparable between habitat types. It was higher in the park and agricultural land (0.65) than that in the pastoral land (0.4), indicating greater dominance of a few species in the pastoral land (Table 6, Supplementary Table S6). Bray–Curtis coefficients of similarity in composition among the three land uses divided the study species into two major groups (Figure 9). The first group, representing the pastoral land, was more discordant from, and shared only 22% of the species with, the park and agricultural land. The two other groups, representing the park and agricultural land, had 38% similarity in species composition. Although similarity in species composition across the land uses was less than 50%, the park and agricultural land shared more species than they did with the pastoral land.
Table 6 Tests of fixed effects of land use and habitat type on variation in small-mammal species evenness in the Serengeti ecosystem during 2017 and 2018 based on the gamma regression model. Table legend: NDF and DDF are the numerator and denominator degrees of freedom, respectively.
Figure 9 Similarity clusters based on the Bray–Curtis similarity index (Single Average Linkage) for the three land uses in the Serengeti ecosystem during 2017 and 2018.
Relative abundance varied evidently across the three land uses and was highest in the pastoral land, middling in the park, and lowest in the agricultural land, deviating from the initial expectation that it should be highest in the park, intermediate in the pastoral land, and least in the agricultural land. This deviation is primarily attributable to the dominance in the pastoral land of the two most common species: A. niloticus and M. natalensis. These two species are generalists, able to produce many young, attain high densities in relatively short time frames, colonize, and adapt to a wide range of habitat types (Mulungu et al., 2011). The numerical dominance of these two species suggests that human activities have likely modified the habitats in the pastoral land, rendering them suitable for only a few generalists (Byrom et al., 2015; Chidodo et al., 2020). Human activities in pastoral lands likely reduce structural habitat complexity and heterogeneity both of which are crucial in shaping small-mammal community assemblages, thus favoring generalist species able to use a wider range of habitats at the expense of the other sympatric small-mammal species (Duplantier and Sene, 2000; Mulungu et al., 2011) and can rapidly shift their habitat associations when faced with temporarily shifting food resources (Seamon and Adler, 1996). Alternatively, these species are more adaptable and able to inhabit disturbed vegetation due to a superior ability to detect predators and balance the trade-off between food availability and predation (Wells et al., 2021).
In contrast, the abundance of specialist species adapted to life in narrowly defined habitats is more sensitive to fluctuations in their preferred food items and suitable shelter due to human land use (Martínez et al., 2014). The lower abundance of the specialist species in the pastoral land is thus consistent with their greater sensitivity to human land use impacts. However, further research would be needed to quantitatively assess how land use affects the availability of their preferred food items and shelter and how these influences affect the abundance of both the specialist and generalist species.
Relative abundance also varied across habitat types and was highest in the shrubland in the pastoral land. Notably, A. niloticus (78%) was the most abundant species in the shrubland in the pastoral land where sustained livestock grazing may have increased woody cover at the expense of herbaceous plant cover (Ofori et al., 2014). This accords with the observation that heavy livestock grazing can reduce plant species diversity and homogenize natural habitats (Cao et al., 2016). By increasing shrub cover and reducing primary productivity of the aboveground biomass, livestock grazing can negatively affect plant diversity and food availability for small mammals (Goheen et al., 2018). Although shrubland in the park also had high relative abundance, it had less small-mammal species dominance and possessed relatively many habitat specialists, indicating greater habitat heterogeneity in the park, as also suggested by Tabeni and Ojeda (2005). On the other hand, small mammals were also abundant in the cropland habitat in the pastoral land due primarily to the numerical dominance of M. natalensis in the postharvest period (dry season) when food and cover are still relatively plentiful. This species is common in croplands due to its preference for grains, generalist foraging behavior, and high food availability in this habitat type after harvest (Caro, 2001; Mulungu et al., 2011; Magige, 2016).
In contrast, cropland had lower relative abundance in the agricultural land than the pastoral land likely due to differences in the cropping systems used in the two land uses. In the pastoral land, farms are fenced to prevent livestock from raiding crops (Monica Shilereyo, Pers. Obs, 2018). The vegetation fringing the fences provides habitats for small mammals. In addition, during harvests, crop farmers in the pastoral land often leave some maize stocks and cobs on the farm for livestock. Also, after harvest, farmers in the pastoral land take a relatively longer time before preparing land by hand hoes for the next planting season. Thus, the land remains relatively intact for some months. By contrast, fencing farms is not common in the agricultural land, and it typically takes less than a month to prepare land by oxen and replant because most of the farmers cultivate crops for cash income (Monica Shilereyo, Pers. Obs, 2018). Thus, the cropping system used in the cropland habitat in the pastoral land likely contributed to a relatively stable supply and availability of high-quality food and shelter for the small mammals after harvest. Preparing land and replanting soon after harvesting, as done in the cropland in the agricultural land, remove vegetation cover, destroy mounds and nesting sites, and hence reduce food availability and shelter for small mammals (Hieronimo et al., 2014). This likely exposes small mammals to greater food shortages and higher predation risk, forcing them to seek safer habitat types elsewhere. Changes in the quality and quantity of resources associated with cultivation can thus greatly influence the relative abundance of small mammals. Specifically, the land preparation methods used in the cropland in the pastoral land may favor M. natalensis species because it disturbs the habitat much less than oxen or a tractor does (Massawe et al., 2006).
The higher relative abundance of small mammals in the riverine forest and in the wooded grassland in the park than that in the latter habitat in the agricultural land may reflect greater habitat heterogeneity in the park because of less human activities (Byrom et al., 2015). Specifically, the higher abundance of D. melanotis in the wooded grassland and shrubland in the park than that in the agricultural land could be due to advanced locomotor adaptations and mode of foraging of D. melanotis. This species is an agile climber that prefers tall vegetation (Skinner and Chimimba, 2005) prevalent in the park. Although it occurs in both relatively pristine and human-dominated habitats, disturbance such as from agriculture may cause its temporal migration (Child and Monadjem, 2016). Thus, the park likely provides habitats safer from human disturbance than the human-dominated lands besides providing habitats with tall vegetation favored by this species. Nevertheless, the lower relative abundance in the grassland in both land uses is likely due to disturbance by large herbivores that may cause small mammals to migrate. This is because ungulates may temporarily avoid woody habitats likely due to inadequate forage because woody plants typically displace herbaceous plants important for ungulates (Anadón et al., 2013; Espunyes et al., 2019). Alternatively, fire may easily penetrate grassland than woody habitats, resulting in lower relative abundance (Salvatori et al., 2001).
The higher relative abundance in the dry season in both the pastoral and agricultural lands is partially consistent with H2. Seasonality alters vegetation cover and food availability and thus small mammal abundance (Mulungu et al., 2011; Byrom et al., 2015). The higher abundance in the dry season indicates elevated food availability due to high rainfall in the preceding wet season. This reinforces the view that food and shelter rank among the key factors that determine small-mammal abundance (Crespin et al., 2008). Surprisingly, the park had higher relative abundance in the wet season. This may be due to the higher relative abundance of the Crocidura spp., which is strongly influenced by moisture availability. Although there is no direct evidence for it, the preference of this species for moist areas suggests that it may dig burrows when foraging or to avoid predators (Dickman, 1995). The increase in invertebrate abundance during the wet season likely explains the higher abundance of this species in the moist wet season (McCay and Storm, 1997; Ivanter and Makarov, 2002). However, the higher relative abundances of G. murinus and Crocidura spp. in the wet season contradict findings of two previous studies that found higher abundances in the dry season for both species in the Serengeti (Timbuka and Kabigumila, 2006; Lamani, 2014). This implies substantial interannual variation in abundance likely linked to a similar underlying variation in rainfall and hence in food availability and quality.
In aggregate, these results support the well-known notion that human activities, such as livestock grazing and agriculture, modify natural habitats. This is reinforced by the higher abundance of A. niloticus in the shrubland and M. natalensis in the cropland in the pastoral land. Both species are habitat generalists able to vary the size of their home ranges depending on seasonal food availability and to persist in disturbed areas (Odhiambo et al., 2008; Mulungu et al., 2011). This conforms with the general view that human-dominated habitats should harbor many generalist small-mammal species (Byrom et al., 2015). Thus, by creating habitats that favor generalists at the expense of specialist species, human activities modify ecosystem function and suitability for small-mammal communities.
The robustness of the general patterns of relative abundance may depend upon the stability of the landscape patterns over space and time. Our study covered only 10 habitats (out of a possible total of 15) and a period of only 2 years, but more spatial and temporal replication may be necessary to more comprehensively assess distributional patterns of small-mammal communities because they can exhibit marked spatial gradients and interannual fluctuations. For example, M. natalensis, a dominant species in agricultural landscapes, can show population cycles with some years showing massive population outbreaks (Leirs et al., 1997; Stenseth et al., 2001). As a result, if fieldwork is conducted during an outbreak year for M. natalensis, the broad patterns may be substantially different from those for a typical year. Furthermore, a complete factorial design with many spatial replicates would be required to clearly disentangle habitat effects from land use effects as well as from effects of habitat homogenization.
As hypothesized, small-mammal species richness and diversity were higher inside the park than those in either the pastoral or agricultural land, consistent with H1 and demonstrating that protection in the park is crucial in safeguarding wildlife (Wasiolka and Blaum, 2011; Watson et al., 2016). This is further reinforced by the observation that most of the species that had low relative abundance occurred in the park, indicating speciality. Furthermore, the park is the least modified by human activities and likely has high vegetation heterogeneity and intactness, both crucial to supporting a variety of small-mammal species. Habitat heterogeneity is one of the most important factors influencing small-mammal richness and diversity (Shilereyo et al., 2021). These findings concur with those of Magige and Senzota (2006) who also recorded the highest small-mammal diversity in the protected park. They are also consistent with the general notion that higher species diversity is associated with greater habitat diversity. However, the apparent lack of similarity in species composition between the land use types should be interpreted with caution because the park and the agricultural land have three habitat types in common (wooded grassland, shrubland, and grassland), whereas in the pastoral land, only one of these was included (shrubland) not because wooded grassland and grassland did not exist in the pastoral land but because access was not granted to these habitats. It follows that, despite accounting for variation in sampling effort, using a fixed sampling season, number and type of traps, number of visits per day, and time of visit in a day, the lack of similarity may very well be, or partly be, the result of this design bias rather than of true ecological difference. This can only be conclusively resolved by carrying out a similar study with a fully factorial design and sufficient spatial and temporal replication.
Similarly, the higher species diversity for the park reflects the importance of protection in maintaining high habitat and species diversity in ecosystems. High habitat diversity is essential for high species diversity because the presence of habitat specialists is conditional on the presence of their favored habitat types. Thus, for example, the riverine forest harbored mainly G. murinus and Grammomys spp., both of which prefer trees and intact forest cover for nesting. The selection of the riverine forest by G. murinus has also been noted previously (Madikiza et al., 2010) and is indicative of habitat specificity. Higher species diversity in the wooded grassland and cropland is likely due to more microhabitats in the wooded grassland and high food availability in the cropland, which attracts diverse species (Magige, 2016).
Variation in species diversity between the habitat types and land uses was evident, as species richness and diversity were higher in the wooded grassland and grassland in the park than those in the same habitat types in either the pastoral or agricultural land. This was attributed to the presence of relatively more habitat specialists in the shrubland in the park than that in the pastoral land, which supported a few dominant species, resulting in low species richness and diversity. The impact of livestock grazing in the shrubland in the pastoral land was manifested in the lower diversity there than that in the other habitat types. Moreover, the relatively lower species evenness (40%) in the pastoral land reaffirms the role of livestock grazing as one of the anthropogenic activities that alters vegetation structure and promotes generalist species over habitat specialists. Such a higher abundance of certain small-mammal species in human-dominated landscapes, such as the pastoral land, can elevate the risk of infection with zoonotic diseases and possibly human–small mammal conflicts (Young et al., 2017).
So, how does livestock grazing reduce small-mammal species diversity? One plausible mechanism is that grazing increases shrub cover and patches and hence nesting and refuge sites for small mammals but reduces vegetation diversity and ground cover (Blaum et al., 2007). Thus, continuous livestock grazing decreases small-mammal species diversity by reducing their food diversity and habitat quality and increasing competition with larger herbivores and predation risk (Cao et al., 2016). Consequently, human activities, such as livestock grazing, are detrimental to ecosystems, as they reduce small-mammal species diversity, yet small mammals play central roles in food webs and other ecosystem services.
Moreover, species diversity was higher in the dry season likely because of seasonal variation in weather and activity patterns of the small mammals. Small mammals move more in the dry season and may therefore have a higher risk of being trapped. Also, more juveniles are recruited into the population in the dry season (Shilereyo et al., 2020). The higher species diversity in the park and agricultural land than that in the pastoral land in the dry season (when forage is scarce) is thus likely due to greater livestock densities and grazing activities in the pastoral land in the dry season. However, higher species diversity in the wooded grassland, shrubland, and cropland than in the grassland might be due to the presence of more cover in the dry season (Kawamura et al., 2019). The higher diversity in the forest habitat in the wet season is probably due to the presence of many species that seek moisture and cool temperatures. However, the fact that species richness did not vary between seasons suggests that the species are common to the habitat types.
Definitive explanations for the observed patterns would require well-designed observational field studies or experiments to disentangle the mechanisms through which land use and its changes and seasonality affect availability of food and shelter, habitat suitability, competition, and predation and how alterations to these factors, in turn, influence small-mammal abundance, species diversity, and composition. Based on our observations and interpretations of our results, such studies should test predictions of the following testable hypotheses.
(1) Human activities will modify habitats in the pastoral land, making them suitable for only a few generalists at the expense of specialist species. (2) Generalists will more rapidly shift their habitat associations than specialists when faced with temporarily shifting food resources because generalists are better adapted to using altered habitats (Andren, 1999). (3) The abundance of specialist species will be more sensitive to human land use because it more strongly reduces their preferred food items and suitable shelter and hence their distribution. (4) The relative abundance of small mammals will be lower in pastoral lands because livestock grazing reduces plant species diversity, homogenizes natural habitats, increases shrub cover, and reduces primary productivity of the aboveground biomass, thus negatively affecting plant diversity and food availability for small mammals. (5) Relative abundance of small mammals dominated by a few habitat specialists will be higher in the park because of its higher habitat heterogeneity. (6) Small mammals will be more abundant in the cropland habitat in the pastoral land due to the numerical dominance of a few generalist species that prefer grains in the postharvest period (dry season) when food and cover are more plentiful.
(7) The cropping system used in cultivation will affect the relative abundance of small mammals through its influence on variation in the quality and quantity of critical resources. (7a) Relative abundance will be lower in cropland areas using mechanized tools for tillage (e.g., tractor and oxen) than that in areas using less mechanized tools (e.g., hand hoe). (7b) Relative abundance will be lower in the cropland in the agricultural land than that in the pastoral land because of differences in the typical cropping system used in each. (7c) Relative abundance will be higher on the cropland in the pastoral land because the vegetation fringing fenced farms in the pastoral land will provide better habitats for small mammals than unfenced farms in the cropland in the agricultural land. (7d) Relative abundance will also be higher in the cropland in the pastoral land than that in the agricultural land because crop farmers in the pastoral land leave some maize stalks and cobs on the farm for livestock after harvest, whereas farmers in the agricultural land typically do not. (7e) Relative abundance will be higher in the cropland in the pastoral land than that in the agricultural land because farmers in the pastoral land take longer to till the land and replant after harvest than farmers in the agricultural land. (7f) Relative abundance will be higher in the cropland in the pastoral land than that in the agricultural land because the cropping system used in the cropland in the pastoral land yields a more stable supply and availability of high-quality food and shelter for the small mammals after harvest than those in the agricultural land. (7g) Relative abundance will be higher in the cropland in the pastoral land than that in the agricultural land because preparing land and replanting soon after harvest in the cropland in the agricultural land reduces shelter and increases predation risk for small mammals, forcing them to seek safer habitats elsewhere.
(8a) Relative abundance of small mammals will be higher in the riverine forest and in the wooded grassland in the park than that in the latter habitat in the agricultural land because of greater habitat heterogeneity in the park due to less human activities. (8b) Disturbance, such as from agriculture, will cause temporary migration of some small-mammal species to seek less disturbed habitats they favor elsewhere. (8c) Relative abundance will be lower in the grassland than that in the woody habitats because fires penetrate grassland more readily than woody habitats and small mammals may migrate due to disturbance by large herbivores in the grassland habitat. (9a) Relative abundance will be higher in the dry season in both the pastoral and agricultural lands due to elevated food availability linked to high rainfall in the preceding wet season. (9b) The park will have a higher relative abundance of a few species strongly attracted to moisture availability in the moist wet season, which influences the availability of surface drinking water and food, such as invertebrates, and ease of digging burrows to avoid predators.
(10a) Small-mammal species richness and diversity will be higher in the park than those in either the pastoral or agricultural land and most species occurring in the park will have low relative abundance, indicating speciality. This is because the park is the least modified by human activities and has higher vegetation heterogeneity and intactness, crucial to supporting a higher small-mammal species diversity. (10b) Livestock grazing in the shrubland in the pastoral land will reduce species diversity by increasing shrub cover, nesting and refuge sites while simultaneously reducing vegetation diversity and ground cover for suitable refuges for small mammals. (10c) High habitat diversity will lead to higher species diversity because of more habitat types favored by habitat specialists. Consequently, species diversity will be higher in the wooded grassland with more microhabitats. (10d) Species richness and diversity will be higher in the wooded grassland and grassland in the park than those in the same habitat types in either the pastoral or agricultural land because more habitat specialists occur in the park than in the pastoral land, which supports only a few dominant species, resulting in low species richness and diversity. (10e) Continuous livestock grazing in the pastoral land will decrease small-mammal species diversity by reducing its food diversity and habitat quality and increasing exploitative competition with larger herbivores as well as predation risk.
(11) Species diversity will be higher in the dry season because small mammals move more at this time and therefore are more likely to be trapped (and probably also killed by predators due to higher encounter rates). (12) Species diversity will be higher in the park and agricultural land than that in the pastoral land in the dry season (when forage is scarce) because of higher livestock densities and more intense grazing in the pastoral land in the dry season. (13) Species diversity will be higher in the wooded grassland, shrubland, and cropland than that in the grassland because the former three habitats have more cover in the dry season. (14) Species diversity will be higher in the forest habitat in the wet season because more species seek moisture and cool temperatures there at this time. (15) Species richness in the habitats should not vary between seasons because the same species are found in each habitat regardless of season.
The pastoral land had higher relative abundance of small mammals than either the park or agricultural land, but species richness and diversity were both highest in the park. Relative abundance and diversity both varied across seasons, with both the pastoral and agricultural lands having higher relative abundances in the dry season but the park having a higher relative abundance in the wet season. Species diversity was higher in the dry season in both the park and agricultural land but in the wet season in the pastoral land. These differences likely reflect seasonality in food availability, habitat selection, and activity patterns of the small mammals in the ecosystem. The pastoral land had a high relative abundance of a few generalists, mostly pest species, resulting in low species evenness and diversity. This suggests the role of reduced habitat heterogeneity that can increase the risk of zoonotic diseases and human–small mammal conflicts. Future studies should therefore quantitatively assess habitat heterogeneity at the plot level and use it as a predictor variable in models of small-mammal species abundance and diversity.
The higher species richness and diversity in the park than those in the agricultural or pastoral land imply a relatively higher habitat heterogeneity and suitability for sustaining high small-mammal species diversity. The agricultural land supported less abundant but more diverse small-mammal communities than the pastoral land probably due to greater food and shelter availability postharvest than those in the pastoral land. Thus, some human activities apparently exert deleterious effects on some small-mammal species by reducing the suitability of their habitats, species richness and diversity. These findings substantiate the importance of protection in parks in sustaining small-mammal species richness and diversity and of protected areas, generally, as baselines for assessing the impacts of human land use on small mammals. Overall, our findings suggest the importance of improving agricultural and pastoral practices in tropical regions to minimize their deleterious effects on small mammals, such as through agroforestry, fallowing, and rotational grazing.
The raw data supporting the conclusions of this article will be made available by the authors, without undue reservation.
The study design was approved by the Tanzania Wildlife Research Institute (TAWIRI) and the permit to conduct the research was obtained from the Tanzania Commission of Science and Technology (COSTECH) and Tanzania National Parks (TANAPA). All captured small mammals were handled according to the approved permit and released immediately at the point of capture after observation.
FM, JO, and ER have contributed equally to this work by designing the research, analyzing the data, and reviewing all versions of the article. MS conceived the project, collected the data, and wrote the first draft of the paper. All authors contributed to the article and approved the submitted version.
This project has received funding from the European Union’s Horizon 2020 research and innovation program under grant agreement No. 641918 through the AfricanBioServices Project. JO was additionally supported by the German Research Foundation (DFG, Grant # 257734638). The funders had no role in the study design, data collection and analysis, decision to publish, or preparation of the article.
We thank the Tanzania Commission of Science and Technology (COSTEC), Tanzania National Parks (TANAPA), and the Tanzania Wildlife Research Institute (TAWIRI) for permission to conduct this study. We also thank the District Executive Offices for Serengeti and Ngorongoro Districts for study permits for the village lands. We extend our appreciation to Mr. Raymond E. Okick of TAWIRI for his assistance with habitat and vegetation identification.
The authors declare that the research was conducted in the absence of any commercial or financial relationships that could be construed as a potential conflict of interest.
All claims expressed in this article are solely those of the authors and do not necessarily represent those of their affiliated organizations, or those of the publisher, the editors and the reviewers. Any product that may be evaluated in this article, or claim that may be made by its manufacturer, is not guaranteed or endorsed by the publisher.
The Supplementary Material for this article can be found online at: https://www.frontiersin.org/articles/10.3389/fcosc.2023.981424/full#supplementary-material
Supplementary Data Sheet 1 | All the species captured in each habitat type, land use and season in the Serengeti Ecosystem in 2017 and 2018. Also provided are the time of capture (morning or evening), total effort per land use type and for the entire study period.
Anadón J. D., Sala O. E., Turner B. L., Bennett E. M. (2013). Effect of woody-plant encroachment on livestock production in north and south America. Int. J. Biol. Sci. 111 (35), 12948–12953. doi: 10.1073/pnas.132058511
Andren H. (1999). Habitat fragmentation, the random sample hypothesis and critical thresholds. Oikos 84 (2), 306–308.
Anley M. A., Minale A. S., Haregeweyn N., Gashaw T. (2022). Assessing the impacts of land use/cover changes on ecosystem service values in rib watershed, upper blue Nile basin, Ethiopia. Trees Forests People 7, 1–10. doi: 10.1016/j.tfp.2022.100212
Barnosky A. D., Hadly E. A., Gonzalez P., Head J., Polly P. D., Lawing A. M., et al. (2017). Merging paleobiology with conservation biology to guide the future of terrestrial ecosystems. Science 355 (6325), eaah4787. doi: 10.1126/science.aah4787
Bergstrom B. J. (2013). Would East African savanna rodents inhibit woody encroachment? evidence from stable isotopes and microhistological analysis of faeces. J. Mammal. 94 (2), 436–447. doi: 10.1644/12-MAMM-A-146.1
Blaum N., Rossmanith E., Popp A., Jeltsch. F. (2007). Shrub encroachment affects mammalian carnivore abundance and species richness in semiarid rangelands. Acta Ecol. 1, 86–92. doi: 10.1016/j.actao.2006.10.004
Bray J. R., Curtis J. T. (1957). An ordination of the upland forest communities of southern Wisconsin. Ecol. Monogr. 27 (4), 325–349. doi: 10.2307/1942268
Butchart S. H., Walpole M., Collen B., Van Strien A., Scharlemann J. P., Almond R. E., et al. (2010). Global biodiversity: indicators of recent declines. Science 328 (5982), 1164–1168. doi: 10.1126/science.1187512
Byrom A. E., Craft M. E., Durant S. M., Nkwabi A. J., Metzger K., Hampson K., et al. (2014). Episodic outbreaks of small mammals influence predator community dynamics in an east African savanna ecosystem. Oikos 8, 1014–1024. doi: 10.1111/oik.00962
Byrom A. E., Nkwabi A., Metzger J. K., Mduma S. A., Forrester G. J., Ruscoe W. A., et al (2015). Anthropogenic stressors influence small mammal communities in tropical East African savanna at multiple spatial scales. J. Wildl. Res. 42 (2), 119–131. doi: 10.1071/WR14223
Cao C., Shuai L.-Y., Xin X.-P., Liu Z.-T., Song Y.-L., Zeng Z.-G. (2016). Effects of cattle grazing on small mammal communities in the hulunber meadow steppe. PeerJ 4, e2349. doi: 10.7717/peerj.2349
Caro T. (2001). Species richness and abundance of small mammals inside and outside an African national park. Conserv. Biol. 3, 251–257. doi: 10.1016/S0006-3207(00)00105-1
Catzeflis F. M., Lim B. K., Da Silva C. R. (2019). Litter size and seasonality in reproduction for guianan rodents and opossums. Stud. Neotropical Fauna Environ. 54 (1), 31–39. doi: 10.1080/01650521.2018.1528655
Cavia R., Cueto G. R., Suárez O. V. (2012). “Techniques to estimate abundance and monitoring rodent pests in urban environments,” in Integrated pest management and pest control-current and future tactics (Buenos, Aires: IntechOpen).
Cazalis V., Princé K., Mihoub J. B., Kelly J., Butchart S. H., Rodrigues A. S. (2020). Effectiveness of protected areas in conserving tropical forest birds. Nat. Commun. 11 (1), 4461. doi: 10.1038/s41467-020-18230-0
Chai Z., Wang D. (2016). A comparison of species composition and community assemblage of secondary forests between the birch and pine-oak belts in the mid-altitude zone of the qinling mountains, China. PeerJ 4, e1900. doi: 10.7717/peerj.1900
Chao A., Chazdon R. L., Colwell R. K., Shen T. J. (2005). A new statistical approach for assessing similarity of species composition with incidence and abundance data. Ecol. Letter 8 (2), 148–159. doi: 10.1111/j.1461-0248.2004.00707.x
Chao A., Gotelli N. J., Hsieh T. C., Sander E. L., Ma K. H., Colwell R. K., et al. (2014). Rarefaction and extrapolation with hill numbers: a framework for sampling and estimation in species diversity studies. Ecol. Monogr. 84 (1), 45–67. doi: 10.1890/13-0133.1
Chidodo S., Kilawe C. J., Mnyone L. L., Broecke B. V., Mulungu L. S. (2020). Factors affecting the composition of rodent assemblages in the north uluguru mountains, Tanzania. J. Vertebrate Biol. 69 (2), 20047. doi: 10.25225/jvb.20047
Crespin L., Papillon Y., Abdoulaye D., Granjon L., Sicard B. (2008). Annual flooding, survival and recruitment in a rodent population from the Niger river plain in Mali. J. Trop. Eco. 4, 375–386. doi: 10.1017/S0266467408005105
Dickman C. R. (1995). Diets and habitat preferences of three species of crocidurine shrews in arid southern Africa. J. Zool 3, 499–514. doi: 10.1111/j.1469-7998.1995.tb02777.x
Duplantier J. M., Sene M. (2000). Rodents as reservoir hosts in the transmission of Schistosoma mansoni in Richard-Toll, Senegal, West Africa. J. Helminthol. 129–135.
Du Toit J. T., Kock R., Deutsch J. (2012). Wild rangelands: conserving wildlife while maintaining livestock in semi-arid ecosystems (ZSL, UK: John Wiley & Sons).
Ellis E. C., Ramankutty N. (2008). Putting people in the map: anthropogenic biomes of the world. Front. Ecol. Environ. 8, 439–447. doi: 10.1890/070062
Espunyes J., Lurgi M., Büntgen U., Bartolomé J., Calleja J. A., Gálvez-Cerón A., et al. (2019). Different effects of alpine woody plant expansion on domestic and wild ungulates. Glob Chang Biol. 25, 1808–1819. doi: 10.1111/gcb.14587
Estes A. B., Kuemmerle T., Kushnir H., Radeloff V. C., Shugart H. H. (2012). Land-cover change and human population trends in the greater Serengeti ecosystem from 1984–2003. Biol. Conserv. 1, 255–263. doi: 10.1016/j.biocon.2012.01.010
Gbogbo F., Tabiri K., Yahaya M. (2017). Diversity and abundance of small mammals along a disturbance gradient on a university campus in Ghana. Int. J. Ecol. Dev. 1, 54–65. doi: 10.1007/s1111
Goheen J. R., Augustine D. J., Veblen K. E., Kimuyu D. M., Palmer T. M., Porensky L. M., et al. (2018). Conservation lessons from large-mammal manipulations in East African savannas: the KLEE, UHURU, and GLADE experiments. Ann. New York Acad. Sci. 1429, 31–49. doi: 10.1111/nyas.13848
Haines-Young R. (2009). Land use and biodiversity relationships. J. Land Use Policy 265), 178–186. doi: 10.1016/j.landusepol.2009.08.0
Hieronimo P., Kimaro D. N., Kihupi N. I., Gulinck H., Mulungu L. S., Msanya B. M., et al. (2014). Land use determinants of small mammal abundance and distribution in a plague endemic area of Lushoto District, Tanzania. Tanzania J. Health Res. 16 (3). doi: 10.4314/thrb.v16i3.8
Hsieh T., Ma K., Chao A. (2016). iNEXT: an r package for rarefaction and extrapolation of species diversity (Hill numbers). Methods Ecol. Evol. 12, 1451–1456. doi: 10.1111/2041-210X.12613
Hurst Z. M., McCleery R. A., Collier B. A., Silvy N. J., Taylor P. J., Monadjem A. (2014). Linking changes in small mammal communities to ecosystem functions in an agricultural landscape. Mamm. Bio 1, 17–23. doi: 10.1016/j.mambio.2013.08.008
IPBES (2019). Global assessment report on biodiversity and ecosystem services of the intergovernmental science-policy platform on biodiversity and ecosystem services. Eds. Brondizio E. S., Settele J., Díaz S., Ngo H. T. (Bonn, Germany: IPBES secretariat), 1148. doi: 10.5281/zenodo.3831673
Ivanter E., Makarov A. (2002). Daily activity and mobility of the common shrew (Sorex araneus l.). Russ J. Ecol. 33 (4), 280–285. doi: 10.1023/A:1016224522263
Kavana P. Y., Mahonge C. P., Sangeda A. Z., Mtengeti E. J., Fyumagwa R., Nindi S., et al. (2017). Panorama of agro-pastoralism in western Serengeti: a review and synthesis. Livest. Res. Rural. Dev. 29, (10).
Kawamura K., Yamaura Y., Senzaki M., Ueta M., Nakamura F. (2019). Seasonality in spatial distribution: climate and land use have contrasting effects on the species richness of breeding and wintering birds. Ecol. Evol. 13, 7549–7561. doi: 10.1002/ece3.5286
Keesing F., Ostfeld R. S., Okanga S., Huckett S., Bayles B. R., Chaplin-Kramer R., et al. (2018). Consequences of integrating livestock and wildlife in an African savanna. Nat. Sustain 10, 566–573. doi: 10.1038/s41893-018-0149-2
Kija H. K., Ogutu J. O., Mangewa L. J., Bukombe J., Verones F., Graae B., et al. (2020). Spatio-temporal changes in wildlife habitat quality in the greater Serengeti ecosystem. Sustainability 6, 2440. doi: 10.3390/su12062440
Lamani S. (2014). Diet and microhabitat use of the woodland dormouse graphiurus murinus at the great fish river reserve, Eastern cape, south Africa (University of Fort Hare), 109.
Lehikoinen P., Santangeli A., Jaatinen K., Rajasärkkä A., Lehikoinen A. (2018). Protected areas act as a buffer against detrimental effects of climate change–evidence from large-scale, long-term abundance data. Global Change Biol. 25 (1), 304–313. doi: 10.1111/gcb.14461
Leirs H., Stenseth N., Nichols J. D., Hines J. E., Verhagen R., Verheyen W. (1997). Stochastic seasonality and nonlinear density-dependent factors regulate population size in an African rodent. Nature 6647, 176–180. doi: 10.1038/38271
Macchi M. (2010). Mountains of the world: ecosystem services in a time of global and climate change. seizing opportunities: meeting challenges. paper presented at the mountains of the world: ecosystem services in a time of global and climate change. seizing opportunities: meeting challenges (Kathmandu, Nepal: International Centre for Integrated Mountain Development).
Madikiza Z. J., Bertolino S., Baxter R. M., Do Linh San E. (2010). Seasonal, sexual and age-related variations in the live-trapping success of woodland dormice graphiurus murinus. Zool Stud. 6, 797–805. doi: 10.6620/ZS
Magige F. (2012). Human-wildlife interaction in Serengeti and ngorongoro districts of Tanzania: a case study on small mammals. Tanz. J. Sci. 1, 95–103.
Magige F. J. (2013). Rodent species diversity in relation to altitudinal gradient in northern Serengeti, Tanzania. Afr. J. Ecol. 4, 618–624. doi: 10.1111/aje.12075
Magige F. (2016). Variation of small mammal populations across different habitat types in the Serengeti ecosystem. Tanz. J. Sci. 1, 15–23.
Magige F., Senzota R. (2006). Abundance and diversity of rodents at the human–wildlife interface in western Serengeti, Tanzania. Afr. J. Ecol. 3, 371–378. doi: 10.1111/j.1365-2028.2006.00641.x
Martínez U. J., Millien V., Simone I., Priotto J. W. (2014). Ecological preference between generalist and specialist rodents: spatial and environmental correlates of phenotypic variation. Biol. J. Linn. Soc 112, 180–203. doi: 10.1111/bij.12268
Massawe A., Rwamugira W., Leirs H., Makundi R., Mulungu L. S. (2006). Do farming practices influence population dynamics of rodents? a case study of the multimammate field rats, mastomys natalensis, in Tanzania. Afr. J. Ecol. 3, 293–301. doi: 10.1111/j.1365-2028.2006.00709.x
Maxwell S. L., Butt N., Maron M., McAlpine C. A., Chapman S., Ullmann A., et al. (2019). Conservation implications of ecological responses to extreme weather and climate events. Divers. Distrib. 25 (4), 613–625. doi: 10.1111/ddi.12878
McCay T. S., Storm G. L. (1997). Masked shrew (Sorex cinereus) abundance, diet and prey selection in an irrigated forest. Am. Midl. Nat. 138 (2), 268–275. doi: 10.2307/2426820
Mukhopadhyay S., Ogutu J. O., Bartzke G., Dublin H. T., Piepho H. P. (2019). Modelling spatio-temporal variation in sparse rainfall data using a hierarchical Bayesian regression model. J. Agric. Biol. Environ. Stat. 24 (2), 369–393. doi: 10.1007/s13253-019-00357-3
Mulungu (2017). “Control of rodent pests in maize cultivation: the case of Africa,” in Achieving sustainable maize cultivation, vol. 2 . Ed. Watson D. (London: Francis Dodds, Burleigh Dodds Science Publishing), 317–337.
Mulungu L. S., Themb’alilahlwa A. M., Massawe A. W., Kennis J., Crauwels D., Eiseb S., et al. (2011). Dietary differences of the multimammate mouse, mastomys natalensis (Smith 1834), across different habitats and seasons in Tanzania and Swaziland. Wildl. Res. 7, 640–646. doi: 10.1071/WR11028
Newbold T., Bentley L. F., Hill S. L., Edgar M. J., Horton M., Su G., et al. (2020). Global effects of land use on biodiversity differ among functional groups. Funct. Ecol. 34 (3), 684–693. doi: 10.1111/1365-2435.13500
Niamir-Fuller M., Reid C., R. and Milner-Gulland E. (2012). Co-Existence of wildlife and pastoralism on extensive rangelands: competition or compatibility? Pastoralism: Res. Policy Pract. 2 (8), 1–14. doi: 10.1186/2041-7136-2-8
Norton-Griffiths M., Herlocker D., Pennycuick L. (1975). The patterns of rainfall in the Serengeti ecosystem, Tanzania. Afr. J. Ecol. 4, 347–374. doi: 10.1111/j.1365-2028.1975.tb00144.x
Odhiambo R. O., Makundi R. H., Leirs H., Verhagen R. (2008). Dietary selection in mastomys natalensis (Rodentia: muridae) in the maize agro-ecosystems of central and southwestern Tanzania. Journal of Mammalia 72, 3, 169–177. doi: 10.1515/MAMM.2008.007
Ofori B. Y., Garshon R. A., Jones K., Daniel K. (2014). Preliminary checklist and aspects of the ecology of small mammals at the university of Ghana botanical garden, Accra plains, Ghana. J. Biodivers. Environ. Sci. 4, 323–333.
Ogutu J. O. N., Piepho H.-P., Said M. Y., Kifugo S. C., Reid R. S., Gichohi H., et al. (2013). Changing wildlife populations in Nairobi national park and adjoining athi-kaputiei plains: collapse of the migratory wildebeest. Conserv. Biol. 1, 11–26. doi: 10.2174/1874839201307010011
Ogutu J. O., Piepho H. P., Said M. Y., Ojwang G. O., Njino L. W., Kifugo S. C., et al. (2016). Extreme wildlife declines and concurrent increase in livestock numbers in Kenya: what are the causes? PloS One 9, e0163249. doi: 10.1371/journal.pone.0163249
Osbourne J. D., Anderson J. T., Spurgeon A. M. (2005). Effects of habitat on small-mammal diversity and abundance in West Virginia. Wildlife Soc. Bulletin 33 (3), 814–822. doi: 10.2193/0091-7648(2005)33[814:EOHOSD]2.0.CO;2
Panzacchi M., Linnell J. D., Mellis C., Odden M., Odden J., Gorin L., et al. (2009). Effect of land-use on small mammal abundance and diversity in a forest-farmland mosaic landscape in south-eastern Norway. For. Ecol. Manage. 259 (8), 1536–1545. doi: 10.1016/j.foreco.2010.01.030
Ralaizafisoloarivony N. A., Kimaro D. N., Kihupi N. I., Mulungu L. S., Leirs H., Msanya B. M., et al. (2014). Vegetation habitats and small mammals in a plague endemic area in Western usambara mountains, Tanzania. Tanzania J. Health Res. 16, (3). doi: 10.4314/thrb.v16i3.6
Said M. Y., Ogutu J. O., Kifugo S. C., Makui O., Reid R. S., de Leeuw J. (2016). Effects of extreme land fragmentation on wildlife and livestock population abundance and distribution. J. Nat. Conserv. 34, 151–164. doi: 10.1016/j.jnc.2016.10.0051617-1381/
Salvatori V., Egunyu F., Skidmore A., De Leeuw J., Van Gils H. (2001). The effects of fire and grazing pressure on vegetation cover and small mammal populations in the maasai Mara national reserve. Afr J. Ecol. 2, 200–204. doi: 10.1046/j.1365-2028.2001.00295.x
Schmidt N. M., Olsen H., Bildsøe M., V. and Leirs H. (2005). Effects of grazing intensity on small mammal population ecology in wet meadows. Basic Appl. 1, 57–66. doi: 10.1016/j.baae.2004.09.009
Seamon J. O., Adler G. H. (1996). Population performance of generalist and specialist rodents along habitat gradients. Can. J. Zool. 74, 1130–1139. doi: 10.1139/z96-125
Shilereyo M., Magige F. J., Ogutu J. O., Røskaft E. (2020). Small mammal community demography and reproductive seasonality under protection, pastoralism and agriculture in the Serengeti ecosystem, Tanzania. Int. J. Biodivers. 4, 253–269. doi: 10.5897/IJBC2020.1397
Shilereyo M. T., Magige F. J., Ogutu J. O., Røskaft E. (2021). Land use and habitat selection by small mammals in the Tanzanian greater Serengeti ecosystem. Glob. Ecol. Conserv. 27, e01606. doi: 10.1016/j.gecco.2021.e01606
Sinclair A. (2008). “Integrating conservation in human and natural systems,” in Serengeti III: human impacts on ecosystem dynamics. Eds. Sinclair A. R. E., Packer C., Mduma S. A. R., Fryxell J. M. (Chicago: University of Chicago Press), 471–495.
Skinner J. D., Chimimba C. T. (2005). The mammals of the southern African sub-region (Cambridge University Press).
Stanley W. T., Goodman S. M., Newmark W. D. (2011). Small mammal inventories in the East and West usambara mountains, tanzania. 1. study areas, methodologies, and general results. Fieldiana Zool. 4, 1–17. doi: 10.3158/2158-5520-4.1.1
Stenseth N. C., Leirs N. H., Mercelis S., Mwanjabe P. (2001). Comparing strategies for controlling an African pest rodent: an empirically based theoretical study. J. Appl. Ecol. 38, 1020–1031. doi: 10.1046/j.1365-2664.2001.00656.x
Tabeni S., Ojeda R. (2005). Ecology of the Monte desert small mammals in disturbed and undisturbed habitats. J. Arid Environ. 1, 244–255. doi: 10.1016/j.jaridenv.2005.03.009
Timbuka C., Kabigumila J. (2006). Diversity and abundance of small mammals in the Serengeti kopjes. Tanzania Tanz. J. Sci. 1, 1–12. doi: 10.4314/tjs.v32i1.18424
Trisurat Y., Shirakawa H., John M., Johnston J. M. (2019). Land-Use/Land-Cover change from socio-economic drivers and their impact on biodiversity in nan province, Thailand. J. Sustainability 11 (649), 1–22. doi: 10.3390/su11030649
Wasiolka B., Blaum N. (2011). Comparing biodiversity between protected savanna and adjacent non-protected farmland in the southern Kalahari. J. Arid Environments 75, 836–841. doi: 10.1016/j.jaridenv.2011.04.011
Watson J. E., Shanahan D. F., Marco M., Allan J., Laurance W. F., Sanerson E. W., et al. (2016). Catastrophic declines in wilderness areas undermine global environment targets. Journal of Current Biology 26, 21, 2929–2934. doi: 10.1016/j.cub.2016.08.049
Welegerima K., Meheretu Y., Haileselassie T. H., Gebre B., Kidane D., Massawe A. W., et al. (2020). Abundance and microhabitat use of rodent species in crop fields and bushland in Ethiopia. J. Vertebrate Biol. 69 (2), 20054–20051. doi: 10.25225/jvb.20054
Wells H. B., Kimuyu D. M., Odadi W. O., Dougill A. J., Stringer L. C., Young T. P. (2021). Wild and domestic savanna herbivores increase smaller vertebrate diversity, but less than additively. J. Appl. Ecol. 58, 953–963. doi: 10.1111/1365-2664.13843
Wilting H. C., Schipper A. M., Bakkenes M., Meijer J. R., Huijbregts M. A. (2017). Quantifying biodiversity losses due to human consumption: a global-scale footprint analysis. Environ. Sci. Technol. 51 (6), 3298–3306. doi: 10.1021/acs.est.6b05296
Winkler K., Fuchs R., Rounsevell M., Herold M. (2021). Global land use changes are four times greater than previously estimated. Nat. Commun. 12 (1), 2501. doi: 10.1038/s41467-021-22702-2
Keywords: small mammals, relative abundance, species diversity, rainfall seasonality, land use, Serengeti ecosystem
Citation: Shilereyo MT, Magige FJ, Ogutu JO and Røskaft E (2023) Small-mammal abundance and species diversity: land use and seasonal influences in the Serengeti Ecosystem, Tanzania. Front. Conserv. Sci. 4:981424. doi: 10.3389/fcosc.2023.981424
Received: 29 June 2022; Accepted: 30 June 2023;
Published: 25 July 2023.
Edited by:
Mayukh Chatterjee, North of England Zoological Society, United KingdomReviewed by:
Didas Kimaro, Mwenge Catholic University (MWECAU), TanzaniaCopyright © 2023 Shilereyo, Magige, Ogutu and Røskaft. This is an open-access article distributed under the terms of the Creative Commons Attribution License (CC BY). The use, distribution or reproduction in other forums is permitted, provided the original author(s) and the copyright owner(s) are credited and that the original publication in this journal is cited, in accordance with accepted academic practice. No use, distribution or reproduction is permitted which does not comply with these terms.
*Correspondence: Eivin Røskaft, ZWl2aW4ucm9za2FmdEBudG51Lm5v
†These authors have contributed equally to this work
Disclaimer: All claims expressed in this article are solely those of the authors and do not necessarily represent those of their affiliated organizations, or those of the publisher, the editors and the reviewers. Any product that may be evaluated in this article or claim that may be made by its manufacturer is not guaranteed or endorsed by the publisher.
Research integrity at Frontiers
Learn more about the work of our research integrity team to safeguard the quality of each article we publish.