- 1MIX Research Sweden AB, Uppsala, Sweden
- 2WWF-US Arctic Program, World Wildlife Fund, Inc. (WWF), Anchorage, AK, United States
- 3Circular Economy Department, Swedish Environmental Protection Agency, Stockholm, Sweden
- 4Department of Game and Wildlife, Västra Götalands County Administrative Board, Vänersborg, Sweden
- 5Genomic Medicine Group, J. Craig Venter Institute, La Jolla, CA, United States
- 6WWF Arctic Programme, Worldwide Fund for Nature, Solna, Sweden
Polar bears (Ursus maritimus), Eurasian lynx (Lynx lynx) and snow leopards (Panthera uncia) are elusive large carnivores inhabiting snow-covered and remote areas. Their effective conservation and management are challenged by inadequate population information, necessitating development of novel data collection methods. Environmental DNA (eDNA) from snow tracks (footprints in snow) has identified species based on mitochondrial DNA, yet its utility for individual-based analyses remains unsolved due to challenges accessing the nuclear genome. We present a protocol for capturing nuclear eDNA from polar bear, Eurasian lynx and snow leopard snow tracks and verify it through genotyping at a selection of microsatellite markers. We successfully retrieved nuclear eDNA from 87.5% (21/24) of wild polar bear snow tracks, 59.1% (26/44) of wild Eurasian lynx snow tracks, and the single snow leopard sampled. We genotyped over half of all wild polar bear samples (54.2%, 13/24) at five loci, and 11% (9/44) of wild lynx samples and the snow leopard at three loci. Genotyping success from Eurasian lynx snow tracks increased to 24% when tracks were collected by trained rather than untrained personnel. Thirteen wild polar bear samples comprised 11 unique genotypes and two identical genotypes; likely representing 12 individual bears, one of which was sampled twice. Snow tracks show promise for use alongside other non-invasive and conventional methods as a reliable source of nuclear DNA for genetic mark-recapture of elusive and threatened mammals. The detailed protocol we present has utility for broadening end user groups and engaging Indigenous and local communities in species monitoring.
1 Introduction
Effective conservation and management of many large carnivores is seriously challenged by inadequate knowledge of their ecology and behaviour, the size and connectivity of populations, and their adaptive capacity (Doran-Myers et al., 2021). While genetic tools can help address these questions, difficulty accessing necessary material from target individuals remains. Polar bears (Ursus maritimus), Eurasian lynx (Lynx lynx) and snow leopards (Panthera uncia) share common ecological attributes including expansive home ranges, low population densities, and solitary behaviour (Amstrup et al., 2003; Barea-Azcón et al., 2007; Alexander et al., 2016; von Arx, 2020). They come into conflict with people in many places, either directly, or through loss of property or predation of pets or livestock (Inskip and Zimmermann, 2009; Clark et al., 2012). Subject to management regimes that necessitate regular monitoring, they are good candidates for exploring the utility of environmental DNA (eDNA) as a tool to help inform these.
Conventional techniques for collecting genetic material from living large carnivores such as polar bears, snow leopards and Eurasian lynx can be labour intensive, costly, potentially hazardous for personnel, and stress-inducing for animals (Adams et al., 2019). Techniques may include capture, handling, and close physical proximity (e.g., Vongraven et al., 2012). The advancement of molecular techniques has enabled genetic information to be obtained from lower quality and quantity source material. This includes eDNA, defined here as the genetic traces that living organisms leave behind in the environment (Taberlet et al., 2012). eDNA can be captured and analysed from cells shed in urine, faeces, sweat, breath, skin and other sources using similar field and molecular methods to those in forensic science (Taberlet et al., 2012; Pedersen et al., 2015; Bruce et al., 2021). eDNA has been successfully extracted and amplified from water (Ficetola et al., 2008; Kelly et al., 2014; Thomsen, 2015), soil (Guerrieri et al., 2021) and air (Bohmann and Lynggaard., 2023; Garrett et al., 2023). It is becoming a routine biological survey tool for detecting and quantifying species and describing community assemblages because of the advantages it offers to traditional sampling (Beng and Corlett, 2020; Bruce et al., 2021).
eDNA is still in its infancy as a tool for obtaining population genetic information, which often requires analysis at the individual level (Adams et al., 2019). However, non-invasive population genetic analyses using eDNA are increasing used in conservation and ecology (Broquet et al., 2007), e.g., water samples in studies of whale sharks (Sigsgaard et al., 2017; Dugal et al., 2022), hair samples in studies of Eurasian lynx, wolverines and snow leopards (Ulizio et al., 2006; Janečka et al., 2008; Davoli et al., 2013), and scats for population genetics of brown bears and wolverines (Bellemain et al., 2005; Ulizio et al., 2006). While fecal DNA is a common source of eDNA for non-invasive population genetics (Broquet et al., 2007), collection from wild populations of large carnivores such as polar bears, lynx and snow leopards can potentially interfere with territorial behaviours (Brzeziński and Romanowski, 2006; Lefort et al., 2015).
Even if individual material can be sourced, it may not be from a medium that preserves the DNA at a high enough quality to yield genetic information (Harrison et al., 2019). For individual identification, yield is especially important because of the relative scarcity of the material needed: mammalian cells each contain only two copies of nuclear DNA compared with thousands of copies of mitochondrial DNA (mtDNA). Working with degraded and/or trace amounts of DNA presents its own set of challenges for genetic analysis, which is a well-known issue for ancient and modern eDNA (Pedersen et al., 2015). However, if efficient, simple and cost-effective collection methods can be developed that overcome these issues, eDNA as a population genetic tool offers promise for research and monitoring of species, including large carnivores. The benefits of such a tool could reduce field sampling costs, enable sample collection by non-experts, and achieve more comprehensive representation of wild populations.
The nivean habitats in which the three species live offer a promising alternative: sloughed skin cells in their snow tracks (footprints left in snow). The benefits of snow tracks are that they are attributable to individual animals, especially by hunters and trackers, and they occur at cold temperatures, which are likely to slow down eDNA degradation rate (Hellström et al., 2019). While snow as a medium has been used to identify forest-dwelling mammals to species level using eDNA (Dalén et al., 2007; Franklin et al., 2019; Kinoshita et al., 2019; Barber-Meyer et al., 2020), no studies to date have reported successful use of eDNA from snow for individual-level analyses.
The objectives of this study were to 1) determine success of nuclear eDNA retrieval from polar bear, Eurasian lynx and snow leopard snow tracks towards individual identification, verified by genotyping at a selection of multilocus markers commonly used for this purpose, and 2) provide a detailed collection protocol for capturing nuclear eDNA from the snow tracks of these species.
2 Methods
2.1 Sample collection
We collected snow from polar bears and Eurasian lynx snow tracks in the wild and in captivity, and from a snow leopard in captivity. For all three species we retrieved reference material (hair, saliva, nasal mucus) as positive controls to refine methods and verify the accuracy of snow tracks for microsatellite genotyping. Hair samples were stored in dry envelopes at room temperature (RT), and saliva samples were dried and stored at RT. The field protocol for collecting eDNA from polar bear and snow leopard snow tracks was adapted from previous trials with Eurasian lynx and European otter (Lutra lutra) in Sweden in 2017 and 2018. It includes detailed field sampling, filtering, and fixation protocols (Supplementary Material S1). Information recorded during sample collection included date, number of snow tracks (individual paw impressions), air temperature, geographical coordinates of sampling locations, collection vessels, type of filters, and volume of melted snow filtered (Table S1). All samples were fixed in ethanol 200 proof Molecular Biology Grade (Thermo Fisher Scientific Inc., Waltham, MA, USA).
Wild polar bear snow tracks were collected in the vicinity of Utqiaġvik, Alaska, United States of America (Figure 1) in January 2019 and May 2022. Field crews included staff from the North Slope Borough Department of Wildlife Management, Utqiaġvik, Alaska and individuals from the Inupiat community, who had extensive knowledge of the area, environment and working in extreme conditions. Snow tracks were detected visually from snow mobiles and collected when discovered. Tracks clearly created by more than one individual were not collected. Sampling of fresh snow tracks and corresponding hair samples as positive controls from captive polar bears took place in 2018 from Orsa predator park, Sweden and Rauna Zoo, Finland.
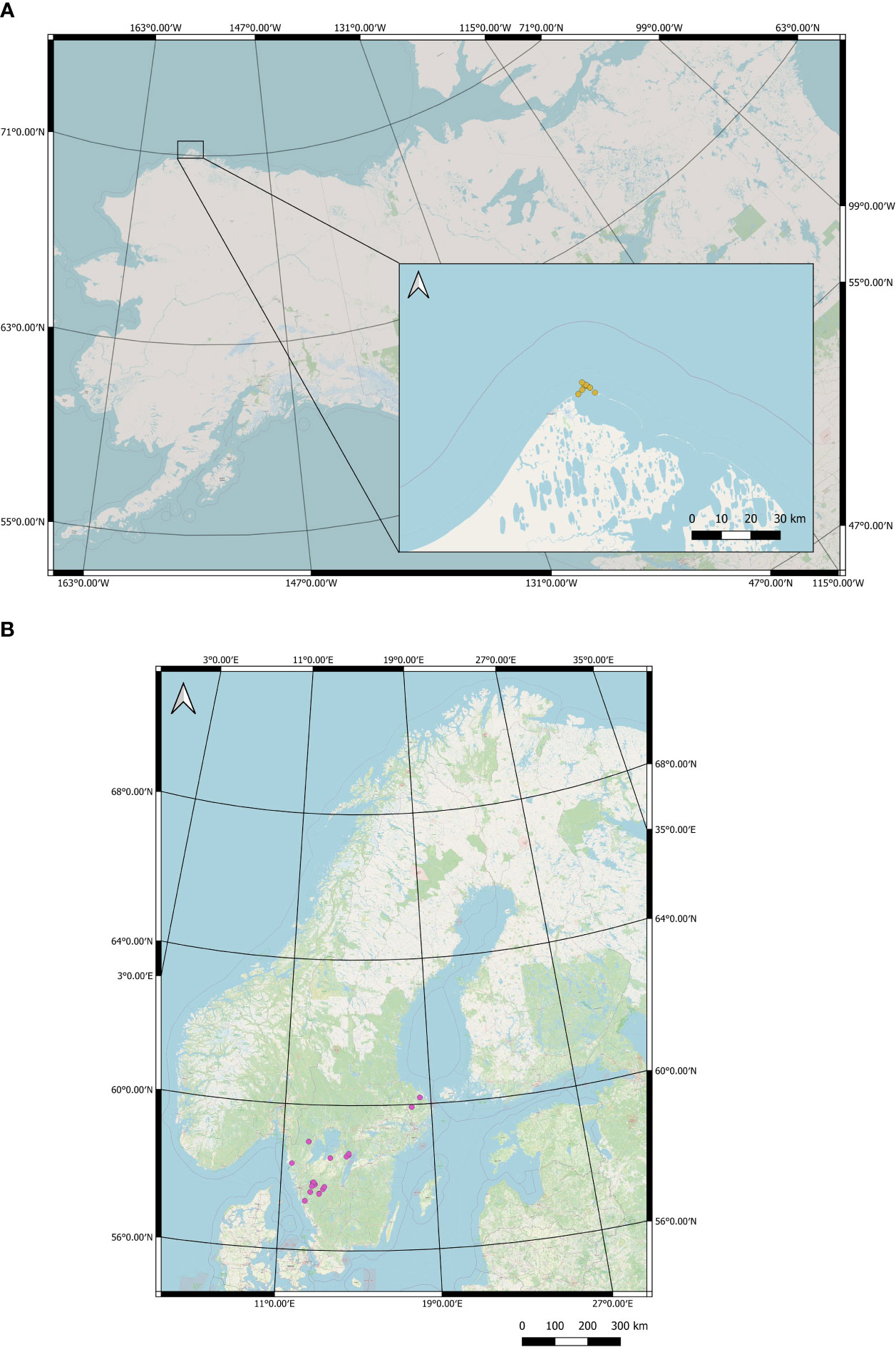
Figure 1 Collection sites of (A) wild polar bear (U. maritimus) snow tracks in the vicinity of Utgiaqvik, USA and (B) wild Eurasian lynx (L. lynx) snow tracks in Sweden.
Wild Eurasian lynx snow tracks were collected from several locations across central and southern Sweden in December 2018, January 2019, and January and February 2021, with the assistance of local hunters, and wildlife trackers and staff from five Swedish County Administrative boards (Figure 1). Eurasian lynx regularly move in family groups of females with kittens, and multiple individuals walk in the same footprints (Doran‐Myers et al., 2021). Trackers are skilled at detecting the point at which individuals deviate from shared footprints and form individual tracks. Care was taken to collect tracks most likely to be from single individuals. Fresh snow tracks, together with hair and saliva as positive controls were collected from captive Eurasian lynx at Rauna Zoo, Finland, and Nordens Ark, Sweden. Samples collected from wild lynx were divided into those taken by trained personnel (n = 25) and those taken by untrained personnel and volunteers (n = 19) to assess potential differences in success between the groups. Trained personnel constitute individuals who were provided written protocols and completed a one-hour theoretical training, observed sample collection conducted by experts, and then collected samples under the supervision of trainers. Untrained personnel and volunteers had access to written protocols but did not receive hands-on training prior to collecting samples.
Snow tracks from one snow leopard were collected from Nordens Ark, Sweden in 2021 using fresh snow brought in from a nearby forest. Nasal mucus was collected from the same individual as a positive control.
2.2 eDNA extraction
After snow was melted and filtered, eDNA was extracted from the filters in facilities purpose-built for aquatic eDNA analysis (Bruce et al., 2021), following the ethanol extraction protocol outlined in Spens et al. (2017). Additional steps to the protocol included overnight pooling of the lysate from the pellet in ethanol with the lysate from the filter capsules. DNA was extracted from hair, saliva, and nasal mucus using the Qiagen Blood and Tissue kit following the manufacturer’s instructions. The protocol was modified by adding 70 μl TE buffer pH 8 (Promega, Germany) instead of the AE buffer, heated to 70°C. Samples were stored at -20°C until further processing.
The extracted DNA showed signs of inhibition, so all samples were concentrated and cleaned using the Genomic DNA Clean & Concentrator Kit (Zymo Research, Carlsbad, CA) following the manufacturer’s instructions and using TE buffer pH 8 (Promega, Germany) heated to 70°C. LoBind tubes (Eppendorf, Sweden) were used to avoid DNA absorption into the plastic. We measured total DNA concentrations using the dsDNA Qubit high-sensitivity assay (Thermo Fisher Scientific, Carlsbad, CA) following the manufacturer’s protocol.
2.3 Microsatellite analysis
The aim of microsatellite analysis from nuclear DNA was to determine whether eDNA collected from snow is a suitable source for future population analyses, and we used a subset of microsatellites to investigate this. For full population analyses, six replicates of each marker and sample would be required to quantify genotyping errors such as allelic dropout and false alleles.
We analysed a total of 30 polar bear samples (24 wild-collected and four captive-collected snow track samples, two positive controls). We pre-screened a subset of samples (n = 5) at 10 polymorphic microsatellite loci that were purpose-designed to maximize genotyping success from degraded DNA (Brandt et al., 2014). PCR products were visualized on a 2% agarose gel. We selected five microsatellites that showed clean bands within the expected allelic size range for genotyping: Uma 14, Uma 40, Uma 42, Uma 73 and Uma 95 (Brandt et al., 2014). Forty-eight Eurasian lynx samples (44 wild-collected and two captive-collected snow track samples, two positive controls) and two snow leopard samples (one captive-collected snow track sample, one positive control) were genotyped at feline microsatellites FCA001, FCA026 and F115 (Menotti-Raymond et al., 1999). All PCRs were run in single-plex format to maximise performance and minimise errors.
PCR amplification conditions following published protocols for polar bears (Brandt et al., 2014) and felid protocols for Eurasian lynx and snow leopards (Menotti-Raymond et al., 1999) resulted in very poor amplification success. Therefore, we adjusted the protocols to include a 2-step touch-down PCR. Each primer set was run in separate tubes and was not pooled during PCR. For all samples, PCRs were performed in 10 μl reaction volumes: 2 × multiplex PCR master mix (Qiagen Multiplex kit), 0.3 μM F and R primer and 2 μl template DNA. Annealing temperatures were initially set at 57°C and decreased (touched-down) by 0.5°C per cycle until 50°C, as follows: 95°C 5 min, 14 (95°C 20 s, 57 – 50°C 30 s, 72°C 20 s), 25 (95°C 20 s, 50°C 30 s, 72°C 20 s), 72°C 5 min. PCR products were then used as template DNA for the second PCR, with amplification conditions of 95°C 5 min, 25 (95°C 20 s, 51°C 30 s, 72°C 20 s) 72°C 5 min.
Final PCR products were visualized on a 2% agarose gel to confirm amplification success and sent to Macrogen Europe for fragment analysis using GeneScan™ GS350 size standard (Applied Biosystems). Three different people visualized and scored the data using the software Peak Scanner 1.0 (Applied Biosystems). Allele boundaries were set at 0.5 bp on either side of allele peaks for dinucleotide repeat loci and 1.0 bp for tetra- and penta- nucleotide repeat loci. Negative controls were included for every tenth sample.
Where full (5-locus) genotypes were obtained for polar bear snow track samples, we used the Matches function for multilocus data in GENALEX v. 6.5 (Smouse and Peakall, 2012) to automatically detect repeated genotypes within the dataset and determine the number of unique and duplicate genotypes.
3 Results
3.1 Nuclear eDNA capture from snow tracks and microsatellite genotyping success
Total DNA concentrations from snow track samples were low, especially from wild polar bear and Eurasian lynx tracks (polar bear: 0.02 - 7.06 ng/µl, mean 0.92, median 0.37, Eurasian lynx: 0.01 - 32.00 ng/µl, mean 3.83, median 1.18, snow leopard:1.2 ng/µl). We successfully captured nuclear eDNA, verified by microsatellite genotyping, from 87.5% (21/24) of wild polar bear snow track samples, 59.1% (26/44) of wild Eurasian lynx snow track samples, and the single snow leopard sampled (Table 1; Figure S1).
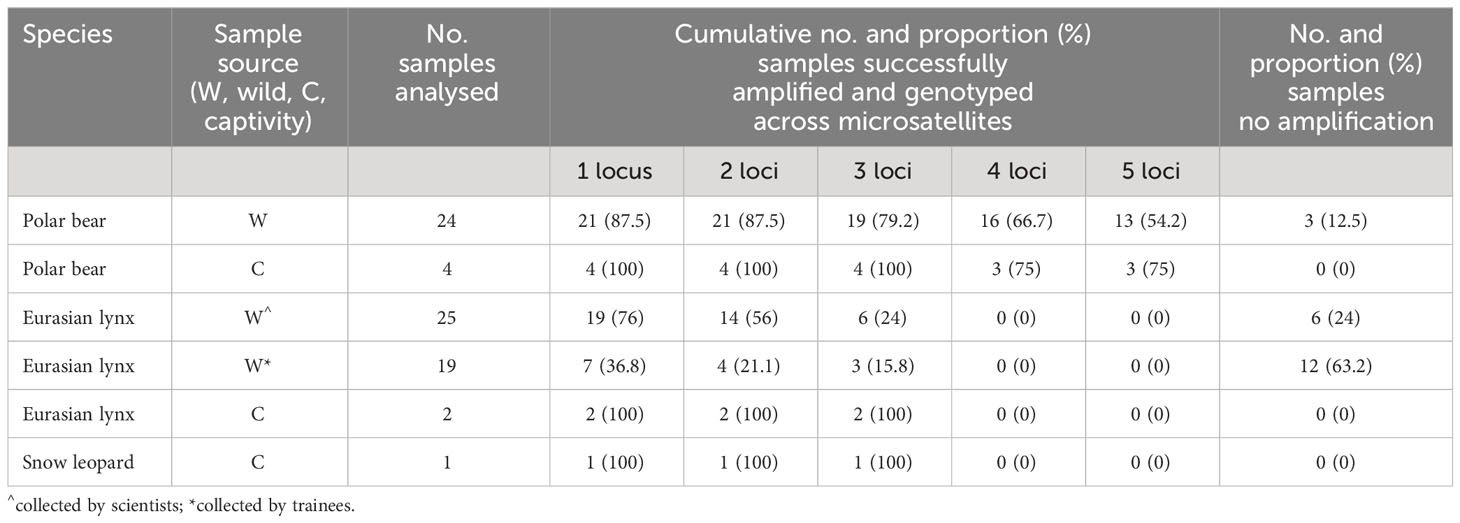
Table 1 Success of microsatellite amplification in wild and captive polar bears, U. maritimus, Eurasian lynx, L. lynx and captive snow leopards, P. uncia, from nuclear eDNA isolated from their snow tracks.
Genotyping performance varied among species. Over half of all wild polar bear snow track samples were genotyped across all five microsatellites screened (54.2%, 13/24, Table 1), compared with one fifth of wild Eurasian lynx samples genotyped across all three microsatellites screened (20.5%, 9/44, Table 1). Snow track samples from captive lynx, the captive snow leopard and three out of four captive polar bears were genotyped at all loci screened (Table 1). All negative controls were negative.
Genotyping success from Eurasian lynx snow tracks was affected by the training level of personnel collecting snow tracks. We retrieved nuclear eDNA from three-quarters (76%, 19/25) of all lynx snow tracks collected by trained personnel, and genotyped around a quarter (24%, 6/25) of samples at three microsatellites. This was significantly higher than for samples collected by untrained personnel and volunteers, where we retrieved nuclear eDNA from 36.8% (7/19) of tracks and genotyped 15.7% (3/19) at three microsatellites (X2 = 6.85, d.f. = 1, p = 0.01, Table 1).
Three wild polar bear and 18 Eurasian lynx snow track samples did not amplify scorable genotypes at any loci. Three wild polar bear snow track samples amplified three alleles at multiple loci, suggesting more than one individual in the sample.
Of the 13 wild polar bear snow track samples scored at all five loci, 11 had unique genotypes. The remaining two samples were identical at all five loci and were collected on consecutive days. Based on this, the 13 snow track samples are likely attributable to 12 polar bears, with one of those bears sampled on two occasions.
3.2 Deviations from expected microsatellite allele signatures
For two of the five polar bear microsatellite loci screened, allele sizes were inconsistent with their nucleotide repeat type. Uma 14 (hexanucleotide, (AAATG)8) and Uma 95 (tetranucleotide, (ATCC)9) displayed a two base-pair (bp) shift in allele size that was sustained for all subsequent larger alleles.
In Eurasian lynx, locus F115 contained two different allele length ranges, with the majority of alleles between 122-142 bp, alleles at 163 and 169 bp and one allele at 229 bp, expressed as a homozygote in three individuals. This locus has been omitted from previous studies of African lions (Panthera leo) and sequencing of alleles revealed size homoplasy in one (Miller et al., 2014).
3.3 Evidence of nuclear eDNA integrity
Genotypes from hair, nasal mucus and saliva (positive controls) were identical to those from their corresponding snow tracks across all microsatellite loci and species for the paired samples we collected. In these, we did not observe amplification errors that may be indicative of allelic dropout or false alleles across 19 genotypes, of which 10 were heterozygous and nine were homozygous.
4 Discussion
Using the detailed collection protocol presented, we have successfully and reliably retrieved nuclear eDNA from snow tracks of three carnivore species and generated multilocus microsatellite genotypes at a selection of markers commonly used for individual identification. Nuclear eDNA retrieval was high from wild polar bear snow tracks (87.5%) and wild lynx snow tracks (76% from samples collected by trained personnel and 59% overall). We genotyped over half of all polar bear snow track samples at five microsatellites, which were sufficiently polymorphic to enable differentiation of genotypes. Eleven unique genotypes and two matching genotypes from thirteen sets of snow tracks likely represented 12 polar bears, one of which was sampled on different days. Around a quarter of all wild lynx snow tracks were successfully genotyped at all three loci screened, as was the single snow leopard: a promising step towards the use of snow tracks for individual identification of these species.
To our knowledge, this is the first time nuclear eDNA retrieval from snow tracks in Eurasian lynx, polar bears and a snow leopard has been reported. Sample sizes for lynx and polar bears were able to provide estimates of genotyping success from snow tracks, which we can relate to other non-invasive and traditional sampling methods for the species. In polar bears, tissue sampling with remote biopsy darting reported genotyping success of 79% (Pagano et al., 2014), compared with 43% success from scats (within Jensen et al., 2020), and 54% success from snow tracks, reported here. Microsatellite genotyping success of Eurasian lynx tissue samples in France was 88% (Huvier et al., 2023), while hair samples resulted in amplification (to PCR product) in 67% of samples, and 12-locus genotypes in 48% of samples (Davoli et al., 2013). We amplified 59% of lynx snow tracks to PCR product at one locus and genotyped 11 – 24% of lynx snow tracks at three loci. Finally, in Mongolia, up to 54% of putative snow leopard scats collected in the field were misidentified as red fox. Microsatellite genotypes at seven loci were obtained for 71 – 73% of those identified correctly, resulting in 33% success from scats (Janečka et al., 2008). While genotyping success from materials collected by non-invasive sampling is generally lower than that of tissue sampling, the abundance and relative ease with which hair and tracks can be encountered make them useful to augment sample sizes for population genetic analyses.
4.1 Viability of snow tracks for individual-level population genetic analyses
Post-sampling challenges of eDNA for population genetic analyses include amplification errors associated with degraded DNA, such as allelic dropout and false alleles (Taberlet and Luikart, 1999; Adams et al., 2019). If not identified and/or overcome, these errors can jeopardize the accuracy of population genetic analyses and subsequent findings related to e.g., parentage and mating systems, species hybridization, and population connectivity (Piggott and Taylor, 2003). Access to captive polar bears, Eurasian lynx and a snow leopard enabled us to preliminarily assess nuclear eDNA integrity from snow tracks by comparing genetic results with corresponding source material, including hair with follicles, saliva and nasal mucus. For all samples across all loci amplified, we retrieved identical genotypes from the snow tracks and their respective positive controls, both for homozygous and heterozygous genotypes: an encouraging result. However, because of the low quantity of DNA we retrieved from snow tracks in the wild, and our small number of positive controls, the risk of amplification error remains and should be accounted for in population genetic analyses that use snow tracks as a source of nuclear eDNA. Following best practices for molecular analyses derived from the fields of forensics, ancient DNA research and non-invasive genetic sampling will help to safeguard against and recognize errors (Piggott and Taylor, 2003; Broquet et al., 2007; Pedersen et al., 2015). Additional screening of known mother-offspring pairs and positive controls could further inform the reliability of snow tracks for microsatellite analyses. Finally, genome-wide protocols for snow samples could help to overcome downstream error specific to microsatellites (Coates et al., 2009).
4.2 Potential benefits of the method for the research field
Carnivore monitoring and conservation requires suitable and efficient sampling protocols (Barea-Azcón et al., 2007). Our study shows that snow tracks have good potential as a reliable source of nuclear DNA compared to other non-invasive sources and could complement conventional sources. The method offers several benefits. Firstly, the non-invasive nature of collecting snow tracks greatly reduces direct disturbance to animals and offers a culturally considerate method of studying populations. Disturbance of polar bears associated with research activities has been cause for concern by some Arctic Indigenous communities, and polar bear snow tracks have already been explored as a non-invasive way to assess individual characteristics (sex and size of bears) through visual assessment by Inuit hunters (Wong et al., 2011; Wong et al., 2017). Secondly, the availability of snow tracks of many species year-round in the Arctic and seasonally elsewhere makes them a promising additional source of nuclear eDNA for population monitoring, especially in regions where few animals but many footprints are observed, e.g., snow leopards in Gansu province, China, and polar bears in Russia-USA (Alexander et al., 2016; Conn et al., 2021). Finally, in our experience, the collection protocol presented, and the materials required to collect snow tracks, should be non-technical enough to be carried out by non-scientists once trained. This study demonstrated the need for investment into appropriate field training of non-professionals, as genotyping success increased substantially when samples were collected by trained personnel, rather than by untrained personnel and volunteers following written protocols alone. Furthermore, individuals who underwent theoretical and technical sample collection training contributed to the improvement of the written protocols through their interactions with trainers over the course of the training. Trainers amended the written protocols based on questions posed by the trainees during the training. Thus, the combination of detailed protocols and interactive field training with experts could broaden the range of end user groups to include hunters, trackers and Indigenous and local community members or volunteers. Snow tracks could be collected by such groups during existing programs, such as annual management inventories of large snow-dwelling mammals and community-based monitoring networks in Arctic sub-regions.
4.3 Potential conservation applications of the method
Obtaining estimates of abundance and trends for populations is deemed essential for monitoring responses to climate change (e.g., Vongraven et al., 2012), direct use, and other management measures. Especially if a variety of end user groups could collect snow tracks, genetic mark-recapture databases could be augmented by such sampling, thus achieving more complete representations of populations in time and space. Currently, 10 of the 19 polar bear subpopulations lack the data necessary to determine short-term trends in population status, and only three subpopulations have sufficient data to determine long-term trends (PBSG, 2021). Sampling from snow tracks could also further knowledge of ecological connectivity, which has been identified as a population attribute to understand, maintain and restore, both for snow leopard conservation globally and for Eurasian lynx conservation in Italy and Austria (Li et al., 2020; von Arx, 2020). Finally, as all three species are threatened by conflict with people (human-wildlife conflict, Inskip and Zimmermann, 2009; Clark et al., 2012), sampling of “problem animals” from their snow tracks could help identify and characterize individuals to inform correct management responses.
4.4 Next steps
Total DNA yield from snow tracks was sufficient to capture nuclear eDNA and successfully amplify a selection of microsatellites. Further experimental field work could include determining the minimum number and maximum age of snow tracks required to obtain high quality nuclear eDNA (Hellström et al., 2019; Barber-Meyer et al., 2020), understanding how environmental variables affect DNA from tracks (e.g., high winds, air temperatures, snow types, ultraviolet light exposure) with the aim of producing optimized collection guidelines, and testing the method in wild populations of snow leopards. PCR protocols were adjusted with a two-step PCR to increase success rates in this study, but more optimization of PCR protocols could further improve success. Screening samples at larger panels of microsatellite loci currently used in population genetic analyses of these species would also be informative to precisely compare success rates of snow tracks with other sources of DNA, generate robust genotyping error rates, and assess relative cost-effectiveness of snow tracks with other genetic sampling methods. Finally, familiarizing the method with researchers, managers at local, national and international scales, and people living alongside polar bears, Eurasian lynx and snow leopards, is necessary to mainstream it as an additional tool for conservation and management of these and other snow-living species.
Data availability statement
The raw data supporting the conclusions of this article will be made available by the authors, without undue reservation.
Ethics statement
Ethical approval was not required for the study involving animals in accordance with the local legislation and institutional requirements because eDNA from snow tracks required no approvals. Sample collection from captive animals was performed by staff of the captive institutions as part of their institutionally approved animal procedures.
Author contributions
EK, MH, and ML: concept for polar bears, wild polar bear sample collections, writing of manuscript. MB, and MH: concept for lynx. MH: concept for snow leopard. JN, MB, MH, PH, and VB: sample collections and field protocol adjustments, Sweden. MB: coordination of collection by hunters and trackers, Sweden. MH, PH, and VB: designing final field protocol. MH, AE and RA: molecular methods and laboratory methods design and changes. MH, RA and AE: Laboratory work. MH, ML, AE, PH, VB and RA: data analysis. VB and PH: Map design. All authors: sampling design and testing of snow track collection protocols, contribution to earlier versions or sections of manuscript, approval of final manuscript. All authors contributed to the article and approved the submitted version.
Funding
The authors declare that this study received funding from WWF Global Arctic Programme and World Wildlife Fund, Inc. (polar bears), Swedish Environmental Protection Agency (lynx; grant 331-18-016 and 331-19-005) and by MIX Research Sweden AB (snow leopard).
Acknowledgments
We are grateful to Billy Adams and Bobby Sarren for guidance in the field and assistance with sample collection in the vicinity of Utqiaġvik, Alaska. We thank Alaska Nannut Co-Management Council board members and polar bear subsistence hunters of Utqiaġvik for generously sharing Indigenous Knowledge of polar bears. We thank Andrew Von Duyke from the North Slope Borough in Utqiaġvik for organizing accommodation, research permits and field logistics in Alaska and assisting in sample collections. We appreciate Eben Hopson for field support in Utqiaġvik. Nicklas Wijkmark and Pähr Hellström collected samples in Alaska, Finland, and Sweden. Cecilia Edbom Blomstrand and Liselott Rasmussen collected snow samples in Sweden. Hunters and trackers at Västra Götaland-, Blekinge-, Halland-, Jönköping-, Östra Götaland- and Uppsala County Administrative Boards assisted with field collections of Lynx. Staff at Rauna Zoo in Finland, Orsa Predator Park and Mats Niklasson at Nordens Ark in Sweden assisted in snow collection and provided positive control samples in the parks. Gert Polet and Norwegian Polar Institute biologists Jon Aars and Magnus Andersson assisted with snow track collection in early iterations of the project. Cuong Tang at NatureMetrics Ltd, UK for fruitful discussions and performing initial laboratory trials. Jessica Sjöstedt MoRe Research, Sweden, Sabrina Neyrink INBO Belgium extracted DNA and Hannah Phillips assisted with extractions at J Craig Venter Institute USA. Samples in Alaska were collected under USFWS permit #MA80164B-0. Polar bear samples collected in 2019 were sent to Sweden to be processed under permit CITES 19US33153D/9. Polar bear samples collected in 2022 were processed at J Craig Venter Institute in La Jolla, San Diego USA.
Conflict of interest
Authors MH, PH, VB and RA were employed by the company MIX Research Sweden AB.
The remaining authors declare that the research was conducted in the absence of any commercial or financial relationship that could be constricted as a potential conflict of interest.
MIX Research Sweden AB funded the laboratory work for the snow leopard data. The funder was not involved in the study design, collection, analysis, interpretation of data, the writing of this article or the decision to submit it for publication.
Publisher’s note
All claims expressed in this article are solely those of the authors and do not necessarily represent those of their affiliated organizations, or those of the publisher, the editors and the reviewers. Any product that may be evaluated in this article, or claim that may be made by its manufacturer, is not guaranteed or endorsed by the publisher.
Supplementary material
The Supplementary Material for this article can be found online at: https://www.frontiersin.org/articles/10.3389/fcosc.2023.1250996/full#supplementary-material
References
Adams C. I., Knapp M., Gemmell N. J., Jeunen G. J., Bunce M., Lamare M. D., et al. (2019). Beyond biodiversity: Can environmental DNA (eDNA) cut it as a population genetics tool? Genes 10 (3), 192. doi: 10.3390/genes10030192
Alexander J. S., Gopalaswamy A. M., Shi K., Hughes J., Riordan P. (2016). Patterns of snow leopard site use in an increasingly human-dominated landscape. PloS One 11 (5), e0155309. doi: 10.1371/journal.pone.0155309
Amstrup S. C., Feldhamer G. A., Thompson B. C., Chapman J. A. (2003). “Polar bear, Ursus maritimus,” in Wild Mammals of North America: biology, management, and conservation (Baltimore: John Hopkins University Press), 587–610.
Barber-Meyer S. M., Dysthe J. C., Pilgrim K. L. (2020). Testing environmental DNA from wolf snow tracks for species, sex, and individual identification. Can. Wildlife Biol. Manage. 9 (1), 12–20.
Barea-Azcón J. M., Virgós E., Ballesteros-Duperon E., Moleón M., Chirosa M. (2007). Surveying carnivores at large spatial scales: a comparison of four broad-applied methods. Vertebrate Conserv. biodiversity 16, 1213–1230. doi: 10.1007/s10531-006-9114-x
Bellemain E. V. A., Swenson J. E., Tallmon D., Brunberg S., Taberlet P. (2005). Estimating population size of elusive animals with DNA from hunter-collected feces: four methods for brown bears. Conserv. Biol. 19 (1), 150–161. doi: 10.1111/j.1523-1739.2005.00549.x
Beng K. C., Corlett R. T. (2020). Applications of environmental DNA (eDNA) in ecology and conservation: opportunities, challenges and prospects. Biodiversity Conserv. 29, 2089–2121. doi: 10.1007/s10531-020-01980-0
Bohmann K., Lynggaard C. (2023). Transforming terrestrial biodiversity surveys using airborne eDNA. Trends Ecol Evol. 38 (2), 119–121. doi: 10.1016/j.tree.2022.11.006
Brandt J. R., Van Coeverden de Groot P. J., Zhao K., Dyck M. G., Boag P. T., Roca A. L. (2014). Development of nineteen polymorphic microsatellite loci in the threatened polar bear (Ursus maritimus) using next generation sequencing. Conserv. Genet. Resour. 6, 59–61. doi: 10.1007/s12686-013-0003-9
Broquet T., Ménard N., Petit E. (2007). Noninvasive population genetics: a review of sample source, diet, fragment length and microsatellite motif effects on amplification success and genotyping error rates. Conserv. Genet. 8, 249–260. doi: 10.1007/s10592-006-9146-5
Bruce K., Blackman R. C., Bourlat S. J., Hellström M., Bakker J., Bista I., et al. (2021). A practical guide to DNA-based methods for biodiversity assessment. (Sofia, Bulgaria: Pensoft Advanced Books), 90. doi: 10.3897/ab.e68634
Brzeziński M., Romanowski J. (2006). Experiments on sprainting activity of otters (Lutra lutra) in the bieszczady mountains, southeastern Poland/Observations des épreintes de la loutre (Lutra lutra) sur les montagnes du bieszczady au sud-est de la pologne. Mammalia 70 (1-2), 58–63. doi: 10.1515/MAMM.2006.019
Clark D. A., van BEEST F. M., Brook R. K. (2012). Polar bear-human conflicts: state of knowledge and research needs. Can. Wildlife Biol. Manage. 1 (1), 21–29.
Coates B. S., Sumerford D. V., Miller N. J., Kim K. S., Sappington T. W., Siegfried B. D., et al. (2009). Comparative performance of single nucleotide polymorphism and microsatellite markers for population genetic analysis. J. Heredity 100 (5), 556–564. doi: 10.1093/jhered/esp028
Conn P. B., Chernook V. I., Moreland E. E., Trukhanova I. S., Regehr E. V., Vasiliev A. N., et al. (2021). Aerial survey estimates of polar bears and their tracks in the Chukchi Sea. PloS One 16 (5), e0251130. doi: 10.1371/journal.pone.0251130
Dalén L., Götherström A., Meijer T., Shapiro B. (2007). Recovery of DNA from footprints in the snow. Can. Field-Naturalist 121 (3), 321–324. doi: 10.22621/cfn.v121i3.482
Davoli F., Schmidt K., Kowalczyk R., Randi E. (2013). Hair snaring and molecular genetic identification for reconstructing the spatial structure of Eurasian lynx populations. Mamm. Biol. 78, 118–126. doi: 10.1016/j.mambio.2012.06.003
Doran-Myers D., Kenney A. J., Krebs C. J., Lamb C. T., Menzies A. K., Murray D., et al. (2021). Density estimates for Canada lynx vary among estimation methods. Ecosphere 12 (10), e03774. doi: 10.1002/ecs2.3774
Dugal L., Thomas L., Jensen M. R., Sigsgaard E. E., Simpson T., Jarman S., et al. (2022). Individual haplotyping of whale sharks from seawater environmental DNA. Mol. Ecol. Resour. 22 (1), 56–65. doi: 10.1111/1755-0998.13451
Ficetola G. F., Miaud C., Pompanon F., Taberlet P. (2008). Species detection using environmental DNA from water samples. Biol. Lett. 4 (4), 423–425. doi: 10.1098/rsbl.2008.0118
Franklin T. W., McKelvey K. S., Golding J. D., Mason D. H., Dysthe J. C., Pilgrim K. L., et al. (2019). Using environmental DNA methods to improve winter surveys for rare carnivores: DNA from snow and improved noninvasive techniques. Biol. Conserv. 229, 50–58. doi: 10.1016/j.biocon.2018.11.006
Garrett N. R., Watkins J., Simmons N. B., Fenton B., Maeda-Obregon A., Sanchez D. E., et al. (2023). Airborne eDNA documents a diverse and ecologically complex tropical bat and other mammal community. Environ. DNA. 5 (2), 350–362. doi: 10.1002/edn3.385
Guerrieri A., Bonin A., Münkemüller T., Gielly L., Thuiller W., Francesco Ficetola G. (2021). Effects of soil preservation for biodiversity monitoring using environmental DNA. Mol. Ecol. 30 (13), 3313–3325. doi: 10.1111/mec.15674
Harrison J. B., Sunday J. M., Rogers S. M. (2019). Predicting the fate of eDNA in the environment and implications for studying biodiversity. Proc. R. Soc. B 286 (1915), 20191409. doi: 10.1098/rspb.2019.1409
Hellström M., Wijkmark N., Edbom-Blomstrand C., Hellström P., Näslund J. (2019). Footsteps in the snow-Pilot study for future monitoring of individual lynx (Lynx lynx) from eDNA in snow tracks. AquaBiota Rep. 10.
Huvier N., Moyne G., Kaerle C., Mouzon-Moyne L. (2023). Time is running out: Microsatellite data predict the imminent extinction of the boreal lynx (Lynx lynx) in France. Front. Conserv. Sci. 4, 1080561. doi: 10.3389/fcosc.2023.1080561
Inskip C., Zimmermann A. (2009). Human-felid conflict: a review of patterns and priorities worldwide. Oryx 43 (1), 18–34. doi: 10.1017/S003060530899030X
Janečka J. E., Jackson R., Yuquang Z., Diqiang L., Munkhtsog B., Buckley-Beason V., et al. (2008). Population monitoring of snow leopards using noninvasive collection of scat samples: a pilot study. Anim. Conserv. 11 (5), 401–411. doi: 10.1111/j.1469-1795.2008.00195.x
Jensen E. L., Tschritter C., de Groot P. V., Hayward K. M., Branigan M., Dyck M., et al. (2020). Canadian polar bear population structure using genome-wide markers. Ecol. Evol. 10 (8), 3706–3714. doi: 10.1002/ece3.6159
Kelly R. P., Port J. A., Yamahara K. M., Crowder L. B. (2014). Using environmental DNA to census marine fishes in a large mesocosm. PloS One 9 (1), e86175. doi: 10.1371/journal.pone.0086175
Kinoshita G., Yonezawa S., Murakami S., Isagi Y. (2019). Environmental DNA collected from snow tracks is useful for identification of mammalian species. Zoological Sci. 36 (3), 198–207. doi: 10.2108/zs180172
Lefort M. C., Boyer S., Barun A., Khoyi A. E., Ridden J., Smith V. R., et al. (2015). Blood, sweat and tears: non-invasive vs. non-disruptive DNA sampling for experimental biology. PeerJ PrePrints 3, e655v3. doi: 10.7287/peerj.preprints.655v3
Li J., Weckworth B. V., McCarthy T. M., Liang X., Liu Y., Xing R., et al. (2020). Defining priorities for global snow leopard conservation landscapes. Biol. Conserv. 241, 108387. doi: 10.1016/j.biocon.2019.108387
Menotti-Raymond M., David V. A., Lyons L. A., Schäffer A. A., Tomlin J. F., Hutton M. K., et al. (1999). A genetic linkage map of microsatellites in the domestic cat (Felis catus). Genomics 57 (1), 9–23. doi: 10.1006/geno.1999.5743
Miller S. M., Harper C. K., Bloomer P., Hofmeyr J., Funston P. J. (2014). Evaluation of microsatellite markers for populations studies and forensic identification of African lions (Panthera leo). J. Heredity 105 (6), 856–866. doi: 10.1093/jhered/esu054
Pagano A. M., Peacock E., McKinney M. A. (2014). Remote biopsy darting and marking of polar bears. Mar. Mammal Sci. 30 (1), 169–183. doi: 10.1111/mms.12029
PBSG (2021). Status report on the world’s polar bear subpopulations (Gland, Switzerland: IUCN: IUCN/SSC Polar Bear Specialist Group), 1–52.
Pedersen M. W., Overballe-Petersen S., Ermini L., Sarkissian C. D., Haile J., Hellstrom M., et al. (2015). Ancient and modern environmental DNA. Philos. Trans. R. Soc. B: Biol. Sci. 370 (1660), 20130383. doi: 10.1098/rstb.2013.0383
Piggott M. P., Taylor A. C. (2003). Remote collection of animal DNA and its applications in conservation management and understanding the population biology of rare and cryptic species. Wildlife Res. 30 (1), pp.1–pp13. doi: 10.1071/WR02077
Sigsgaard E. E., Nielsen I. B., Bach S. S., Lorenzen E. D., Robinson D. P., Knudsen S. W., et al. (2017). Population characteristics of a large whale shark aggregation inferred from seawater environmental DNA. Nat. Ecol. Evol. 1 (1), 0004. doi: 10.1038/s41559-016-0004
Smouse R. P. P., Peakall R. (2012). GenAlEx 6.5: genetic analysis in Excel. Population genetic software for teaching and research—an update. Bioinformatics 28 (19), 2537–2539.
Spens J., Evans A. R., Halfmaerten D., Knudsen S. W., Sengupta M. E., Mak S. S., et al. (2017). Comparison of capture and storage methods for aqueous macrobial eDNA using an optimized extraction protocol: advantage of enclosed filter. Methods Ecol. Evol. 8 (5), pp.635–pp.645. doi: 10.1111/2041-210X.12683
Taberlet P., Luikart G. (1999). Non-invasive genetic sampling and individual identification. Biol. J. Linn. Soc. 68 (1-2), pp.41–pp.55. doi: 10.1111/j.1095-8312.1999.tb01157.x
Taberlet P., Coissac E., Hajibabaei M., Rieseberg L. H. (2012). Environmental DNA. Mol. Ecol. 21 (8), 1789–1793. doi: 10.1111/j.1365-294X.2012.05542.x
Thomsen P. F., Willerslev E. (2015). Environmental DNA–an emerging tool in conservation for monitoring past and present biodiversity. Biol. Conserv. 183, 4–18. doi: 10.1016/j.biocon.2014.11.019
Ulizio T. J., Squires J. R., Pletscher D. H., Schwartz M. K., Claar J. J., Ruggiero L. F. (2006). The efficacy of obtaining genetic-based identifications from putative wolverine snow tracks. Wildlife Soc. Bull. 34 (5), 1326–1332. doi: 10.2193/0091-7648(2006)34[1326:TEOOGI]2.0.CO;2
von Arx M. (2020). “Lynx lynx (Europe assessment) (amended version of 2018 assessment),” in The IUCN Red List of Threatened Species 2020, e.T12519A177350310. doi: 10.2305/IUCN.UK.2020-3.RLTS.T12519A177350310.en
Vongraven D., Aars J., Amstrup S., Atkinson S. N., Belikov S., Born E. W., et al. (2012). A circumpolar monitoring framework for polar bears. Ursus 23 (sp2), pp.1–pp66. doi: 10.2192/URSUS-D-11-00026.1
Wong P. B. Y., de Groot P. V. C., Fekken C., Smith H., Pagès M., Boag P. T. (2011). Interpretations of polar bear (Ursus maritimus) tracks by Inuit hunters: inter-rater reliability and inferences concerning accuracy. Can. Field-Naturalist 125 (2), 140–153. doi: 10.22621/cfn.v125i2.1197
Keywords: nuclear eDNA, snow, snow track, individual, polar bear (Ursus maritimus), Eurasian lynx (Lynx lynx), snow leopard (Panthera uncia), sampling protocol
Citation: Hellström M, Kruger E, Näslund J, Bisther M, Edlund A, Hernvall P, Birgersson V, Augusto R and Lancaster ML (2023) Capturing environmental DNA in snow tracks of polar bear, Eurasian lynx and snow leopard towards individual identification. Front. Conserv. Sci. 4:1250996. doi: 10.3389/fcosc.2023.1250996
Received: 30 June 2023; Accepted: 11 October 2023;
Published: 04 December 2023.
Edited by:
Arne Ludwig, Leibniz Institute for Zoo and Wildlife Research (LG), GermanyReviewed by:
Nicholas W. Pilfold, San Diego Zoo Institute for Conservation Research, United StatesLaura Iacolina, University of Primorska, Slovenia
Copyright © 2023 Hellström, Kruger, Näslund, Bisther, Edlund, Hernvall, Birgersson, Augusto and Lancaster. This is an open-access article distributed under the terms of the Creative Commons Attribution License (CC BY). The use, distribution or reproduction in other forums is permitted, provided the original author(s) and the copyright owner(s) are credited and that the original publication in this journal is cited, in accordance with accepted academic practice. No use, distribution or reproduction is permitted which does not comply with these terms.
*Correspondence: Melanie L. Lancaster, melanie.lancaster@wwf.se; Micaela Hellström, micaela@mixresearch.se
†Present address: Anna Edlund, OATH, Inc., Mill Valley, CA, United States