- 1Applied Aquatic Ecology, Arthur Rylah Institute for Environmental Research, Department of Environment, Land, Water and Planning, Heidelberg, VIC, Australia
- 2Institute for Land Water and Society, Charles Sturt University, Albury, NSW, Australia
- 3Operations Improvements, Murray–Darling Basin Authority, Canberra, ACT, Australia
- 4School of Geography, Earth and Atmospheric Sciences, The University of Melbourne, Melbourne, VIC, Australia
Introduction: Humans have substantially altered landscapes across the globe, generating novel ecosystems with varying states of modification. The principles of reconciliation ecology emphasise that such novel ecosystems must also be considered for conservation outcomes. This requires an understanding of how anthropogenic habitat alterations in a novel ecosystem may disrupt life cycle processes of key biota, thereby enabling the development of management strategies that may bypass or ameliorate potential bottlenecks in life history stages. We explore this by assessing Murray cod (Maccullochella peelii), a native fish species of considerable cultural, recreational and conservation significance in a severely altered river reach in south-eastern Australia. This species was considered to have disappeared in the reach following alterations to the river’s thermal profile (cold water pollution - CWP), but instead has persisted.
Methods: We examined the life cycle of Murray cod and use multiple lines of evidence to assess the impacts of CWP pollution on key processes such as adult migration, recruitment and juvenile survival. We also evaluated the potential for recovery and persistence of the species in this novel system using mitigation measures such as flow management and stocking and opportunistically tested their effectiveness.
Results: We found the key impact pathway of CWP on the Murray cod population is via its effects on the key processes influencing natural recruitment. Conversely, the effects of CWP on emigration and survival of larger juveniles and adults did not appear to be a major bottleneck for the population. Using a stochastic population model, we showed that the population could be sustained by stocking and opportunistic water management while still meeting irrigation demands. Monitoring to test our recommendations showed natural recruitment and improved survival of stocked fish during years with limited flow releases, thereby corroborating our predictions.
Discussion: Our use of multiple lines of evidence to assess a species’ responses to impacts of habitat alteration in a novel ecosystem enabled the formulation of recommendations for management that could then be tested. The success of such actions add to the growing body of literature that shows species of conservation significance can be maintained in novel ecosystems which should be specifically considered within the context of conservation planning across the landscape.
Introduction
Humans have substantially altered the major components and drivers of ecosystem functionality across the globe. The Anthropocene has seen ecosystems across the globe subject to rapid, novel, and substantial changes (Vitousek et al., 1997), with landscapes characterized by a mosaic of ecosystems in varying states of modification (Hobbs et al., 2014). Maintaining ecosystems and species in the face of these alterations and against the backdrop of climate change requires a pragmatic approach that moves beyond solely preservation and restoration of ‘natural ecosystems’ to incorporate the ecological services that altered systems can provide: the basis of ‘reconciliation ecology’ (Rosenzweig, 2003; Hobbs et al., 2006). Indeed, (Hobbs et al., 2014) point out that although ‘hybrid’ or ‘novel’ systems are the result of human alteration of the environment, that does not mean they cannot be manipulated to meet desired ecological outcomes. Furthermore, they present both challenges and opportunities that must be considered in broader conservation planning.
Escalating demand for fresh water by humans has severely altered rivers across the world through damming, diversions and flow regulation (Bunn and Arthington, 2002; Vörösmarty et al., 2010; Postel and Richter, 2012). Almost 47% of the world’s large rivers are affected by upstream dams (Lehner et al., 2011) while only 37% of rivers longer than 1,000 kilometres remain free-flowing over their entire length (Grill et al., 2019). The extent and likely continued development of riverine ecosystems make the principles of reconciliation ecology particularly relevant for conservation of freshwater ecosystems or significant species they support (Dudgeon et al., 2005).
The practical challenges that must be overcome in an altered river for an ecosystem or species to persist or recover can be immense. Scientists and managers require an understanding of how anthropogenic alterations to a habitat have disrupted the life cycle processes of the target biota, thereby enabling the development of management strategies that may bypass potential bottlenecks in life history stages. Native riverine fish populations have undergone dramatic declines globally, especially due to the construction of large storages for hydropower, irrigation and potable water that has altered hydrological connectivity and flow regimes. These impacts on fish populations occur through disruptions to life cycle processes such as spawning and migration (Lake, 1967; Dugan et al., 2010; Tonkin et al., 2019), the survival of early life stages (Baumgartner et al., 2006), and riverine productivity (Martinez et al., 1994; Robertson et al., 2001). Consequently, various management strategies have been adopted to address such disruptions and help recover fish populations in altered rivers for conservation, social, recreational and/or commercial benefits. Such programs include the construction of fishways on dams and weirs to restore fish passage (Lucas and Baras, 2008) and the use of environmental water to restore important flow regime components (Poff and Zimmerman, 2010).
Many of the world’s river reaches below large dams have had their temperature profiles severely altered. The release of water from low-level outlets positioned within the cool stratified layer of reservoirs (the hypolimnion) can severely reduce water temperatures in comparison to the natural profile of the system (Parisi et al., 2020), particularly during spring and summer (Lugg and Copeland, 2014; Gray et al., 2019). This cold water pollution (CWP) can impact remnant ‘warm water’ fish populations by disturbances to overall food availability (Todd et al., 2005), increasing competition with ‘cold water’ specialist species (Koehn et al., 1995), reducing cues for spawning and migration (Salinger and Anderson, 2006; Koehn et al., 2009; Moore et al., 2021), reducing survival of early life stages (Rowland, 1998; Clarkson and Childs, 2000; Todd et al., 2005; VanDeHey et al., 2013), and reducing growth and developmental rates (Ryan et al., 2003; Kaemingk et al., 2014). While many of the pathways by which CWP has impacted native fish populations are well established, the management of these reaches beyond permanent remediation via removal or engineering solutions (e.g. Gray et al., 2019; Michie et al., 2020) to achieve outcomes for remnant fish populations and still meet human needs (e.g. water transfer or power generation) has received less attention.
Over 3,000 km of Australia’s Murray-Darling Basin (MDB) are altered and subject to CWP as a consequence of low-level (below the hypolimnion) releases from dams (Lugg and Copeland, 2014). Native fish populations have declined by an estimated 90% in the MDB since European settlement, with CWP considered to be a major cause in many reaches (Koehn and Lintermans, 2012; Lugg and Copeland, 2014). It is often assumed that the functionality of native fish populations in these CWP reaches may be irredeemable without dam removal (unlikely) or permanent engineering solutions (Clarkson and Childs, 2000; Gray et al., 2019) because the novel thermal regime severely reduces reproductive success (e.g. Todd et al., 2005) and aspects of swimming capacity, particularly for juvenile fish (Whiterod, 2013). For other key life cycle processes such as growth, migration and survival, the impacts are less clear. However, evidence that some CWP-affected river reaches still support some of the species predicted to be impacted raises the question of how these species persist, and therefore, how these systems might be managed for species’ recovery or persistence in these altered habitats.
We present a case study of a native fish of the MDB, Murray cod Maccullochella peelii, a species of considerable economic (Ernst and Young, 2009), cultural (Ginns, 2012), recreational (Henry and Lyle, 2003) and conservation value. It is one of the largest freshwater fish in the world, historically growing up to 113 kg; now commonly to 40 kg (Lintermans, 2007). Murray cod has declined steeply in abundance, particularly in the last 70 years, and is listed as Vulnerable nationally under the Environment Protection and Biodiversity Conservation Act 1999 (www.environment.gov.au/resource/murray-cod-maccullochella-peelii-peelii). Reasons for decline include habitat loss and degradation, barriers to fish passage, flow regulation, CWP in reaches below impoundments, poor water quality and harvesting (in the past from commercial and now from legal recreational angling and illegal fishing) (Rowland, 2005; Koehn et al., 2020). Given the species value, there is need to manage Murray cod in altered systems, particularly given the extent of its current range encompass river reaches with severe regulation and temperature alterations (Lugg and Copeland, 2014).
Our study was conducted in an altered river reach where Murray Cod was presumed to have disappeared following dam construction and subsequent CWP impacts (Koehn et al., 1995) but has since persisted and even showed signs of recovery. We use multiple lines of evidence, spanning aspects of the life cycle of Murray cod, to test hypotheses (Table 1) that assess: 1) impacts of CWP on key population processes such as migration, spawning and juvenile survival, and 2) potential for recovery and persistence of Murray cod in this novel ecosystem using mitigation measures such as flow management and stocking. Opportunistic monitoring enabled us to test key results from management recommendations - an aspect of adaptive management that is rarely assessed or reported.
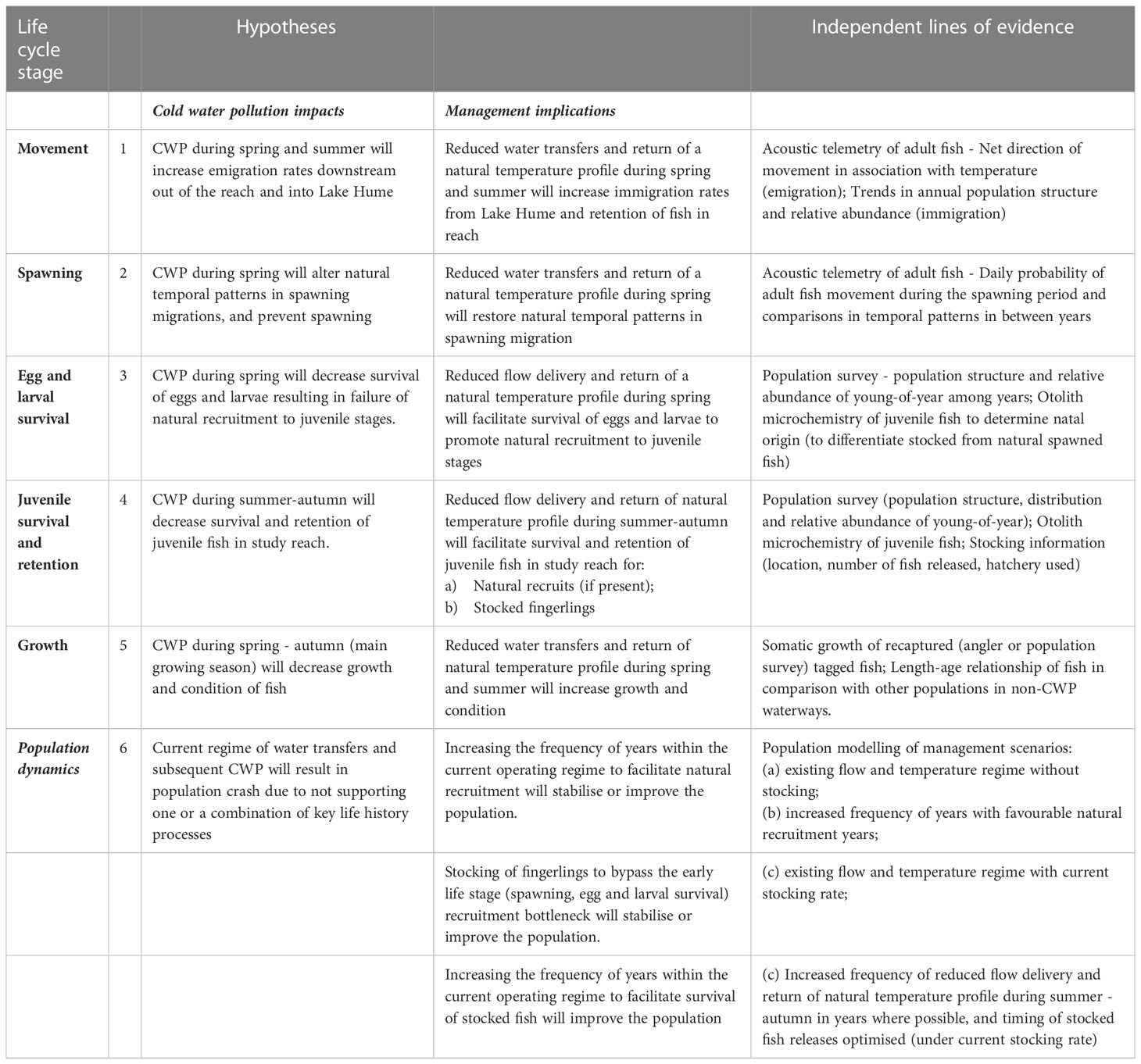
Table 1 Hypotheses for the effects of cold water pollution (CWP) on key life cycle stages and population dynamics of Murray cod; management implications or opportunities for each; and the lines of evidence used in this study to test these hypotheses.
Methods
Study species
Murray cod is a long-lived (maximum 48 years) apex predator that matures at around five years of age (Anderson et al., 1992; Koehn, 2009). Mature fish display limited movements and high site fidelity for much of the year, but do migrate during spawning in late winter/early spring (cued by either temperature or day length) (Koehn et al., 2009; Tonkin et al., 2022). Movements can also be associated with shifts in home range for recovery and dispersal (e.g. Thiem et al., 2017; Lyon et al., 2019; Tonkin et al., 2022).
Murray cod forms breeding pairs and lays adhesive eggs on hard substrata in spring, at temperatures greater than 15°C (Humphries, 2005; Koehn and Harrington, 2005; Koehn and Harrington, 2006), with the eggs then being guarded by the male fish (Rowland, 1998). The survival of fertilized eggs declines by 50% if water temperatures fall below 14.8°C, with 100% mortality occurring at 13°Cm (Todd et al., 2005). Larvae hatch after approximately 5−13 days, then disperse either actively or via downstream drift (Humphries, 2005; Koehn and Harrington, 2005; Koehn and Harrington, 2006) before settlement in the main river channel (Koehn and Harrington, 2006).
Study site
This study focussed on a reach of the lower Mitta Mitta River, a highly regulated floodplain river of south-eastern Australia (Figure 1) and typifies the many regulated reaches across the MDB. The study reach is approximately 48 km in length and makes up almost half the length of the regulated lower reach with a flow regime largely governed by releases from a large reservoir, Lake Dartmouth (36°30’S, 147°30’E). The Lake has a depth of 161 m and capacity of 3 856 GL and is the largest water storage in south-eastern Australia’s MDB. Construction was completed in 1979, with the purpose of augmenting irrigation water supplies to the Murray River system and providing electricity generation. Water is released from two outlets, 62 m and 121 m below Lake Dartmouth’s full supply level. The water within the lake stratifies, with a thermocline generally occurring at 5 to 10 m below the surface from October to January, and up to 40 m in August to September (Ebsary, 1990). As a result, water releases from Lake Dartmouth reduce water temperatures in the reach of the Mitta Mitta River between the dam and Lake Hume (approximately 100 km) during spring and summer by up to 12°C below natural levels (Ryan et al., 2003; Todd et al., 2005).
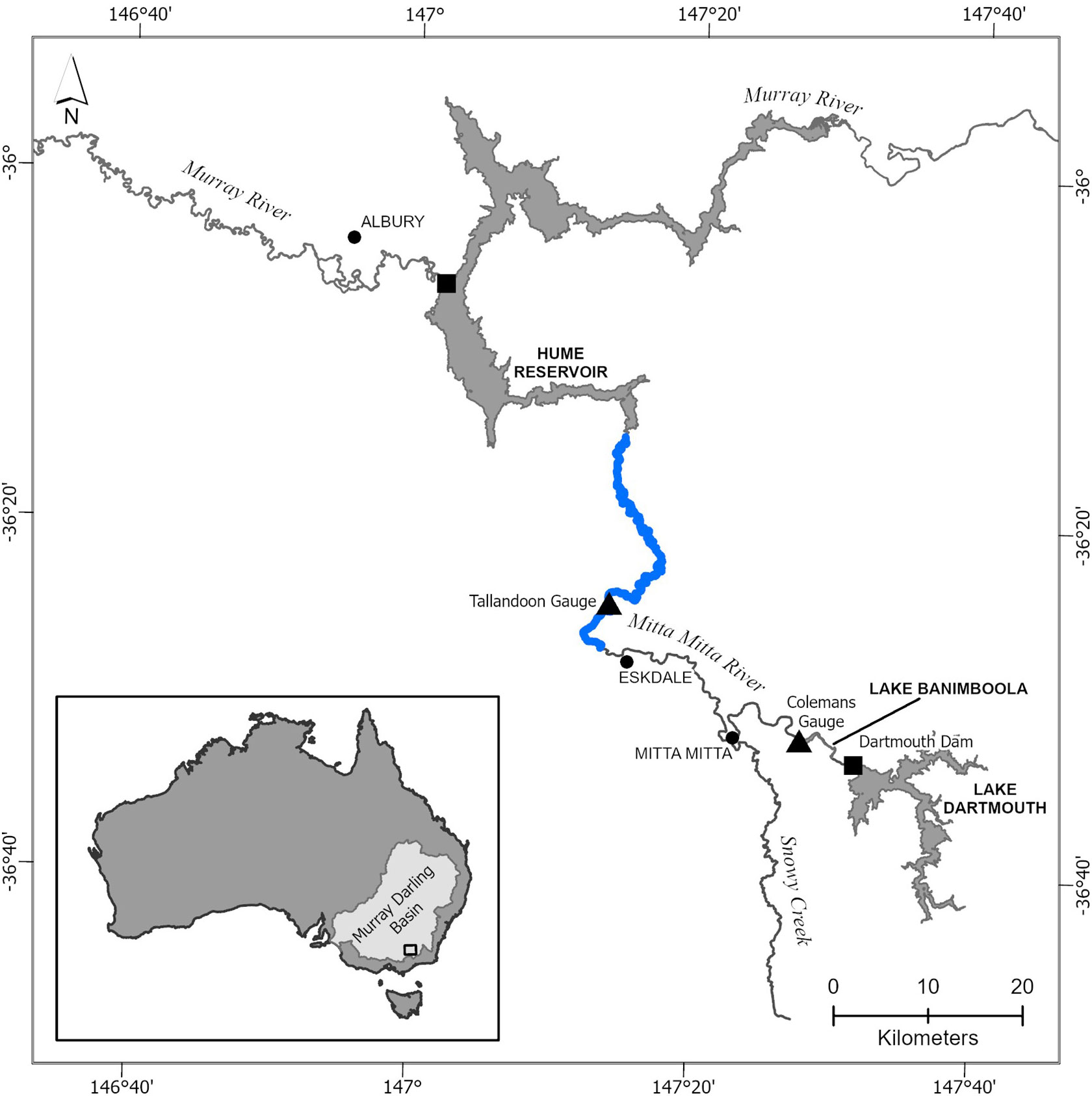
Figure 1 Map of the lower Mitta Mitta River. Focus river reach of the study highlighted in blue (48 km). The CWP reach extends from immediately below Lake Dartmouth to Hume Reservoir, approximately 100 km in length.
Environmental studies demonstrated substantial changes in the aquatic fauna in the lower Mitta Mitta River post construction/operation of the dam, including a shift from a ‘warm water’ native fish population to one dominated by introduced ‘cold water’ species, particularly brown trout Salmo trutta (Koehn et al., 1995). Of particular note was the apparent disappearance by 1992, of self-sustaining populations of Murray cod, Trout cod (M. macquariensis) and Macquarie perch (Macquaria australasica) (Koehn et al., 1995). Nevertheless, recreational fisher reports and fish surveys conducted since 2010 recorded an increasing number of Murray cod in the lower Mitta Mitta River (Lieschke et al., 2013). This has prompted waterway managers to reconsider their requirements for managing this river reach for improved ecological and recreational benefits while still achieving irrigation transfers and hydroelectric generation.
Existing infrastructure (only two water outlets) allows only a narrow window of opportunity to release water with no or minimal reductions from natural temperatures, occurring just three times in the past decade (Murray-Darling Basin Authority, unpublished data). The study reach receives inflows from several smaller unregulated tributaries but these have very little influence on downstream water temperatures during sustained periods of above-minimum water releases from Lake Dartmouth. From 2006 – 2018, the reach has also been stocked with 10 000 – 40 000 hatchery-produced Murray cod fingerlings each year (January and February liberation).
River hydrology and thermal conditions during the study period
The first three years of the study (May 2013–May 2016) coincided with prolonged periods of high spring flows in the Mitta Mitta River. During this period, water was released from below the thermocline meaning that temperatures in the Mitta Mitta River (recorded at Tallandoon) during the spring spawning period for Murray cod (October and November) only occasionally exceeded the 50%-mortality threshold temperature for larvae of 14.8°C (Todd et al., 2005) and frequently dropped below the 100%-mortality threshold of 13°C (Figure 2). Discharge in early 2016 was also high throughout January, with a gradual reduction to < 1 000 ML day-1 in March (Figure 2). Water temperatures in early 2016 were much higher than the previous two summers, even during high-flow periods, increasing in early January from 15°C to a high of 23.4°C in early March.
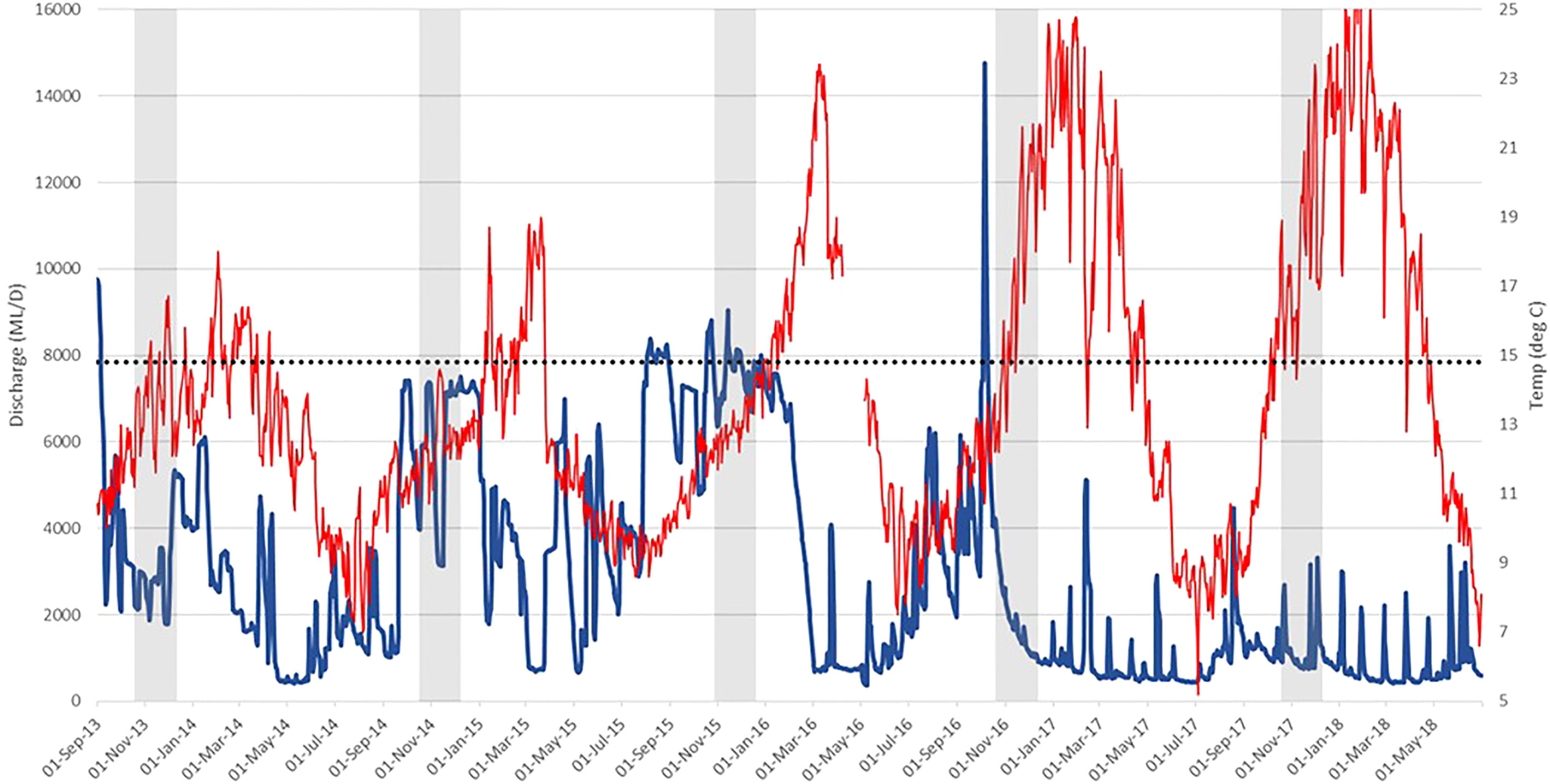
Figure 2 Average daily discharge (blue) and water temperature (red) data for the lower Mitta Mitta River during the core study period from 2013 – 2018. Dotted horizontal line = egg and larval survival threshold of 14.8°C (50% survival as predicted by Todd et al., 2005). Vertical grey bars represent core egg and larval period for Murray cod.
River flow and water temperatures in the lower Mitta Mitta River during the final two years of the study (May 2016–May 2017) differed greatly from those in the previous three years (Figure 2). Discharge at Tallandoon in early October 2016 reached the highest levels during the study (14,765 ML day-1), due largely to high inflows of unregulated tributaries, but then declined by the end of November, with minimal contribution from Lake Dartmouth releases. These flows resulted in water temperatures during the Murray cod spawning period above 14.8°C for more than 50% of the time, peaking at 21.6°C and 23.4°C in 2016 and 2017, respectively (Figure 2). Summer and autumn water temperatures in these years generally ranged between 20–25°C. There were, however, drops in water temperatures with minimums as low as 12.8°C and 14.3°C during November 2016 and 2017 respectively, and 12.9°C and 12.8°C in February 2017 and March 2018, respectively.
Finally, following the results and management recommendations generated during the core data collection period from 2013-2018, discussions with river operators indicated potential for minimal water transfers through the study reach during both spring and summer in 2020/21. This enabled discharge during the core spawning period of Murray cod in spring 2020 to remain low, driven primarily by unregulated tributary inflows. As such, water temperature only briefly dipped below 14.8°C in mid-October, remaining above this survival threshold for the remainder of the spawning period (Supplementary Material 1). Summer discharge also remained low, with water temperatures from summer through to early autumn remaining above 19°C (and usually >21°C).
Assessing influence of CWP on key vital rates and population dynamics of Murray cod
We used a range of techniques and multiple independent lines of evidence to assess impacts of CWP on key population processes of movement, natal origin, age structure, and stocked fish survival. We then used our leanings to help refine a population model developed for Murray cod (see the sections below) to assess population dynamics and also explore the potential for recovery and persistence of the species using mitigation measures such as flow management and stocking.
Population demography
Under low flow conditions, the study reach is on average approximately 20m wide, very low turbidity (mostly <10 NTU) and rarely exceeds 3-4m in maximum depth. As such, data generated using standardised boat electrofishing surveys were used for determining population demography. The method was used from 2011–2021, specifically to capture fish for implanting with telemetry tags (see Migration section below), collect juvenile fish for natal origin (see Natal origin section below) and generate data to assess population structure and subsequent growth, survival and year-class strength (YCS). Between five and 40 sites were surveyed in 2011 (five sites; Lieschke et al., 2013), 2013 (seven sites), 2015, 2017, 2018 and 2021 (40 sites each year). In each of these years, surveys were conducted between March and May, a period specifically selected to coincide with periods of low river levels (i.e. periods of minimum irrigation transfers) and minimise the effect of changing river levels on electrofishing efficiency.
Electrofishing at each site consisted of single-pass, boat-mounted electrofishing (Smith Root® Model 5 KVa generator-powered pulsator), conducted in an upstream direction. This technique has been used extensively across the MDB to assess fish populations, including a basin wide long term monitoring program (Sustainable Rivers Audit; Lieschke et al., 2013). Sites were approximately 100 m long, usually along an outside bend of the river (noting the adjacent bank was usually too shallow to navigate and indeed, hold fish). All wetted accessible habitats were surveyed between the pre-defined downstream start and upstream site end points. Parameters recorded at each site included: electrofishing effort (total seconds fished), distance fished and site depth (average and maximum). All fish captured were identified and counted. For Murray cod, their total length (mm) and weight (nearest gram) were recorded. All Murray cod greater than 200 mm total length were fitted with an external dart or T-Bar tag to provide addition information on estimates of growth, movement, and mortality (from recreational fishing) via catch-mark-recapture data. All fish were released at the site of capture after being processed.
The size structure of fish populations was first explored using size-frequency histograms generated from Murray cod length data collected during each electrofishing survey of the Mitta Mitta River from 2011–2018. This exploration revealed a bimodal distribution of fish in some years. Therefore, to assess temporal changes in the length distributions, we used quantile regressions using the rq() function in the quantreg package (Koenker, 2017) in the statistical program R (R Core Team 2021). First, we assessed whether median lengths changed linearly over time by running a quantile regression that included sample year as the interaction, and then whether the lower 20th percentile of fish lengths (juveniles) changed among years.
Movement
We used acoustic telemetry to investigate our hypotheses regarding the effects of CWP on emigration and spawning migration behaviour (Table 1). In May 2013, ten acoustic receiver stations (Model VR2W; Vemco, Nova Scotia, Canada) were installed along the study reach. This was immediately followed by the 2013 electrofishing surveys, during which 25 adult Murray cod (486–1055 mm TL (Supplementary Material 2) were implanted with Vemco Model V16-4x, 69-kHz acoustic transmitters (estimated battery life of 2356 days). While fish were distributed throughout the study reach, a greater proportion of fish was tagged in the most downstream sections (Supplementary Material 3). Details of surgical procedures are described in (Tonkin et al., 2022). Acoustic receivers were visited annually between March and May from 2014–2018, for battery replacement and data download.
We analysed movement as changes in location (defined as the reach between listening stations) and direction. A change in location was defined as a fish being detected at a station not associated with its previous location, with the direction a fish had moved (upstream or downstream) also determined (change relative to its previous position). We then investigated how flow, temperature and time of year influenced these movements, especially the effect of CWP on emigration and spawning migration behaviour. We hypothesised that the influence of these factors would be greater prior to June 2016 (characterised by unseasonal high discharge and low water temperatures during spring and summer) and less in the subsequent years that had low discharge and more seasonal water temperatures during spring and summer.
For these two periods, the effects of flow, temperature and time of year on the likelihood of detection, movement and direction of movement were analysed using general additive mixed models (GAMM). In particular, we considered fixed effects related to flow and temperature, their variability, and change from the previous measure. The time of year was considered in two separate ways, either as a smoothed time-of-year or as a factor with levels of spawning, return from spawning and all other times.
The direction of fish movement, upstream or downstream, was first analysed by determining the independence of direction of movement and the time-of-year as a factor using a χ2 test. Then, the effect of two periods, discharge, temperature and time of year (as fixed effects) and individual fish (as a random effect) on the likelihood of a movement being upstream (as opposed to downstream) was analysed using a GAMM. The effect of time of year was done using a cyclic smoother over the days of the year for each period separately. The analysis was conducted using the statistical program R 3.4.4 (R Core Team 2021) and the package gamm4 (Wood and Scheipl, 2014). Final model selection was achieved using Akaike information criteria (AICc), corrected for small samples to determine the model with the most evidence (Burnham and Anderson, 2010).
Natal origin
Distinguishing fish as either stocked or naturally spawned was integral to the objectives of this project and we used two methods to determine this. Firstly, all Murray cod stocked between 2013 and 2015 (inclusive) were chemically marked (calcein) allowing their identification as hatchery-produced fish. Detection of these marked fish required otoliths to be removed, thin-sectioned and viewed microscopically for the presence of the calcein mark using fluorescence backscatter (e.g. Crook et al., 2016). The second method used the fish’s otolith chemical composition to identify its birthplace. Specifically, we used laser ablation inductively coupled plasma mass spectrometry (LA-ICPMS, strontium isotope ratios) to discriminate between recently stocked and naturally spawned fish based on otolith strontium isotope ratios (87Sr/86Sr) as this partially reflects the chemistry of ambient water at the time of deposition (Campana and Thorrold, 2001). In rivers and streams, dissolved 87Sr/86Sr are an artefact of catchment geology and are increasingly being used to discern the natal habitats and movements of numerous diadromous and freshwater fishes in the southern MDB (e.g. Thiem et al., 2022; Zampatti et al., 2022).
In autumn 2017, 2018 and 2021, otoliths were collected from 35 juvenile (<150 mm TL, age <2 years) Murray cod in the Mitta Mitta River. We then sampled the 87Sr/86Sr at the core and edge of otolith transverse sections which represent the fish’s place of birth and capture, respectively. We also compared 87Sr/86Sr of the samples from the Mitta Mitta River with the otolith core values generated from 47 Murray cod fingerlings sourced from each of the hatcheries used to stock the Mitta Mitta River during from 2016–2021.
Sagittal otoliths from juvenile fish were removed and prepared for ageing and LA-ICPMS using transverse sections. The age (and therefore year class) of each fish was determined by macroscopic age analysis of each otolith section as described in (Anderson et al., 1992). We then used microchemistry LA-ICPMS analysis of 87Sr/86Sr ratios from the otolith edge (from wild fish) and core (hatchery and wild fish) using an experimental system consisting of a ‘Nu Plasma’ multi-collector ICPMS (Nu Instruments, Wrexham, UK), coupled to a ‘RESOlution’ 193-nm excimer laser ablation system (Applied Spectra, USA). Each otolith was ablated on the primordium (core) and the dorsal margin at the widest radius using a 60-µm laser spot. Ablation was performed under a pure helium (He) atmosphere followed by rapid transport of the ablated products to the MC-ICPMS in the argon carrier gas. Methods for online correction for isobaric interferences and mass bias are described in (Woodhead et al., 2005), while accuracy and reproducibility was checked after every 10 otolith ablations using methods described in (Thiem et al., 2022).
We first tested whether the hatchery and Mitta Mitta River fish had different 87Sr/86Sr values and whether these differences varied between years. To do this, we used an Analysis of Variance (ANOVA), comparing 87Sr/86Sr values at the otolith edge from fish captured in the Mitta Mitta River and the otolith core from hatchery fish. We then ran a simulation to determine whether 87Sr/86Sr measured from the otolith core (natal origin) of each juvenile Murray cod captured in the Mitta Mitta River was more closely matched with the otolith edge chemical signatures of the Mitta Mitta River (wild fish) or the hatchery (stocked fish) for each year. Natal origin as either a wild or stocked recruit was only assigned if it was a ≥95% match with the chemical signature to either the Mitta Mitta River or the hatchery.
Age structure and stocked fish survival
We used a sample of sagittal otoliths from 39 Murray cod (49–790 mm TL) collected and euthanized during electrofishing throughout the project. As well as enabling the natal origin of juvenile fish to be determined, it also provided a broad assessment of length-age relationships to determine year-class strength. Based on the ages of fish generated from the otolith assessment, it was determined that the length-age relationship of Murray cod in the Mitta Mitta River was similar to other populations in the region (Supplementary Material 4). As such, a length dataset from all Murray cod captured during electrofishing surveys in the Mitta Mitta River from 2011–2018 was used to estimate ages of individuals using the species growth model reported in (Todd and Koehn, 2008; see also description in Supplementary Material 4).
We used our estimates of age to assess how flows and temperature in the lower Mitta Mitta River influenced YCS. It was determined that all juvenile fish captured between 2016 and 2018 were of stocked origin (see results Natal origin). Therefore, YCS was estimated by calculating a relative offspring survival probability using the ratio of catch of each year class vs the number of fingerlings stocked into the Mitta Mitta River for the same year (source https://vfa.vic.gov.au/db/native-fish-release-summary). We focussed our analysis on age classes 1 – 3 years due to the low confidence of age estimates for larger fish, and age 0 (YOY) fingerlings sometimes released just weeks before sampling. Offspring of the three age classes was modelled separately as a binomial variable, with a logit link function using generalised linear models. In these models, fixed-effect predictors were electrofishing seconds (to account for effort in detection; null model) and various metrics representing the temperature and discharge dynamics (mean, minimum, extreme events and variation) during the stocking period (day of and 30 days following fish release) that may influence fingerling survival (Table 2). As expected, several of the flow and temperature metrics were correlated so only four of these fixed effects were tested (see Table 2). Each age-based model had a stocking window metric compared to the null model using the Akaike information criteria corrected for small samples (AICc) to identify the model which best fit the data.
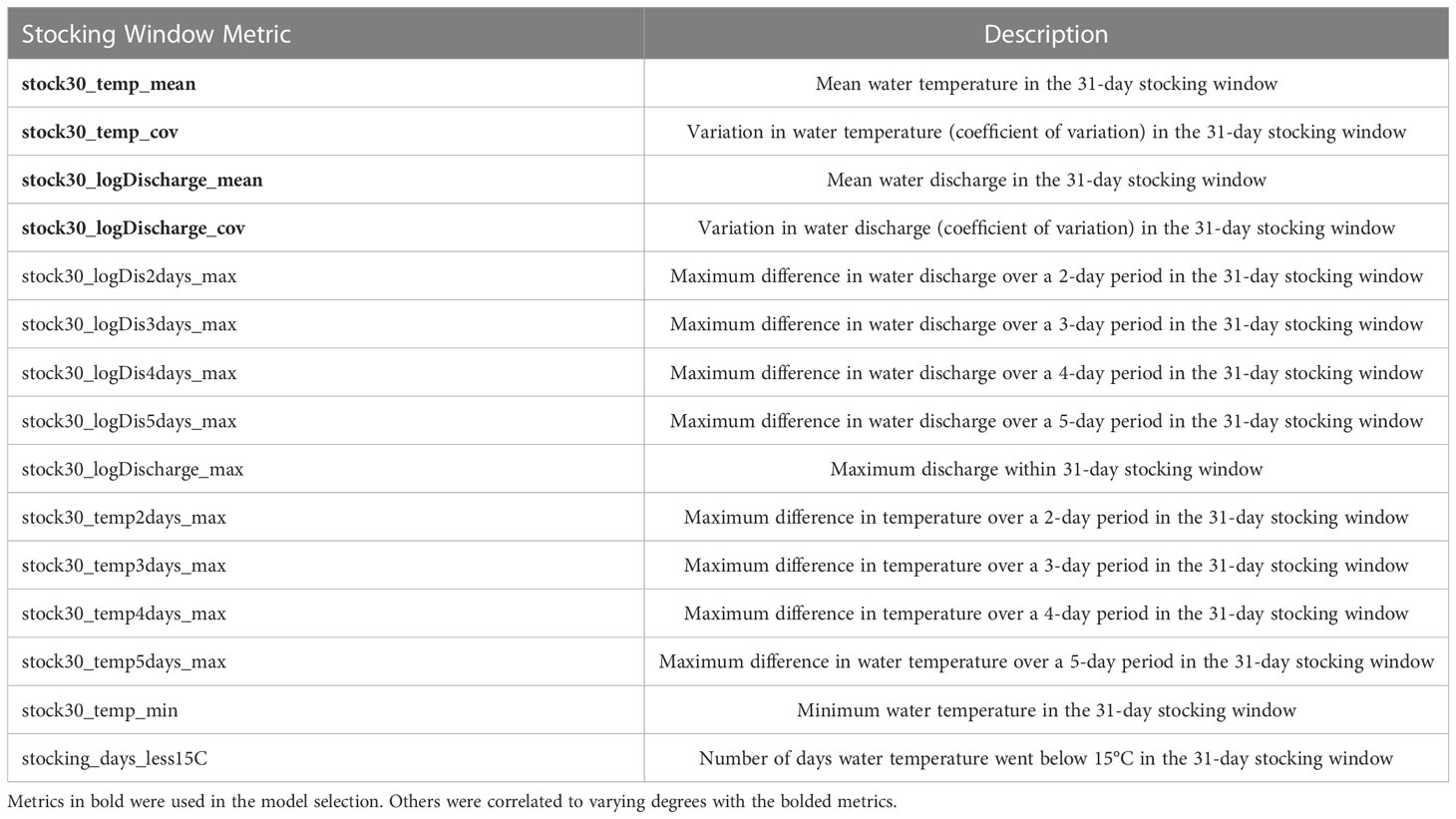
Table 2 Temperature and river flow covariates used to predict survival of Murray cod fingerlings in the lower Mitta Mitta River.
Assessing population dynamics under variety of management scenarios
A Murray cod population model previously developed for the MDB (Todd and Koehn, 2008; Koehn and Todd, 2012) was refined (see below) and used to examine the likely influence of past temperature impacts on survival for two vital rates (see below) and subsequent population dynamics; and use these outputs to explore different management scenarios, specifically altered flow delivery and stocking. The model summarises the life history of Murray cod by explicitly representing 50 age classes, where entry into the first age class combines spawning, egg, larval and early juvenile survival, and where early juvenile fish are defined as those less than one year old.
Murray cod are assumed to become sexually mature at five years of age, with fecundity increasing depending on the maximum size of fish in the population. For the Mitta Mitta River population, the average maximum size is approximately 110 cm. The model only accounts for females, as males are not considered to limit the population in any way (Todd and Koehn, 2008; Koehn and Todd, 2012), although it is recognised that males are likely to play an integral role in the successful development of eggs hatching into larvae (Rowland, 1998). A 1:1 sex ratio was assumed. The model includes stochastic processes for modelling variation in fecundity rates in all adult age classes and variation in survival in all age and stage classes each year. Variability in fecundity and survival rates was incorporated to simulate the process of natural variation in the environment by modelling demographic and environmental stochasticity (Akçakaya, 1991; Todd and Ng, 2001). Age-specific variation in growth was included as were processes for modelling the effects of density-dependence, with the choice of carrying capacity set by the user. For the purposes of this study, a long-term average of 10,000 female adults was used as the level above which density-dependence acts on the adult population size (for details see Todd and Koehn, 2008; Koehn and Todd, 2012), based on an assumed maximum density of one female adult Murray Cod every 10 m. This assumption was supported by detailed catch–mark–recapture data from the Murray River at Yarrawonga, which supports the estimates of population size (Lyon et al., 2019). We used an initial population size of 1500 female adults (an assumed density of one female adult Murray Cod every 66m) with all scenarios executed over 45 timesteps, equivalent to 45 years. Given the results of the movement component that found fish were largely sedentary in the reach (see Migration results below), immigration and emigration were not included in the model.
A time series of temperature from the Tallandoon gauge on the Mitta Mitta River was collected from 2004 to 2018 (15 years of daily water temperature records). This datum was analysed for the thermal effects on survival of:
1) eggs and larvae, and therefore the likely natural recruitment of Murray cod in the Mitta Mitta River: using the logistic equation from Todd et al. (2005) modelling temperature effects on eggs and larval survival combined with the likelihood of spawning based on a right-skewed beta distribution for the spawning season October 1st to December 31st to generate a probability of spawning success for that year, and;
2) stocked fingerlings, using the relationship generated in this study (see Stocked fish survival results below).
The scenarios, generated from the past 15 years and used to model the trajectory of the population and its risk of falling below 500 adult females were:
Scenario 1: Actual natural recruitment conditions without stocking using the observed thermal time series and subsequent likely natural recruitment success (see Supplementary Material 5; equivalent to 2 in 15 years of successful recruitment).
Scenario 2: Modified natural recruitment conditions without stocking using a modified thermal time series to test variations in the frequency of natural recruitment above and below the existing recruitment frequency (equivalent to 1 in 15 years, 3 in 15 years, and 4 in 15 years).
Scenario 3: Scenario 1 with the observed stocking (timing and numbers of fish released; see Supplementary Material 6) which accounted for the river temperature at the time of release. This scenario represents what has occurred in the Mitta Mitta River the past 15 years.
Scenario 4: Optimal summer flow and temperature conditions and stocked fish release times using the same stocking time series together with temperature records, releasing the fish at the optimal thermal level for that season. This was calculated by firstly using an alternative summer water temperature in a retrospective manner where water could have been released earlier in the season rather than the actual summer release dates (Murray-Darling Basin Authority unpublished data). Secondly, the date that fish were released was altered so as to align with the optimal summer thermal period in each season (warmest 30-day period), thus giving the optimal scenario for the survival of stocked fish.
The number of stocked fish was halved to represent the female proportion of fish when included in the population model, assuming an even sex ratio of stocked fish. The thermal time series and stocking time series were replicated three times to derive an overall time series equivalent to 45 years to capture consequences for the population dynamics over the longer term. All scenarios have a background angler capture rate of 10% (chance of capture) of fish in the legal-size range 55 cm to 75 cm.
Trajectory summaries, including the average (± 1 standard deviation), maximum and minimum female adult population size from each scenario were used to provide a scenario overview. To compare the four thermal time series, the average female adult population size only was used; similarly, for the stocking time series. In addition to using trajectory summary information, risk curves and expected minimum population sizes were used for comparing scenarios. Population risk is characterised by changes in the cumulative frequency distribution of the minimum population size, also known as a risk curve. The risk curve represents the probability of falling to or below a given population size or threshold population size over the period for which the model is run and is commonly used in conservation management (Akçakaya, 1991; Todd and Ng, 2001). To quantify differences between risk curves, the expected minimum population size was calculated as the expected value of the cumulative frequency distribution and may be compared among scenarios.
Results
Population demography
The size structure of Murray cod captured in the study reach varied considerably in each survey year. This was driven largely by changes in the abundance of juvenile fish in the system (Figure 3). The median length of Murray cod captured in the Mitta Mitta River significantly increased from 204 mm in 2011 to 288 mm and 386 mm in 2013 and 2015, respectively (Supplementary Material 7). Conversely, the median length of Murray cod captured in 2017 was significantly lower than in 2011, 2013 and 2015. This change was driven by variation in the abundance of juvenile fish with the analysis of fish in the lower 20th percentile of size also varying across the survey years (Figure 3). The median sizes of juvenile fish captured in 2011, 2017 and 2018 were 161 mm, 98 mm and 196 mm, respectively (compared to 279 mm and 306 mm in 2013 and 2015). In 2021 (our opportunistic monitoring), the median fish size was 137 mm, within the range of 2011, 2017 and 2018 values.
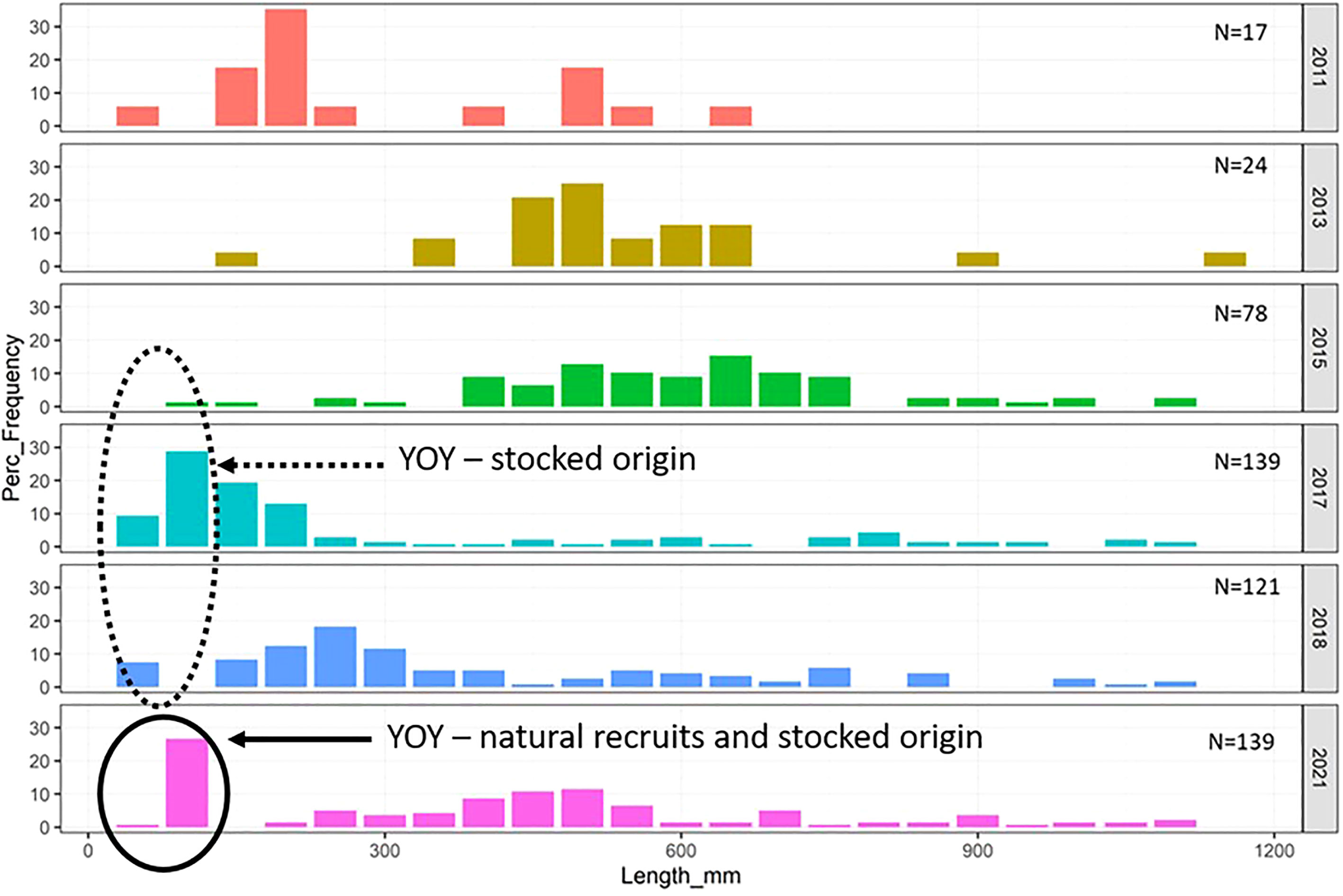
Figure 3 Length (mm) Frequency (%) of all Murray cod captured during electrofishing surveys in the lower Mitta Mitta River from 2011–2021. The presence of young-of-year (YOY) fish (<120 mm length) with a summary of their natal origin is also shown.
Migration
Of the 25 tagged Murray cod, three fish were not detected again after their release, although one of these fish was caught and released by an angler close to its original tagging location. More than 1 800 000 detections (condensed to 4 850 daily detections) from 22 of the 25 tagged fish were investigated for movement. In general, the 23 tagged fish displayed strong site fidelity with 10 fish detected on fewer than 20 days of the study period and a very low probability of movement beyond their tagging location (estimated 1-5% on any given day, Figure 4; Supplementary Material 2). Nevertheless, 21 fish (84% of tagged fish) moved beyond their tagging location in the form of either a seasonal spawning-type behaviour (with return movements) or a change in location (Supplementary Material 2). There were some large movements, with one fish moving more than 50 km upstream (in 26 days) and returning the same distance downstream (taking just 16 days) in each of the three years during the study. No fish were recorded at the most upstream receiver during the first two years of the study.
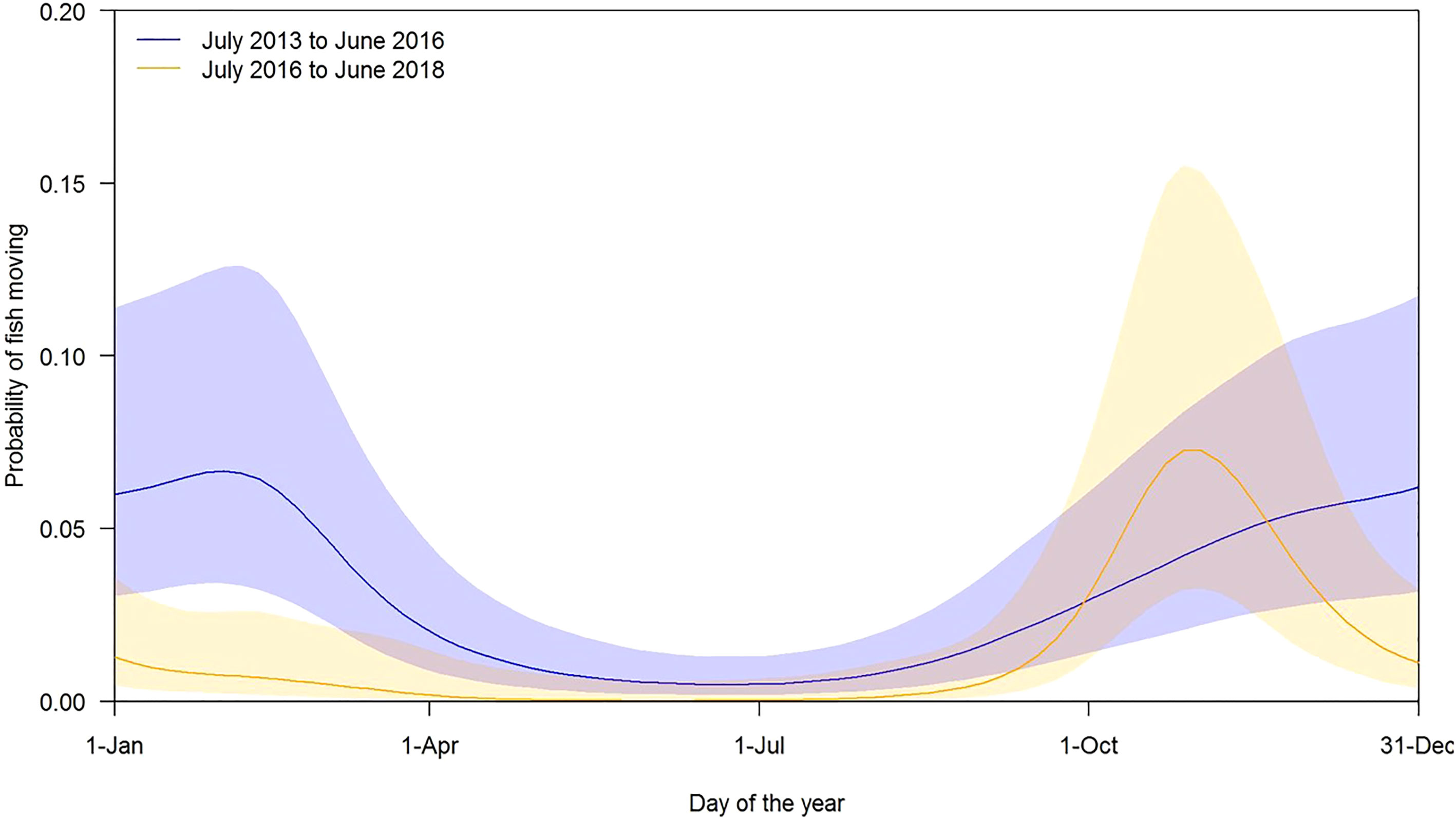
Figure 4 The expected probability of adult Murray cod moving throughout the year during each of the study periods, given average temperatures for that time of the year.
Detections were analysed over a fortnightly (14-day) time-step because of the sparseness of daily and weekly data. There is little evidence that temperature, discharge and time of year affect the direction of Murray cod movement in this study (Supplementary Materials 8A, B). Of the fish that moved, most were downstream, however, the χ2 test for independence did not show any significant departure from independence (p-value = 0.33). Furthermore, when other explanatory variables were used to analyse the data, the null GAMM model had the smallest AICc (Supplementary Material 8A).
The best model explaining movement (location change) included temperature, time-frame (before or after June 2016) and the cyclic smoothed day of the year as explanatory variables (Supplementary Materials 8C–E). The model explained only a small amount of the variation (adjusted R2 = 3.0%). Peak movement times differed before and after July 2016 (Figure 4). Prior to July 2016, movement rates peaked during February while after July 2016, the peak movement period was much earlier in the season, from October to November (Figure 4). Movements were very unlikely (<1%) from May to August (Figure 4). The model also showed that at higher temperatures, the likelihood of a Murray cod moving increases. The coefficient (on the logit scale) for the standardised temperature is 0.55 (95% CI is 0.04 to 1.05) with the odds of a fish moving increasing by a factor of 4.3 when the temperature increases from 12°C to 23°C. In general, there was less fish movement in the later period, with the odds of movement being reduced by an average of 86% (95% CI from 61% to 95%).
Natal origin
Just three fish captured in 2013 and 2015 were of a size estimated to represent fish stocked or spawned in the 2013–2015 period inclusive (112–161 mm TL). All three fish were determined to be of stocked origin, based on the presence of a calcein mark on their otoliths. For latter years, our comparison of otolith edge 87Sr/86Sr ratios of fish caught from the Mitta Mitta River with otolith core values from hatchery-reared fish indicated that all but one hatchery (used in a single stocking event) had distinguishable otolith 87Sr/86Sr ratios for their respective stocking years. Specifically, despite significant differences in the otolith 87Sr/86Sr ratios within the Mitta Mitta River amongst years (ANOVA, p<0.05), these values were significantly different from the Murray Darling Fisheries (MDF), Snobs Creek and Murrumbidgee hatcheries in their respective years (Figure 5; all p<0.001, Supplementary Materials 9, 10). Only at the Maintongoon hatchery, that was used to stock some fish in 2017, were the otolith 87Sr/86Sr ratios indistinguishable from the Mitta Mitta River (ANOVA, p>0.05, Supplementary Materials 9, 10).
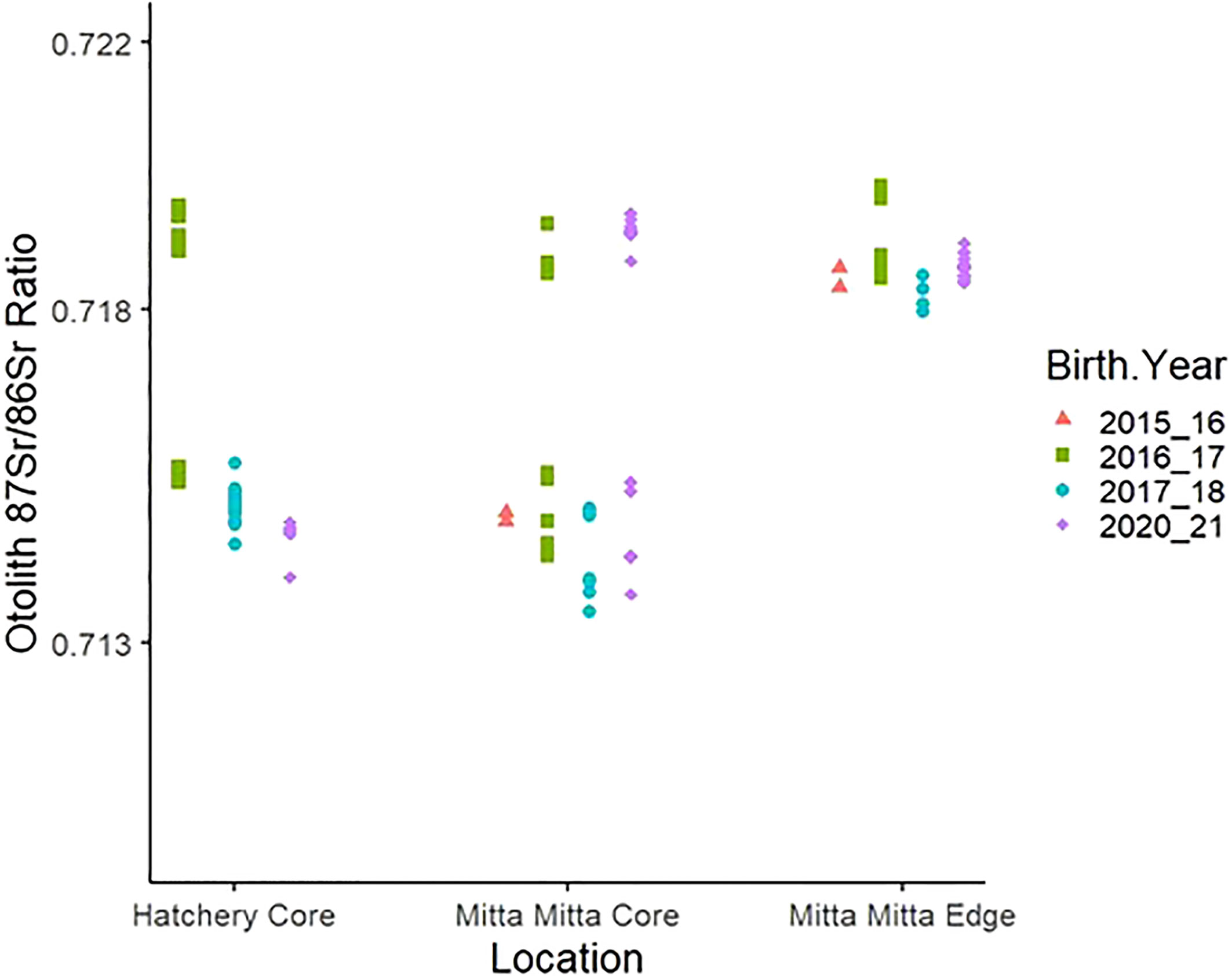
Figure 5 Otolith 87Sr/86Sr ratios measured from the otolith core (natal signature) of each individual hatchery fish and Mitta Mitta River-caught fish, and the otolith edge of each the Mitta Mitta River-caught fish (river signature).
All juvenile Murray cod captured in the Mitta Mitta River which had a birth year of 2015/16 and 2017/18 were found to be hatchery-reared (Figure 5). For the 2016/17 year class, 45% (n=5) of fish displayed natal origin (otolith core 87Sr/86Sr ratios) analogous to the Mitta Mitta River. However, closer investigation revealed these fish were captured at, or near (<1 km), a late-season stocking of Murray cod that were produced at the Maintongoon hatchery located on the upper Goulburn River. These fish were 60% smaller in size (length) than other YOY confirmed to have been stocked three months prior (mean TL = 96.33 ± 6.19mm and 57.80 ± 8.58mm, respectively). Taking this into consideration, we expect that the 2016/17 cohort of fish assigned natal origin belonging to the Mitta Mitta River are in fact stocked from Maintongoon hatchery (the only time this hatchery was used for the waterway), which shares a similar chemical signature value with the Mitta Mitta River (Figure 5). Of the 16 Murray cod samples from the 2020/21 cohort, 70% (n=11) were assigned as wild-spawned natal origin whereas five fish (all of which were collected from the stocking location or sites in close proximity downstream) were identified as stocked from the hatchery (Figure 5). This represented the first confirmed natural recruitment of Murray cod in the lower Mitta Mitta River during the entire study period.
Age structure and stocked fish survival
As verified by our ageing data, our estimates of age structure (derived from age-length relationships and otolith data) for fish captured from 2011–2018 used for our survival assessment revealed a high number of fish belonging to the 2015/16 and 2016/17 year classes, with earlier surveys showing 2008/09, 2009/10 and 2010/11 year classes were also prominent (Supplementary Material 11). All of these years coincided with relatively high summer water temperatures, usually (but not always) associated with minimal water releases from Lake Dartmouth.
Binomial models catering for fishing effort (electrofishing seconds) showed that the model which included average temperature for the 30 days following stocking best fit the data for predicting capture of a stocked cohort (our proxy for the survival rate of stocked fish) from 1 to 3 years of age (Supplementary Material 12). About 99% of the variation in survival of stocked fish captured at age 1 and age 3 was explained by fishing effort and average stocking window temperature, while it was 61% for catches of age 2 fish (Supplementary Material 13). There was a significant positive relationship between average temperature within the first 30 days of stocking and survival (Supplementary Material 13). The model for catches of the 1-year age-class equated to a 63% reduction in survival for every one-degree reduction in mean daily water temperature (Figure 6). Effectively, survival (and capture) of fish was very unlikely if mean water temperature was less than 20°C for the 30-day period following fish release (during summer or early autumn).
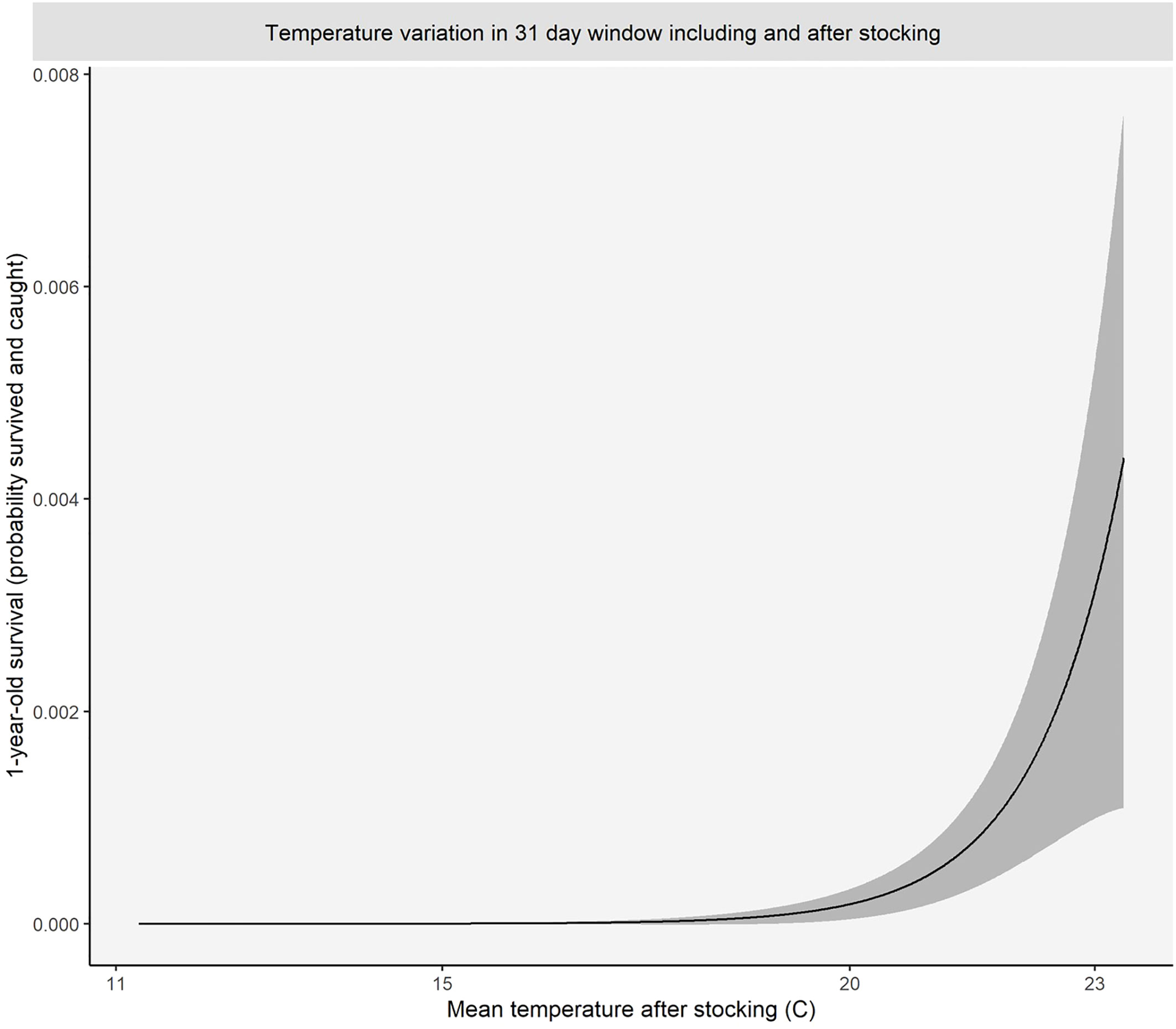
Figure 6 Predicted survival of fingerlings to age 1+ based under varying water temperatures in the 31 days following release in summer (based on average fishing effort).
Assessing population dynamics under variety of management scenarios
In Scenario 1, the observed thermal time series (2 in 15 years of successful recruitment) indicates that the Murray cod population would be in decline in all but a few trajectories over the 45-year time span (Figure 7A). In Scenario 2, which changed the thermal time series and subsequent recruitment frequency to 1 in 15, 3 in 15 and 4 in 15, on average, none of the modelled natural recruitment achieves population growth; however, the 4-in-15 scenario achieves the largest average female adult population size (Figure 7B).
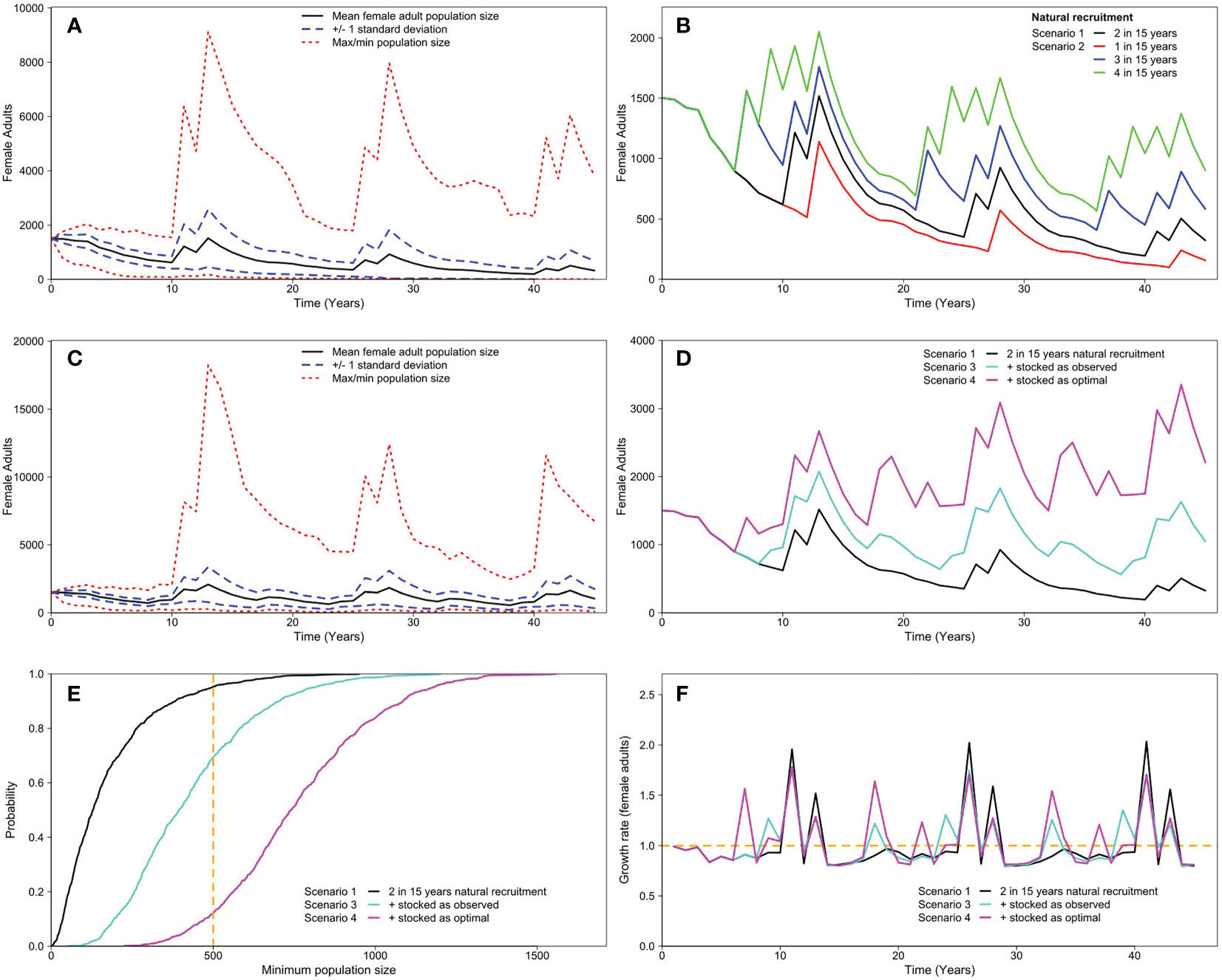
Figure 7 Summary of the modelled female Murray cod adult population trajectories in the Mitta Mitta River for (A) Scenario 1 - the observed thermal time series (2 in 15 years) for natural recruitment; (B) Scenario 2 - modified thermal time series for natural recruitment (Red: 1 in 15 years; Blue: 3 in 15 years; Green: 4 in 15 years) in comparison with Scenario 1; (C) Scenario 3 - the observed stocked and natural recruitment time series in the Murray cod population model for the Mitta Mitta River; (D) Scenario 1, Scenario 3 and Scenario 4 - the optimal stocked time series; (E) the associated risk curves for each of the scenarios presented in (D) with the vertical dashed line the threshold population size of 500 female adults and (F) the associated female adult growth rate to the scenarios presented in (D).
In Scenario 3, the observed stocking and natural recruitment time series, there were periods of population increase and population decrease but overall the average trajectory is relatively stable through time (Figure 7C). The average female adult population size from this actual scenario is compared with the estimates from the natural recruitment conditions without stocking (Scenario 1), and the average female adult population size from the optimal stocking time series (Figure 7D). Our model predicted a 145% increase in the expected average minimum female adult population size under the current stocking program in comparison to the current conditions (2: 15 years recruitment without stocking). This increased to a 336% change for the optimal stocked scenario. The risk curves show the probability of falling to 500 female adults at least once over the 45-year model period was 95% for the actual recruitment scenario (Scenario 1), but this reduced to 69% with stocked as observed scenario, and to 12% for the optimal stocking scenario (Figure 7E). The growth rate through time shows that there are periods where the growth rate is less than 1 indicating periods of population decline and years when the growth rate is greater than 1 indicating an increase in the population size (Figure 7F). The overall average growth rate was 1.0024 (Scenario 1); 1.0172 (Scenario 3); and 1.0399 (Scenario 4) (Figure 7F). Under optimal stocking (Scenario 4) there remains periods where the population growth rate is less than 1, however the growth rate was greater than 1 42% of the time, 31% of the time for Scenario 3, and only 13% of the time for Scenario 1, even though the overall average the growth rate was marginally greater than 1.
Discussion
Our study has used multiple lines of evidence that encompassed a range of life history processes for our study species to assess the impacts of CWP and whether management options within the current operating framework can facilitate sustaining or recovering a long-lived native fish species in an altered and now novel river reach. We found that the key pathway to impacts of CWP on this fish population was via its effects on natural recruitment. Evidence for this included the negative associations of CWP on spring spawning migration behaviour (Hypothesis 2), spawning conditions (Hypothesis 3), and early juvenile survival during summer (Hypothesis 4). Conversely, the effects of CWP on emigration and growth of larger juveniles and adults did not appear to be a major bottleneck for the population (Hypotheses 1 and 5). As such, we found that the population could be sustained by stocking and opportunistic water management (within the current operating climate) that reduced the frequency of CWP during summer (Hypothesis 6).
Using multiple lines of evidence to assess the impacts of CWP
Our results show how key population processes of a threatened freshwater fish are influenced by CWP, how this is likely to influence population dynamics and how we can use these insights to inform specific conservation actions and outcomes. For those population processes that contribute to recruitment, we demonstrated how CWP impacts spawning migration behaviour and YCS. Natural recruitment occurred in just one of the study years (our opportunistic sampling to test management outcomes), thereby supporting our hypothesis (Hypothesis 3) and previous work of (Todd et al., 2005) to show that water temperatures that fall below a critical survival threshold during the core egg and larval period will impact recruitment.
The results showing the influence of CWP on spawning migration behaviour and juvenile survival (Hypotheses 2 and 4) are novel and have not previously been investigated. The greatest number of juvenile fish captured and the subsequent strong year-classes of Murray cod occupying the Mitta Mitta River were associated with higher summer water temperatures, and typically not associated with large releases of cold water from Lake Dartmouth from January–March. Despite a relatively constant stocking rate since 2006, there was still high variability in the survival of fingerlings stocked in the Mitta Mitta River. Like its impacts on spawning and larval survival (Todd et al., 2005), our results indicate CWP also impacts juvenile Murray cod survival, with detection of a stocked cohort very unlikely if mean water temperatures were less than 18-20°C for the 30-day period following the release of fingerlings (during summer or early autumn). These results highlight those conditions influencing juvenile survival must also be considered in addition to those associated with spawning conditions (e.g. Tonkin et al., 2019) when assessing important drivers of recruitment strength for Murray cod.
An important first step in the recruitment process of Murray cod is the spawning migration of adult fish (Koehn et al., 2009). Our results provide evidence of unseasonal cold water releases altering the spawning migration behaviour of Murray cod (Hypothesis 2). Water temperature impacts the swimming performance of warm water fishes (e.g. Golden Perch, Macquaria ambigua; Lyon et al., 2008), with cold water and irrigation releases impacting the swimming capacities and energetics of Murray cod (Whiterod, 2013; Whiterod et al., 2018). We found that the likelihood of movement was positively associated with temperature, with the odds of a fish moving increasing by a factor of 4.3 when the temperature increases from 12°C to 23°C. With this, the timing of peak movement occurred in later spring and summer during the first three years of the study (when CWP was most pronounced). This timing is much later compared to the final two years of the study and previous research on migration and spawning which report larger movements of Murray cod (>10 km) typically undertaken in late-winter and spring (Koehn et al., 2009); (Tonkin et al., 2022), with spawning typically occurring during October and November (Humphries, 2005; Koehn and Harrington, 2006). Altered thermal regimes in rivers below large dams have been linked to changes in migration behaviour of fish in other parts of the world (e.g. Goniea et al., 2006; Thorstad et al., 2008; Tornabene et al., 2020; Moore et al., 2021).
Our analysis of direction of movement did not support our hypothesis of fish moving in a downstream direction and vacating the river reach in response to unseasonal spring and summer cold water releases (Hypothesis 1). This was despite spawning movement behaviour of Murray cod being altered by CWP. We found little evidence that temperature, discharge or time of year affected the direction of movement of Murray cod. Whiterod (2013) reports that Murray cod exhibits broad swimming capacity with low energy demands, therefore reflecting their evolution in some of the world’s most hydrologically and thermally variable rivers. Further to this, our results indicate that once established, Murray cod will remain in the reach even during successive years of elevated discharge and unseasonal water temperatures. As such, other management interventions such as riparian and instream habitat interventions that have been and are underway in the reach are likely to continue to provide benefits to these resident fish (e.g. Lyon et al., 2019; Tonkin et al., 2020).
While the study was able to specifically test the frequency and role of flows in influencing emigration of fish out of the study reach, we did not assess the role of immigration in governing the dynamics of Murray cod in the Mitta Mitta River. The contribution of immigrating fish as a key driver of population dynamics (e.g. Lyon et al., 2021) is likely to be minor given that our data showed no major changes in size structure of the population across years beyond that of small juvenile fish that were assigned as stocked or recruited in situ and very unlikely to have migrated large distances upstream into the study reach.
Finally, in support of our final hypothesis (Hypothesis 6), the output from the population modelling revealed the abundance of fish would very likely decline without any interventions over the 45-year model period. Even with minimal influence of CWP on retention, and growth and survival of larger fish, the conditions required for natural recruitment (spawning and juvenile survival) were still too infrequent to yield a stable population. Indeed, this emphasises the need for management interventions in this altered ecosystem, and relevance of reconciliation ecology for altered rivers globally (see below).
Using multiple lines of evidence to inform management actions for future conservation outcomes in a novel ecosystem
Our results clearly illustrate that due to the impact of cold water on the key processes governing recruitment, a population of a fish species with considerable cultural, recreational and conservation significance will not persist in the CWP-impacted reach of the Mitta Mitta River. Our results do, however, support several of our management hypotheses that indicate that interventions to bypass or overcome such recruitment bottlenecks can result in the recovery of the species. The otolith microchemistry results together with our population model predictions indicate that without ameliorating the CWP completely (e.g. Gray et al., 2019), stocking is required for this population to persist. Our model predicted a 145% increase of the expected average minimum female adult population size under the current stocking program (which commenced in 2006) and is likely to be a key factor in the apparent resurgence of Murray cod in the study reach since this time. Indeed, stocking has been shown to be of most benefit in systems where the conditions required for a self-sustaining population are not met (Secor et al., 2000; Forbes et al., 2016). Similarly, aquaculture-based restoration used to boost fish numbers and to overcome spawning habitat and recruitment deficiencies was critical in rebuilding and maintaining some stocks of Caspian Sea sturgeons (Secor et al., 2000).
It should also be noted that stocked fish were not always released in our study system under what we considered as the optimal temperatures. Hatchery-reared fish are faced with a variety of factors influencing their survival during the first year of life (Sutton and Ney, 2001). Some studies suggest most mortality occurs in the first few days following release due to predation, competition and biological metabolic thresholds such as temperature (Fielder, 1992; Blaxter, 2000; Svåsand et al., 2000; Brown and Day, 2002). Our results indicated that stocking the same number of fish with a more suitable thermal profile would significantly improve survival. Model predictions of the optimally stocked scenario indicated an increase in female adult population size from 145% (for the current stocking scenario) to 336% in comparison to the non-stocked scenario. Moreover, our scenario analyses showed that the same volume of water could still be released to meet human needs, but the timing of the release of both water and stocked fish would need to be slightly altered to improve the survival of juvenile fish.
We also showed that modifications to water transfers at critical times to reduce CWP impacts on the Murray cod population can also be extended to improve natural recruitment by minimising water transfers during the core spawning period. However, our modelling showed population decline continued even if managers could double the current natural recruitment frequency (to 4 in 15 years). Indeed, our opportunistic flow management and monitoring year provided an example of improvements to both natural recruitment and stocked fish survival that can be achieved through improved communications among researchers, fisheries and water management and operational agencies (Koehn et al., 2019).
A valid question remains regarding whether the reliance on stocking hatchery-reared fish and managing water releases to maintain a population in a severely altered system constitutes conservation management? We argue yes, specifically if viewed beyond an individual population in isolation, and instead considered in a reconciliation ecology and novel ecosystem setting within a landscape management framework (see Hobbs et al., 2014). Collectively, given that Murray cod is found across a spectrum of altered waterways in south-eastern Australia, managing this and other populations across the landscape will provide a greater range of intervention opportunities, use limited resources more effectively, and increase the chances of achieving management goals for the species. For example, Murray cod is an important recreational species, but such harvest pressure has been shown have a significant influence on its populations (Koehn and Todd, 2012). Simply maintaining populations across the landscape will absorb fishing pressure in the region that would otherwise be occurring at a few locations that contain self-sustaining remnant stocks of Murray cod. Also, if the hatchery and stocking practices used to maintain this population is undertaken with genetic diversity and variation considerations (e.g. Lyon et al., 2012), the population can be a source of broodfish for future captive breeding programs for the whole species.
Maintaining a spectrum of novel ecosystems across the landscape should also make species more resilient to larger perturbations such as climate change. Specifically, some populations occupying some rivers may come under increased threat from reduced or more frequent or severe variations in streamflow (e.g. Balcombe et al., 2011). Conversely, the opportunities for modifications to water management to achieve improvements to the processes supporting populations in novel systems such as our study reach may increase under climate change. As such, considering climate change impacts across the landscape and spectrum of novel ecosystems is essential to more effectively achieve outcomes for individual ecosystems and species across the landscape (e.g. Nelson et al., 2009; Hobbs et al., 2014). We therefore suggest an exploration of climate change impacts as well as different management approaches for the species across the landscape would be a valuable exercise. Specifically, an extension of our population modelling approach would help guide prioritisation of management actions across a spectrum of novel ecosystems with varying degrees of habitat modification and population losses under a changing climate.
Conclusions
Where ecosystems have been pushed beyond their historical range of variability, it may not be practical to maintain or restore them to past conditions. In such cases, new tools and approaches could help guide managers in deciding when and how to intervene. A review by (Radeloff et al., 2015) notes that novel ecosystems present opportunities to test ecological theories and challenge the predictive ability of ecological models and their validation. This review paper also emphasises that biodiversity conservation action is necessary along the continuum of novel ecosystems, including the development of flexible management approaches, and establishing experiments to test such. Our study has provided an example of both. It is a rare example where multiple lines of evidence underpinning a species’ biology, all of which contribute its conservation success, have been assessed in a novel ecosystem and management options have been proposed that have then been tested. Moreover, our approach not only provides evidence of impact and recovery pathways for Murray cod, but also gives managers and other stakeholders a clear choice on the trajectory of the population into the future. Our results add to the growing body of literature to show how species of conservation significance can be maintained in novel ecosystems and should be specifically considered in these contexts when conservation planning.
Data availability statement
The original contributions presented in the study are included in the article/Supplementary Material. Further inquiries can be directed to the corresponding author.
Ethics statement
The animal study was reviewed and approved by The Department of Environment, Land, Water and Environment Animal Ethics Committee. Animal ethics approval no. 14/04.
Author contributions
ZT: Conceptualization, field data collection, Investigation, Methodology, Project administration, Writing – original draft, Writing – review and editing. JK: Writing – original draft, Writing – review and editing. SC: Conceptualisation, administration, funding acquisition, Writing – original draft. GH: Field data collection, otolith processing, Writing – original draft. AH: Otolith processing, Writing – original draft. AK: Statistical analysis, Visualisation, Writing –original draft. JL: Conceptualisation, Writing –original draft. PM: Statistical analysis, Visualisation, Writing –original draft. CT: Statistical analysis, Visualisation, Writing – review and editing. All authors contributed to the article and approved the submitted version.
Funding
We thank the Murray Darling Basin Authority for funding and continued interest in this research. The production of this paper was supported by the Applied Aquatic Ecology writing retreat, through the Capability Fund of the Arthur Rylah Institute.
Acknowledgments
We thank the following individuals and groups for their support during the project: Peter Liepkalns (Goulburn Murray Water); Will Lucardie, Kris Kleeman, Damien Green, Hugo Bowman, Trevor Jacobs and Kerry Greenwood (MDBA); Michael Broughton and Andrew Briggs (North-east Catchment Management Authority); Anthony Wilson (Commonwealth Environmental Water Office); Angus Sutherland (DELWP); Kade Small (River Murray Works); Kris Pitman (Fishology Consulting). Andrew Boulton (University of New England) commented on earlier drafts. This work was carried out under the Victorian Fisheries Research Permit RP827, FFG Research Permit no. 10007273 and animal ethics approval no. 14/04.
Conflict of interest
The authors declare that the research was conducted in the absence of any commercial or financial relationships that could be construed as a potential conflict of interest.
The reviewer IS declared a past collaboration with the authors ZT, JK, JL, and CT to the handling editor.
Publisher’s note
All claims expressed in this article are solely those of the authors and do not necessarily represent those of their affiliated organizations, or those of the publisher, the editors and the reviewers. Any product that may be evaluated in this article, or claim that may be made by its manufacturer, is not guaranteed or endorsed by the publisher.
Supplementary material
The Supplementary Material for this article can be found online at: https://www.frontiersin.org/articles/10.3389/fcosc.2023.1103256/full#supplementary-material
References
Akçakaya H. R. (1991). A method for simulating demographic stochasticity. Ecol. Model. 54 (1-2), 133–136. doi: 10.1016/0304-3800(91)90103-8
Anderson J., Morison A., Ray D. (1992). Age and growth of Murray cod, Maccullochella peelii (Perciformes: Percichthyidae), in the lower Murray-darling basin, Australia, from thin-sectioned otoliths. Mar. Freshw. Res. 43 (5), 983–1013. doi: 10.1071/MF9920983
Balcombe S. R., Sheldon F., Capon S. J., Bond N. R., Hadwen W. L., Marsh N., et al. (2011). Climate-change threats to native fish in degraded rivers and floodplains of the Murray–darling basin. Mar. Freshw. Res. 62 (9), 1099–1114. doi: 10.1071/MF11059
Baumgartner L. J., Reynoldson N., Gilligan D. M. (2006). Mortality of larval Murray cod (Maccullochella peelii peelii) and golden perch (Macquaria ambigua) associated with passage through two types of low-head weirs. Mar. Freshw. Res. 57 (2), 187–191. doi: 10.1071/MF05098
Blaxter J. H. S. (2000). The enhancement of marine fish stocks. Adv. Mar. Biology Acad. Press 38, 1–54. doi: 10.1016/S0065-2881(00)38002-6
Brown C., Day R. L. (2002). The future of stock enhancements: Lessons for hatchery practice from conservation biology. Fish Fisheries 3 (2), 79–94. doi: 10.1046/j.1467-2979.2002.00077.x
Bunn S. E., Arthington A. H. (2002). Basic principles and ecological consequences of altered flow regimes for aquatic biodiversity. Environ. Manage. 30 (4), 492–507. doi: 10.1007/s00267-002-2737-0
Burnham K. P., Anderson D. R. (2010). Model selection and multi-model inference: A practical information-theoretic approach (Second) (New York, New York: Springer).
Campana S. E., Thorrold S. R. (2001). Otoliths, increments, and elements: keys to a comprehensive understanding of fish populations?". Can. J. Fisheries Aquat. Sci. 58 (1), 30–38. doi: 10.1139/f00-177
Clarkson R. W., Childs M. R. (2000). Temperature effects of hypolimnial-release dams on early life stages of Colorado river basin big-river fishes. Copeia 2000 (2), 402–412. doi: 10.1643/0045-8511(2000)000[0402:TEOHRD]2.0.CO;2
Crook D. A., O’Mahony D. J., Gillanders B. M., Munro A. R., Sanger A. C., Thurstan S., et al. (2016). Contribution of stocked fish to riverine populations of golden perch (Macquaria ambigua) in the Murray–darling basin, australia. Mar. Freshw. Res. 67 (10), 1401–1409. doi: 10.1071/MF15037
Dudgeon D., Arthington A. H., Gessner M. O., Kawabata Z.-I., Knowler D. J., Lévêque C., et al. (2005). Freshwater biodiversity: Importance, threats, status and conservation challenges. Biol. Rev. 81 (2), 163–182. doi: 10.1017/S1464793105006950
Dugan P. J., Barlow C., Agostinho A. A., Baran E., Cada G. F., Chen D., et al. (2010). Fish migration, dams, and loss of ecosystem services in the Mekong basin. AMBIO 39 (4), 344–348. doi: 10.1007/s13280-010-0036-1
Ebsary R. (1990). Effect of Dartmouth Reservoir on stream temperatures in the Mitta Mitta River. (Canberra: Murray-Darling Basin Commission).
Ernst, Young (2009). Economic study of recreational fishing in Victoria Murray cod assessment (Melbourne: Ernst & Young).
Fielder D. G. (1992). Evaluation of stocking walleye fry and fingerlings and factors affecting their success in lower lake oahe, south dakota. North Am. J. Fisheries Manage. 12 (2), 336–345. doi: 10.1577/1548-8675(1992)012<0336:EOSWFA>2.3.CO;2
Forbes J., Watts R. J., Robinson W. A., Baumgartner L. J., McGuffie P., Cameron L. M., et al. (2016). Assessment of stocking effectiveness for Murray cod (Maccullochella peelii) and golden perch (Macquaria ambigua) in rivers and impoundments of south-eastern australia. Mar. Freshw. Res. 67 (10), 1410–1419. doi: 10.1071/MF15230
Goniea T. M., Keefer M. L., Bjornn T. C., Peery C. A., Bennett D. H., Stuehrenberg L. C. (2006). Behavioral thermoregulation and slowed migration by adult fall Chinook salmon in response to high Columbia river water temperatures. Trans. Am. Fisheries Soc. 135 (2), 408–419. doi: 10.1577/T04-113.1
Gray R., Jones H. A., Hitchcock J. N., Hardwick L., Pepper D., Lugg A., et al. (2019). Mitigation of cold-water thermal pollution downstream of a large dam with the use of a novel thermal curtain. River Res. Appl. 35 (7), 855–866. doi: 10.1002/rra.3453
Grill G., Lehner B., Thieme M., Geenen B., Tickner D., Antonelli F., et al. (2019). Mapping the world’s free-flowing rivers. Nature 569 (7755), 215–221. doi: 10.1038/s41586-019-1111-9
Henry G. W., Lyle J. M. (2003). The national recreational and indigenous fishing survey. final report (Canberra: Australian Government Department of Agriculture, Fisheries and Forestry).
Hobbs R. J., Arico S., Aronson J., Baron J. S., Bridgewater P., Cramer V. A., et al. (2006). Novel ecosystems: theoretical and management aspects of the new ecological world order. Global Ecol. Biogeography 15 (1), 1–7. doi: 10.1111/j.1466-822X.2006.00212.x
Hobbs R. J., Higgs E., Hall C. M., Bridgewater P., Chapin F. S. III, Ellis E. C., et al. (2014). Managing the whole landscape: Historical, hybrid, and novel ecosystems. Front. Ecol. Environ. 12 (10), 557–564. doi: 10.1890/130300
Humphries P. (2005). Spawning time and early life history of Murray cod, Maccullochella peelii peelii (Mitchell) in an Australian river. Environ. Biol. Fishes 72, 392–407. doi: 10.1007/s10641-004-2596-z
Kaemingk M. A., Graeb B. D., Willis D. W. (2014). Temperature, hatch date, and prey availability influence age-0 yellow perch growth and survival. Trans. Am. Fisheries Soc. 143 (4), 845–855. doi: 10.1080/00028487.2014.886622
Koehn J. D. (2009). Multi-scale habitat selection by Murray cod Maccullochella peelii peelii in two lowland rivers. J. Fish Biol. 75 (1), 113–129. doi: 10.1111/j.1095-8649.2009.02270.x
Koehn J. D., Balcombe S. R., Zampatti B. P. (2019). Fish and flow management in the Murray–darling basin: Directions for research. Ecol. Manage. Restor. 20 (2), 142–150. doi: 10.1111/emr.12358
Koehn J. D., Doeg T. J., Harrington D. J., Milledge G. A. (1995). The effects of Dartmouth dam on the aquatic fauna of the mitta mitta river (Arthur Rylah Institute for Environmental Research, Victoria: Report to the Murray-Darling Basin Commission).
Koehn J. D., Harrington D. J. (2005). Collection and distribution of the early life stages of Murray cod (Maccullochella peelii peelii) in a regulated river. Aust. J. Zoology 53, 137–144. doi: 10.1071/ZO04086
Koehn J. D., Harrington D. J. (2006). Environmental conditions and timing for the spawning of Murray cod (Maccullochella peelii peelii) and the endangered trout cod (M. macquariensis) in southeastern Australian rivers. River Res. Appl. 22 (3), 327–342. doi: 10.1002/rra.897
Koehn J. D., Lintermans M. (2012). A strategy to rehabilitate fishes of the Murray-darling basin, south-eastern australia. Endanger. Species Res. 16 (2), 165–181. doi: 10.3354/esr00398
Koehn J. D., McKenzie J. A., O’Mahony D. J., Nicol S. J., O’Connor J. P., O’Connor W. G. (2009). Movements of Murray cod (Maccullochella peelii peelii) in a large Australian lowland river. Ecol. Freshw. Fish 18 (4), 594–602. doi: 10.1111/j.1600-0633.2009.00375.x
Koehn J. D., Raymond S. M., Stuart I., Todd C. R., Balcombe S. R., Zampatti B. P., et al. (2020). A compendium of ecological knowledge for restoration of freshwater fishes in australia’s Murray–darling basin. Mar. Freshw. Res. 71 (11), 1391–1463. doi: 10.1071/MF20127
Koehn J. D., Todd C. R. (2012). Balancing conservation and recreational fishery objectives for a threatened fish species, the Murray cod, Maccullochella peelii.". Fisheries Manage. Ecol. 19 (5), 410–425. doi: 10.1111/j.1365-2400.2012.00856.x
Koenker R. (2017) Quantile regression [R package version 5.33]. Available at: www.CRAN.R-project.org/package=quantreg.
Lake J. (1967). Rearing experiments with five species of Australian freshwater fishes. i. inducement to spawning. Mar. Freshw. Res. 18 (2), 137–154. doi: 10.1071/MF9670137
Lehner B., Liermann C. R., Revenga C., Vörösmarty C., Fekete B., Crouzet P., et al. (2011). High-resolution mapping of the world's reservoirs and dams for sustainable river-flow management. Front. Ecol. Environ. 9 (9), 494–502. doi: 10.1890/100125
Lieschke J. A., Dodd L., Stoessel D., Cable A., Raadik T., Kitchinghan A., et al. (2013). Assessment of the health of Victorian river basins: An analysis of sustainable rivers audit and southern basins project fish theme data. part b (Department of Sustainability and Environment, Heidelberg, Victoria: Technical Report prepared for the Murray Darling Basin Authority).
Lintermans M. (2007). Fishes of the Murray-darling basin: An introductory guide (Murray-Darling Basin Commission: Canberra).
Lucas M., Baras E. (2008). Migration of freshwater fishes. Blackwell Science. doi: 10.1002/9780470999653
Lugg A., Copeland C. (2014). Review of cold water pollution in the Murray–darling basin and the impacts on fish communities. Ecol. Manage. Restor. 15 (1), 71–79. doi: 10.1111/emr.12074
Lyon J. P., Bird T. J., Kearns J., Nicol S., Tonkin Z., Todd C. R., et al. (2019). Increased population size of fish in a lowland river following restoration of structural habitat. Ecol. Appl. 29 (4), e01882. doi: 10.1002/eap.1882
Lyon J. P., Bird T., Tonkin Z., Raymond S., Sharley J., Hale R. (2021). Does life history mediate discharge as a driver of multi-decadal changes in populations of freshwater fish?". Ecol. Appl. 31 (8), e02430. doi: 10.1002/eap.2430
Lyon J., Ryan T., Scroggie M. (2008). Effects of temperature on the fast-start swimming performance of an Australian freshwater fish. Ecol. Freshw. Fish 17 (1), 184–188. doi: 10.1111/j.1600-0633.2007.00244.x
Lyon J. P., Todd C., Nicol S. J., MacDonald A., Stoessel D., Ingram B. A., et al. (2012). Reintroduction success of threatened Australian trout cod (Maccullochella macquariensis) based on growth and reproduction. Mar. Freshw. Res. 63 (7), 598. doi: 10.1071/MF12034
Martinez P. J., Chart T. E., Trammell M. A., Wullschleger J. G., Bergersen E. P. (1994). Fish species composition before and after construction of a main stem reservoir on the white river, colorado. Environ. Biol. Fishes 40 (3), 227–239. doi: 10.1007/BF00002509
Michie L. E., Hitchcock J. N., Thiem J. D., Boys C. A., Mitrovic S. M. (2020). The effect of varied dam release mechanisms and storage volume on downstream river thermal regimes. Limnologica 125760. doi: 10.1016/j.limno.2020.125760
Moore M. J., Paukert C. P., Brooke B. L., Moore T. L. (2021). Lake sturgeon seasonal movements in regulated and unregulated Missouri river tributaries. Ecohydrology, 15 (1), e2362. doi: 10.1002/eco.2362
Nelson E., Mendoza G., Regetz J., Polasky S., Tallis H., Cameron D., et al. (2009). Modeling multiple ecosystem services, biodiversity conservation, commodity production, and tradeoffs at landscape scales. Front. Ecol. Environ. 7 (1), 4–11. doi: 10.1890/080023
Parisi M. A., Cramp R. L., Gordos M. A., Franklin C. E. (2020). Can the impacts of cold-water pollution on fish be mitigated by thermal plasticity?". Conserv. Physiol. 8 (1). doi: 10.1093/conphys/coaa005
Poff N. L., Zimmerman J. K. H. (2010). Ecological responses to altered flow regimes: a literature review to inform the science and management of environmental flows. Freshw. Biol. 55 (1), 194–205. doi: 10.1111/j.1365-2427.2009.02272.x
Postel S., Richter B. (2012). Rivers for life: managing water for people and nature (Washington, D. C.: Island Press).
R Core Team. 2021. R: A Language and Environment for StatisticalComputing. Vienna, Austria: R Foundation for Statistical Computing. https://www.R-project.org/.
Radeloff V. C., Williams J. W., Bateman B. L., Burke K. D., Carter S. K., Childress E. S., et al. (2015). The rise of novelty in ecosystems. Ecol. Appl. 25 (8), 2051–2068. doi: 10.1890/14-1781.1
Robertson A. I., Bacon P., Heagney G. (2001). The responses of floodplain primary production to flood frequency and timing. J. Appl. Ecol. 38 (1), 126–136. doi: 10.1046/j.1365-2664.2001.00568.x
Rosenzweig M. L. (2003). Reconciliation ecology and the future of species diversity. Oryx 37 (2), 194–205. doi: 10.1017/S0030605303000371
Rowland S. J. (1998). Aspects of the reproductive biology of Murray cod, Maccullochella peelii peelii.". Proc. - Linn. Soc. New South Wales 120, 147–162.
Rowland S. J. (2005). “Overview of the history, fishery, biology and aquaculture of Murray cod (Maccullochella peelii peelii),” in Management of Murray cod in the Murray-darling basin: Statement, reccomendatioons and supporting papers. proceedings of a workshop held in Canberra ACT, 3-4 June 2004. Eds. Lintermans M., Phillips B. (Canberra, Australia: Murray-Darling basin Commision).
Ryan T., Lennie R., Lyon J. (2003). Thermal rehabilitation of the southern Murray-darling basin: Final report to agriculture, forestry, fisheries Australia MD 2001 fish rehab program. Arthur Rylah Institute Environ. Research Victorian Department Natural Resour. Environment. 82 pp.
Salinger D. H., Anderson J. J. (2006). Effects of water temperature and flow on adult salmon migration swim speed and delay. Trans. Am. Fisheries Soc. 135 (1), 188–199. doi: 10.1577/T04-181.1
Secor D. H., Arefjev V., Nikolaev A., Sharov A. (2000). Restoration of sturgeons: lessons from the Caspian Sea sturgeon ranching programme. Fish Fisheries 1 (3), 215–230. doi: 10.1046/j.1467-2979.2000.00021.x
Sutton T. M., Ney J. J. (2001). Size-dependent mechanisms influencing first-year growth and winter survival of stocked striped bass in a Virginia mainstream reservoir. Trans. Am. Fisheries Soc. 130 (1), 1–17. doi: 10.1577/1548-8659(2001)130<0001:SDMIFY>2.0.CO;2
Svåsand T., Kristiansen T. S., Pedersen T., Salvanes A. G. V., Engelsen R., Nævdal G., et al. (2000). The enhancement of cod stocks. Fish Fisheries 1 (2), 173–205. doi: 10.1046/j.1467-2979.2000.00017.x
Thiem J. D., Baumgartner L. J., Fanson B., Sadekov A., Tonkin Z., Zampatti B. P. (2022). Contrasting natal origin and movement history informs recovery pathways for three lowland river species following a mass fish kill. Mar. Freshw. Res. 73 (2), 237–246. doi: 10.1071/MF20349
Thiem J. D., Wooden I. J., Baumgartner L. J., Butler G. L., Forbes J. P., Conallin J. (2017). Recovery from a fish kill in a semi-arid Australian river: Can stocking augment natural recruitment processes?". Austral. Ecol. 42 (2), 218–226. doi: 10.1111/aec.12424
Thorstad E. B., Økland F., Aarestrup K., Heggberget T. G. (2008). Factors affecting the within-river spawning migration of Atlantic salmon, with emphasis on human impacts. Rev. Fish Biol. Fisheries 18 (4), 345–371. doi: 10.1007/s11160-007-9076-4
Todd C. R., Koehn J. D. (2008). Murray Cod modelling to address key management actions: Final report for project MD745 (Department of Sustainability and Environment: Heidelberg: Arthur Rylah Institute for Environmental Research Progress Report to the Murray Darling Basin Commission).
Todd C. R., Ng M. P. (2001). Generating unbiased correlated random survival rates for stochastic population models. Ecol. Model. 144 (1), 1–11. doi: 10.1016/S0304-3800(01)00343-X
Todd C. R., Ryan T., Nicol S. J., Bearlin A. R. (2005). The impact of cold water releases on the critical period of post-spawning survival and its implications for Murray cod (Maccullochella peelii peelii): A case study of the mitta mitta river, southeastern australia. River Res. Appl. 21 (9), 1035–1052. doi: 10.1002/rra.873
Tonkin Z., Kitchingman A., Fanson B., Lyon J., Ayres R., Sharley J., et al. (2020). Quantifying links between instream woody habitat and freshwater fish species in south-eastern Australia to inform waterway restoration. Aquat. Conservation: Mar. Freshw. Ecosyst. 30 (7), 1385–1396. doi: 10.1002/aqc.3352
Tonkin Z., Moloney P., Lyon J., Kitchingman A., O'Mahony J., Raymond S., et al. (2022). Movement behavior of a threatened native fish informs flow management in a modified floodplain river system. Ecosphere 13 (1), e3916. doi: 10.1002/ecs2.3916
Tonkin Z., Stuart I., Kitchingman A., Thiem J. D., Zampatti B., Hackett G., et al. (2019). Hydrology and water temperature influence recruitment dynamics of the threatened silver perch bidyanus bidyanus in a regulated lowland river. Mar. Freshw. Res. 70, 1333–1344. doi: 10.1071/MF18299
Tornabene B. J., Smith T. W., Tews A. E., Beattie R. P., Gardner W. M., Eby L. A. (2020). Trends in river discharge and water temperature cue spawning movements of blue sucker, cycleptus elongatus, in an impounded great plains river. Copeia 108 (1), 151–162. doi: 10.1643/CI-19-256
VanDeHey J., Kaemingk M., Jansen A., Graeb B., Dembkowski D., Willis D. (2013). Effects of simulated cold fronts on the survival and behaviour of yellow perch perca flavescens yolk-sac fry. J. Appl. Ichthyol. 29 (2), 364–367. doi: 10.1111/jai.12115
Vitousek P. M., Mooney H. A., Lubchenco J., Melillo J. M. (1997). Human domination of earth's ecosystems. Science 277 (5325), 494–499. doi: 10.1126/science.277.5325.494
Vörösmarty C. J., McIntyre P. B., Gessner M. O., Dudgeon D., Prusevich A., Green P., et al. (2010). Global threats to human water security and river biodiversity. Nature 467 (7315), 555–561. doi: 10.1038/nature09440
Whiterod N. S. (2013). The swimming capacity of juvenile Murray cod (Maccullochella peelii): An ambush predator endemic to the Murray-darling basin, australia. Ecol. Freshw. Fish 22 (1), 117–126. doi: 10.1111/eff.12009
Whiterod N. S., Meredith S. N., Humphries P., Sherman B. S., Koehn J. D., Watts R. J., et al. (2018). Flow alteration and thermal pollution depress modelled growth rates of an iconic riverine fish, the Murray cod maccullochella peelii. Ecol. Freshw. Fish 27 (3), 686–698. doi: 10.1111/eff.12384
Wood S., Scheipl F. (2014) gamm4: Generalized additive mixed models using mgcv and lme4. Available at: https://CRAN.R-project.org/package=gamm4.
Woodhead J., Swearer S., Hergt J., Maas R. (2005). In situ Sr-isotope analysis of carbonates by LA-MC-ICP-MS: Interference corrections, high spatial resolution and an example from otolith studies. J. Anal. At. Spectrom. 20 (1), 22–27. doi: 10.1039/b412730g
Keywords: novel ecosystem, reconciliation ecology, conservation, thermal pollution, population modelling, stocking, Murray cod, Murray-Darling Basin
Citation: Tonkin Z, Koehn J, Commens S, Hackett G, Harris A, Kitchingman A, Lyon J, Moloney P, Todd C and Woodhead J (2023) Using multiple lines of evidence to assess recovery potential of a warm water fish population in a cold water impacted river. Front. Conserv. Sci. 4:1103256. doi: 10.3389/fcosc.2023.1103256
Received: 20 November 2022; Accepted: 14 February 2023;
Published: 01 March 2023.
Edited by:
Ronald R. Swaisgood, San Diego Zoo Wildlife Alliance, United StatesReviewed by:
Ivor Stuart, Charles Sturt University, AustraliaAdi Barocas, San Diego Zoo Global, United States
Copyright © 2023 Tonkin, Koehn, Commens, Hackett, Harris, Kitchingman, Lyon, Moloney, Todd and Woodhead. This is an open-access article distributed under the terms of the Creative Commons Attribution License (CC BY). The use, distribution or reproduction in other forums is permitted, provided the original author(s) and the copyright owner(s) are credited and that the original publication in this journal is cited, in accordance with accepted academic practice. No use, distribution or reproduction is permitted which does not comply with these terms.
*Correspondence: Zeb Tonkin, Zeb.Tonkin@delwp.vic.gov.au