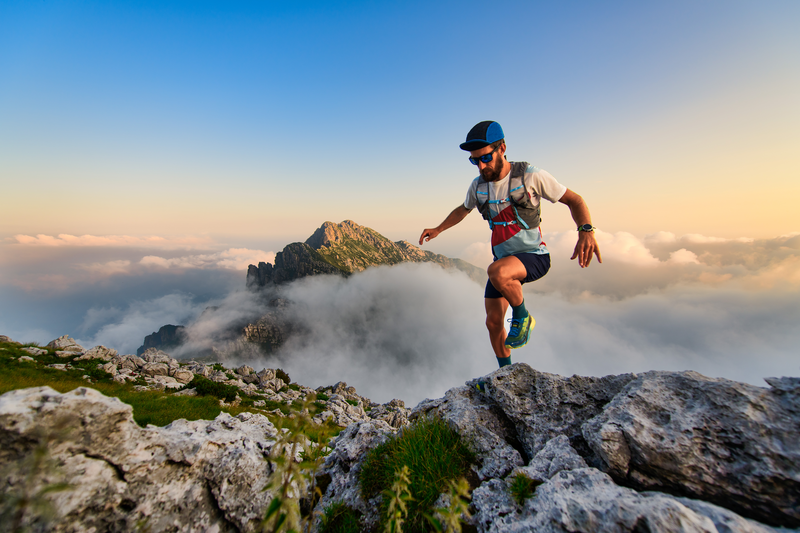
94% of researchers rate our articles as excellent or good
Learn more about the work of our research integrity team to safeguard the quality of each article we publish.
Find out more
ORIGINAL RESEARCH article
Front. Conserv. Sci. , 29 June 2023
Sec. Animal Conservation
Volume 4 - 2023 | https://doi.org/10.3389/fcosc.2023.1226658
Introduction: Breeding-site fidelity can occur at various spatial scales and can vary in strength across these scales. Understanding this variation, and rules governing individual site-fidelity decisions, can have important implications for the conservation and management of threatened species. Globally, amphibians are in rapid decline and breeding-site fidelity appears to be widespread, yet few studies have investigated patterns of lifetime breeding-site fidelity, and no studies have explored decision-making rules in situ.
Methods: We investigated lifetime patterns of breeding site fidelity in the brown toadlet Pseudophryne bibronii, a species displaying population declines and local extinction throughout its range. We monitored a single population for a period spanning 10 years to establish the extent that males express site fidelity at the scale of the breeding site and the breeding patch. We also examined male nest-site fidelity between breeding years in relation to mating success to examine if toadlets follow a Win-stay/Lose shift strategy.
Results: Overall, we found that males displayed extreme lifetime fidelity to specific breeding patches within the breeding site, but that males regularly moved nest-site locations between breeding years. The degree of nest-site fidelity was related to male-mating success, whereby successful males established nests closer to a previous years’ nest location than unsuccessful males.
Discussion: Our findings suggest that brown toadlets display extreme site fidelity at the scale of the breeding patch, but that within patches male nesting decisions are flexible and follow a win-stay/lose-shift strategy. These results provide novel evidence that breeding site fidelity in amphibians can vary depending on spatial scale (indicative of scale-dependent information use) and that a rule-based learning strategy can influence the degree of nest-site fidelity. Breeding patch fidelity and capacity for spatial learning may be widespread in long-lived amphibians and necessitate in situ conservation strategies that protect known breeding patches (and adjacent habitat) whilst enabling unconstrained localised movement.
Breeding-site fidelity, the behavioural propensity for iteroparous animals to repeatedly use distinct geographic locations for breeding, is ubiquitous across taxa with examples spanning both invertebrate and vertebrate phyla (mollusca, arthropoda, chordata) (Gerber et al., 2019; Merkle et al., 2022). As the number of examples of breeding site fidelity grows there is increasing recognition that the phenomenon can occur at various spatial scales. In some species, individuals return to general breeding grounds and remain faithful to sites where they completed early development (natal site fidelity) or to profitable sites encountered following dispersal (Switzer, 1993; Piper, 2011; Merkle et al., 2022). In other species, however, breeding site fidelity occurs at much finer scales, with reports of individuals returning to breed at specific breeding patches withing breeding sites, or even to specific points within a breeding patch (Schaefer et al., 2000). For instance, in oviparous species it is not uncommon for members of one or both sexes to re-use the same nest sites between breeding seasons (nest-site fidelity) (Sonerud, 2021).
In addition to species displaying variation in breeding-site fidelity at different spatial scales, species can vary considerably in the strength of breeding site fidelity. At one extreme, species may be behaviourally rigid and constrained to breeding at the same site every breeding season (Merkle et al., 2022). At the other extreme, species may be behaviourally flexible, with individuals deciding to move between breeding sites, breeding patches or nest sites within or between breeding seasons (Beever et al., 2017). This flexibility indicates an ability to make informed decisions and has motivated research into decision-making strategies and rules (Piper, 2011). Decisions to return to breeding sites, patches or nest sites may be critically dependent on past experience. One decision making rule that has drawn considerable research attention is the win-stay lose-shift rule. The rule postulates that the attraction of animals to sites is maintained until those sites fail to yield successful outcomes (Switzer, 1993; Schmidt, 2004). Specifically, it is hypothesised that individuals should be faithful to breeding sites if the site yields reproductive success and fitness benefits (win stay), but endeavour to improve breeding outcomes in the next breeding cycle if reproductive outcomes at the site are suboptimal (lose switch) (Schmidt et al., 2010; Gerber et al., 2019). The adaptive benefit of the strategy hinges on the quality of breeding habitat being temporally autocorrelated (i.e. predicable) across breeding seasons, and decisions to stay or leave maximising reproductive success (Switzer, 1993; Schmidt et al., 2010; Gerber et al., 2019). While there is widespread evidence for a win-stay lose-shift strategy influencing site fidelity in birds and mammals (Schmidt, 2004; Campomizzi et al., 2012; Morrison et al., 2021), evidence in lower vertebrates remains limited to a small number of fish species (Bartlett et al., 2010). With the objective of better understanding the generality of decision-making rules governing breeding-site fidelity in animals, studies exploring links between individual-breeding success and movement behaviour will be needed for a greater diversity of taxa.
Variation in the scale and strength of breeding site fidelity can have profound ecological and evolutionary consequences. Mounting evidence points towards strong effects of breeding-site fidelity on demography, population structure, and trophic interactions (Schmidt, 2004; Bauer and Hoye, 2014). In turn, breeding site fidelity can influence the genetic composition of populations, standing levels of genetic variation, the likelihood of genetic divergence and the risk of inbreeding and outbreeding depression (Sugg et al., 1996; Gerber et al., 2019; Byrne and Silla, 2022). Tied to increased knowledge about these ecological and evolutionary consequences, there has been a rapidly growing interest in the conservation implications of breeding-site fidelity. A major concern is that species with strong breeding site fidelity and weak behavioural flexibility may be susceptible to ‘fidelity-induced ecological traps’, whereby strong faithfulness to a site confers lower fitness outcomes following rapid environmental change (Merkle et al., 2015; Merkle et al., 2022). Fuelling this concern, are increased reports across various migratory mammal, bird and fish species that individuals continue to return to breeding sites even following significant experimental or human induced reductions in site quality (Wiens et al., 1986; Liermann et al., 2012; Merkle et al., 2015; Wyckoff et al., 2018; Merkle et al., 2022). For these and other groups with limited movement plasticity there may be a heightened risk of population decline and local extinction (Beever et al., 2017; Merkle et al., 2022). With this in mind, gathering information about the extent of behavioural flexibility at different spatial scales (e.g. breeding site, breeding patch, nest site) and associated decision making strategies may help inform and optimise management approaches for threatened species.
One taxonomic group where long-term studies into breeding site fidelity and the capacity for behavioural flexibility are urgently needed is amphibians. Globally, amphibians are declining faster than any other vertebrate group with an estimated 41% of species currently listed as threatened with extinction (Scheele et al., 2019). A diversity of amphibians are known to display strong breeding-site fidelity, with mark-recapture studies revealing repeated use of breeding sites such as ponds and creeks between breeding seasons (Landler, 2022). For certain species, there is also evidence for fine-scale homing abilities, with displaced individuals showing a remarkable ability to return to familiar sites (Shaykevich et al., 2022). Compared to mammals and birds, it has long been thought that amphibians lack behavioural flexibility and the capacity for spatial learning, but this view is rapidly changing (Liu et al., 2016). There are now reports that amphibians can solve mazes and correctly orient during displacement tests using memorised motor strategies (i.e. learning to turn left or right) or by learning to use static visual, olfactory, geometric or magnetic cues (Crane and Mathis, 2011; Daneri et al., 2011; Sotelo et al., 2015; Liu et al., 2016). There is also some recent evidence for complex spatial cognition. Laboratory based learning experiments in a poison frog have demonstrated that individuals use visual cues for spatial discrimination and employ a rule-based learning strategy (win-stay/lose shift) to modify their visual associations in reversal tasks with changing reward contingencies (Liu et al., 2016). Despite this knowledge, for the vast majority of amphibian species there remains no knowledge of lifetime breeding-site fidelity, the scale of fidelity within breeding sites, or the capacity for behavioural flexibility. For threatened species, this knowledge could be vital for predicting responses to environmental change and planning conservation responses (Joly, 2019).
In the present study we perform a mark recapture study spanning a ten-year period for an entire population of the Australian brown toadlet Pseudophryne bibronii. Our aims were to; 1) gain preliminary insights into patterns of site fidelity at different spatial scales (breeding site, breeding patch and nest site) and 2) explore relationships between individual mating success and the degree of nest-site fidelity between breeding years. Brown toadlets have a terrestrial mode of reproduction that involves males building nests (shallow burrows) in soil underneath leaf litter in dry creek lines and drainage pans (Byrne and Keogh, 2009). In a previous displacement study investigating re-settlement patterns within a breeding season we showed that 95% (38/40) of males returned to their breeding patch, but only 20% of males returned to within one metre of the original nest site, and only 0.08% (3/40) of males resettled on their original nest site (Heap et al., 2015). Based on these findings we made two main predictions for the present study. First, we predicted a high proportion of toadlets would return to the breeding site each year and that across breeding years site fidelity would be stronger at the scale of the breeding patch than the nest site. Second, we predicted that if nest site fidelity is conditional on past mating success that between breeding years males would return closer to a nest site if they achieved mating success in the previous year (in line with a win-stay/lose Shift strategy).
The brown toadlet (Pseudophryne bibronii) is a small Myobatrachid frog endemic to temperate regions of south-eastern Australia. The species is characterised by a terrestrial mode of reproduction, laying eggs in shallow soil depressions underneath leaf litter, vegetation, and woody debris (Woodruff, 1977) (Figure 1). Breeding occurs between March and June (Austral autumn-early winter) and is restricted to ephemeral waterbodies that retain freshwater pools after heavy rainfall, allowing for an aquatic larval phase (Pengilley, 1971; Woodruff, 1977). After the first significant autumn rains (typically in March or April), males move into breeding sites from adjacent woodland and establish nests. Males use two distinct call types to advertise nests to females and defend territories from rivals (Byrne, 2008). After males have established territories, gravid (egg carrying) females enter breeding sites after rainfall events (correlated with increased calling activity) and routinely spend several days assessing multiple males/nests before mating (Byrne and Keogh, 2009). On average, females deposit 47 eggs (range = 26–78), with eggs split between the nests of up to eight males (Byrne and Keogh, 2009). Tadpoles develop within egg capsules and enter a state of suspended development until nests are flooded and hypoxic conditions trigger hatching (Bradford and Seymour, 1988). Larval development occurs in shallow ephemeral pools over winter (June-August) with metamorphosis occurring in early- to mid- spring (September, October). Breeding finishes when a breeding site becomes inundated, at which point toadlets move back into surrounding bushland, where they seek refuge under leaflitter, rocks and logs.
Figure 1 (A) Adult male brown toadlet Pseudophryne bibronii guarding a nest with eggs. (B) Terrestrial nest sites were marked with individually numbered reflective plastic tags. Photographs courtesy of AS.
The study took place in a population of brown toadlets located in Wrights Beach in the Jervis Bay region of NSW, Australia. The study site was defined by the intersection of two ephemeral creek lines (one running in a north-south direction and the other in an east-west direction), which created three distinct breeding patches used by toadlets (Figure 2). In a previous study these patches were defined as the north patch, the east patch and the south patch (hereafter referred to as north creek, east creek and south creek). A granite rocky outcrop at the point where the two creeks intersected provided a clear boundary between the three breeding patches as no toadlets could nest in this zone (Heap et al., 2015). Over a ten-year period (2004-2015) we collected all males in the population in 8 survey years (2004, 2005, 2008, 2010, 2012, 2013, 2014, 2015). The type of data collected differed between survey years. In the first two survey years (2004 and 2005) the breeding site was surrounded with drift fences and pit traps to capture all toadlets entering the breeding site prior to nesting, as part of a study on polyandrous mating behaviour (Byrne and Keogh, 2009). In the following survey years (2008, 2010, 2012, 2013, 2014, 2015) toadlets were allowed to freely enter the site. In each of the eight survey years, the precise location of male nests was determined by pinpointing calls using a directional microphone (NTG2, Rode Microphones, Sydney, Australia) connected to a Marantz Professional handheld solid-state recorder (Model No. PMD661MKII, D&M Holdings Inc. Tokyo, Japan). In the first two survey years (2004, 2005) nest locations were determined during nightly monitoring throughout the breeding season. In the following survey years (2008, 2010, 2012, 2013, 2014, 2015) nests were located at the end of the breeding season before heavy rainfall inundated the site. During these survey years, the site was visited between dusk and dawn over four consecutive nights. Collection of males took place over 1-3 nights (2004: May 23-25; 2005: June 9-11; 2008: April 30-May 2; 2010: May 17-19; 2012: May 23; 2013: May 22-23; 2014: May 29-31; 2015: May 6). How quickly males were marked and captured was influenced by how consistently males were calling. Males were systematically collected from sections of each breeding patch, and to ensure that no males were missed each section was re-visited multiple times during the collection night, as well as the following night. At the time of male collection, nests were marked with an individually numbered plastic ID tag (Figure 1) and males were caught by hand. In 2004 and 2014 ID tags were not collected so that we could calculate the distance that nesting males moved between breeding years. Males were held in plastic zip lock bags and transported to a field station located approximately 1km from the study site. On the day after collection males were weighed and photographed, then released back at their site of capture after nightfall (between 4 and 5 am). When any male was first caught, they were also toe clipped. While the dorsal colouration of brown toadlets is largely monomorphic, each individual has a unique ventral pattern that remains static throughout adult life (Figure 3). As such, annual photographs were used to identify individuals between survey years, with photographic identification validated against the toe clip records. Using this method we tracked the 2004 cohort over the study period to establish the proportion of males that returned to the breeding site in each survey year, and how breeding patches were used for nesting by individual males over their lifetime. In addition, for all the males caught at the breeding site over the entire study period, we established the incidence of recapture and the number of breeding patches used for nesting.
Figure 2 Schematic of the breeding site showing the approximate orientation and length of the three breeding patches: north creek, east creek, and south creek.
Figure 3 (A) Image showing an adult male brown toadlet with typical dorsal colouration. (B). A representative selection of images showing the unique ventral patterns of individuals which can be used to accurately identify individual males throughout adulthood. Photographs courtesy of AS.
To assess the relationship between a male’s past breeding experience and variation in nest -site fidelity we mapped the nest site locations of breeding males between two consecutive breeding years (2004 and 2005) and recorded male-mating success at the end of the first breeding season. This study was then repeated in 2014 and 2015, to provide a temporal replicate that allowed us to investigate whether there was temporal consistency in the nesting strategy expressed by males. Nests were marked by placing reflective plastic ID tags (Figure 1) in soil 2cm to the right of the edge of the nest, which was a depression in soil underneath leaf litter, rocks, or logs or at the base of reeds. After males were captured at the end of the breeding season, we immediately searched nests for eggs and scored each male as ‘mated’ or ‘unmated’.
We described recapture patterns for the 2004 male cohort by calculating the percentage of males recaptured one or more times in each of the subsequent study years (2005, 2008, 2010, 2012, 2013, 2014 and 2015). We calculated the mean ± standard error mean (SEM) number of years that males were re-captured and the mean (± SEM) number of actual years that males were present at the study site. We also calculated the percentage of males that nested in each of the three breeding patches (north creek, south creek, or east creek) during the study period. To explore the expression of site fidelity for the 2004 cohort of males, as well as all males captured during the study period, we used binomial probability tests to determine if the proportion of males re-captured at the scale of the breeding site and the breeding patch was higher than expected by chance. All binomial tests were one tailed with the specified proportion being 0.5 (to reflect an equal chance of males being re-captured at the breeding site versus another site, or in one breeding patch versus other patches), with significance set at p < 0.05. To test for a win stay/lose shift strategy of nest-site selection between breeding years we used linear mixed effects models fitted with restricted maximum likelihood (REML). For the analyses, mating success in the first year of capture (year 1) was included as a fixed categorical effect. To account for repeated observations of the same males over multiple years, we included male ID as a random effect. Two separate models were run for the 2004-2005 data set and the 2014-2015 data set. All statistical analyses were performed using JMP Pro 16 (SAS Institute Inc. North Carolina, USA).
All activities outlined in the present study were approved by the Australian National University Animal Experimentation Ethics Committee (Protocol No. F.BTZ.64.04), the Monash University Animal Ethics Committee (BSCI/2007/14), and the University of Wollongong’s Animal Ethics Committee (AE1208, AE14/17) and were authorised by New South Wales National Parks & Wildlife Service (SI2552, S11005, SL101436).
A total of 43 nesting males were captured in the first year of the study (2004). For this cohort, 72.09% (31/43) of males were recaptured one or more times in the following seven survey years, spanning a ten-year period. The percentage of frogs recaptured progressively declined over the entire study period (Table 1). The number of survey years in which males were captured ranged from 1 to 7 (mean ± SEM = 2.277 ± 0.195), with the actual number of years that males were present at the breeding site ranging from 1 to 12 years (mean ± SEM = 3.418 ± 0.495). Assuming that males were a minimum one year old when they first entered the breeding site, a small percentage of the 2004 cohort (6.97%, 3/43) were at least 13 years old when the study concluded in 2015. Of the 2004 males recaptured at the breeding site, 70.96% (22/31) only nested in one of the three breeding patches (north creek, south creek, or east creek) during the study period. The percentage of males that nested in two breeding patches was 25.80% (8/31), and only male (1/31) nested in all three breeding patches. The proportion of males that showed fidelity to a single breeding patch was significantly higher than expected by chance (binomial test: z = 2.15, p = 0.015). Over the entire study period, a total of 193 frogs were caught (including the 2004 cohort and new recruits each survey year). The percentage of frogs recaptured in each survey year ranged between 35.89% and 62.5% (see Table 2). Excluding new males (n = 30) entering the site for the first time in 2015 (as these males were only sampled in one year), 60.73% of males (99/163) were recaptured at least once during the study period. This incidence of recapture is higher than expected by chance (binomial test: z = 2.66, p = 0.0038). For all of the males recaptured during the study period (n = 99), 75.75% (75/99) nested in a single breeding patch, 23.23% (23/99) nested in two patches and 1.01% (1/99) nested in all three patches. The proportion of males restricting nesting to a single patch was significantly higher than expected by chance (binomial test: z = 5.025, p < 0.000001).
Table 1 The number and percentage of the 2004 cohort of male brown toadlets (n=43) recaptured in a single breeding site in seven survey years between 2005 and 2015.
Table 2 The number of male brown toadlets captured, and the number and percentage of toadlets recaptured, from a single breeding site in eight survey years between 2004 and 2015.
In 2005, recaptured males from 2004 (n = 31) nested on average (± SEM) 5.376 ± 1.307 metres from the nest site they used in 2004 (range = 0-23 metres). Whether males gained mating success in 2004 had an influence on how far away they nested the following year. Males that gained mating success in 2004 (14/31, 46.66%) nested more than four times closer to their 2005 nest compared to males that failed to gain mating success (17/31, 54.84%) (Figure 4A). The effect of mating success on distance between nest sites over successive breeding years was highly significant (LME: F1,29= 6.808, p = 0.0142). A total of four males (all of which gained mating success in 2004) returned to the exact same nest site.
Figure 4 (A) Relationship between male-mating success in the 2004 breeding season and distance between a male’s nest in 2004 and his nest in 2005 (n = 31 males). (B) Relationship between male-mating success in the 2014 breeding season and distance between a male’s nest in 2014 and his nest in 2015 (n = 28 males). Data shown are untransformed means (± sem).
In 2015, recaptured males from 2014 (n = 28) nested on average (± SEM) 1.857± 0.379 metres from the nest site they used in 2014 (range = 0-6.60 metres). Similar to the 2004/2005 data set, whether or not males gained mating success in the first year had an influence on how far away a male constructed a nest the following year. Males that gained mating success in 2014 (11/28, 39.28%) nested more than five times closer to their 2015 nest than males that failed to gain mating success (17/28, 60.71%) (Figure 4B). The effect of mating success on distance between nest sites was highly significant (LME: F1,26 = 13.019, p = 0.0013). A total of seven males (five of which gained mating success in 2004) returned to the exact same nest site.
Our findings show that brown toadlets display extreme lifetime site fidelity at the scale of the breeding patch, but that within patches male nesting decisions are flexible and follow a win-stay/lose-shift strategy. These findings suggest scale-dependent information use as well as rule based-decision making and have important ecological, evolutionary and conservation implications.
The high level of site fidelity at the patch level may have several explanations. One possibility is that toadlets show adaptive movement towards essential breeding resources (Krebs, 1971). If these resources are spatially heterogenous in the landscape, yet temporally predictable, site fidelity could be the outcome of individuals revisiting high-quality sites and avoiding poor-quality sites (Riotte-Lambert and Matthiopoulos, 2020). For toadlets, there are very specific requirements for terrestrial breeding and a certain level of predictability in hydric, lighting, and thermal conditions is essential for reproductive success. Specifically, toadlets require an ephemeral drainage line/pan that is dry throughout summer and early autumn and then floods in late autumn to early winter to produce shallow pools with suitable light and thermal regimes to support larval development over winter and early spring. Sites with these characteristics are widely dispersed in the landscape. Therefore, once toadlets encounter suitable breeding sites, attraction to familiar sites could strengthen breeding site fidelity. Familiarity with suitable breeding sites may yield considerable benefits, including improved efficiency at exploiting food resources (Van Moorter et al., 2009), more effective predation response (Piper, 2011), and improved dominance interactions between territorial males (Kokko et al., 2006). These benefits may outweigh any benefits of exploring for new sites that may be difficult to locate and of potentially lower quality (Piper, 2011; Morrison et al., 2021), especially when considering costs of exploration, such as elevated risk of predation (Metzgab, 1967) and energetic and time expense for movement and habitat sampling (Stamps, 1995).
Fidelity to breeding patches in toadlets may be reinforced through natal imprinting and natal homing (returning to breed at the site of egg deposition or hatching). There are two lines of evidence to suggest that toadlets possess homing abilities. First, in a previous study we swapped males in our study population between breeding patches and they almost all (95%) returned to their original patch within a matter of weeks (Heap et al., 2015). Second, during a manipulative field study two females from our study population were released at a population approximately 400m away (separated by thick bush with no drainage lines connecting the sites) and were recaptured at the original site twenty days and twenty two days later. Recapture data from the 2004 and 2005 breeding seasons (when pit traps were used) also indicates that a high percentage of females return to the same breeding site between years. Of the females captured at the breeding site in 2004 (n = 35), 42.85% (15/35) were recaptured during the 2005 breeding season. Together, this information indicates that both sexes may have sophisticated homing abilities. While the potential for natal imprinting and natal homing has been reported in various amphibian species, Gamble et al., 2007) (Shaykevich et al., 2022), the mechanisms underpinning natal homing are not well understood/One hypothesis is that amphibians imprint on magnetic fields unique to the natal area (geomagnetic imprinting) and then use magnetoreception to navigate home. Another hypothesis is that larvae imprint on the chemical signature of their natal site (olfactory imprinting) and rely on chemical cues and olfactory senses for navigation. (Ogurtsov, 2003; Shakhparonov and Ogurtsov, 2003; Landler and Gollmann, 2011). Moving forward, in an effort to understand the causes of site fidelity in toadlets, tracking studies testing for natal homing ability and associated homing mechanisms, coupled with an assessment of the genetic relatedness of breeding frogs, would be a valuable next step.
To some extent, inter-annual decisions to remain at the breeding patch (and the strength of patch fidelity) may also be influenced by prior experience and private information. Toadlets may remain faithful to a breeding patch until it yields unsuccessful reproductive outcomes. However, because so few toadlets (approximately 29%) left a breeding patch over their lifetime it seems that site fidelity at this scale is controlled by a different mechanism. One possibility is that toadlets use public information to assess whether a breeding patch is profitable. The ‘public information strategy’ argues that individuals vicariously acquire information on the reproductive success of conspecifics and make site-fidelity decisions based on the average breeding success of the population (Campomizzi et al., 2012). While this strategy is yet to be examined in amphibians, there is observational evidence that it influences breeding decisions in various bird species. (Danchin et al., 1998). Although toadlets breed in concealed nests, males may assess the breeding activity and success of conspecifics using acoustic cues. When interacting with females, male toadlets significantly increase their calling effort and switch to distinctive courtship calls immediately prior to amplexus and oviposition (Byrne, 2008). It is conceivable that males could use these cues to assess the average level of mating activity in a breeding patch. In addition, because frogs have the ability to visually observe tadpoles in pools and make movement decisions based on this information (Ringler et al., 2018), it is possible that males assess the total reproductive output for a patch by inspecting breeding pools at the end of a breeding season.
At the scale of the nest site, our findings provide evidence to support a win-stay/lose shift strategy of nest-site selection. After occupying a profitable nest, males may be more responsive to visual or chemical cues associated with that nest and be drawn closer to that site the following year. This strategy could yield significant fitness benefits if previously successful nests (or nesting areas) are more likely to be successful in the future. However, it must be recognised that not all males adhered to the rule. Some males achieved mating success yet moved nest location the following year, while others gained no mating success and returned to the same nest. This variation suggests that individuals may be adjusting their breeding strategy based on other sources of information not collected in the present study. One possibility is that toadlets rely on information gathered across multiple years to make nesting decisions. This seems feasible given our finding that males are relatively long lived and can frequent the same breeding patch for twelve years or more. Another possibility is that toadlets use combinations of private and public information to assess breeding success. This capacity has been demonstrated in the great cormorant, whereby nest site selection is influenced by an individuals’ own success as well as that of their nearest neighbours (Schjørring et al., 2000). An alternative explanation is that nesting decisions are context dependent. For instance, the risk of nest disturbance or nest predation in one year might influence nesting decisions the following year. We have no data on this for toadlets, but effects of predation have been reported in various bird species (Sonerud, 2021).
Variation in the strength of male nest-site fidelity might also be explained by fluctuations in the density of males within a breeding patch. In a previous displacement study where males were forced to resettle as a group, and breeding density was artificially inflated, we found that male nesting behaviour was sensitive to the proximity of conspecifics (Heap et al., 2015). As local density within a patch increased, the probability that a male remained at a nest site significantly decreased, with no significant influence of a male’s mating status prior to displacement on nest location after re-settlement (Heap et al., 2015). While we did not measure density in the present study, it is certainly possible that local shifts in density between years could influence the frequency of agonistic interactions, the costs of territory defence and the strength of nest-site fidelity. We know that territorial calling in toadlets facilitates the establishment and maintenance of nest-site boundaries. We also know that territorial calling increases significantly and can last several hours (or even days) when intruding males attempt nest takeover, representing a form of endurance rivalry (O'Brien et al., 2018). If density is higher than a previous year (for instance if there are more first-time breeders attempting to settle), elevated costs of nest defence may force prior residents to settle away from preferred nest sites. Of note, male toadlets also produce biologically active skin alkaloids (pseudophrynamines and pumiliotoxins) which appear to play a role in marking nests and mediating male-male competition (Mitchell, 2005; Byrne and Keogh, 2007). Inter-individual differences in concentrations of these compounds might influence nesting decisions in a state-dependent fashion, as reported for a salamander (Bucciarelli et al., 2016). Importantly, such state-dependent decision making might also arise due to variation in other phenotypic traits, such as body size or body condition (Kopecký et al., 2010). Investigating whether toadlets rely on combinations of information from multiple sources to modify their nesting decisions, and/or whether decisions are context- or state dependent, could help explain movement patterns of males within breeding sites between breeding years (Chalfoun and Martin, 2010).
The novel evidence we provide for lifetime breeding site fidelity in brown toadlets sheds new light on toadlet ecology and evolution. Previously, anecdotal observations of brown toadlet nesting behaviour have suggested that males return to the same breeding patch between years (Mitchell, 2005). These early observations were made in a population towards the western boundary of the species distribution. That we report breeding site fidelity in a population located towards the eastern boundary of the distribution (separated by more than 1,200km) provides evidence that breeding site fidelity is a widespread behavioural trait in brown toadlets. Theoretically, for species with strong breeding site fidelity we should expect reduced gene flow between populations, high levels of genetic structuring, local adaptation and an increased risk of outbreeding depression (Sugg et al., 1996; Beebee, 2005; Gerber et al., 2019; Byrne and Silla, 2022). A growing body of evidence points towards these exact outcomes for brown toadlets. Phylogenetic studies have shown very high levels of genetic divergence between populations over very small geographical scales (Donnellan, S.C. unpublished data). In addition, quantitative-genetic breeding experiments have revealed high levels of genetic incompatibility between toadlet pairs from neighbouring populations (Byrne and Silla, 2020). This level of fitness reduction is highly suggestive of genetic incompatibility between individuals from different populations and outbreeding depression, which typically results from the breakup of co-adapted gene complexes found in locally-adapted populations (Byrne and Silla, 2022). In principle, once neighbouring populations become evolutionary distinct, outbreeding depression has the potential to drive further genetic divergence by selectively favouring reproduction between close relatives. In line with this notion, a population-wide genomic study investigating patterns of mate choice in red backed toadlets, a sister species to brown toadlets, recently revealed that females prefer to mate with more closely-related males (O’Brien et al., 2019). Red backed toadlets and brown toadlets share a similar reproductive ecology, so we predict that brown toadlets will also display preferential inbreeding.
Throughout their range, brown toadlets are showing signs of population decline thought to be linked to habitat destruction, infectious disease caused by the amphibian chytrid fungus, and altered fire and flood regimes linked to climate change (Scheele et al., 2019). Given these threats, our findings raise concerns that toadlets may be susceptible to a ‘fidelity induced ecological trap’, whereby faithfulness to a breeding site confers lower fitness outcomes following rapid environmental change (Merkle et al., 2022). Forecasting this outcome, specific management actions may be required to protect threatened populations. At present, a recommended approach for conserving species with strong breeding-site fidelity is to prioritise the protection of known breeding areas with a view towards mitigating threatening processes (Merkle et al., 2022). Given the extreme level of site fidelity and fine-scale genetic structuring evident in brown toadlets, preserving breeding habitat seems logical. However, because brown toadlets move into surrounding bushland between breeding seasons, protection zones will need to encompass areas larger than breeding sites alone. Within protected areas, we recommend management actions that permit unconstrained local movement of individuals. Our finding that males follow a win-stay/lose-shift strategy indicates that individuals may have sufficient cognitive ability and behavioural flexibility to express adaptive spatial responses to fluctuating conditions at a local scale. This ability to learn may be critical for dealing with mild to moderate levels of change within breeding patches, especially given that natural selection is unlikely to keep pace with rapid environmental change.
In instances where conserving toadlet populations in situ is not an option, attention may turn towards translocation and/or captive breeding and re-introduction as alterative management options. However, implementing such actions may require a nuanced approach to overcome issues linked to extreme breeding site fidelity. In the case of translocation, there is a risk that individuals might leave foreign sites in an effort to return to familiar habitats (Bell et al., 2010). There are potential methods to address this issue. For instance, practitioners could try to acclimate individuals to translocation sites using a soft release approach, familiarise individuals with cues from the release sites prior to release, simulate breeding choruses using playbacks to connect frogs to new sites, or select release sites with habitats similar to threatened sites (Berger-Tal et al., 2020). However, these methods may prove futile if animals have imprinted on the natal site. In the case of captive breeding and reintroduction, behavioural problems might arise if juveniles imprint on captive environments. If so, egg clutches generated in captivity might need to be reared at the release site soon after fertilisation or hatching to facilitate imprinting on local cues. To inform and direct such activities, experimental work will be essential to identify whether imprinting occurs, whether imprinting occurs during a critical period of development, and the type of imprinting mechanism in operation. Such work will facilitate a much-needed integration of ecological and evolutionary theory into amphibian conservation practice (Joly, 2019; Berger-Tal et al., 2020).
Although breeding-site fidelity has been reported for a diversity of amphibians, there remains a dearth of information regarding lifetime breeding-site fidelity for long-lived species. Our findings provide new evidence that amphibians can remain connected to specific breeding sites for many years, spanning multiple overlapping generations. Our findings are also congruous with emerging evidence that a large diversity of amphibians display fine-scale genetic structuring (Beebee, 2005). This alignment suggests that high breeding site fidelity may provide a universal proxy for demographic isolation and population genetic structure in this vertebrate class. More broadly, our findings shed new light on amphibian cognition and rule-based learning. A long-standing belief has been that amphibians lack behavioural flexibility and advanced cognitive abilities, but this view is rapidly changing. Experimental work has demonstrated that amphibians have the ability to solve mazes and correctly orient during displacement tests (either by using memorised motor strategies or by learning to use static cues) (Crane and Mathis, 2011; Daneri et al., 2011; Sotelo et al., 2015; Liu et al., 2016). Moreover, recent laboratory-based learning experiments in a poison frog have demonstrated that anurans can use visual cues for spatial discrimination and employ a rule-based learning strategy (win-stay/lose shift) to modify their visual associations in reversal tasks with changing reward contingencies (Liu et al., 2016). Our finding that brown toadlets modify their nesting decisions based on prior mating experience (following a win-stay/lose-shift rule) provides novel evidence that amphibians can employ a rule-based learning strategy to control breeding decisions under natural conditions. Taken together, this information suggests that the cognitive abilities of amphibians may be far more advanced than currently realised.
The raw data supporting the conclusions of this article will be made available by the authors, without undue reservation.
The animal study was reviewed and approved by the Australian National University Animal Experimentation Ethics Committee (Protocol No. F.BTZ.64.04), the Monash University Animal Ethics Committee (BSCI/2007/14), and the University of Wollongong’s Animal Ethics Committee (AE1208, AE14/17) and were authorised by New South Wales National Parks & Wildlife Service (SI2552, S11005, SL101436).
PB and AS conceived, designed, and prepared the study (including submission and approval of the scientific licence and animal ethics application). PB and AS collected the data. PB analysed the data and wrote the manuscript with contributions from AS. Both authors contributed to the article and approved the submitted version.
The study was funded by a grant from the NSW Department of Environment, Climate Change and Water (3900722), Australian Research Council (DP) awarded to PB and the University of Wollongong SMAH Small Project Grant (262 27 0976) awarded to PB and AS.
The authors would like to acknowledge the Yuin People as the traditional custodians and cultural knowledge holders of the Jervis Bay region, the land on which this research was conducted.
The authors declare that the research was conducted in the absence of any commercial or financial relationships that could be construed as a potential conflict of interest.
All claims expressed in this article are solely those of the authors and do not necessarily represent those of their affiliated organizations, or those of the publisher, the editors and the reviewers. Any product that may be evaluated in this article, or claim that may be made by its manufacturer, is not guaranteed or endorsed by the publisher.
Bartlett J. A., Ward M. P., Landsman S., Epifanio J. M. (2010). Nest-site fidelity in parental male bluegill lepomis macrochirus: spatial patterns and the influence of prior mating success. J. Fish Biol. 77, 890–906. doi: 10.1111/j.1095-8649.2010.02724.x
Bauer S., Hoye B. J. (2014). Migratory animals couple biodiversity and ecosystem functioning worldwide. Science 344, 1242552. doi: 10.1126/science.1242552
Beebee T. (2005). Conservation genetics of amphibians. Heredity 95, 423–427. doi: 10.1038/sj.hdy.6800736
Beever E. A., Hall L. E., Varner J., Loosen A. E., Dunham J. B., Gahl M. K., et al. (2017). Behavioral flexibility as a mechanism for coping with climate change. Front. Ecol. Environ. 15, 299–308. doi: 10.1002/fee.1502
Bell B. D., Bishop P. J., Germano J. M., Wellington P. (2010). Lessons learned from a series of translocations of the archaic hamilton’s frog and Maud island frog in central new Zealand. Global RE-INTRODUCTION PERSPECTIVES: Additional case-studies around globe 81, 81–87.
Berger-Tal O., Blumstein D., Swaisgood R. R. (2020). Conservation translocations: a review of common difficulties and promising directions. Anim. Conserv. 23, 121–131. doi: 10.1111/acv.12534
Bradford D. F., Seymour R. S. (1988). Influence of environmental PO2 on embryonic oxygen consumption, rate of development, and hatching in the frog Pseudophryne bibroni. Physiol. Zoology 61, 475–482. doi: 10.1086/physzool.61.6.30156155
Bucciarelli G. M., Green D. B., Shaffer H. B., Kats L. B. (2016). Individual fluctuations in toxin levels affect breeding site fidelity in a chemically defended amphibian. Proc. R. Soc. B: Biol. Sci. 283, 20160468. doi: 10.1098/rspb.2016.0468
Byrne P. G. (2008). Strategic male calling behavior in an Australian terrestrial toadlet (Pseudophryne bibronii). Copeia 2008, 57–63. doi: 10.1643/CE-05-294
Byrne P. G., Keogh J. S. (2007). Terrestrial toadlets use chemosignals to recognize conspecifics, locate mates and strategically adjust calling behaviour. Anim. Behav. 74, 1155–1162. doi: 10.1016/j.anbehav.2006.10.033
Byrne P. G., Keogh J. S. (2009). Extreme sequential polyandry insures against nest failure in a frog. Proc. R. Soc. B: Biol. Sci. 276, 115–120. doi: 10.1098/rspb.2008.0794
Byrne P. G., Silla A. J. (2020). An experimental test of the genetic consequences of population augmentation in an amphibian. Conserv. Sci. Pract. 2, e194. doi: 10.1111/csp2.194
Byrne P. G., Silla A. J. (2022). Genetic management of threatened amphibians; using artificial fertilisation (AF) technologies to facilitate genetic rescue and assisted gene flow (pp124–146). IN Silla AJ, Kouba AJ, Heatwole H (Eds). Reproductive Technologies and Biobanking for the Conservation of Amphibians. CSIRO, Melbourne, Australia.
Campomizzi A. J., Morrison M. L., Dewoody J. A., Farrell S. L., Wilkins R. N. (2012). Win-stay, lose-switch and public information strategies for patch fidelity of songbirds with rare extra-pair paternity. Sci. Rep. 2, 1–6. doi: 10.1038/srep00294
Chalfoun A. D., Martin T. E. (2010). Facultative nest patch shifts in response to nest predation risk in the brewer’s sparrow: a “win-stay, lose-switch” strategy? Oecologia 163, 885–892. doi: 10.1007/s00442-010-1679-0
Crane A. L., Mathis A. (2011). Landmark learning by the Ozark zigzag salamander Plethodon angusticlavius. Curr. Zoology 57, 485–490. doi: 10.1093/czoolo/57.4.485
Danchin E., Boulinier T., Massot M. (1998). Conspecific reproductive success and breeding habitat selection: implications for the study of coloniality. Ecology 79, 2415–2428. doi: 10.1890/0012-9658(1998)079[2415:CRSABH]2.0.CO;2
Daneri M. F., Casanave E., Muzio R. N. (2011). Control of spatial orientation in terrestrial toads (Rhinella arenarum). J. Comp. Psychol. 125, 296. doi: 10.1037/a0024242
Gamble L. R., McGarigal K., Compton B. W. (2007). Fidelity and dispersal in the pond-breeding amphibian, ambystoma opacum: implications for spatio-temporal population dynamics and conservation. Biol. Conserv. 139, 247–257. doi: 10.1016/j.biocon.2007.07.001
Gerber B. D., Hooten M. B., Peck C. P., Rice M. B., Gammonley J. H., Apa A. D., et al. (2019). Extreme site fidelity as an optimal strategy in an unpredictable and homogeneous environment. Funct. Ecol. 33, 1695–1707. doi: 10.1111/1365-2435.13390
Heap S. M., Stuart-Fox D., Byrne P. G. (2015). Reduction in site fidelity with smaller spatial scale may suggest scale-dependent information use. Behav. Ecol. 26, 543–549. doi: 10.1093/beheco/aru230
Joly P. (2019). Behavior in a changing landscape: using movement ecology to inform the conservation of pond-breeding amphibians. Front. Ecol. Evol. 155. doi: 10.3389/fevo.2019.00155
Kokko H., López-Sepulcre A., Morrell L. J. (2006). From hawks and doves to self-consistent games of territorial behavior. Am. Nat. 167, 901–912. doi: 10.1086/504604
Kopecký O., Vojar J., Denoël M. (2010). Movements of alpine newts (Mesotriton alpestris) between small aquatic habitats (ruts) during the breeding season. Amphibia-Reptilia 31, 109–116. doi: 10.1163/156853810790457821
Krebs J. R. (1971). Territory and breeding density in the great tit, parus major l. Ecology 52, 2–22. doi: 10.2307/1934734
Landler L. (2022). Orientation and emigration of larval and juvenile amphibians: selected topics and hypotheses. Amphibia-Reptilia 43, 1–11. doi: 10.1163/15685381-bja10081
Landler L., Gollmann G. (2011). Magnetic orientation of the common toad: establishing an arena approach for adult anurans. Front. zoology 8, 1–9. doi: 10.1186/1742-9994-8-6
Liermann C. R., Nilsson C., Robertson J., Ng R. Y. (2012). Implications of dam obstruction for global freshwater fish diversity. BioScience 62, 539–548. doi: 10.1525/bio.2012.62.6.5
Liu Y., Day L. B., Summers K., Burmeister S. S. (2016). Learning to learn: advanced behavioural flexibility in a poison frog. Anim. Behav. 111, 167–172. doi: 10.1016/j.anbehav.2015.10.018
Merkle J. A., Abrahms B., Armstrong J. B., Sawyer H., Costa D. P., Chalfoun A. D. (2022). Site fidelity as a maladaptive behavior in the anthropocene. Front. Ecol. Environ. 20, 187–194. doi: 10.1002/fee.2456
Merkle J. A., Cherry S. G., Fortin D. (2015). Bison distribution under conflicting foraging strategies: site fidelity vs. energy maximization. Ecology 96, 1793–1801. doi: 10.1890/14-0805.1
Metzgab L. H. (1967). An experimental comparison of screech owl predation on resident and transient white-footed mice (Peromyscus leucopus). J. Mammalogy 48, 387–391. doi: 10.2307/1377771
Mitchell N. J. (2005). Nest switching in the brown toadlet (Pseudophryne bibroni): do males use chemical signals? Herpetological Rev. 36, 19–20. doi: 10.1098/rspb.2000.1334
Morrison T. A., Merkle J. A., Hopcraft J. G. C., Aikens E. O., Beck J. L., Boone R. B., et al. (2021). Drivers of site fidelity in ungulates. J. Anim. Ecol. 90, 955–966. doi: 10.1111/1365-2656.13425
O'Brien D. M., Keogh J. S., Silla A. J., Byrne P. G. (2018). The unexpected genetic mating system of the red-backed toadlet (Pseudophryne coriacea): a species with prolonged terrestrial breeding and cryptic reproductive behaviour. Mol. Ecol. 27, 3001–3015. doi: 10.1111/mec.14737
O’Brien D. M., Keogh J. S., Silla A. J., Byrne P. G. (2019). Female choice for related males in wild red-backed toadlets (Pseudophryne coriacea). Behav. Ecol. 30, 928–937. doi: 10.1093/beheco/arz031
Ogurtsov S. (2003). “Basis of native pond fidelity in anuran amphibians: the case of chemical learning.” In Herpetologia Petropolitana. Proc. of the 12th Ord Gen. Meeting of the Soc. Eur. Herpetol, pp. 198–200.
Pengilley R. (1971). Calling and associated behaviour of some species of Pseudophryne (Anura: Leptodactylidae). J. Zoology 163, 73–92. doi: 10.1111/j.1469-7998.1971.tb04525.x
Piper W. H. (2011). Making habitat selection more “familiar”: a review. Behav. Ecol. Sociobiology 65, 1329–1351. doi: 10.1007/s00265-011-1195-1
Ringler E., Szipl G., Harrigan R. J., Bartl-Binder P., Mangione R., Ringler M. (2018). Hierarchical decision-making balances current and future reproductive success. Mol. Ecol. 27, 2289–2301. doi: 10.1111/mec.14583
Riotte-Lambert L., Matthiopoulos J. (2020). Environmental predictability as a cause and consequence of animal movement. Trends Ecol. Evol. 35, 163–174. doi: 10.1016/j.tree.2019.09.009
Schaefer J. A., Bergman C. M., Luttich S. N. (2000). Site fidelity of female caribou at multiple spatial scales. Landscape Ecol. 15, 731–739. doi: 10.1023/A:1008160408257
Scheele B. C., Pasmans F., Skerratt L. F., Berger L., Martel A., Beukema W., et al. (2019). Amphibian fungal panzootic causes catastrophic and ongoing loss of biodiversity. Science 363, 1459–1463. doi: 10.1126/science.aav0379
Schjørring S., Gregersen J., Bregnballe T. (2000). Sex difference in criteria determining fidelity towards breeding sites in the great cormorant. J. Anim. Ecol. 69, 214–223. doi: 10.1046/j.1365-2656.2000.00385.x
Schmidt K. A. (2004). Site fidelity in temporally correlated environments enhances population persistence. Ecol. Lett. 7, 176–184. doi: 10.1111/j.1461-0248.2003.00565.x
Schmidt K. A., Dall S. R., Van Gils J. A. (2010). The ecology of information: an overview on the ecological significance of making informed decisions. Oikos 119, 304–316. doi: 10.1111/j.1600-0706.2009.17573.x
Shakhparonov V., Ogurtsov S. (2003). The role of the native pond odor in orientation of the green toad (Bufo viridis laur.) youngs-of-the-year. Herpetologia Petropolitana: 12th Ordinary General Meeting Societas Europaea Herpetologica Russian Journal of Herpetology 209–212.
Shaykevich D. A., Pašukonis A., O'Connell L. A. (2022). Long distance homing in the cane toad (Rhinella marina) in its native range. J. Exp. Biol. 225, jeb243048. doi: 10.1242/jeb.243048
Sonerud G. A. (2021). Win–and stay, but not too long: cavity selection by Boreal owls to minimize nest predation by pine marten. J. Ornithology 162, 839–855. doi: 10.1007/s10336-021-01876-y
Sotelo M. I., Bingman V. P., Muzio R. N. (2015). Goal orientation by geometric and feature cues: spatial learning in the terrestrial toad rhinella arenarum. Anim. Cogn. 18, 315–323. doi: 10.1007/s10071-014-0802-8
Stamps J. (1995). Motor learning and the value of familiar space. Am. Nat. 146, 41–58. doi: 10.1086/285786
Sugg D. W., Chesser R. K., Dobson F. S., Hoogland J. L. (1996). Population genetics meets behavioral ecology. Trends Ecol. Evol. 11, 338–342. doi: 10.1016/0169-5347(96)20050-3
Switzer P. V. (1993). Site fidelity in predictable and unpredictable habitats. Evolutionary Ecol. 7, 533–555. doi: 10.1007/BF01237820
Van Moorter B., Visscher D., Benhamou S., Börger L., Boyce M. S., Gaillard J. M. (2009). Memory keeps you at home: a mechanistic model for home range emergence. Oikos 118, 641–652. doi: 10.1111/j.1600-0706.2008.17003.x
Wiens J. A., Rotenberry J. T., Van Horne B. (1986). A lesson in the limitations of field experiments: shrubsteppe birds and habitat alteration. Ecology 67, 365–376. doi: 10.2307/1938579
Woodruff D. S. (1977). Male Postmating brooding behavior in three Australian Pseudophryne (Anura: Leptodactylidae). Herpetologica 33 (3), 296–303.
Keywords: breeding site fidelity, breeding decisions, nesting strategies, amphibian, in situ conservation
Citation: Byrne PG and Silla AJ (2023) Lifetime breeding-site and nest-site fidelity in a declining terrestrial toadlet: evidence for a win-stay/lose-shift strategy. Front. Conserv. Sci. 4:1226658. doi: 10.3389/fcosc.2023.1226658
Received: 22 May 2023; Accepted: 20 June 2023;
Published: 29 June 2023.
Edited by:
David R. Breininger, University of Central Florida, United StatesReviewed by:
Jonathan Webb, University of Technology Sydney, AustraliaCopyright © 2023 Byrne and Silla. This is an open-access article distributed under the terms of the Creative Commons Attribution License (CC BY). The use, distribution or reproduction in other forums is permitted, provided the original author(s) and the copyright owner(s) are credited and that the original publication in this journal is cited, in accordance with accepted academic practice. No use, distribution or reproduction is permitted which does not comply with these terms.
*Correspondence: Phillip G. Byrne, cGJ5cm5lQHVvdy5lZHUuYXU=
Disclaimer: All claims expressed in this article are solely those of the authors and do not necessarily represent those of their affiliated organizations, or those of the publisher, the editors and the reviewers. Any product that may be evaluated in this article or claim that may be made by its manufacturer is not guaranteed or endorsed by the publisher.
Research integrity at Frontiers
Learn more about the work of our research integrity team to safeguard the quality of each article we publish.