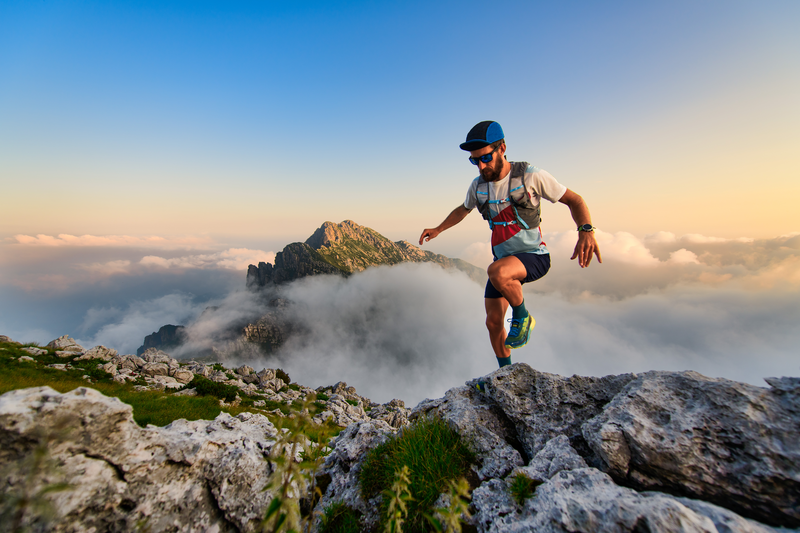
94% of researchers rate our articles as excellent or good
Learn more about the work of our research integrity team to safeguard the quality of each article we publish.
Find out more
REVIEW article
Front. Conserv. Sci. , 24 May 2023
Sec. Animal Conservation
Volume 4 - 2023 | https://doi.org/10.3389/fcosc.2023.1133329
The viability of large herbivore populations in the face of climate change, environmental variability, disease and predation will be determined by their freedom to assess and respond to these factors through access to a range of functional seasonal resources and habitats. Their responses will be contingent upon various organismal traits, such as body size, mouth and digestive anatomy, which also facilitate coexistence among sympatric species. In this paper we develop a functional adaptive forage resource framework based on plant biomass, quality and phenology responses on ecological productivity gradients. We show how large herbivores coexist and respond to environmental variability, disease and predation by their foraging responses in relation to functional adaptive resources, as mediated by their anatomical traits. Below a critical body size, where predation limits population size, large herbivores adopt a variety of predation avoidance strategies, which are linked to their anatomical traits and foraging strategies. Mouth anatomy, and its interaction with body size, appears to be the major anatomical trait determining large herbivore selection for grass height. Body size is the major trait determining vulnerability to predators. Ecological productivity gradients underlain by variation in soil moisture availability over the annual cycle support high quality forage in the least productive (driest) regions, which promote growth and reproduction (a fecundity resource). Reserves of adequate quality forage in moderate productivity regions and buffers of low-quality forage in wetter and more productive regions of the gradient prevent loss of body stores over the dry season and starvation during droughts. Fire and grazing contribute towards providing high quality forage by removing old, low-quality material and preventing forage maturation. Consuming a high diversity of plant species distributed across ecological gradients promotes phytochemical diversity in the diet, which functions as medicinal resources to promote health while combating disease and parasites. Large herbivores are becoming increasingly restricted by ecosystem fragmentation in their access to the full range of these functional resource classes. The negative consequences for large herbivore populations of reduced access to these resource classes is compounded by climate change, where conditions are hotter and drought frequency and intensity is expected to be higher.
For large herbivore populations to persist, they must meet needs through each stage of the annual cycle during favourable years, while minimising loss of body stores in adverse years (Parker et al., 2009). Large herbivores must cope with seasonal and inter-annual variation in plant growth and hence in the quantity and quality of food resources, dependent largely on rainfall in savannah ecosystems (Sinclair, 1975; Ellis and Swift, 1988; Owen-smith, 2008), in contrast to temperature at higher latitudes (Mysterud et al., 2001). Temporal variation in resources may be counteracted by exploiting spatial heterogeneity (Scoones, 1995; Owen-Smith, 2002; Owen-Smith, 2004; Fryxell et al., 2005; Stokes et al., 2006; Hobbs et al., 2008; Prins and van Langevelde, 2008), but only if animals can access key components of this heterogeneity. Environmental patchiness or spatial heterogeneity has long been recognised as a key mechanism facilitating access to resources and an optimal diet (MacArthur and Pianka, 1966). Environmental patchiness becomes critical for the survival of a species when different patches provide different non-substitutable resources (Hobbs et al., 2008). For example, moose (Alces alces) in Isle Royale National Park, Michigan, obtain sufficient essential sodium intake (Kaspari, 2020) in one specialised habitat (wetlands), while they maximise their energy intake in woodland habitats (Belovsky, 1978). Adaptive foraging and access to essential non-substitutable resources is facilitated by freedom of movement across broad heterogenous landscapes or ecological gradients supporting diverse functional forage resources (Belovsky, 1978; Senft et al., 1987; Scoones, 1995; Fryxell et al., 2005; Stokes et al., 2006; Hobbs et al., 2008).
Ecological productivity gradients, such as rainfall or hydrological gradients, that influence the spatial distribution of soil nutrients, forage biomass, quality and phenological patterns across the annual cycle determine the distribution of functional seasonal forage resources (McNaughton, 1985; Murray, 1995; Hopcraft et al., 2010; Fynn et al., 2014; Sianga and Fynn, 2017; Keemekae, 2022). In turn, the distribution of these various forage resources influences large herbivore seasonal movements and facilitates adaptive foraging responses to seasonal and inter-annual variability in forage biomass and quality (Bell, 1970; Maddock, 1979; Hopcraft et al., 2010; Fynn et al., 2015). For example, the wetter and higher productivity regions of ecological gradients, such as in high-rainfall areas or in wetlands, support more reliable, adequate-quality (green) forage during the dry season where plant growth ceases and forage becomes scarce and of low quality in drier regions of the gradient (Vesey-FitzGerald, 1960; Sheppe and Osborne, 1971; Acres et al., 1985; McNaughton, 1985; Fryxell and Sinclair, 1988; Pamo, 1998; Kamweneshe, 2000; Scholte and Brouwer, 2008; Fynn et al., 2014; Fynn et al., 2015). A reserve of adequate-quality forage during the dry season is, therefore, essential for reducing loss of body stores of large herbivores and even population collapse during droughts (Illius and O’Connor, 2000; Owen-Smith, 2004). The drier and less productive regions of ecological gradients support shorter and more digestible and nutritious forage (McNaughton, 1985; Fryxell and Sinclair, 1988; Murray, 1995; Hopcraft et al., 2010; Fynn et al., 2014), which is essential for growth and reproduction (White, 1983; Gordon, 1988; Owen-Smith, 1990; Gaillard et al., 2000; Person et al., 2003; Pettorelli et al., 2003; Cook et al., 2004; Owen-Smith, 2004; Parker et al., 2009).
Consequently, for large herbivores to assess and respond to seasonal and inter-annual variability of forage quantity and quality, as well as to variability in their own resource needs, influenced by seasons of growth and reproduction, they need to be able to move across ecological gradients to access functional heterogeneity in forage resources (Owen-Smith, 2004; Hopcraft et al., 2010). Climate change is expected to increase temperatures and environmental variability, especially the frequency and intensity of droughts (Cai et al., 2014; Engelbrecht et al., 2015). Higher temperatures will increase disparity between rainfall and evapotranspiration leading to shorter growing seasons and thereby a shorter duration of green forage for large herbivores; this will likely make it more difficult for large herbivores to meet their nutritional requirements over the annual cycle (Ogutu and Owen-Smith, 2003). The range of different forage resources with distinct functional roles that enable adaptation to environmental variability and climate change is more nuanced and diverse than the simple dichotomy of wet and dry season forage resources.
Thus, knowledge of the full range and function of various forage resource classes and how they are distributed on ecological gradients is essential for understanding how large herbivores assess and respond to seasonal and inter-annual variability in forage quantity and quality, as well as to climate change. Such an understanding will play a key role in planning for the effects of climate change and in designing protected areas (PAs). Anatomical traits of herbivores, such as body size and mouth anatomy, can strongly influence how various large herbivore species perceive the functionality of different types of forage resources. Body size is considered a key trait influencing niche differentiation along forage quality gradients (Owen-Smith, 1988; Hopcraft et al., 2010). While body size does not appear to influence mean retention time of forage and digestive efficiency (Müller et al., 2013; Steuer et al., 2014), there are several mechanisms through which larger bodied herbivores can be more tolerant of lower quality forage. First, larger body size may promote greater ability to handle and process low-quality forage (Steuer et al., 2014). Second, larger body size may allow greater forage intake relative to basal metabolism, allowing large-bodied herbivores to survive better on lower quality forage (Müller et al., 2013). Another key factor that may promote coexistence of large herbivores on forage quality gradients is that with low quality forage, where lignin content is high, a longer retention time of forage in the gut will not be as advantageous for nutrient extraction than having a higher intake rate of forage (Müller et al., 2013). Therefore, hindgut fermenters are likely to be more tolerant of low-quality forage than ruminants because their intake and passage rates of low-quality forage is greater than that of ruminants (Müller et al., 2013). Body size also influences predation risk with population size of very large-bodied herbivores (buffalo and upwards) being unconstrained by predation (Sinclair et al., 2003; Hopcraft et al., 2012).
Perhaps of greater significance than body size for coexistence on forage quality gradients is mouth anatomy, which influences intake rates of forage in different grassland structural states where broad-mouthed grazers can achieve sufficient bite size to meet intake requirements in short grassland with the reverse being true for narrow-mouthed grazers in taller grassland (Murray and Illius, 2000; Arsenault and Owen-Smith, 2008). Finally, adaptive resilience in large herbivore populations will be determined by their ability to assess and respond to predation risk, which if compromised by anthropogenic effects, can cause the collapse of herbivore populations (Bergerud and Elliot, 1986; Harrington et al., 1999; Rettie and Messier, 2000; Wittmer et al., 2005). Understanding how forage resource classes and anatomical traits are related to predation risk is essential for understanding the adaptive foraging strategies of large herbivores and how these may by disrupted by anthropogenic effects. The anatomical traits of large herbivores, therefore, provides a mechanism for coexistence by facilitating partitioning of forage resources, as well as affecting predation avoidance strategies.
Another important concept not yet integrated into our understanding of functional heterogeneity of forage resources and adaptive foraging strategies is the concept of medicinal resources. Most discussion has centred around energy and protein in forage and predation risk as drivers of adaptive foraging movements (e.g. Parker et al., 2009; Hopcraft et al., 2010). Wild herbivores face the same challenges from internal parasites, ticks and disease as domestic ones, whose populations in confinement are maintained through veterinary inputs in the form of synthetic drugs and antibiotics (Acha and Melendez, 1983). However, when domestic herbivores have access to high plant and phytochemical diversity (secondary metabolites) across broad landscapes, they have much less need for veterinary inputs (see Provenza et al., 2003; Villalba et al., 2006; Provenza et al., 2007; Villalba and Provenza, 2007; Provenza and Villalba, 2010; Gessner et al., 2017; Villalba et al., 2017; Provenza, 2018). It follows, therefore, that with wild herbivores having no access to veterinary interventions, their populations, together with genetic adaptation to disease through natural selection, must rely on plant secondary metabolites to remain healthy and productive. In this light, we suggest that various medicinal resources in the form of a diverse array of plant secondary metabolites (phytochemical diversity) represents an essential aspect of functional resource heterogeneity for large herbivores but is yet to be appropriately integrated into a foraging ecology framework.
In summary, for large herbivores to maintain viable populations and to coexist with other sympatric large herbivore species requires assessment of and response to various abiotic and biotic factors, with responses being mediated by various contingency factors (Figure 1). In this paper, we synthesise and describe the nature and interactions of the various components of Figure 1. While functional forage resource classes have been developed for African browsers (Owen-Smith, 2002), a similar framework for African grazers is lacking, especially in understanding how these resources are distributed on ecological productivity gradients. Moreover, while much attention has been given to body size as a key anatomical trait of large herbivores affecting foraging and anti-predation strategies, much less attention has been given to mouth anatomical traits. Understanding is needed on the link between mouth anatomy and foraging strategies and how these determine anti-predation strategies. Thus, there is a gap in our understanding of the biotic component of Figure 1 and how it relates to the abiotic and contingency components. We, therefore, focus on developing an understanding of the range of functional forage resource classes that large herbivore grazers in African savannas require to respond to environmental variability and disease and how these resources are distributed on ecological gradients. In addition, we show how large herbivore anatomical traits influence their perceptions of the functionality of these forage resources and how this influences predation avoidance strategies and overall species coexistence.
Figure 1 Conceptual model of the factors influencing large herbivore population productivity and coexistence among species.
Decades of detailed observations on diet selection by kudu Tragelaphus strepsiceros and several other browser species enabled linking of the physical and chemical traits of plants to key adaptive foraging strategies over the annual cycle (Owen-Smith et al., 1983; Owen-Smith and Cooper, 1987; Cooper et al., 1988; Owen-Smith and Cooper, 1989; Owen-Smith, 1994). This enabled empirical analysis and modelling of the influence of different forage resource classes on population dynamics (Owen-Smith, 1990; Owen-Smith, 2002, p 255). For browsers, key plant traits determining seasonal adaptive foraging were protein content - condensed tannin ratios (nutrient supply vs. chemical defences), physical defences (thorns) and seasonal availability of forage determined by phenological traits (see Owen-Smith et al., 1983; Owen-Smith and Cooper, 1987; Cooper et al., 1988; Owen-Smith and Cooper, 1989; Owen-Smith, 1994). Linking these plant traits to adaptive foraging strategies was achieved by developing six generic functional resource classes (Owen-Smith, 2002, p 255): high-quality resources as soft leaves and stems of digestible, protein-rich forbs; restricted-intake resources, such as protein-rich leaves of thorny species, where thorns limit intake; staple resources as leaves of palatable deciduous species that provide a more reliable intake of relatively protein-rich forage, low in tannins; reserve resources as leaves of palatable evergreens that provide adequate-quality forage during the dry season when more preferred forages are depleted; transient bridging resources as palatable emerging (unpalatable when mature), relatively protein-rich leaves on unpalatable deciduous trees during the late dry season; and low-quality buffer resources as leaves of unpalatable evergreens. Generic functional resource’s enable browsers to meet requirements for growth and reproduction, while minimising body store depletion during the dry season.
Building on the generic functional resource concept we develop a forage resource – ecological gradient framework for grazers in African savannas, which we refer to as functional adaptive resources (FARs). We propose seven FAR classes in African grazing ecosystems that are distributed across soil moisture (Figure 2) and disturbance (Figure 3) gradients.
Figure 2 Conceptual model of the distribution of functional adaptive resources (FARs) along various soil-moisture-driven productivity gradients at different scales in African savannahs. Each FAR class is a functionally-distinct or non-substitutable resource.
Figure 3 Conceptual model of the distribution of functional adaptive resources (FARs) along disturbance gradients of grazing intensity and fire frequency.
Large herbivores must assess and respond not only to variable forage quantity and quality over the annual cycle but also to variation in their requirements for energy, protein and minerals, which become greatly elevated during the season of growth and reproduction (Murray, 1995; Parker et al., 2009; Augustine et al., 2011). A fecundity resource is most effective at meeting the elevated energy and nutrient intake requirements required to enable optimal growth and reproduction (fecundity) of large herbivore populations. Demand for these resources peaks during the wet season when large herbivores enter the final stages of foetus development, drop calves, lactate and when new born calves must grow rapidly. The probability of conception after calving is determined by body condition at calving (Michael et al., 2019). Conception rates, age at first conception, birth rates, calf growth rates and calf survival are determined largely by the quality of forage during the wet season (Belovsky, 1978; White, 1983; Gordon, 1988; Owen-Smith, 1990; Gaillard et al., 2000; Person et al., 2003; Pettorelli et al., 2003; Cook et al., 2004; Michael et al., 2019). For example, a moose calf cannot survive without its mother’s milk as a supplement if it does not reach a specific body weight in its first season of growth, nor can a female moose produce a calf without achieving a minimum body weight (Belovsky, 1978). Likewise, the survival of kudu calves is determined by access to sufficient high-quality forbs during the wet season (Owen-Smith, 1990). Thus, herbivore populations without access to forage that is highly digestible, with high concentrations of energy, protein and minerals and of optimal height and bite size for their specific body size and mouth anatomy, will be unproductive or even decline (Owen-Smith, 2004; Parker et al., 2009).
Traditionally, a key resource has been considered as taller, lower-quality dry season forage that prevents mass starvation and population collapse during droughts, thereby maintaining the size of large herbivore populations (Illius and O’Connor, 2000). Considering, however, the critical effect that a fecundity resource has on reproduction and calf survival, large herbivores cannot attain a large population size without access to a fecundity resource (e.g. Owen-Smith, 2004). A fecundity resource is also selected by large herbivores because it is generally associated with low-predation risk, such as short, open high-quality grassland for short grass grazers (Hopcraft et al., 2012; Yoganand and Owen-Smith, 2014). Tall grass grazers select taller grassland and woodland far from permanent water where predator concentrations are low (Harrington et al., 1999; Hensman et al., 2013; Havemann et al., 2022). Calves are generally the demographic stage most vulnerable to predation in large herbivore populations (Gaillard et al., 2000), highlighting the critical value of a fecundity resource having both high forage quality and low predation risk (Gaillard et al., 2000). Loss of these predation refuges can dramatically reduce the size of, or even locally extirpate, large herbivore populations (Bergerud and Elliot, 1986; Harrington et al., 1999; Rettie and Messier, 2000; Wittmer et al., 2005). Therefore, some propose the key resource concept must be expanded to include the critical role that a fecundity resource plays in determining population size through its effects on reproduction rate, calf survival and predation risk (Yoganand and Owen-Smith, 2014).
The primary functional aspect of a saline fecundity resource (Figure 2) is the ability to provide above-reproductive level requirements of essential minerals, such as Na, Ca, Mg, K and P. Failure to attain these minerals in sufficient amounts in forage results in poor or failed reproduction through conception failure, poor foetus development and poor lactation (Belovsky, 1978; Murray, 1995; Parker et al., 2009; Augustine et al., 2011; Velladurai et al., 2016; Kaspari, 2020). Sodium is a critical limiting element for herbivores, regulating osmotic gradients in cells, catalysing the use of N and P in metabolism, maintaining the gut microbiome and helping to deal with tannins in forage (Kaspari, 2020). Of great significance for growth and reproduction, large herbivores cannot use fresh high-quality forage if they don’t obtain sufficient Na in their diet. This is because they cannot maintain critical Na:K ratios in their cells without an additional source of Na because Na:K ratios can be disrupted by fresh, high-quality forage, which is often rich in K but low in Na (Kaspari, 2020). As a consequence of these factors, the availability of Na in landscapes can determine survival and abundance of large herbivores (Belovsky, 1978; Kaspari, 2020). Thus, the occurrence of habitats supporting mineral-rich forage (saline sites) in the landscape forms a critical part of functional heterogeneity for large herbivores in African savannas (McNaughton, 1988; McNaughton, 1990; Murray, 1995; McNaughton et al., 1997; Grant and Scholes, 2006; Prins and van Langevelde, 2008; Anderson et al., 2010; Augustine et al., 2011; Fynn et al., 2014). A saline fecundity resource is generally found in the least productive habitats constrained by soil-moisture stress under saline conditions (Noy-Meir, 1973; Figures 2, 4). Consequently, saline fecundity resources have the shortest growing season of all FAR classes (Breman and De Wit, 1983; McNaughton, 1985; Figure 4). In addition to low-rainfall, additional factors are needed to concentrate minerals in soils to form saline soils. Mechanisms through which minerals are concentrated in soils include paleo-ecological, geomorphological and anthropogenic inputs. These include, volcanic ash deposits (Sinclair, 1979), evaporative concentration in endorheic paleolakes (Fynn et al., 2014; Figure 5A), downslope colluvial deposits (Grant and Scholes, 2006), evapotranspiration and precipitation of salts on islands in wetlands (McCarthy, 2006), and livestock and wildlife dung accumulation to form nutrient hotspots (Muchiru et al., 2009; Anderson et al., 2010; Augustine et al., 2011).
Figure 4 Relationship between annual rainfall and the number of growing season days a year for sites across the Serengeti rainfall gradient (adapted from McNaughton, 1985). Open circles, short grass sites; closed circles, medium grass sites and crosses, tall grass sites. Each resource class (FAR) occurs at progressively higher rainfall, enabling increasing growing-season days per year. Variation in growing season days at a given level of rainfall is likely due to topographic effects on drainage and soil depth, which determine soil moisture availability, hence overlap of resource classes on the rainfall gradient. Note that year-round growth, as occurs in deep-flooded zones of wetlands, only occurs at 1000 mm rainfall per annum (or greater). Permission has been granted to the authors by the publisher to reuse this figure published in Ecological Monographs (McNaughton, 1985).
Figure 5 (A) Migratory zebra in their wet-season range on the Mababe Depression, Botswana, a saline paleolake system, supporting short highly nutritious grasses, rich in energy, protein and minerals – a saline fecundity resource (photo credit, Amanda Stronza). For details on this ecosystem see Fynn et al., 2014). (B) Buffalo grazing on high-quality regrowth during the wet season after prescribed fire in Kruger National Park, South Africa – a non-saline fecundity resource (photo credit, Richard Fynn).
Forage quality declines rapidly with increasing height and biomass because of decreasing digestibility and dilution of nutrients by carbon (Jarrell and Beverley, 1981; Wilmshurst et al., 2000; Owen-smith, 2008). Thus, regrowth of medium height grasses in non-saline habitats, such as Themeda triandra or Digitaria eriantha, after fire or grazing can provide digestible forage rich in energy and protein to promote growth and reproduction (Van der Vijver et al., 1999; Verweij et al., 2006; Anderson et al., 2007; Sensenig et al., 2010; Voeten et al., 2010; Eby et al., 2014). This leafy high-quality forage constitutes a non-saline fecundity resource (Figures 2, 5B). Sedentary large herbivores, such as sable antelope (Hippotragus niger), roan antelope (Hippotragus equinus) and buffalo (Syncerus caffer) favour leafy medium height grasses in woodlands during the wet season as non-saline fecundity resources (Grobler, 1981; Taylor, 1985; Prins, 1996; Hensman et al., 2013; Bennitt et al., 2016; Sianga et al., 2017a). Short grass grazers will select regrowth of these grasses soon after fire or grazing but tall grass grazers only when leaves have exceeded a critical minimum height (Grobler, 1981). The absence of high soil salinity results in non-saline fecundity resources being more productive and having a longer growing season than saline fecundity resources (Noy-Meir, 1973; Figure 4). In these regions, non-migratory large herbivores obtain their mineral requirements by moving to local saline habitats known as nutrient hotspots, as well as in sodic zones (McNaughton, 1988; Grant and Scholes, 2006; Anderson et al., 2010; Augustine et al., 2011). In northern Botswana, non-migratory large herbivores obtain non-saline fecundity resources as leafy medium height grasses in sandveld woodland (Hensman et al., 2013; Bennitt et al., 2016; Sianga et al., 2017a). It is likely that they obtain their saline fecundity resources in adjacent patches of stunted mopane woodland, which are highly saline (Dye and Walker, 1980; Romanens et al., 2019). Because of their differences in phenology and their different functional roles for large herbivores, we distinguish between saline and non-saline fecundity resources in our FAR framework (Figure 2).
A restricted intake resource is forage that is too short to provide sufficient fibre and sufficient bite size to allow optimal intake rates of energy, protein and minerals by large herbivores. Thus, even though its quality may be very high, a restricted intake resource will not be selected preferentially, though it may supplement taller forages of lower quality (Belovsky, 1978; Prins, 1996). Restricted intake resources occur in the most heavily grazed areas of landscapes, and are dominated by short grass species tolerant of heavy grazing (Hempson et al., 2015), hence their restricted nature (Figure 3). Optimal height for maximum intake rates will depend on body size and mouth anatomy (Murray and Illius, 2000; Wilmshurst et al., 2000; Arsenault and Owen-Smith, 2008). Consequently, a grazing lawn dominated by short grass species may be a fecundity resource for wildebeest Connochaetes taurinus and gazelles but a restricted intake resource for buffalo (e.g. Prins, 1996; Bhola et al., 2012; Hopcraft et al., 2012). The analogy for browsers is the effect of thorns reducing intake rate, where again differences in anatomical traits, such as tongue anatomy, can result in kudu and giraffe Giraffa camelopardalis perceiving Acacia spp. as a restricted intake and fecundity resource, respectively (Owen-Smith, 2002). Restricted intake resources promote a balanced diet as components of a ranked sequence of forages whose biomass and quality are inversely related (Owen-Smith and Novellie, 1982; Prins, 1996). For example, during the dry season, buffalo cannot maximise protein intake without eating a restricted intake resource of the short grass, Cynodon dactylon. They also must forage in taller sedgeland to obtain sufficient forage (Prins, 1996). Similarly, moose cannot maximise energy intake without a diet that combines a lower quality but higher intake component of leaves of deciduous trees and a higher quality but lower intake component of forbs (Belovsky, 1978). Several key points become apparent regarding restricted intake resources: (1) they cannot maximize intake of energy or protein when eaten in isolation, only when they supplement a lower quality but higher intake resource and (2) they cannot provide the fibre needed for a balanced diet, which can only come from taller, lower quality forage.
A reserve resource becomes important when more favoured fecundity resources are depleted following cessation of rainfall. Reserve resources have a longer growing season and are intermediate in productivity (Figures 2, 4). For grazers, reserve resources occur in regions of intermediate wetness/productivity on ecological gradients. These regions include areas of intermediate rainfall (e.g. Maddock, 1979; McNaughton, 1985) or intermediate soil depth on midslopes (Bell, 1970; Owen-Smith, 1988) or shallow to intermediate inundated floodplains (Fynn et al., 2015). In addition, being less preferred forage, reserve resources occur in light to moderately grazed parts of landscapes, which support taller grasses and a reserve of forage (Figure 3). The functional basis of a lower-quality but higher biomass reserve of forage for resource-limited periods has been developed through models (Owen-Smith and Novellie, 1982; Illius and O’Connor, 2000; Owen-Smith, 2002; Owen-Smith, 2004), supported by empirical observations (Bell, 1970; Maddock, 1979; Owen-Smith and Cooper, 1987; Walker et al., 1987; Owen-Smith and Cooper, 1989; Owen-Smith, 1994).
Dry reserve resources are less preferred, moderately digestible grasses, growing in dryland habitats that do not remain green during the dry season (Figure 2). They enable large herbivores to maintain intake of energy and protein when availability of fecundity resources is insufficient to maintain required intake rates, especially during the dry season. Dry reserve resources can also help to provide a more balanced diet for large herbivores during the wet season. In this case, the fecundity resources provide the bulk of the energy and nutrient intake and the dry reserve resources provide the fibre intake and gut fill (Prins, 1996). Thus, dry reserve resources can make an important contribution to diet in both the wet and dry season, and consequently can also be referred to as staple resources (Owen-Smith, 2002, p255). Examples of dry reserve resources are medium-height grasses of intermediate quality, such as T. triandra, which dominates on midslopes (Bell, 1970) or in intermediate rainfall regions (McNaughton, 1983; McNaughton, 1985). In Serengeti National Park (SNP) wildebeest temporally move to these mid-grass-dominated habitats during periods of low-rainfall within the wet season or once rains have ceased at the commencement of the dry season (Maddock, 1979). This same movement pattern can be observed at a local landscape-scale with non-migratory large herbivores on productivity gradients determined by increasing soil depth down the catena in western Serengeti (Bell, 1970). Use of T. triandra as a reserve forage during the dry season is widespread across Africa for many species, including warthog Phacochoerus aethiopicus, impala Aepyceros melampus, gazelles, wildebeest, zebra Equus quagga, buffalo and white rhino Ceratotherium simum (Maddock, 1979; McNaughton, 1985; Owen-Smith, 1988; Venter and Watson, 2008; Kleynhans et al., 2011). Reserves of forage as standing hay during the dry season (a dry reserve resource) would be the major food source for large herbivores in semi-arid savannas of PAs or cattle ranches, where wetlands or high-rainfall regions (>1000mm annual rainfall) are absent.
In addition to reserves of forage that dry out (senesce) during the dry season, grasses and sedges growing in wetlands or in high-rainfall regions, which remain green during the dry season (Figures 6B, 7A), constitute an important reserve of green forage for large herbivores (a green reserve resource). The protein and energy content of forage drops dramatically as it dries and senesces (Sinclair, 1975; Ellis and Swift, 1988; Owen-smith, 2008), underscoring the value of green forage during the dry season. Widely distributed medium and tall floodplain grasses, such as Echinochloa spp., Panicum repens, Setaria sphacelata, Ischaemum spp., Loudetia simplex, Leersia hexandra and a wide range of sedge species, are characteristic of shallow- and intermediate-flooded zones on African floodplains. These grasses form important reserves of green forage for species such as wildebeest, zebra, buffalo, kob (Kobus kob), lechwe (Kobus leche), warthog and hippo over the early- and mid-dry season (see Fynn et al., 2015). Green forage is available longer into the dry season with increasing annual rainfall (Breman and De Wit, 1983; McNaughton, 1985) or with increasing flood depth and duration (Vesey-FitzGerald, 1960; Sheppe and Osborne, 1971; Tinley, 1977; Kamweneshe, 2000; Fynn et al., 2015). Thus, large herbivores follow the receding floodwaters towards the deeper flooded areas as the dry season progresses (Fynn et al., 2015; Keemekae, 2022). In moist riparian habitats with a dense tree canopy, Panicum maximum remains green over the early dry season and provides an important source of green forage for several large herbivore species (Owen-Smith, 1988; Ben-Shahar, 1991; Kleynhans et al., 2011).
Figure 6 Forage biomass and greenness dynamics across seasons in northern Botswana (Fynn et al., 2014). Note the importance of wetland habitats (dambos and floodplains) for providing sufficient biomass (A) and greenness (B) of forage during the late dry season. Wet season grass biomass (C) and wet season grass greenness (D). Permission has been granted to the authors by the publisher to reuse this figure published in the South African Journal of Wildlife Research (Fynn et al., 2014).
Figure 7 (A) Buffalo foraging on medium-height green forage on floodplains of the Savuti Channel, Botswana during the late dry season – a green reserve resource (photo credit, Richard Fynn). (B) Zebra and cattle on floodplains of the Okavango Delta, Botswana benefiting from fresh regrowth after fire during the late dry season – a bridging resource (photo credit, Richard Fynn). The late dry season is the hottest and driest time of the year in African savannahs with at least four to five months since the end of the rains, hence forage quantity and quality in drylands has reached its lowest levels. In this context, access to green reserve resources and bridging resources is exceptionally important for maintaining energy and protein intake during the late dry season.
At the wet end of rainfall- or flooding-induced productivity gradients (Figure 2), where growth can be maintained all year (Figures 4, 6), tall, robust grasses and sedges and reeds (Phragmites spp.) may dominate. These robust plants, when mature, provide forage that is too coarse and indigestible for use by large herbivores in most years, so they receive the least grazing pressure (Figure 3). In the event of a drought year, however, these coarse, high-biomass species may provide the only available forage, which despite its low quality, provides some energy and protein, thus reducing the rate of use of body stores over the drought period. Ungrazed, low-quality forage can buffer large herbivore populations against collapse during extreme drought events (e.g. Walker et al., 1987; Macandza et al., 2004); hence the term buffer resource (Owen-Smith, 2002, p255 Figure 2) or key resource (Illius and O’Connor, 2000).
Typical examples would be tall, coarse grasses such as Hyparrhenia spp. and Loudetia kagerensis in the highest rainfall regions of the Serengeti (1000-1200mm). These provide “…the last reservoir of green forage available at the end of the dry season” (McNaughton, 1985). The sedges of the Amboseli Swamps in Kenya provided critical buffer resources during the drought of 1969, supporting an estimated 75 000 kg of large herbivore biomass km-2 (Western, 1973). Grasses with high concentrations of aromatic compounds, such as various Cymbopogon spp. and Bothriochloa spp., are often ignored by large herbivores in years with normal rainfall (e.g. McNaughton, 1983; Perrin and Brereton-Stiles, 1999; Macandza et al., 2004), but are readily eaten in dry years when more preferred forages have been depleted (e.g. Macandza et al., 2004). When grasses and sedges are depleted during the dry season and drought years, cattle, elephant and a variety of other large herbivores, including wildebeest, increase intake of browse (Hensman et al., 2012; Selebatso et al., 2018; Weeber et al., 2020). Browse is forage at the wet end of moisture gradients because trees with their deep root systems can access the water table during the dry season, thereby providing green forage in drylands when grasses have dried out. The key difference between reserve and buffer resources is whether they are eaten every dry season (reserve) or only during drought years (buffer). The differentiation of reserve and buffer resources is determined by habitat productivity effects on the biomass and quality of forage or by species-specific effects on the concentration of aromatic compounds (phytochemicals).
A bridging resource (Owen-Smith, 2002, p243, 344) provides a critical ‘steppingstone’ of relatively energy- and protein-rich forage across seasonal periods of resource scarcity (late dry season). Without access to bridging resources in the late dry season large herbivores may suffer declining body stores (Owen-Smith and Cooper, 1989; Owen-Smith, 2002, p344; Parrini and Owen-Smith, 2010). In addition, bridging resources may play a critical role in enabling pregnant females to meet their rapidly increasing energy and protein intake requirements as their foetus enters its final months of development before the rains arrive (Taylor, 1985). Thus, a bridging resource substitutes for a fecundity resource at the end of the dry season when fecundity resources are dry and depleted and nutritional and heat stress for large herbivores is at its highest (in dryland savannas lacking bridging resources). Consequently, apart from its importance for preventing loss of body condition, a bridging resource is a critical resource for ensuring optimal foetus development and ultimately high birth rates and high calf size at birth. Calf size at birth determines calf survival and sets the stage for maximum reproductive output over the lifetime of female calves (Michael et al., 2019). To meet elevated energy and protein demands, a bridging resource must be relatively digestible and rich in energy and protein (Figures 7B, 8). During the late dry season, bridging resources are provided by fresh regrowth of grasses and sedges after fire or grazing in habitats at the wet end of ecological productivity gradients (Figures 2), such as wetlands or very high rainfall areas (Vesey-FitzGerald, 1960; Breman and De Wit, 1983; McNaughton, 1985; Parrini and Owen-Smith, 2010; Fynn et al., 2015; Figure 7B).
Figure 8 Estimated protein requirements for buffalo relative to estimated protein intake in Matusadonna National Park, Zimbabwe (adapted from Taylor, 1985). As the lake level drops during the late dry season (September to mid-November), which is the hottest and most resource limited time of the annual cycle, the lake shore grasslands become exposed allowing new growth of Panicum repens, thereby forming a bridging resource that elevated protein intake by buffalo well above requirements (grey shaded area). Open circles, estimated protein requirements; closed circles, estimated protein intake. Dr Russell Taylor has granted permission to the authors to reuse this figure from his PhD thesis (Taylor, 1985).
For example, regrowth of Panicum repens resulted in a positive protein balance for buffalo during the hottest and most resource limited time of the annual cycle in lake shore grasslands after flood recession and warming during the late dry season in Matusadona National Park, Zimbabwe, (Taylor, 1985; Figure 8). Thus, while large herbivores in dryland savanna regions during the late dry season are generally failing to meet even baseline maintenance requirements for energy and protein (e.g. Sinclair, 1975), these buffalo were exceeding the elevated requirements for a pregnant female carrying a rapidly developing foetus (Figure 8). In addition to the production of bridging resources in wetland habitats, high-rainfall regions (>900 mm m.a.p.) also have sufficient soil moisture during the late dry season to support bridging resources. For example, in the high rainfall regions of the Serengeti/Mara system up to 40% of annual rainfall may occur during the dry season, stimulating regrowth after grazing or fire (McNaughton, 1985).
Historically, ecologists and agronomists focused on the negative effects of secondary compounds on large herbivores. They were viewed as digestion inhibitors and toxins that set limits on the amount of forage and animal could consume (Rhoades, 1979). Thus, agronomists selected against secondary compounds in the forage varieties they developed, rather than considering the importance of diverse arrays of plant species and secondary compounds in promoting health (Freeland and Janzen, 1974). As a result, we little appreciate how herbivores might benefit from secondary compounds, and we know little about their roles in the movement patterns that emerge as wild or domesticated herbivores forage on different plant species across landscapes.
As case in point, tannins, once notorious for their adverse effects on herbivores (Rhoades, 1979), are increasingly recognised as important in health (Min et al., 2003). Eating plants high in tannins is a way for herbivores to reduce internal parasites (Min and Hart, 2003). Tannins also alleviate bloat by binding to proteins in the rumen (Waghorn, 1990; Tanner et al., 1995; Min et al., 2006). By making protein unavailable for digestion and absorption until it reaches the more acidic abomasum, tannins also enhance nutrition by providing high-quality protein to the small intestines (Barry et al., 2001), which enhances immune responses and increases resistance to gastrointestinal nematodes (Niezen et al., 2002; Min et al., 2004). The increase in essential and branched-chain amino acids improves reproductive efficiency (Min et al., 2001). Tannins in the diet are a natural way to reduce methane emission in ruminants (Woodward et al., 2004), a key issue regarding ongoing efforts to diminish the influence of livestock on global warming. Finally, tannins and many other secondary compounds eaten in modest amounts by herbivores can improve the quality of meat for human consumption (van Vliet et al., 2020; van Vliet et al., 2021).
Phytochemically-rich diets for large herbivores include primary compounds, such as energy, protein, minerals, and vitamins, and a host of other so-called secondary compounds—including but not limited to phenolics, terpenoids, and alkaloids—that can also have health benefits (Engel, 2002; Provenza et al., 2015; Provenza, 2018). While meeting needs for macronutrients requires a high proportion of energy- and protein-rich plants in the diet, this is not likely to be the case for meeting medicinal needs. Targeted intake of plants rich in specific secondary metabolites will likely suffice for medicating therapeutically and eating small amounts of a variety of plants regularly will suffice prophylactically. Thus, the dominant plants in the diet are likely selected for nutritional purposes, though they may also provide serendipitous prophylactic effects against parasites and disease, while plants rarely eaten might be selected for therapeutic medicinal purposes (Provenza, 2018).
The importance of secondary compounds in the health, productivity, and well-being of herbivores is important given the rise in drug-resistant bacteria and parasites (Provenza, 2018). Reliance on synthetic drugs increases greatly for livestock fed low diversity, phytochemically impoverished diets, such as animals restricted to feeding on monospecific planted pastures or grain-based rations in feedlots (Provenza et al., 2015; Provenza et al., 2019). Conversely, livestock given access to phytochemically rich pastures are healthier and more productive due to enhanced immune responses, fewer internal parasites, and less susceptibility to diseases (Provenza and Villalba, 2010). The logical conclusion is that wild herbivores, who lack access to veterinary inputs, must counter disease and parasites by eating a wide variety of phytochemicals in the diverse plant communities of their landscapes.
While any primary or secondary compound can be toxic when eaten in excess, they can also have health benefits when consumed in moderation and combinations with different secondary compounds (Provenza et al., 2003; Provenza et al., 2015). Plant diversity thus influences diet diversity (Pansu et al., 2019) and diversity of medicinal resources (French et al., 2018), which all affect health (Provenza et al., 2015; Provenza, 2018; Provenza et al., 2019). Phytochemicals in diverse mixes of rangeland plants reduce parasite egg and larval production (Moreno et al., 2012; Acharya et al., 2014; Mengistu et al., 2017), and worm loads (Lange et al., 2006; Shaik et al., 2006). Combinations of secondary compounds—rather than reliance on individual compounds to which parasites can easily adapt—maintain various parasites at low levels and play a vital role in maintaining body stores and increasing vigour by enhancing immune responses and reducing losses of nutrients (Provenza and Villalba, 2010).
Livestock can self-medicate therapeutically and prophylactically (Provenza, 2018). For example, in the Mediterranean scrublands of Israel, Damascus and Mamber breeds of goats have different proclivities to use the high-tannin foliage of Pistacia lentiscus (Landau et al., 2010). Damascus goats eat Pistacia lentiscus in ways that act prophylactically. Conversely, Mamber goats, who reluctantly dine on Pistacia lentiscus, use foliage from the plant only therapeutically. Either way, use of Pistacia lentiscus alleviates nematode infection in all goats. Likewise, goats infected with gastro-intestinal nematodes eat more tannin-rich browse than non-infected goats, which strongly reduces faecal egg counts of the parasite (Amit et al., 2013). Similar results were shown for sheep (Lisonbee et al., 2009; Villalba et al., 2010; Juhnke et al., 2012). Medicinal resources are not restricted to antimicrobial and anthelmintic effects but can act on physiological processes and organ function. For example, glycosides at the correct dose may improve heart function (Durmic and Blache, 2012).
Livestock learn to use different medicines to rectify different maladies. Sheep learn to use sodium bicarbonate to alleviate illness from eating too much grain, polyethylene glycol to counteract food containing high levels of tannins, and dicalcium phosphate to counteract food with high levels of oxalic acid (Villalba et al., 2006). When fed grain or food with tannins or food with oxalic acid, sheep choose the medicine that rectifies the malady.
While livestock can self-medicate therapeutically, eating a variety of phytochemically rich plants also enables health prophylactically and to protect against diseases and pathogens through anti-microbial, anti-parasitic, immune-boosting, and anti-inflammatory properties (Provenza and Villalba, 2010; Villalba et al., 2010; Villalba et al., 2017; Beck and Gregorini, 2020). Phytochemicals reduce oxidative stress and inflammation, and enhance immunocompetence (Provenza, 2018). Compared with pastures that lack plant diversity, animal welfare and wellbeing—including nutritional, physiological, and immunological status—all improve when livestock forage on diverse mixtures of plants (Villalba et al., 2017; Villalba et al., 2019; Beck and Gregorini, 2020; Redoy et al., 2020; Garrett et al., 2021; Nakajima et al., 2021; Garrett et al., 2022). This helps explain why they require fewer antiparasitic drugs or antibiotics; they also have low levels of morbidity and mortality compared with animals forced to forage on pastures with few plant species devoid of phytochemical richness (Glasser et al., 2009; Provenza et al., 2019).
For many herbivores, the bulk of any meal is composed of 3 to 5 plants, but they often eat small amounts of 50 to 75 plants during the day. Historically, we did not appreciate the medicinal properties of these minor components of the diet, best eaten in variety and small doses. In that regard, large herbivores feeding in diverse landscapes eat a high-diversity of plant species that likely promote their health (Owen-Smith and Cooper, 1987; Kartzinel et al., 2015). Kudu at Nylsvlei Nature Reserve in South Africa eat 60 woody species and over 100 forb species (Norman Owen-Smith pers. com.). While the amount of phytochemically rich plants (forbs and woody species) in the diet of large herbivores varies greatly across species, most grazers include a significant amount of these non-grass/sedge species in their diets (Kartzinel et al., 2015; Selebatso et al., 2018). For example, cattle and buffalo included over 40% of non-grass species in their wet season diets (Kartzinel et al., 2015), while wildebeest, gemsbok Oryx gazella and springbok Antidorcus marsupialis included 5%, 29% and 56% of these non-grass species in their dry season diets, respectively (Selebatso et al., 2018), although non-grasses in some wildebeest populations can exceed 33% during the dry season (Weeber et al., 2020).
Complementarities among secondary compounds enable herbivores to meet needs for energy and protein while eating health-promoting phytochemicals. For example, mule deer eat more when offered sagebrush and juniper (12.3 g/kg BW), plants with different terpenes, than when they are offered only sagebrush (4.2 g/kg BW) or juniper (7.8 g/kg BW) (Smith, 1959). Lambs eat more of foods that contain either nitrates or oxalates than when they can eat food that contains only one of these secondary compounds (Burritt and Provenza, 2000). Availability of nutritious alternatives and experience learning to mix different compounds both influence food choice when the preferences of lambs with 3 months’ experience mixing tannin, terpenes, and oxalates were compared with lambs naive to the toxin-containing foods (Villalba et al., 2004). Throughout the study, lambs with experience ate remarkably more than naive lambs of the foods containing the secondary compounds, whether access to nutritious alternatives was ad libitum (811 vs. 71 g/d) or restricted (1509 vs. 607 g/d).
While complementarities among secondary compounds are an important but little understood area of plant-herbivore interactions, even less is known about how the sequences of eating plants with different compounds affects foraging. Sheep eat much more food with terpenes when they first eat food with tannins (Mote et al., 2008). These findings are consistent with landscape-level studies that show ewes with a high preference for sagebrush, a shrub high in terpenes, also consume more bitterbrush, a shrub high in tannins (Seefeldt, 2005). Cattle decrease time eating endophyte-infected tall fescue when they first graze tall fescue alone for 30 minutes followed by trefoil, alfalfa, or alfalfa-trefoil combination for 60 minutes (Lyman et al., 2011). Conversely, when the sequence is reversed, cattle forage actively on trefoil, alfalfa, or trefoil-alfalfa combination and then forage actively on fescue throughout the 90-min meal. These patterns of foraging are similar for sheep (Lyman et al., 2013). By herding in grazing circuits, shepherds in France use empirical understanding of complementarities and landscape-level plant diversity to stimulate food intake and more fully use the range of plants available (Meuret and Provenza, 2015).
As with nutritional resources, the distribution and variety of medicinal resources can be linked to ecological gradients across landscapes because beta diversity is maximised across ecological gradients with different soil nutrient and moisture characteristics (Figure 2). Ecological gradients influence plant composition and beta diversity, while disturbance gradients promote alpha and beta diversity (Burkepile et al., 2017), both of which promote phytochemical diversity in large herbivore diets (Provenza et al., 2009; Pansu et al., 2019). The concentrations of primary and secondary compounds are influenced by environmental conditions – soil fertility, soil moisture, sunlight, past herbivory – and the associated plant composition and diversity (Bryant et al., 1983; Coley et al., 1985). Changes in phytochemicals produced by plants with changing soil nutrient and moisture status (Bryant et al., 1983; Coley et al., 1985), combined with species-specific effects on the nature of phytochemicals produced, maximises phytochemical diversity across ecological gradients.
Large herbivores may respond to heterogeneity in plant phytochemicals across ecological gradients through adaptive foraging driven by learned diet and habitat selection behaviors for specific plant species and combinations of species and their phytochemicals (Provenza et al., 2007; Provenza, 2018). Thus, large herbivores may respond therapeutically to disease and parasites but can also benefit prophylactically by having high diversity diets through foraging across ecological gradients (Figure 1). Large herbivores that have learned to use these phytochemically-rich landscapes have an advantage of living in landscapes that are nutrition centres and pharmacies with vast arrays of primary and secondary compounds. The prophylactic and therapeutic basis of plant secondary metabolites for enabling large herbivores to deal with various diseases, parasitic infections, and toxic compounds in forage is, thus, well established. The implication is that secondary compounds have key functional roles as medicinal resources for sustaining the health of large herbivores, and thereby the productivity of their populations, and should be considered as a class of FARs (Figure 2).
When considering FARs for large herbivores it is essential to recognise that their body size, digestive anatomy and mouth anatomy greatly affects the functionality of different resource types for maximising intake rates (Murray and Illius, 2000; Arsenault and Owen-Smith, 2008; Codron et al., 2008; Hopcraft et al., 2012). Thus, selection of specific resource classes and habitat types is dependent on abiotic and biotic factors determining the types of resources and predation risk in combination with the contextual factors of anatomical traits and environmental variation, as well as anthropogenic impacts (Figure 1). Body size is an important factor determining selection for different grassland structural states because greater intake requirements with increasing body size necessitates consumption of more abundant lower quality forage to meet their intake requirements (Hopcraft et al., 2010; Sensenig et al., 2010; Müller et al., 2013; Steuer et al., 2014). However, specialised mouth adaptations may greatly modify intake rates of large herbivores on different grass heights (Murray and Illius, 2000), resulting in mouth anatomy being a key factor determining selection for grass height (Arsenault and Owen-Smith, 2008). Narrow-mouthed large herbivores (beyond a certain body size) cannot maintain sufficient intake rates on short grass, with the reverse being true for broad-mouthed large herbivores on tall grass (Murray and Illius, 2000).
Mouth anatomy (together with body size) determines the height of grass considered as a fecundity resource during the wet season for the large herbivore species in question. Narrow-mouthed grazers, such as roan and sable antelope, waterbuck (Kobusellipsiprymnus) and reedbuck (Redunca arundinum), can be more selective of plant parts, selecting fresh digestible leaves on medium height and tall grasses while avoiding unintentional intake of indigestible stems and low-quality dead leaves (Jungius, 1971; Grobler, 1981; Schuette et al., 1998; Murray and Illius, 2000; Knoop and Owen-Smith, 2006; Kassa et al., 2007; Havemann et al., 2022). During the wet season, roan and sable antelope eat high-quality leafy grasses of medium height, such as Andropogon spp., Panicum maximum, Panicum coloratum, Digitaria eriantha and Andropogon and Hyparhenia spp (Grobler, 1981; Schuette et al., 1998; Knoop and Owen-Smith, 2006; Hensman et al., 2013; Havemann et al., 2022). Thus, these medium height leafy grasses, as opposed to lawn grasses of short grass grazers, can be fecundity resources for narrow-mouthed grazers. The greater selective capacity of narrow mouthed grazers enables them to switch to very tall grasses (>1.8m) growing in drainage sumps and to browse in woodlands during the dry season facilitating intake of green forage when most grasses have dried out (Schuette et al., 1998; Knoop and Owen-Smith, 2006; Kassa et al., 2007; Hensman et al., 2012; Selebatso et al., 2018). Trees and shrubs have much deeper root systems than grasses, enabling them to access soil moisture in deeper layers and maintain green leaves much later into the dry season than grasses. Very tall grasses growing in poorly drained sumps (wet meadows, vleis, dambos) also retain green leaves much later into the dry season than short grasses (Knoop and Owen-Smith, 2006; Fynn et al., 2014). Most narrow-mouthed tall grass grazers it appears are non-migratory, often living in woodlands all year round (Jungius, 1971; Grobler, 1981; Schuette et al., 1998; Murray and Illius, 2000; Knoop and Owen-Smith, 2006; Kassa et al., 2007), where the only source of green forage in the dry season is the leaves of woody species or on very tall grasses in poorly drained sumps.
Thus, having a narrow mouth is a key adaptation for being able to forage effectively in lower quality taller grass habitats in backcountry woodlands, thereby avoiding competition with high densities of short grass grazers in high-quality short grasslands. Short grasslands are generally favoured by wildebeest, gazelles, impala, warthog and zebra (Arsenault and Owen-Smith, 2008; Bhola et al., 2012). Thus, variation in mouth anatomy provides a key mechanism facilitating coexistence among a guild of large herbivore species by enabling spatial partitioning of foraging sites across landscapes. In addition, by restricting themselves to lower quality tall grasslands in backcountry woodlands, narrow-mouthed grazers avoid high densities of predators associated with high densities of grazers (Harrington et al., 1999).
By contrast, the broad-mouths of short grass grazers, such as wildebeest, enable greater bite size on short grasses than narrow-mouthed grazers (Murray and Illius, 2000). However, their inability to forage effectively on tall grasses and browse (Murray and Illius, 2000), constrains broad-mouthed grazers to foraging dominantly on shorter grasses throughout the year with much less browse intake during the dry season than narrow-mouthed grazers (Selebatso et al., 2018). Consequently, broad-mouthed grazers often migrate to floodplains or high-rainfall areas during the dry season where they can find grasses retaining green leaf (Maddock, 1979; Fynn et al., 2014; Naidoo et al., 2014; Bennitt et al., 2016; Sianga et al., 2017a). With broad-mouthed grazers requiring short, high-quality forage they favour fresh regrowth of grasses after fire or grazing (Vesey-FitzGerald, 1960; Sensenig et al., 2010; Hempson et al., 2015). Spatial partitioning of foraging sites due to mouth anatomy is also observed with woodland caribou (Rangifer tarandus) in Boreal regions. Woodland caribou have specialised mouth adaptations to forage on lichens in old growth forests, thereby avoiding much overlap of moose, and their associated predators (wolves), where moose favour browse on regrowth in clear felled forests (Bergerud and Elliot, 1986; Rettie and Messier, 2000; Wittmer et al., 2005). It appears, therefore, that mouth anatomy plays a critical role in niche diversification and coexistence among large herbivores in both African and North American ungulates.
Several anatomical innovations result in divergences from the typical seasonal foraging patterns of narrow and broad mouthed grazers. While buffalo have a broad mouth, their very large body size combined with use of their tongues to increase bite size, necessitates that instead of short grasses being favoured, they prefer medium height leafy grasses in areas with lower concentrations of grazers (Bhola et al., 2012). Consequently, buffalo favour the same backcountry woodland habitats as roan and sable antelope during the wet season (Vesey-FitzGerald, 1960; Taylor, 1985; Prins, 1996; Bennitt et al., 2016; Sianga et al., 2017a). With their broad mouths, however, buffalo cannot forage effectively for green leaf on tall grasses and browse in woodlands during the dry season so they retreat to floodplains at this time (Vesey-FitzGerald, 1960; Bennitt et al., 2016; Sianga et al., 2017a). In addition, body size can determine predation risk for a large herbivore, which can influence habitat selection for large herbivores smaller than buffalo (Sinclair et al., 2003; Hopcraft et al., 2012; Yoganand and Owen-Smith, 2014). The implication is that a habitat type may be essential for the viability of a large herbivore population, not only through its specific nutritional resources but also by minimising predation risk. For example, wildebeest benefit from open, short grass habitats, not only because they provide higher forage quality than tall grass habitats but also because they provide much better visibility for the detection of predators (e.g. Yoganand and Owen-Smith, 2014). The small-bodied antelope, Oribi (Ourebia ourebi), forage in short grass habitats for the better forage quality and visibility that they provide but rest in tall grass habitats to avoid detection (Everett et al., 1991). Wildebeest drop their calves in open, high visibility habitats, whereas sable and roan antelope hide their calves in tall grass and scrub far from areas with high densities of predators. Large body size also facilitates greater ability to survive on low-quality forage by being more able to cope with processing coarse forage (having a larger mouth and chewing ability) and by having a lower metabolic rate relative to food intake (Müller et al., 2013).
Thus, body size and mouth anatomy are critical morphological adaptations that enable a wide range of different foraging strategies and predator avoidance mechanisms across landscapes and seasons. There appear to be three main foraging and predator avoidance strategies of large herbivore species over the annual cycle. First, those with specialised broad mouths (e.g. wildebeest) or small enough body size (e.g. impala and gazelles) favour habitats with high visibility, thereby minimising predation risk: they rely on early detection and flight from predators. Second, those with narrow mouths (but greater body size than gazelles) can forage in tall grass habitats and woodlands far from high concentrations of short grass grazers where predator densities are high: they rely on spatially avoiding predators. Their ability to use browse effectively in the dry season enables them to remain all year round in these habitats (Hensman et al., 2013; Havemann et al., 2022). Third, those with a tongue sweep strategy for increasing bite size that constrains them to foraging on medium height leafy grasses in woodlands far from high concentrations of short grass grazers (e.g. buffalo). Their large body size and defensive abilities in large herds enables them to forage in woodlands and scrub where visibility is low: they rely on fending off predators. Another anatomical trait that also promotes niche differentiation on forage quality gradients is gut anatomy, with hind gut fermenters being able to ingest more low-quality forage than ruminants, which outweighs any advantage of longer retention times on digestive efficiency (Müller et al., 2013). These different anatomical traits account, to a large degree, for coexistence of the wide diversity of large herbivore species across heterogeneous African savanna landscapes. However, considering the emphasis that has been placed on body size in the Jarman-Bell hypothesis, it appears that mouth anatomy has been greatly neglected as a mechanism of coexistence and in can even override the effect of body size as a determinant of grass height selection e.g. with white rhino (Arsenault and Owen-Smith, 2008).
In this synthesis we attempt to provide an understanding of the range of functional forage resource classes and medicinal resources (FARs) required by large herbivore grazers over the annual cycle in the context of their anatomical traits (Figure 1). Additionally, we develop a framework linking the FAR classes to ecological productivity gradients at various scales determined by spatial variation in soil moisture availability on topographic, rainfall or hydrological gradients at various scales (Figure 3), as well as by spatial variation in grazing intensity (Figure 3). We show how large herbivores rely on a diverse range of functional resource classes (FARs) to enable them to respond to seasonal and interannual variation in forage quantity and quality and to their own variation in intake requirements for various resources. Consequently, reducing access by large herbivores to any class of FARs will negatively impact their populations (Illius and O’Connor, 2000; Owen-Smith, 2002; Owen-Smith, 2004). We also introduce the concept of medicinal resources as a critical functional adaptive resource class that has been all but ignored in large herbivore foraging ecology theory. We show that productive and healthy herbivore populations are dependent upon access to a wide diversity of plants and the associated phytochemicals that they provide for therapeutic and prophylactic defence against toxins, parasites and disease.
We also show how the perception of the functionality of these resources by various large herbivore species is determined by their anatomical traits, such as body size, mouth anatomy and digestive anatomy, which also determines predation avoidance strategies. Body size determines vulnerability to predation, whereas various combinations of body size, digestive anatomy and mouth anatomy determine selection for grass height and also predation avoidance strategies. Although body size is an important trait to account for, mouth anatomy can play a dominant role in determining selection for different grassland structural states. For example, while larger body size generally results in selection for a taller grass, lower quality diet, the exceptionally broad mouth of the very large bodied white rhino enables it to forage effectively on short grazing lawns (Arsenault and Owen-Smith, 2008). By contrast, the narrow mouth of the small-bodied reedbuck enables it to forage effectively in tall grassland (Jungius, 1971). Consequently, niche diversification and large herbivore species coexistence is determined by the various combinations of body size, digestive anatomy and mouth anatomy.
Access to the full range of FARs along environmental productivity gradients is being compromised by anthropogenic effects, such as ecosystem fragmentation and climate change. Lack of access to reserves of forage in the more productive regions of ecological gradients can result in declining body stores and starvation during the dry season and droughts, leading to population collapse (Walker et al., 1987; Ellis and Swift, 1988; Illius and O’Connor, 2000; Owen-Smith, 2004). Thus, the wettest end of productivity gradients is critical for preventing starvation during droughts (a buffer or key resource), thereby pointing to the importance of these wet zones for adaptation to predicted increased temperature and drought frequency under climate change (Cai et al., 2014; Engelbrecht et al., 2015). Lack of access to high-quality (fecundity) resources in the less productive regions of ecological gradients compromises growth and reproduction, which can result in declining population size (Gaillard et al., 2000; Owen-Smith, 2004; Parker et al., 2009). It becomes clear, therefore, that wet season fecundity resources should also be considered as key resources, not just dry season reserve and buffer resources, because large herbivore populations cannot attain a large population size without access to fecundity resources. In addition, without access to appropriate plant diversity distributed across ecological gradients, the health and productivity of large herbivore populations is expected to suffer. The most productive and stable herbivore populations will, therefore, be those with access to the full range of FARs on ecological productivity and disturbance gradients.
Fecundity in large herbivore populations is determined not only by sufficient intake of energy and protein, but also by sufficient intake of key minerals. With minerals such as Ca, Na and P being essential for pregnancy and lactation, as well as for several essential physiological processes (Kaspari, 2020), most known migrations in Africa are to saline regions for the wet season (Maddock, 1979; Breman and De Wit, 1983; Joos-VandeWalle, 2000; Bartlam-Brooks et al., 2011; Fynn and Bonyongo, 2011; Bradley, 2012; Fynn et al., 2014; Naidoo et al., 2014). Non-migratory large herbivores use patchy saline areas in the landscape during the wet season, such as sodic sites and nutrient hotspots (McNaughton, 1988; Grant and Scholes, 2006; Anderson et al., 2010; Augustine et al., 2011). Saline zones of ecological gradients support the lowest plant productivity along these gradients, having the shortest growing season and the lowest biomass production (Figure 4). As conditions progressively dry out after the last rains and fecundity resources and drinking water become depleted, large herbivores tend to move up the productivity gradient as the dry season progresses, selecting taller lower quality grasses and sedges, which function as ungrazed reserves of forage (Bell, 1970; Fryxell and Sinclair, 1988; Hopcraft et al., 2010; Fynn et al., 2015). Large herbivores increasingly forage in deeper-flooded zones of wetlands as the dry season proceeds (Vesey-FitzGerald, 1960; Sheppe and Osborne, 1971; Tinley, 1977; Kamweneshe, 2000), especially during droughts (Western, 1973). Similarly, wildebeest migrate further up the rainfall gradient in Serengeti in dry years than in wetter years (Maddock, 1979).
With these observations as a background, our FAR-ecological gradient framework has important implications for research and for conservation and development planning across broad landscapes, especially in the context of climate change (Cai et al., 2014; Engelbrecht et al., 2015) and accelerating fragmentation of landscapes (Ogutu et al., 2011; Morrison and Bolger, 2014; Løvschal et al., 2017; Veldhuis et al., 2019). For example, data on topography, soils, hydrology, rainfall and remote-sensed seasonal NDVI can be modelled to produce soil moisture/productivity gradients in a GIS, allowing the development of a spatially explicit FAR resource map. FAR mapping can be used to guide development of new PAs or upgrades of existing PAs, by assessing the optimal spatial configuration that a PA requires to include the full range of FARs needed to maintain productive and stable large herbivore populations. FAR mapping will also provide an objective approach to habitat suitability assessments for different large herbivore species, allowing an assessment on whether certain large herbivore species can be introduced to a conservation area and where certain forage resource deficits may occur.
Pereira and Gregorini (2022) show how remote sensing and GIS can be used to assess the spatial distribution of phytochemically-rich plants thus enabling people to visualize potential healthscapes for wild or domestic herbivores. The maps they created using advanced image classification of botanical composition and phytochemicals show the distribution of phytochemicals in plants in ways that enable managers to make better grazing management decisions for more productive, sustainable, and healthy grazing systems. Assessment of healthscapes is a paradigm shift in rangeland management thinking with potential applications for conservation planning, such as in determining the optimal spatial configuration of PAs.
In addition, modelling can be used to assess the role that different FAR classes play in determining the productivity and stability of large herbivore populations, such as what would happen if access to certain FAR classes was lost through ecosystem fragmentation, or if drought frequency was increased under climate change or how the wet end of ecological gradients mediates the impacts of drought (e.g. Illius and O’Connor, 2000; Owen-Smith, 2002, p255; Owen-Smith, 2004). Modelling could also assess how management options, such as implementing fire management or strategic livestock grazing to stimulate regrowth of short, high-quality forage in drylands during the wet season (a non-saline fecundity resource), or in wetlands or high-rainfall regions during the dry season (a bridging resource), would affect large herbivore population productivity, after accounting for large herbivore anatomical traits (e.g. Gordon, 1988; Fynn et al., 2016). Increasing the coverage of short grassland may benefit broad mouthed grazers, such as wildebeest and white rhino, but will likely negatively impact narrow-mouthed grazers (Harrington et al., 1999; Murray and Illius, 2000; Arsenault and Owen-Smith, 2008; Bhola et al., 2012). It is, therefore, essential to consider herbivore anatomical traits to understand how they perceive and respond to various FAR classes and to predation risk (Harrington et al., 1999; Murray and Illius, 2000; Arsenault and Owen-Smith, 2008). For example, populations of narrow-mouthed, tall grass grazers, such as roan and sable antelope in Kruger National Park collapsed after placement of artificial water points in backcountry waterless regions far from perennial rivers (Harrington et al., 1999). This unintended consequence of management could have been avoided by consideration of how FARs are distributed on grazing gradients determined by distance to perennial water (Sianga et al., 2017b; Figure 3). Artificial water points may disrupt these landscape-scale grazing gradients, with various consequences for large herbivores with different anatomical traits (Harrington et al., 1999; Sianga et al., 2017b).
We provide a forage resource-environmental gradient framework for understanding how large herbivores in African savannas assess and respond to variability in forage resources and their seasonal demands for resources, as well as to predators, parasites and disease. We demonstrate that various large herbivore foraging responses and predation avoidance strategies are mediated by their anatomical traits, which also facilitates their coexistence. We highlight that mouth anatomy has been neglected as a key anatomical trait determining large herbivore selection for grass height. Our framework provides a basis for refining and developing our understanding of factors affecting conservation of diverse guilds of large herbivores in African savannas under landscape fragmentation and climate change.
RF conceptualised and developed the nutrition aspects of functional adaptive resources and the mechanisms through which they are distributed on ecological gradients. FP conceptualised and developed the medicinal aspects of functional adaptive resources. Both authors contributed towards the writing and editing of the manuscript. All authors contributed to the article and approved the submitted version.
Innumerable discussions with Norman Owen-Smith over the years have helped to develop understanding on the various concepts of functional heterogeneity and large herbivore species co-existence. The authors are grateful for helpful comments on the manuscript by Norman Owen-Smith, Emily Bennitt and Todd Anderson. The authors thank the three reviewers for their helpful reviews of the manuscript. We thank Theresa Fynn for proofreading the manuscript. RF acknowledges the One whose understanding is infinite (Psalm 147: 5).
The authors declare that the research was conducted in the absence of any commercial or financial relationships that could be construed as a potential conflict of interest.
All claims expressed in this article are solely those of the authors and do not necessarily represent those of their affiliated organizations, or those of the publisher, the editors and the reviewers. Any product that may be evaluated in this article, or claim that may be made by its manufacturer, is not guaranteed or endorsed by the publisher.
Acha P. N., Melendez L. V. (1983). The role of veterinary medicine in animal production and health: its impact on socio-economic development of Latin American and Caribbean countries. Rev. Sci. Tech. Oie. 2, 101–144. doi: 10.20506/rst.2.1.111
Acharya J., Hildreth M. B., Reese R. N. (2014). In vitro screening of forty medicinal plant extracts from the united states northern great plains for anthelmintic activity against haemonchus contortus. Vet. Parasitol. 201, 75–81. doi: 10.1016/j.vetpar.2014.01.008
Acres B. D., Blair Rains A., King R. B., Lawton R. M., Mitchell A. J. B., Rackham L. J. (1985). African Dambos: their distribution, characteristics and use. Zeitschrift fur geomorphologie, N.F. Supplementband 52, 63–86.
Amit M., Cohen I., Marcovics A., Muklada H., Glasser T. A., Ungar E. D., et al. (2013). Self-medication with tannin-rich browse in goats infected with gastro-intestinal nematodes. Vet. Parasitol. 198, 305–311. doi: 10.1016/j.vetpar.2013.09.019
Anderson T. M., Hopcraft J. G. C., Eby S., Ritchie M., Grace J. B., Olff H. (2010). Landscape-scale analyses suggest both nutrient and antipredator advantages to Serengeti herbivore hotspots. Ecology 91, 1519–1529. doi: 10.1890/09-0739.1
Anderson T. M., Ritchie M. E., Mayemba E., Eby S., Grace J. B., McNaughton S. J. (2007). Forage nutritive quality in the Serengeti ecosystem: the roles of fire and herbivory. Am. Nat. 170, 343–357. doi: 10.1086/520120
Arsenault R., Owen-Smith N. (2008). Resource partitioning by grass height among grazing ungulates does not follow body size relation. Oikos 117, 1711–1717. doi: 10.1111/j.1600-0706.2008.16575.x
Augustine D. J., Veblen K. E., Goheen J. R., Riginos C., Young T. P. (2011). “Pathways for productive cattle-wildlife interactions in semi-arid rangelands,” in Conserving wildlife in African landscapes: kenya’s ewaso ecosystem. Ed. Georgiadis N. J. (Washington DC, DC: Smithsonian Institution Scholarly Press), 55–71.
Barry T. N., McNeill D. M., McNabb W. C. (2001). “Plant secondary compounds: their impact on nutritive value and upon animal production,” in Proc. XIX Int. Grass. Conf, Sao Paulo, Brazil. 445–452, Proceedings published by Fundacao de Estudos Agrarios Luiz de Queiroz.
Bartlam-Brooks H. L. A., Bonyongo M. C., Harris S. (2011). Will reconnecting ecosystems allow long-distance mammal migrations to resume? a case study of a zebra Equus burchelli migration in Botswana. Oryx 45, 210–216. doi: 10.1017/S0030605310000414
Beck M. R., Gregorini P. (2020). How dietary diversity enhances hedonic and eudaimonic well-being in grazing ruminants. Front. Vet. Sci. 7. doi: 10.3389/fvets.2020.00191
Bell R. (1970). “The use of the herb layer by grazing ungulates in the Serengeti,” in Animal populations in relation to their food resources. Ed. Watson A. (Oxford, UK: Blackwell), 111–124.
Belovsky G. E. (1978). Diet optimization in a generalist herbivore: the moose. Theor. Popul. Biol. 14, 105–134. doi: 10.1016/0040-5809(78)90007-2
Bennitt E., Bonyongo M. C., Harris S. (2016). Effects of divergent migratory strategies on access to resources for cape buffalo (Syncerus caffer caffer). J. Mammal 97, 1682–1698. doi: 10.1093/jmammal/gyw134
Ben-Shahar R. (1991). Selectivity in large generalist herbivores: feeding patterns of African ungulates in a semi-arid habitat. Afr. J. Ecol. 29, 302–315. doi: 10.1111/j.1365-2028.1991.tb00468.x
Bergerud A. T., Elliot J. P. (1986). Dynamics of caribou and wolves in northern British Columbia. Can. J. Zoolog. 64, 1515–1519. doi: 10.1139/z86-226
Bhola N., Ogutu J., Piepho H. P., Said M., Reid R., Hobbs N. T., et al. (2012). Comparative changes in density and demography of large herbivores in the Masai Mara reserve and its surrounding human-dominated pastoral ranches in Kenya. Biodivers. Conserv. 21, 1509–1530. doi: 10.1007/s10531-012-0261-y
Bradley J. T. (2012). The effect of environmental variability on the foraging behaviour of plains zebra (Equus quagga) in the makgadikgadi, Botswana (Bristol (UK: University of Bristol).
Breman H., De Wit C. T. (1983). Rangeland productivity and exploitation in the sahel. Science 221, 1341–1347. doi: 10.1126/science.221.4618.1341
Bryant J. P., Chapin F. S. III, Klein D. R. (1983). Carbon/nutrient balance of boreal plants in relation to vertebrate herbivory. Oikos 40, 357–368. doi: 10.2307/3544308
Burkepile D. E., Fynn R. W. S., Thompson D. I., Lemoine N. P., Koerner S. E., Eby S., et al. (2017). Herbivore size matters for productivity–richness relationships in African savannas. J. Ecol. 105, 674–686. doi: 10.1111/1365-2745.12714
Burritt E. A., Provenza F. D. (2000). Role of toxins in intake of varied diets by sheep. J. Chem. Ecol. 26, 1991–2005. doi: 10.1023/A:1005565228064
Cai W., Borlace S., Lengaigne M., Van Rensch P., Collins M., Vecchi G., et al. (2014). Increasing frequency of extreme El niño events due to greenhouse warming. Nat. Clim. Change 4, 111–116. doi: 10.1038/nclimate2100
Codron D., Brink J. S., Rossouw L., Clauss M., Codron J., Lee-Thorp J. A. (2008). Functional differentiation of African grazing ruminants: an example of specialized adaptations to very small changes in diet. Biol. J. Linn. Soc. 94, 755–764. doi: 10.1111/j.1095-8312.2008.01028.x
Coley P. D., Bryant J. P., Chapin F. S. III. (1985). Resource availability and plant antiherbivore defense. Science 230, 895–899. doi: 10.1126/science.230.4728.895
Cook J. G., Johnson B. K., Cook R. C., Riggs R. A., Delcurto T., Bryant L. D., et al. (2004). Effects of summer-autumn nutrition and parturition date on reproduction and survival of elk. Wildlife. Monogr. 155, 1–61. doi: 10.2193/0084-0173(2004)155[1:EOSNAP]2.0.CO;2
Cooper S. M., Owen-Smith N., Bryant J. P. (1988). Forage acceptability to browsing ruminants in relation to seasonal changes in leaf chemistry of woody plants in a south African savanna. Oecologia 75, 336–342. doi: 10.1007/BF00376934
Durmic Z., Blache D. (2012). Bioactive plants and plant products: effects on animal function, health and welfare. Anim. Feed. Sci. Tech. 176, 150–162. doi: 10.1016/j.anifeedsci.2012.07.018
Dye P., Walker B. (1980). Vegetation-environment relations on sodic soils of Zimbabwe-Rhodesia. J. Ecol. 68, 589–606. doi: 10.2307/2259424
Eby S. L., Anderson T. M., Mayemba E. P., Ritchie M. E. (2014). The effect of fire on habitat selection of mammalian herbivores: the role of body size and vegetation characteristics. J. Anim. Ecol. 83, 1196–1205. doi: 10.1111/1365-2656.12221
Ellis J. E., Swift D. M. (1988). Stability of African pastoral ecosystems: alternate paradigms and implications for development. J. Range. Manage. 41, 450–459. doi: 10.2307/3899515
Engel C. (2002). Wild health: how animals keep themselves well and what we can learn from them (New York: Houghton Mifflin).
Engelbrecht F., Adegoke J., Bopape M. J., Naidoo M., Garland R., Thatcher M., et al. (2015). Projections of rapidly rising surface temperatures over Africa under low mitigation. Environ. Res. Lett. 10, 85004. doi: 10.1088/1748-9326/10/8/085004
Everett P., Perrin M., Rowe Rowe D. (1991). Responses by oribi to different range management practices in natal. S. Afr. J. Wildl. Res. 21, 114–118.
Freeland W. J., Janzen D. H. (1974). Strategies in herbivory by mammals: the role of plant secondary compounds. Am. Nat. 108, 269–287. doi: 10.1086/282907
French K. E., Harvey J., McCullagh J. S. (2018). Targeted and untargeted metabolic profiling of wild grassland plants identifies antibiotic and anthelmintic compounds targeting pathogen physiology, metabolism and reproduction. Sci. Rep-Uk. 8, 1695. doi: 10.1038/s41598-018-20091-z
Fryxell J. M., Sinclair A. R. E. (1988). Causes and consequences of migration by large herbivores. Trends. Ecol. Evol. 3, 237–241. doi: 10.1016/0169-5347(88)90166-8
Fryxell J. M., Wilmshurst J. F., Sinclair A. R. E., Haydon D. T., Holt R. D., Abrams P. A. (2005). Landscape scale, heterogeneity, and the viability of Serengeti grazers. Ecol. Lett. 8, 328–335. doi: 10.1111/j.1461-0248.2005.00727.x
Fynn R. W. S., Augustine D. J., Peel M. J. S., de Garine-Wichatitsky M. (2016). Strategic management of livestock to improve biodiversity conservation in African savannahs: a conceptual basis for wildlife-livestock co-existence. J. Appl. Ecol. 53, 388–397. doi: 10.1111/1365-2664.12591
Fynn R. W. S., Bonyongo M. C. (2011). Functional conservation areas and the future of africa’s wildlife. Afr. J. Ecol. 49, 175–188. doi: 10.1111/j.1365-2028.2010.01245.x
Fynn R. W. S., Chase M., Roder A. (2014). Functional habitat heterogeneity and large herbivore seasonal habitat selection in northern Botswana. S. Afr. J. Wildl. Res. 44, 1–15. doi: 10.3957/056.044.0103
Fynn R. W. S., Murray-Hudson M., Dhliwayo M., Scholte P. (2015). African Wetlands and their seasonal use by wild and domestic herbivores. Wetl. Ecol. Manage. 23, 559–581. doi: 10.1007/s11273-015-9430-6
Gaillard J. M., Festa-Bianchet M., Yoccoz N. G., Loison A., Toigo C. (2000). Temporal variation in fitness components and population dynamics of large herbivores. Annu. Rev. Ecol. Syst. 31, 367–393. doi: 10.1146/annurev.ecolsys.31.1.367
Garrett K., Beck M. R., Marshall C. J., Fleming A. E., Logan C. M., Maxwell T. M. R., et al. (2021). Functional diversity vs. monotony: the effect of a multiforage diet as opposed to a single forage diet on animal intake, performance, welfare, and urinary nitrogen excretion. J. Anim. Sci. 99, 1–9. doi: 10.1093/jas/skab058
Garrett K., Marshall C. J., Beck M. R., Maxwell T. M. R., Logan C. M., Gregorini P. (2022). A diverse diet as an alternative to ryegrass can improve the total antioxidant status of dams at lambing. Front. Sustain Food Syst. 6. doi: 10.3389/fsufs.2022.885436
Gessner D., Ringseis K. R., Eder K. (2017). Potential of plant polyphenols to combat oxidative stress and inflammatory processes in farm animals. J. Anim. Physiol. An. N. 101, 605–628. doi: 10.1111/jpn.12579
Glasser T. A., Ungar E. D., Landau S. Y., Perevolotsky A., Muklada H., Walker J. W. (2009). Breed and maternal effects on the intake of tannin-rich browse by juvenile domestic goats (Capra hircus). Appl. Anim. Behav. Sci. 119, 71–77. doi: 10.1016/j.applanim.2009.02.028
Gordon I. J. (1988). Facilitation of red deer grazing by cattle and its impact on red deer performance. J. Appl. Ecol. 25, 1–10. doi: 10.2307/2403605
Grant C. C., Scholes M. C. (2006). The importance of nutrient hotspots in the conservation and management of large wild mammalian herbivores in semi-arid savannas. Biol. Conserv. 130, 426–437. doi: 10.1016/j.biocon.2006.01.004
Grobler J. H. (1981). Feeding behaviour of sable Hippotragus niger niger (Harris 1838) in the rhodes matopos national park, Zimbabwe. S. Afr. J. Zool. 16, 15–58.
Harrington R., Owen-Smith N., Viljoen P. C., Biggs H. C., Mason D. R., Funston P. (1999). Establishing the causes of the roan antelope decline in the Kruger national park, south Africa. Biol. Conserv. 90, 69–78. doi: 10.1016/S0006-3207(98)00120-7
Havemann C. P., Retief T. A., Collins K., Fynn R. W. S., Tosh C. A., de Bruyn P. J. N. (2022). Home range and habitat use of roan antelope Hippotragus equinus in northern Botswana. J. Arid Environ. 196, 104648. doi: 10.1016/j.jaridenv.2021.104648
Hempson G. P., Archibald S., Bond W. J., Ellis R. P., Grant C. C., Kruger F. J., et al. (2015). Ecology of grazing lawns in Africa. Biol. Rev. 90, 979–994. doi: 10.1111/brv.12145
Hensman M. C., Owen-Smith N., Parrini F., Bonyongo M. C. (2013). Resource use and the nutritional status of sable antelope in the okavango delta region of northern Botswana. Afr. J. Ecol. 52, 237–245. doi: 10.1111/aje.12113
Hensman M. C., Owen-Smith N., Parrini F., Erasmus B. F. N. (2012). Dry season browsing by sable antelope in northern Botswana. Afr. J. Ecol. 50, 513–516. doi: 10.1111/j.1365-2028.2012.01349.x
Hobbs N. T., Galvin K. A., Stokes C. J., Lackett J. M., Ash A. J., Boone R. B., et al. (2008). Fragmentation of rangelands: implications for humans, animals and landscapes. Glob. Environ. Change 18, 776–785. doi: 10.1016/j.gloenvcha.2008.07.011
Hopcraft J. G. C., Anderson T. M., Perez-Vila S., Mayemba E., Olff H. (2012). Body size and the division of niche space: food and predation differentially shape the distribution of Serengeti grazers. J. Anim. Ecol. 81, 201–213. doi: 10.1111/j.1365-2656.2011.01885.x
Hopcraft J. G. C., Olff H., Sinclair A. R. E. (2010). Herbivores, resources and risks: alternating regulation along primary environmental gradients in savannas. Trends. Ecol. Evol. 25, 119–128. doi: 10.1016/j.tree.2009.08.001
Illius A. W., O’Connor T. G. (2000). Resource heterogeneity and ungulate population dynamics. Oikos 89, 283–294. doi: 10.1034/j.1600-0706.2000.890209.x
Jarrell W. M., Beverley R. B. (1981). The dilution effect in plant nutrition studies. Adv. Agron. 34, 197–224. doi: 10.1016/S0065-2113(08)60887-1
Joos-VandeWalle M. E. J. (2000). Movements of migratory zebra and wildebeest in northern Botswana, [PhD dissertation]: [Johannesburg (RSA)]: University of the Witwatersrand).
Juhnke J., Miller J., Hall J. O., Provenza F. D., Villalba J. J. (2012). Preference for condensed tannins by sheep in response to challenge infection with haemonchus contortus. Vet. Parasitol. 188, 104–114. doi: 10.1016/j.vetpar.2012.02.015
Jungius J. (1971). Studies on the food and feeding behaviour of the reedbuck Redunca arundinum boddaert 1785 In the Kruger national park. Koedoe 14, 65–97. doi: 10.4102/koedoe.v14i1.718
Kamweneshe B. M. (2000). Ecology, conservation and management of the black lechwe (Kobus leche smithemani) in the bangweulu basin, Zambia (Pretoria (RSA): University of Pretoria).
Kartzinel T. R., Chen P. A., Coverdale T. C., Erickson D. L., Kress W. J., Kuzmina M. L., et al. (2015). DNA Metabarcoding illuminates dietary niche partitioning by African large herbivores. P. Natl. Acad. Sci. 112, 8019–8024. doi: 10.1073/pnas.1503283112
Kaspari M. (2020). The seventh macronutrient: how sodium shortfall ramifies through populations, food webs and ecosystems. Ecol. Lett. 23, 1153–1168. doi: 10.1111/ele.13517
Kassa B., Libois R., Sinsin B. (2007). Diet and food preference of the waterbuck (Kobus ellipsiprymnus defassa) in the pendjari national park, Benin. Afr. J. Ecol. 46, 303–310. doi: 10.1111/j.1365-2028.2007.00827.x
Keemekae T. (2022). Ecological moisture and productivity gradients as determinants of forage quantity and quality, and cattle body condition at eretsha and sehithwa, Botswana (Maun (BW): University of Botswana).
Kleynhans E. J., Jolles A. E., Bos M. R. E., Olff H. (2011). Resource partitioning along multiple niche dimensions in differently sized African savanna grazers. Oikos 120, 591–600. doi: 10.1111/j.1600-0706.2010.18712.x
Knoop M. C., Owen-Smith N. (2006). Foraging ecology of roan antelope: key resources during critical periods. Afr. J. Ecol. 44, 228–236. doi: 10.1111/j.1365-2028.2006.00637.x
Landau S., Azaizeh H., Muklada H., Glasser T., Ungar E. D., Baram H., et al. (2010). Anthelmintic activity of pistacia lentiscus foliage in two middle Eastern breeds of goats differing in their propensity to consume tannin-rich browse. Vet. Parasitol. 173, 280–286. doi: 10.1016/j.vetpar.2010.07.006
Lange K., Olcott D. D., Miller J. E., Mosjidis J. A., Terrill T. H., Burke J. M., et al. (2006). Effect of Sericea lespedeza (Lespedeza cuneata) fed as hay, on natural and experimental haemonchus contortus infections in lambs. Vet. Parasitol. 141, 273–278. doi: 10.1016/j.vetpar.2006.06.001
Lisonbee L. D., Villalba J. J., Provenza F. D., Hall J. O. (2009). Tannins and self-medication: implications for sustainable parasite control in herbivores. Behav. Process. 82, 184–189. doi: 10.1016/j.beproc.2009.06.009
Løvschal M., Bocher P. K., Pilgaard J., Amoke I., Odingo A., Thuo A., et al. (2017). Fencing bodes a rapid collapse of the unique greater Mara ecosystem. Sci. Rep-Uk. 7, 41450. doi: 10.1038/srep41450
Lyman T. D., Provenza F. D., Villalba J. J. (2013). Influence of diet sequence on intake of foods containing ergotamine d tartrate, tannins and saponins by sheep. Appl. Anim. Behav. Sci. 144, 57–62. doi: 10.1016/j.applanim.2012.12.006
Lyman T. D., Provenza F. D., Villalba J. J., Wiedmeier R. D. (2011). Cattle preferences differ when endophyte-infected tall fescue, birdsfoot trefoil, and alfalfa are grazed in difference sequences. J. Anim. Sci. 89, 1131–1137. doi: 10.2527/jas.2009-2741
Macandza V. A., Owen-Smith N., Cross P. C. (2004). Forage selection by African buffalo in the late dry season in two landscapes. S. Afr. J. Wildl. Res. 34, 113–121. Available at: https://hdl.handle.net/10520/EJC117195
MacArthur R. H., Pianka E. R. (1966). On optimal use of a patchy environment. Am. Nat. 100 (916), 603–609. doi: 10.1086/282454
Maddock L. (1979). “The migration and grazing succession,” in Serengeti: Dynamics of an ecosystem. Eds. Sinclair A. R. E., Norton-Griffiths M. (Chicago, IL: The University of Chicago Press), 104–129.
McCarthy T. S. (2006). Groundwater in the wetlands of the okavango delta, Botswana, and its contribution to the structure and function of the ecosystem. J. Hydrology 320, 264–282. doi: 10.1016/j.jhydrol.2005.07.045
McNaughton S. J. (1983). Serengeti Grassland ecology: the role of composite environmental factors and contingency in community organization. Ecol. Monogr. 53, 291–320. doi: 10.2307/1942533
McNaughton S. J. (1985). Ecology of a grazing ecosystem: the Serengeti. Ecol. Monogr. 55, 259–294. doi: 10.2307/1942578
McNaughton S. J. (1988). Mineral nutrition and spatial concentrations of African ungulates. Nature 334, 343–345. doi: 10.1038/334343a0
McNaughton S. J. (1990). Mineral nutrition and seasonal movements of African migratory ungulates. Nature 345, 613–615. doi: 10.1038/345613a0
McNaughton S. J., Banyikwa F. F., McNaughton M. M. (1997). Promotion of diet enhancing nutrients by African grazers. Science 278, 1798–1800. doi: 10.1126/science.278.5344.1798
Mengistu G., Hoste H., Karonen M., Salminen J. P., Hendriks W. H., Pellikaan W. F. (2017). The in vitro anthelmintic properties of browse plant species against haemonchus contortus is determined by the polyphenol content and composition. Vet. Parasitol. 237, 110–116. doi: 10.1016/j.vetpar.2016.12.020
Meuret M., Provenza F. D. (2015). When art and science meet: integrating knowledge of French herders with science of foraging behavior. Rangeland Ecol. Manage. 68, 1–17. doi: 10.1016/j.rama.2014.12.007
Michael J. D., Baruselli P. S., Campanile G. (2019). Influence of nutrition, body condition, and metabolic status on reproduction in female beef cattle: a review. Theriogenology 125, 277–284. doi: 10.1016/j.theriogenology.2018.11.010
Min B. R., Barry T. N., Attwood G. T., McNabb W. C. (2003). The effect of condensed tannins on the nutrition and health of ruminants fed fresh temperate forages: a review. Anim. Feed Sci. Technol. 106, 3–19. doi: 10.1016/S0377-8401(03)00041-5
Min B. R., Fernandez J. M., Barry T. N., McNabb W. C., Kemp P. D. (2001). The effect of condensed tannins in Lotus corniculatus upon reproductive efficiency and wool production in ewes during autumn. Anim. Feed Sci. Tech. 92, 185–202. doi: 10.1016/S0377-8401(01)00258-9
Min B. R., Hart S. P. (2003). Tannins for suppression of internal parasites. J. Anim. Sci. 81, E102–E109. doi: 10.2527/2003.8114_suppl_2E102x
Min R., Pinchak W. E., Anderson J.D. Fulford R. C., Puchala R. (2006). Effects of condensed tannins supplementation level on weight gain and in vitro and in vivo bloat precursors in steers grazing winter wheat. J. Anim. Sci. 84, 2546–2554. doi: 10.2527/jas.2005-590
Min B. R., Pomroy W. E., Hart S. P., Sahlu T. (2004). The effect of short-term consumption of a forage containing condensed tannins on gastro-intestinal nematode parasite infections in grazing wether goats. Small Rum. Res. 51, 279–283. doi: 10.1016/S0921-4488(03)00204-9
Moreno F. C., Gordon I. J., Knox M. R., Summer P. M., Skerrat L. F., Benvenutti M. A., et al. (2012). Anthelmintic efficacy of five tropical native Australian plants against haemonchus contortus and trichostrongylus colubriformis in experimentally infected goats (Capra hircus). Vet. Parasitol. 187, 237–243. doi: 10.1016/j.vetpar.2011.12.040
Morrison T. A., Bolger D. T. (2014). Connectivity and bottlenecks in a migratory wildebeest Connochaetes taurinus population. Oryx 48, 613–621. doi: 10.1017/S0030605313000537
Mote T., Villalba J. J., Provenza F. D. (2008). Foraging sequence influences the ability of lambs to consume foods containing tannins and terpenes. Appl. Anim. Behav. Sci. 113, 57–68. doi: 10.1016/j.applanim.2007.10.003
Muchiru A. N., Western D., Reid R. S. (2009). The impact of abandoned pastoral settlements on plant and nutrient succession in an African savanna ecosystem. J. Arid Environ. 73, 322–331. doi: 10.1016/j.jaridenv.2008.09.018
Müller D. W., Codron D., Meloro C., Munn A., Schwarm A., Hummel J., et al. (2013). Assessing the jarman–bell principle: scaling of intake, digestibility, retention time and gut fill with body mass in mammalian herbivores. Comp. Biochem. Phys. A 164, 129–140. doi: 10.1016/j.cbpa.2012.09.018
Murray M. G. (1995). “Specific nutrient requirements and migration of wildebeest,” in Serengeti II: dynamics, management, and conservation of an ecosystem. Eds. Sinclair A. R. E., Arcese P. (Chicago, IL: The University of Chicago Press), 231–256.
Murray M. G., Illius A. W. (2000). Vegetation modification and resource competition in grazing ungulates. Oikos 89, 501–508. doi: 10.1034/j.1600-0706.2000.890309.x
Mysterud A., Langvatn R., Yoccoz N. G., Stenseth N. C. (2001). Plant phenology, migration and geographical variation in body weight of a large herbivore: the effect of a variable topography. J. Anim. Ecol. 70, 915–923. doi: 10.1046/j.0021-8790.2001.00559.x
Naidoo R., Chase M. J., Beytell P., Du Preez P., Landen K., Stuart-Hill G., et al. (2014). A newly discovered wildlife migration in Namibia and Botswana is the longest in Africa. Oryx 50, 138–146. doi: 10.1017/S0030605314000222
Nakajima N., Doi K., Tamiya S., Yayota M. (2021). Potential impact of botanically diverse pasture on the nutritional, physiological, and immunological status of grazing cows. Grassland Sci. 68, 155–164. doi: 10.1111/grs.12351
Niezen J. H., Charleston W. A. G., Robertson H. A., Shelton D., Waghorn G. C., Green R. (2002). The effect of feeding sulla (Hedysarum coronarium) or lucerne (Medicago sativa) on lamb parasite burdens and development of immunity to gastrointestinal nematodes. Vet. Parasitol. 105, 229–245. doi: 10.1016/S0304-4017(02)00014-6
Noy-Meir I. (1973). Desert ecosystems: environment and producers. Annu. Rev. Ecol. Syst. 4, 24–52. doi: 10.1146/annurev.es.04.110173.000325
Ogutu J. O., Owen-Smith N. (2003). ENSO, rainfall and temperature influences on extreme population declines among African savanna ungulates. Ecol. Lett. 6, 412–419. doi: 10.1046/j.1461-0248.2003.00447.x
Ogutu J. O., Owen-Smith N., Piepho H. P., Said M. Y. (2011). Continuing wildlife population declines and range contraction in the Mara region of Kenya during 1977–2009. J. Zool. 285, 99–109. doi: 10.1111/j.1469-7998.2011.00818.x
Owen-Smith R. N. (1988). Megaherbivores: the influence of very large body size on ecology (Cambridge: Cambridge University Press).
Owen-Smith N. (1990). Demography of a large herbivore, the greater kudu, Tragelaphus strepsiceros, in relation to rainfall. J. Anim. Ecol. 59, 893–913. doi: 10.2307/5021
Owen-Smith N. (1994). Foraging responses of kudu’s to seasonal changes in food resources: elasticity in constraints. Ecology 75, 1050–1062. doi: 10.2307/1939429
Owen-Smith N. (2004). Functional heterogeneity in resources within landscapes and herbivore population dynamics. Landscape. Ecol. 19, 761–771. doi: 10.1007/s10980-005-0247-2
Owen-smith N. (2008). “The comparative population dynamics of browsing and grazing ungulates,” in The ecology of browsing and grazing. Eds. Gordon I. J., Prins H. H. T. (New York, NY: Springer), 149–177.
Owen-Smith N., Cooper S. M. (1987). Palatability of woody plants to browsing ruminants in a south African savanna. Ecology 68, 319–331. doi: 10.2307/1939263
Owen-Smith N., Cooper S. M. (1989). Nutritional ecology of a browsing ruminant, the kudu (Tragelaphus strepsiceros), through the seasonal cycle. J. Zool. 219, 29–43. doi: 10.1111/j.1469-7998.1989.tb02563.x
Owen-Smith N., Cooper S. M., Novellie P. (1983). Aspects of the feeding ecology of a browsing ruminant: the kudu. S. Afr. J. Anim. Sci. 13, 35–38.
Owen-Smith N., Novellie P. (1982). What should a clever ungulate eat? Am. Nat. 119, 151–178. doi: 10.1086/283902
Pamo E. T. (1998). Herders and wildgame behavior as a strategy against desertification in northern Cameroon. J. Arid. Environ. 39, 179–190. doi: 10.1006/jare.1998.0400
Pansu J., Guyton J. A., Potter A. B., Atkins J. L., Daskin J. H., Wursten B., et al. (2019). Trophic ecology of large herbivores in a reassembling African ecosystem. J. Ecol. 107, 1355–1376. doi: 10.1111/1365-2745.13113
Parker K. L., Barboza P. S., Gillingham M. P. (2009). Nutrition integrates environmental responses of ungulates. Funct. Ecol. 23, 57–69. doi: 10.1111/j.1365-2435.2009.01528.x
Parrini F., Owen-Smith N. (2010). The importance of post-fire regrowth for sable antelope in a southern African savanna. Afr. J. Ecol. 48, 526–534. doi: 10.1111/j.1365-2028.2009.01143.x
Pereira F. C., Gregorini P. (2022). Applying spatio-chemical analysis to grassland ecosystems for the illustration of chemoscapes and creation of healthscapes. Front. Sustain. Food Syst. 6. doi: 10.3389/fsufs.2022.927568
Perrin M. R., Brereton-Stiles R. (1999). Habitat use and feeding behavior of the buffalo and the white rhinocerous in the hluhluwe-umfolozi game reserve. S. Afr. J. Wildl. Res. 29, 72–80. Available at: https://hdl.handle.net/10520/EJC117074.
Person B. T., Herzog M. P., Ruess R. W., Sedinger J. S., Anthony R. M., Babcock C. A. (2003). Feedback dynamics of grazing lawns: coupling vegetation change with animal growth. Oecologia 135, 583–592. doi: 10.1007/s00442-003-1197-4
Pettorelli N., Dray S., Gaillard J., Chessel D., Duncan P., Illius A., et al. (2003). Spatial variation in springtime food resources influences the winter body mass of roe deer fawns. Oecologia 137, 363–369. doi: 10.1007/s00442-003-1364-7
Prins H. (1996). Ecology and behaviour of the African buffalo: social inequality and decision making (Vol. 1) (New York: Springer).
Prins H. H. T., van Langevelde F. (2008). “Assembling a diet from different places,” in Resource ecology: spatial and temporal dynamics of foraging. Eds. Prins H. H. T., Van Langevelde F. (Wageningen, NL: Springer), 129–155. doi: 10.1007/978-1-4020-6850-8_12
Provenza F. D. (2018). Nourishment: what animals can teach us about rediscovering our nutritional wisdom (White River Junction: Chelsea Green Publishing). doi: 10.2989/10220119.2021.1947374
Provenza F. D., Kronberg S. L., Gregorini P. (2019). Is grassfed meat and dairy better for human and environmental health? Front. Nutr. 6. doi: 10.3389/fnut.2019.00026
Provenza F. D., Meuret M., Gregorini P. (2015). Our landscapes, our livestock, ourselves: restoring broken linkages among plants, herbivores, and humans with diets that nourish and satiate. Appetite 95, 500–519. doi: 10.1016/j.appet.2015.08.004
Provenza F. D., Villalba J. J. (2010). The role of natural plant products in modulating the immune system: an adaptable approach for combating disease in grazing animals. Small Ruminant Res. 89, 131–139. doi: 10.1016/j.smallrumres.2009.12.035
Provenza F. D., Villalba J. J., Dziba L. E., Atwood S. B., Banner R. E. (2003). Linking herbivore experience, varied diets, and plant biochemical diversity. Small Ruminant Res. 49, 257–274. doi: 10.1016/S0921-4488(03)00143-3
Provenza F. D., Villalba J. J., Haskell J. H., Macadam J. A., Griggs T. C., Wiedmeier R. D. (2007). The value to herbivores of plant physical and chemical diversity in time and space. Crop Sci. 47, 382–398. doi: 10.2135/cropsci2006.02.0083
Provenza F., Villalba J., Wiedmeier R., Lyman T., Owens J., Lisonbee L., et al. (2009). Value of plant diversity for diet mixing and sequencing in herbivores. Rangelands 31, 45–49. doi: 10.2111/1551-501X-31.1.45
Redoy M. R. A., Shuvo1 A. A. S., Cheng L., Al-Mamun M. (2020). Effect of herbal supplementation on growth, immunity, rumen histology, serum antioxidants and meat quality of sheep. Animal 14 (11), 2433–2441. doi: 10.1017/S1751731120001196
Rettie W. J., Messier M. (2000). Hierarchical habitat selection by woodland caribou: its relationship to limiting factors. Ecography 23, 466–478. doi: 10.1111/j.1600-0587.2000.tb00303.x
Rhoades D. F. (1979). “Evolution of plant chemical defense against herbivores,” in Herbivores: their interaction with secondary plant metabolites. Eds. Rosenthal G. A., Janzen D. H. (New York: Academic Press), 3–54.
Romanens R., Pellacani F., Mainga A., Fynn R., Vittoz P., Verrecchia E. P. (2019). Soil diversity and major soil processes in the Kalahari basin, Botswana. Geoderma Reg.l 19, e00236. doi: 10.1016/j.geodrs.2019.e00236
Scholte P., Brouwer J. (2008). “Relevance of key resource areas for large-scale movements of livestock,” in Resource ecology: spatial and temporal dynamics of foraging. Eds. Prins H. H. T., van Langevelde F. (Wageningen, NL: Springer), 211–232. doi: 10.1007/978-1-4020-6850-8_18
Schuette J. R., Leslie D. M., Lochmiller R. L., Jenks J. A. (1998). Diets of hartebeest and roan antelope in Burkina Faso: support of the long-faced hypothesis. J. Mammal. 79, 426–436. doi: 10.2307/1382973
Scoones I. (1995). Exploiting heterogeneity: habitat use by cattle in dryland Zimbabwe. J. Arid. Environ. 29, 221–237. doi: 10.1016/S0140-1963(05)80092-8
Seefeldt S. S. (2005). Consequences of selecting ramboulliet ewes for mountain big sagebrush (Artemisia tridentata ssp. vaseyana) dietary preference. Rangeland Ecol. Manage. 58, 380–384. doi: 10.2111/1551-5028(2005)058[0380:COSREF]2.0.CO;2
Selebatso M., Maude G., Fynn R. W. S. (2018). Adaptive foraging of sympatric ungulates in the central Kalahari game reserve, Botswana. Afr. J. Wildl. Res. 48, 023005. doi: 10.3957/056.048.023005
Senft R. L., Coughenour M. B., Bailey D. W., Rittenhouse L. R., Sala O. E., Swift D. M. (1987). Large Herbivore foraging and ecological hierarchies. Bioscience 37, 789–799. doi: 10.2307/1310545
Sensenig R. L., Demment M. W., Laca E. A. (2010). Allometric scaling predicts preference for burned patches in a guild of East African grazers. Ecology 91, 2898–2907. doi: 10.1890/09-1673.1
Shaik S. A., Terrill T. H., Miller J. E., Kouakou B., Kannan G., Kaplan R. M., et al. (2006). Sericea lespedeza hay as a natural deworming agent against gastrointestinal nematode infection in goats. Vet. Parasitol. 139, 150–157. doi: 10.1016/j.vetpar.2006.02.020
Sheppe W., Osborne T. (1971). Patterns of use of a flood plain by Zambian mammals. Ecol. Monogr. 41, 179–205. doi: 10.2307/1942365
Sianga K., Fynn R. (2017). The vegetation and wildlife habitats of the savuti-Mababe-Linyanti ecosystem, northern Botswana. Koedoe 59, a1406. doi: 10.4102/koedoe.v59i2.1406
Sianga K., Fynn R. W. S., Bonyongo M. C. (2017a). Seasonal habitat selection by African buffalo Syncerus caffer in the savuti–Mababe–Linyanti ecosystem of northern Botswana. Koedoe 59, a1382. doi: 10.4102/koedoe.v59i2.1382
Sianga K., van Telgen M., Vrooman J., Fynn R. W., van Langevelde F. (2017b). Spatial refuges buffer landscapes against homogenisation and degradation by large herbivore populations and facilitate vegetation heterogeneity. Koedoe 59, a1434. doi: 10.4102/koedoe.v59i2.1434
Sinclair A. R. E. (1975). The resource limitation of trophic levels in tropical grassland ecosystems. J. Anim. Ecol. 44, 497–520. doi: 10.2307/3608
Sinclair A. R. E. (1979). “The Serengeti environment,” in Serengeti: Dynamics of an ecosystem. Eds. Sinclair A. R. E., Norten-Griffiths M. (Chicago, IL: The University of Chicago Press), 31–45.
Sinclair A. R. E., Mduma S., Brashares J. (2003). Patterns of predation in a diverse predator-prey system. Nature 425, 288–290. doi: 10.1038/nature01934
Smith A. D. (1959). Adequacy of some important browse species in overwintering mule deer. J. Range. Manage 12, 9–13. doi: 10.2307/3895208
Steuer P., Südekum K. H., Tütken T., Müller D. W., Kaandorp J., Bucher M., et al. (2014). Does body mass convey a digestive advantage for large herbivores? Funct. Ecol. 28, 1127–1134. doi: 10.1111/1365-2435.12275
Stokes C. J., McAllister R. R. J., Ash A. J. (2006). Fragmentation of Australian rangelands: processes, benefits and risks of changing patterns of land use. Rangel. J. 28, 83–96. doi: 10.1071/RJ05026
Tanner G. J., Moate P. J., Davis L. H., Laby R. H., Li Y. G., Larkin P. J., et al. (1995). Proanthocyanidins (condensed tannin) destabilize plant protein foams in a dose dependent manner. Aust. J. Agric. Res. 46, 1101–1109. doi: 10.1071/AR9951101
Taylor R. D. (1985). The response of buffalo, syncerus caffer (Sparrman) to the kariba lakeshore grassland (Panicum repens l.) in matusadona national park (Harare (Zw: University of Zimbabwe).
van Vliet S., Provenza F. D., Kronberg S. L. (2021). Health-promoting phytonutrients are higher in grass-fed meat and milk. Front. Sustain Food Syst. 4. doi: 10.3389/fsufs.2020.555426
Van der Vijver C. A. D. M., Poot P., Prins H. H. T. (1999). Causes of increased nutrient concentrations in post-fire regrowth in an East African savanna. Plant Soil 214, 173–185. doi: 10.1023/A:1004753406424
van Vliet S., Kronberg S. L., Provenza F. D. (2020). Plant-based meats, human health, and climate change. Front. Sustain Food Syst. 4. doi: 10.3389/fsufs.2020.00128
Veldhuis M. P., Ritchie M. E., Ogutu J. O., Morrison T. A., Beale C. M., Estes A. B., et al. (2019). Cross-boundary human impacts compromise the Serengeti-Mara ecosystem. Science 363, 1424–1428. doi: 10.1126/science.aav0564
Velladurai C., Selvaraju M., Napolean R. E. (2016). Effects of macro and micro minerals on reproduction in dairy cattle a review. Int. J. Sci. Res. Sci. Tech. 1, 68–74.
Venter J. A., Watson L. H. (2008). Feeding and habitat use of buffalo (Syncerus caffer caffer) in the nama-karoo, south Africa. S. Afr. J. Wildl. Res. 38, 42–51. doi: 10.3957/0379-4369-38.1.42
Verweij R. J. T., Verrelst J., Loth P. E., Heitkonig I. M. A., Brunsting A. M. H. (2006). Grazing lawns contribute to the subsistence of mesoherbivores on dystrophic savannas. Oikos 114, 108–116. doi: 10.1111/j.2006.0030-1299.14209.x
Vesey-FitzGerald D. F. (1960). Grazing succession among East African game animals. J. Mammal. 41, 161–172. doi: 10.2307/1376351
Villalba J. J., Beauchemin K. A., Gregorini P., MacAdam J. W. (2019). Pasture chemoscapes and their ecological services. Transl. Anim. Sci. 3, 829–841. doi: 10.1093/tas/txz003
Villalba J. J., Costes-Thire M., Ginane C. (2017). Phytochemicals in animal health: diet selection and trade-offs between costs and benefits. P. Nutr. Soc 76, 113–121. doi: 10.1017/S0029665116000719
Villalba J. J., Provenza F. D. (2007). Self-medication and homeostatic endeavor in herbivores: learning about the benefits of nature’s pharmacy. Animal 1, 1360–1370. doi: 10.1017/S1751731107000134
Villalba J. J., Provenza F. D., GouDong H. (2004). Experience influences diet mixing by herbivores: implications for plant biochemical diversity. Oikos 107, 100–109. doi: 10.1111/j.0030-1299.2004.12983.x
Villalba J. J., Provenza F. D., Hall J. O., Lisonbee L. D. (2010). Selection of tannins by sheep in response to gastrointestinal nematode infection. J. Anim. Sci. 88, 2189–2198. doi: 10.2527/jas.2009-2272
Villalba J. J., Provenza F. D., Shaw R. (2006). Sheep self-medicate when challenged with illness-inducing foods. Anim. Behav. 71, 1131–1139. doi: 10.1016/j.anbehav.2005.09.012
Voeten M. M., Van De Vijver C. A., Olff H., Van Langevelde F. (2010). Possible causes of decreasing migratory ungulate populations in an East African savannah after restrictions in their seasonal movements. Afr. J. Ecol. 48, 169–179. doi: 10.1111/j.1365-2028.2009.01098.x
Waghorn G. C. (1990). “Beneficial effects of low concentrations of condensed tannins in forages fed to ruminants,” in Microbial and plant opportunities to improve lignocellulose utilization by ruminants. Eds. Akin D. E., Ljungdahl L. G., Wilson J. R., Harris P. J. (New York, NY: Elsevier Sci. Publ.), 137.
Walker B. H., Emslie R. H., Owen-Smith R. N., Scholes R. J. (1987). To cull or not to cull: lessons from a southern African drought. J. Appl. Ecol. 24, 381–401. doi: 10.2307/2403882
Weeber J., Hempson G. P., February E. C. (2020). Large Herbivore conservation in a changing world: surface water provision and adaptability allow wildebeest to persist after collapse of long-range movements. Glob. Change Biol. 26, 2841–2853. doi: 10.1111/gcb.15044
Western D. J. (1973). The structure, dynamics and changes of the amboseli ecosystem (Nairobi (KN: University of Nairobi).
White R. G. (1983). Foraging patterns and their multiplier effects on productivity of northern ungulates. Oikos 40, 377–384. doi: 10.2307/3544310
Wilmshurst J. F., Fryxell J. M., Bergman C. M. (2000). The allometry of patch selection in ruminants. Proc. Biol. Sci. 267, 345–349. doi: 10.1098/rspb.2000.1007
Wittmer H. U., SinclairR A. R. E., Mclellan B. N. (2005). The role of predation in the decline and extirpation of woodland caribou. Oecologia 144, 257–267. doi: 10.1007/s00442-005-0055-y
Woodward S. L., Waghorn G. C., Laboyrie P. G. (2004). Condensed tannins in birdsfoot trefoil (Lotus corniculatus) reduce methane emissions from dairy cows. Proc. New Zeal. Soc An. 64, 160–164.
Keywords: foraging strategies, forage quantity and quality, phytochemicals, seasonal variation, climate change, growth and reproduction, migration, pastoralism
Citation: Fynn RWS and Provenza FD (2023) Functional adaptive resources for large herbivores in African savannas: an ecological-gradient based framework. Front. Conserv. Sci. 4:1133329. doi: 10.3389/fcosc.2023.1133329
Received: 28 December 2022; Accepted: 04 April 2023;
Published: 24 May 2023.
Edited by:
Oded Berger-Tal, Ben-Gurion University of the Negev, IsraelReviewed by:
Burt P. Kotler, Ben-Gurion University of the Negev, IsraelCopyright © 2023 Fynn and Provenza. This is an open-access article distributed under the terms of the Creative Commons Attribution License (CC BY). The use, distribution or reproduction in other forums is permitted, provided the original author(s) and the copyright owner(s) are credited and that the original publication in this journal is cited, in accordance with accepted academic practice. No use, distribution or reproduction is permitted which does not comply with these terms.
*Correspondence: Richard W. S. Fynn, cmZ5bm5AdWIuYWMuYnc=
Disclaimer: All claims expressed in this article are solely those of the authors and do not necessarily represent those of their affiliated organizations, or those of the publisher, the editors and the reviewers. Any product that may be evaluated in this article or claim that may be made by its manufacturer is not guaranteed or endorsed by the publisher.
Research integrity at Frontiers
Learn more about the work of our research integrity team to safeguard the quality of each article we publish.