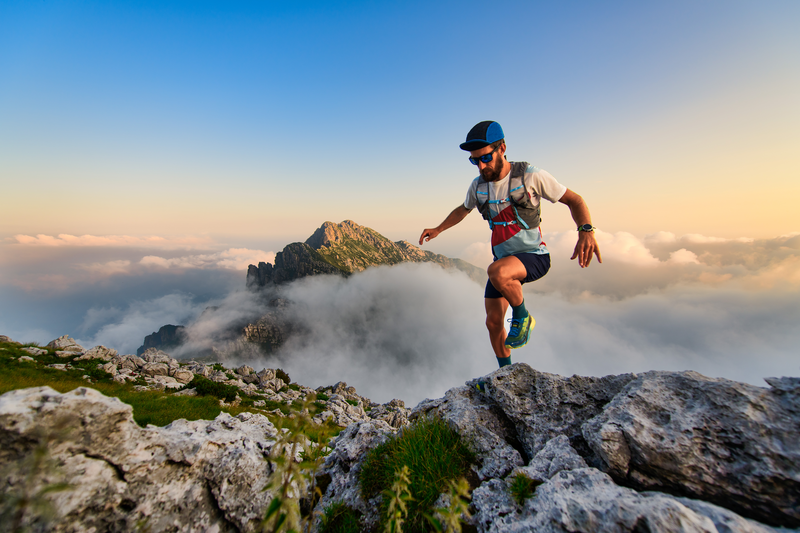
95% of researchers rate our articles as excellent or good
Learn more about the work of our research integrity team to safeguard the quality of each article we publish.
Find out more
SPECIALTY GRAND CHALLENGE article
Front. Conserv. Sci. , 09 February 2023
Sec. Global Biodiversity Threats
Volume 4 - 2023 | https://doi.org/10.3389/fcosc.2023.1147470
A key trait of the Anthropocene is the rapid change of environmental processes (the so-called global change drivers) and social attitudes towards their effects (Alley et al., 2003; Brook et al., 2008; Pereira et al., 2010; Chen et al., 2011; Mace, 2014). Neither drivers nor attitudes are new phenomena. Climate, atmospheric composition, habitats and biological communities are ever changing, and human societies have overexploited, sustainably managed and preserved species, habitats or sites throughout human history. Rapid global change erodes populations, communities and whole ecological systems, usually in non-linear ways. Non-linear effects will eventually lead to tipping points of sudden, irreversible change in populations, communities and whole ecosystems (Brook et al., 2008; Lenton and Williams, 2013; Rohr et al., 2014; Urban, 2015). Extinctions caused by overharvesting or by introduced predators, competitors or diseases; community collapse due to extinction of keystone interactors or to introduction of keystone predators, competitors or parasites; and simplification of ecosystems due to loss of ecosystem engineers, pollution, habitat loss, fragmentation and climate change are classical textbook examples of such tipping points (Leakey and Lewin, 1996; Kolbert, 2014).
While initially local, collapses are becoming increasingly global. Increased human capacities for harvesting and transporting organisms, for exploiting and transforming habitats, and for changing the Earth’s climate are both cause and by-products of globalisation (Diamond, 2011; Harari, 2014). Overexploitation and irreversible change of ecological systems are no longer limited by technology. Solutions to global change threats to biodiversity will thus come from new social attitudes to conservation (Mace, 2014). Sparing areas from people’s use, or preventing intensive uses in protected areas, was the cornerstone of last century conservation policy, and it is still defended by leading scientists (Wilson, 2016). Increasing intensity of exploitation of land outside preserves to fulfil the exploding needs of a growing human population, and spill-over of intensification effects toward land spared for conservation, fuelled the development of land-sharing approaches to conservation (Phalan et al., 2011).
Productive land use systems are integrated with the conservation of wildlife in a shared landscape, where wildlife provides ecosystem services sustaining such productive land uses. This ‘Nature for people’ framework is supported by environmental economic research that integrate commercial and non-commercial values and develop ways for considering non-commercial values in accounting systems (Costanza et al., 2014; Campos et al., 2019). ‘Nature for people’ frameworks underlie the recent history of large-scale land-use policies such as the European Common Agricultural Policy (Díaz et al., 2021a; Pe’er et al., 2022), as well as integrative concepts such as the ecological intensification of agricultural systems (Clough et al., 2011; Geertsema et al., 2016). Limitation of economic theory to estimate the social values that nature gives to people led to the development of the ‘People and Nature’ framework. Ecological, social and economic values are integrated into sustainable socioecological systems (Mace, 2014). Both values and management systems are based on long-term agreements within specific human societies (Díaz et al., 2018). This approach allows for incorporating spiritual and local values and attitudes difficult to generalize to the scale of the global economy, so that they can counteract intensification trends of seemingly ‘green’ policies of energy production, transport systems, and urbanization (Díaz et al., 2018).
Climate change, pollution, biotic changes and land use change have been identified has the main drivers of current global change (Brook et al., 2008). These drivers are ultimately fuelled, or attenuated, by social attitudes towards wildlife use (from overexploitation to sustainable management to recreation), urbanisation, global trade, agricultural patterns, water use, energy-supply systems, and economic policy.
Knowledge on the effects of social trends on drivers, of drivers on biodiversity and ecosystem functioning, and of ecosystem functioning on sustainability of human societies is however still uneven and usually too coarse. For instance, climate change predictions are increasingly detailed and precise, thanks to the sustained work of hundreds of first-class scientists during the last decades under the Intergovernmental Panel on Climate Change (IPCC) platform (Pörtner et al., 2022). How and why predicted climate changes will determine changes in species, communities and ecosystems is still a matter of active research, however (Peterson et al., 2011; Mendoza and Araújo, 2019; Díaz et al., 2021b). Translation of climate-biodiversity relations into policy action to prevent and/or adapt socioeconomic systems to expected consequences of climate change is even more difficult (Polasky et al., 2011). Effects of the remaining global drivers, and of interactions among them, are even less understood, although research is advancing rapidly (Boulangeat et al., 2012; Díaz et al., 2021b). Finally, research on socioecological systems promoted by the Intergovernmental Science-Policy Platform on Biodiversity and Ecosystem Services (IPBES), the biological equivalent to the IPCC, is starting to provide foundations for translating knowledge on global change effects on ecosystems to policy action to counteract them (Chaplin-Kramer et al., 2019).
Although every piece of sound knowledge on the complex issue of dealing with global biodiversity threats is urgently needed, several pressing and promising topics can be highlighted. First, it is still necessary to refine the use of climate change models to predict changes in biodiversity and develop effective conservation policies. Models that integrate additive and interactive effects of other drivers are specially needed to increase predictive power under realistic scenarios (Outhwaite et al., 2022). Routine use of the sophisticated but easily accessible modelling tools to generate simplistic ‘predictions’ of future species distributions and develop ‘effective’ policies are likely to be of very limited value (Pearson and Dawson, 2003). Large-scale modelling exercises, and tests of model predictions with past or future data, are much more promising (Thuiller et al., 2005; Nogués-Bravo et al., 2008; Brodie et al., 2022).
The second promising field of research deals with the development of sound theory on processes underlying extinction and invasion risk. Increasing availability of global databases are depicting detailed patterns of extinction and invasion potential, as well as correlates with them. Such analyses focus on associations between extinction or invasion with species traits (often in a phylogenetic framework) or ecological drivers (Munstermann et al., 2022). Yet we still lack integrated analyses that lead to more general predictions. For instance, the best predictor of invasive potential is whether the species is invasive in other area in the world, together with propagule pressure (i.e., the number of introduction attempts) and tolerance to humans (Møller et al., 2015), but little can be said about species that have not been moved yet to other areas (Hawkins et al., 2015). For this reason, policies tend to rely more on the precautionary principle, declaring potential invasive species on the basis of knowledge derived from other invasions and avoiding any introduction as far as possible. However, since global trade trends often do not follow these precautionary rules we need better models to anticipate invasion potential (Hulme, 2021).
The search for more general patterns and processes underlying global threats to biodiversity should also consider spatial and temporal variation of drivers’ effects. Spatial patterns may arise from latitudinal, longitudinal or altitudinal gradients of environmental suitability. Such gradients usually show decreasing trends of suitability, and increasing effects of drivers, from the centre to the borders of species´ ranges (Brown, 1984). Asymmetric trends in the leading and rear edges of current climate-driven northwards movement of ranges (Hampe and Petit, 2005), areas of high suitability close to borders (Tellería et al., 2021), geographic asymmetries (Díaz et al., 2015) or intercontinental differences (Møller et al., 2014) are most likely due to long-lasting effects of past events, from glacier-age legacies (Hampe and Petit, 2005; Tellería et al., 2021) to history of land uses (Møller et al., 2014; Díaz et al., 2015). Research on processes causing spatial and temporal variation in effects on current biodiversity will help to adapt management recommendations to local realities. It is extremely unlikely that management policies promoting fixed practices across heterogeneous regions will be effective at counteracting threats, as the recent history of the European Common Agricultural Policy shows (Pe’er et al., 2014).
Finally, identifying threats is not enough: management practices aimed at counteracting them should be developed, evaluated, and modified if they are found to be ineffective (Pérez et al., 2012; Díaz and Concepción, 2016). Routine evaluation of the effectiveness of measures using proper controls should be mandatory when developing conservation policies (Kleijn et al., 2006). Both management plans and evaluations should take into account scale issues, as actions are usually applied by owners at the scale of their properties but landscape context may greatly condition action’s effectiveness (Concepcion et al., 2012; Meier et al., 2022). New accounting systems that incorporate non-commercial values of biodiversity (Campos et al., 2019) will help develop large-scale monitoring systems of biodiversity change, which are essential to detect changes in trends and to evaluate whether management actions scale-up to curb negative trend of species or communities (Díaz et al., 2022).
Globalization of commerce has expanded the threats to biodiversity to global scales. Globalisation of conservation may, however, permit the rapid global dissemination of successes and failures of local conservation actions. Scientific journals are the best available tool for such dissemination (Díaz et al., 2016). I encourage our diverse readers and authors to submit papers to Frontiers in Conservation Science that will help us rise to the challenge of defining, understanding, and solving the global biodiversity threats faced in the Anthropocene.
The author confirms being the sole contributor of this work and approved it for publication.
My warmest thanks to Dan Blumstein for the opportunity to contribute to this emerging journal. I wrote the paper during a five-month stay in Dan’s lab at UCLA, funded by a scholar grant of the Fullbright Commission (PRX21/00023).
The author declares that the research was conducted in the absence of any commercial or financial relationships that could be construed as a potential conflict of interest.
All claims expressed in this article are solely those of the authors and do not necessarily represent those of their affiliated organizations, or those of the publisher, the editors and the reviewers. Any product that may be evaluated in this article, or claim that may be made by its manufacturer, is not guaranteed or endorsed by the publisher.
Alley R. B., Marotzke J., Nordhaus W. D., Overpeck J. T., Peteet D. M., Pielke R. A. Jr., et al. (2003). Abrupt climate change. Science 299, 2005–2010. doi: 10.1126/science.1081056
Boulangeat I., Gravel D., Thuiller W. (2012). Accounting for dispersal and biotic interactions to disentangle the drivers of species distributions and their abundances. Ecol. Let. 15, 584–593. doi: 10.1111/j.1461-0248.2012.01772.x
Brodie S., Smith J. A., Muhling B. A., Barnett L. A., Carroll G., Fiedler P., et al. (2022). Recommendations for quantifying and reducing uncertainty in climate projections of species distributions. Glob. Change Biol. 28, 6586–6601. doi: 10.1111/gcb.16371
Brook B. W., Sodhi N. S., Bradshaw C. J. (2008). Synergies among extinction drivers under global change. Trends Ecol. Evol. 23, 453–460. doi: 10.1016/j.tree.2008.03.011
Brown J. H. (1984). On the relationship between abundance and distribution of species. Amer. Natur. 124, 255–279. doi: 10.1086/284267
Campos P., Caparrós A., Oviedo J. L., Ovando P., Álvarez-Farizo B., Díaz-Balteiro L., et al. (2019). Bridging the gap between national and ecosystem accounting application in andalusian forests, Spain. Ecol. Econ. 157, 218–236. doi: 10.1016/j.ecolecon.2018.11.017
Chaplin-Kramer R., Sharp R. P., Weil C., Bennett E. M., Pascual U., Arkema K. K., et al. (2019). Global modeling of nature’s contributions to people. Science 366, 255–258. doi: 10.1126/science.aaw3372
Chen I.-C., Hill J. K., Ohlemüller R., Roy D. B., Thomas C. D. (2011). Rapid range shifts of species associated with high levels of climate warming. Science 333, 1024–1026. doi: 10.1126/science.1206432
Clough Y., Barkmann J., Juhrbandt J., Kessler M., Wanger T. C., Anshary A., et al. (2011). Combining high biodiversity with high yields in tropical agroforests. PNAS 108, 8311–8316. doi: 10.1073/pnas.1016799108
Concepcion E. D., Díaz M., Kleijn D., Baldi A., Batary P., Clough Y., et al. (2012). Interactive effects of landscape context constrain the effectiveness of local agri-environmental management. J. Appl. Ecol. 49, 695–705. doi: 10.1111/j.1365-2664.2012.02131.x
Costanza R., De Groot R., Sutton P., van der Ploeg S., Anderson S. J., Kubiszewski I., et al. (2014). Changes in the global value of ecosystem services. Glob. Environ. Change 26, 152–158. doi: 10.1016/j.gloenvcha.2014.04.002
Diamond J. (2011). Collapse: how societies choose to fail or succeed, revised edition (London: Penguin).
Díaz M., Aycart P., Ramos A., Carricondo A., Concepción E. D. (2022). Site-based vs. species-based analyses of long-term farmland bird datasets: Implications for conservation policy evaluations. Ecol. Ind. 140, 109051. doi: 10.1016/j.ecolind.2022.109051
Díaz M., Concepción E. D. (2016). Enhancing the effectiveness of CAP greening as a conservation tool: a plea for regional targeting considering landscape constraints. Curr. Land. Ecol. Rep. 1, 168–177. doi: 10.1007/s40823-016-0017-6
Díaz M., Concepción E. D., Morales M. B., Alonso J. C., Azcárate F. M., Bartomeus I., et al. (2021a). Environmental objectives of Spanish agriculture: Scientific guidelines for their effective implementation under the common agricultural policy 2023-2030. Ardeola 68, 445–460. doi: 10.13157/arla.68.2.2021.fo1
Díaz M., Cuervo J. J., Grim T., Flensted-Jensen E., Ibáñez-Álamo J. D., Jokimäki J., et al. (2015). Interactive effects of fearfulness and geographical location on bird population trends. Behav. Ecol. 26, 716–721. doi: 10.1093/beheco/aru211
Díaz M., Grim T., Markó G., Morelli F., Ibáñez-Alamo J. D., Jokimäki J., et al. (2021b). Effects of climate variation on bird escape distances modulate community responses to global change. Sci. Rep. 11, 1–9. doi: 10.1038/s41598-021-92273-1
Díaz M., Moreno E., Amat J. A., Arroyo B., Barba E., González-Solís J., et al. (2016). Ardeola, a scientific journal of ornithology: cooperative survivorship within the red queen game. Ardeola 63, 3–14. doi: 10.13157/arla.63.1.2016.ed1
Díaz S., Pascual U., Stenseke M., Martín-López B., Watson R. T., Molnár Z., et al. (2018). Assessing nature’s contributions to people. Science 359, 270–272. doi: 10.1126/science.aap8826
Geertsema W., Rossing W. A., Landis D. A., Bianchi F. J., Van Rijn P. C., Schaminée J. H., et al. (2016). Actionable knowledge for ecological intensification of agriculture. Front. Ecol. Environ. 14, 209–216. doi: 10.1002/fee.1258
Hampe A., Petit R. J. (2005). Conserving biodiversity under climate change: the rear edge matters. Ecol. Let. 8, 461–467. doi: 10.1111/j.1461-0248.2005.00739.x
Hawkins C. L., Bacher S., Essl F., Hulme P. E., Jeschke J. M., Kühn I., et al. (2015). Framework and guidelines for implementing the proposed IUCN environmental impact classification for alien taxa (EICAT). Div. Distr. 21, 1360–1363. doi: 10.1111/ddi.12379
Hulme P. E. (2021). Unwelcome exchange: International trade as a direct and indirect driver of biological invasions worldwide. One Earth 4, 666–679. doi: 10.1016/j.oneear.2021.04.015
Kleijn D., Baquero R. A., Clough Y., Díaz M., De Esteban J., Fernández F., et al. (2006). Mixed biodiversity benefits of agri-environment schemes in five European countries. Ecol. Let. 9, 243–254. doi: 10.1111/j.1461-0248.2005.00869.x
Leakey R. E., Lewin R. (1996). The sixth extinction: patterns of life and the future of humankind (New York: Anchor).
Lenton T. M., Williams H. T. (2013). On the origin of planetary-scale tipping points. Trends Ecol. Evol. 28, 380–382. doi: 10.1016/j.tree.2013.06.001
Møller A. P., Díaz M., Flensted-Jensen E., Grim T., Ibáñez-Alamo J. D., Jokimäki J., et al. (2015). Urbanized birds are superior invaders of novel habitats. Oecologia 178, 943–950. doi: 10.1007/s00442-015-3268-8
Møller A. P., Samia D. S., Weston M. A., Guay P. J., Blumstein D. T. (2014). American Exceptionalism: population trends and flight initiation distances in birds from three continents. PloS One 9 (9), e107883. doi: 10.1371/journal.pone.0107883
Meier E. S., Lüscher G., Knop E. (2022). Disentangling direct and indirect drivers of farmland biodiversity at landscape scale. Ecol. Let. 25, 2422–2434. doi: 10.1111/ele.14104
Mendoza M., Araújo M. B. (2019). Climate shapes mammal community trophic structures and humans simplify them. Nat. Commun. 10, 1–9. doi: 10.1038/s41467-019-12995-9
Munstermann M. J., Heim N. A., McCauley D. J., Payne J. L., Upham N. S., Wang S. C., et al. (2022). A global ecological signal of extinction risk in terrestrial vertebrates. Conserv. Biol. 36, e13852. doi: 10.1111/cobi.13852
Nogués-Bravo D., Rodríguez J., Hortal J., Batra P., Araújo M. B. (2008). Climate change, humans, and the extinction of the woolly mammoth. PloS Biol. 6, e79. doi: 10.1371/journal.pbio.0060079
Outhwaite C. L., McCann P., Newbold T. (2022). Agriculture and climate change are reshaping insect biodiversity worldwide. Nature 605, 97–102. doi: 10.1038/s41586-022-04644-x
Pearson R. G., Dawson T. E. (2003). Predicting the impacts of climate change on the distribution of species: Are bioclimate envelope models useful? Glob. Ecol. Biogeogr. 12, 361–371. doi: 10.1046/j.1466-822X.2003.00042.x
Pe’er G., Dicks L. V., Visconti P., Arlettaz R., Báldi A., Benton T. G., et al. (2014). EU Agricultural reform fails on biodiversity. Science 344, 1090–1092. doi: 10.1126/science.1253425
Pe’er G., Finn J. A., Díaz M., Birkenstock M., Lakner S., Röder N., et al. (2022). How can the European common agricultural policy help halt biodiversity loss? recommendations by over 300 experts. Conserv. Let. 15, e12901. doi: 10.1111/conl.12901
Pereira H. M., Leadley P. W., Proença V., Alkemade R., Scharlemann J. P., Fernandez-Manjarrés J. F., et al. (2010). Scenarios for global biodiversity in the 21st century. Science 330, 1496–1501. doi: 10.1126/science.1196624
Pérez I., Anadón J. D., Díaz M., Nicola G. G., Tella J. L., Giménez A. (2012). What is wrong with current translocations? a review and a decision-making proposal. Front. Ecol. Environ. 10, 494–501. doi: 10.1890/110175
Peterson A. T., Soberón J., Pearson R. G., Anderson R. P., Martínez-Meyer E., Nakamura M., et al. (2011). Ecological niches and geographic distributions (Princeton: Princeton Univ. Press).
Phalan B., Onial M., Balmford A., Green R. E. (2011). Reconciling food production and biodiversity conservation: land sharing and land sparing compared. Science 333, 1289–1291. doi: 10.1126/science.1208742
Polasky S., Carpenter S. R., Folke C., Keeler B. (2011). Decision-making under great uncertainty: environmental management in an era of global change. Trends Ecol. Evol. 26, 398–404. doi: 10.1016/j.tree.2011.04.007
Pörtner H. O., Roberts D. C., Adams H., Adler C., Aldunce P., Ali E., et al. (2022). Climate change 2022: Impacts, adaptation and vulnerability (Brussels: IPCC Sixth Assessment Report).
Rohr R. P., Saavedra S., Bascompte J. (2014). On the structural stability of mutualistic systems. Science 345, 1253497. doi: 10.1126/science.1253497
Tellería J. L., Hernández-Lambraño R. E., Carbonell R. (2021). Ecological and geographical marginality in rear edge populations of palaearctic forest birds. J. Biogeogr. 48, 2538–2549. doi: 10.1111/jbi.14219
Thuiller W., Lavorel S., Araújo M. B., Sykes M. T., Prentice I. C. (2005). Climate change threats to plant diversity in Europe. PNAS 102, 8245–8250. doi: 10.1073/pnas.0409902102
Urban M. C. (2015). Accelerating extinction risk from climate change. Science 348, 571–573. doi: 10.1126/science.aaa4984
Keywords: new integrated approaches, multidisciplinarity, global change drivers, extinctions, anthropocene
Citation: Díaz M (2023) Dealing with global threats to biodiversity: A pressing but realistic challenge. Front. Conserv. Sci. 4:1147470. doi: 10.3389/fcosc.2023.1147470
Received: 18 January 2023; Accepted: 30 January 2023;
Published: 09 February 2023.
Edited and Reviewed by:
Ronald R. Swaisgood, San Diego Zoo Wildlife Alliance, United StatesCopyright © 2023 Díaz. This is an open-access article distributed under the terms of the Creative Commons Attribution License (CC BY). The use, distribution or reproduction in other forums is permitted, provided the original author(s) and the copyright owner(s) are credited and that the original publication in this journal is cited, in accordance with accepted academic practice. No use, distribution or reproduction is permitted which does not comply with these terms.
*Correspondence: Mario Díaz, TWFyaW8uRGlhekBjY21hLmNzaWMuZXM=
†ORCID: Mario Díaz, orcid.org/0000-0002-6384-6674
Disclaimer: All claims expressed in this article are solely those of the authors and do not necessarily represent those of their affiliated organizations, or those of the publisher, the editors and the reviewers. Any product that may be evaluated in this article or claim that may be made by its manufacturer is not guaranteed or endorsed by the publisher.
Research integrity at Frontiers
Learn more about the work of our research integrity team to safeguard the quality of each article we publish.