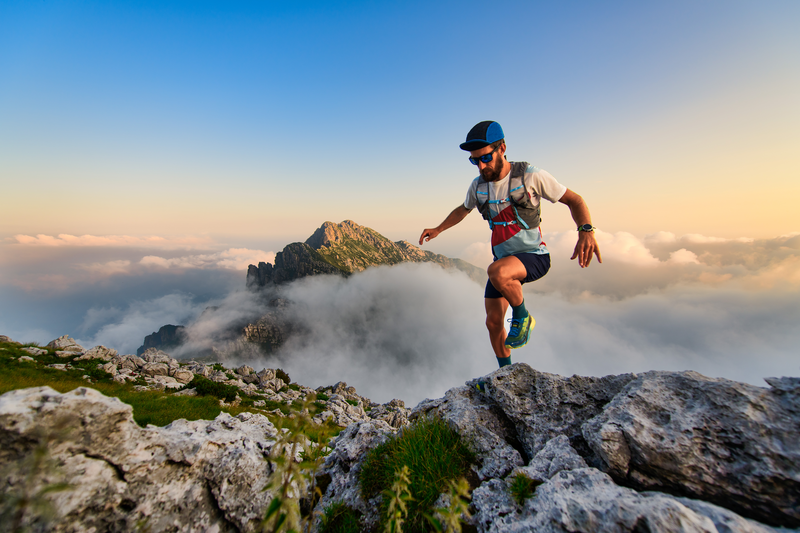
94% of researchers rate our articles as excellent or good
Learn more about the work of our research integrity team to safeguard the quality of each article we publish.
Find out more
ORIGINAL RESEARCH article
Front. Conserv. Sci. , 29 July 2022
Sec. Animal Conservation
Volume 3 - 2022 | https://doi.org/10.3389/fcosc.2022.943078
This article is part of the Research Topic Human Dimensions of Animal Translocations View all 11 articles
Translocations are commonly employed to mitigate human–carnivore conflict but rarely evaluated, resulting in conflicting reports of success, particularly for leopards (Panthera pardus). We evaluate the status of available leopard translocation data, the factors driving the intentional removal of leopards, and the potential causal factors associated with successful and failed translocation events. We obtained data on 60 leopard translocation events across five provinces in South Africa between 1994 and 2021. We considered a successful translocation outcome when (1) the animal was moved outside of its original home range, (2) the animal established a new home range away from the capture site, (3) no substantive livestock losses were linked to the translocated animal in the post-release monitoring period, and (4) the animal survived at least 6 months post-translocation. If mortality occurred due to factors that were equally likely to impact resident individuals and were unrelated to the translocation event (e.g., poaching), the event was not considered a failed effort. Most translocations were the result of human–carnivore conflict (HCC; 82%, n = 49), stressing the high prevalence of HCC and the importance of advocating preventative conflict mitigation efforts to conserve leopards. The leopards were moved distances from 2.5 to 196.3 km (63.3 ± 51.7km). Forty (67%) translocation events had unknown outcomes, indicating the limited data available on translocation outcomes. This also indicates the disparity in the objectives of translocations by various entities involved with translocations and suggests that monitoring be a prerequisite for future translocations. Twenty events offered reliable outcomes by means of post-event monitoring, with seven (12%) considered successful, with three (5%) as failures, and with four (7%) not moved beyond their original home ranges, while six (8%) ended in unrelated deaths. The failed events were attributed to inter/intra-specific competition, and one animal returned to its original home range after a translocation distance of 68 km. Translocation success was strongly explained by translocation distance. We found that damage-causing leopards were successfully translocated under specific conditions, and longer translocation distances increase success. Translocations are commonly employed but are still poorly monitored. We discuss basic standardized protocols to improve future leopard translocations (including pre- and post-monitoring) while advocating alternative non-lethal practices to reduce the prevalence of human–carnivore conflict.
Mitigating human–carnivore conflict (HCC) by balancing often-contradictory human interests and species conservation needs is key to conserving biodiversity (Inskip and Zimmermann, 2009; Seoraj-Pillai and Pillay, 2017; Pooley et al., 2021; Consorte-McCrea et al., 2022). Lethal interventions used to control predator species are largely ineffective at reducing livestock depredation and are increasingly considered inhumane (McManus et al., 2015; Treves et al., 2016; Moreira-Arce et al., 2018; Lorand et al., 2022). Furthermore, lethal interventions have been shown to negatively impact carnivore populations by disturbing their social structure through the loss of breeding individuals, promoting mesopredator release (Stahl et al., 2001; Teichman et al., 2016), or increasing infanticide (Steyaert et al., 2012; Teichman et al., 2016). To address these problems, alternative non-lethal tools have been tested. The employment of guarding animals, herdsmen, barrier methods (e.g., electric fences and protective livestock collars), and other deterrents (e.g., auditory, visual, and olfactory) in livestock husbandry significantly decreases livestock losses due to predation (Rust et al., 2013; McManus et al., 2015; Treves et al., 2016; Moreira-Arce et al., 2018; Khorozyan and Waltert, 2021; Lorand et al., 2022). Translocation is an additional HCC mitigation tool and is considered a humane alternative to killing damage-causing animals (DCA; i.e., habitual livestock killer or an animal that poses a threat to human safety), particularly for vulnerable species (Fontúrbel and Simonetti, 2011; Treves et al., 2016; Moreira-Arce et al., 2018; Berger-Tal et al., 2020). However, translocations can be controversial, with conflicting reports of success (Athreya et al., 2011; Fontúrbel and Simonetti, 2011; Berger-Tal et al., 2020; Lorand et al., 2022).
A variety of carnivore species have been successfully translocated, including DCA (Goodrich and Miquelle, 2005; Fontúrbel and Simonetti, 2011; Weise et al., 2015b; Bauder et al., 2021; Stenhouse et al., 2022)—for example, in Wisconsin, USA, 86% of black bear (Ursus americanus) translocations were considered successful when the animals had established home ranges, and no evidence of mortality was found after the translocation (Bauder et al., 2020). In gray wolves (Canis lupus), 80% of the translocated animals stayed at the release areas in northwestern USA (Bradley et al., 2005). In addition, translocations can help reinforce genetic diversity among populations where landscapes are fragmented and the connectivity of extant population genetics is low (Johnson et al., 2010; Tensen et al., 2019; Miller et al., 2020). Translocations have also been used to reintroduce species previously extirpated from their historical range (Clark et al., 2002; Mowry et al., 2015; Mueller et al., 2020). However, for large felids, translocation attempts are sometimes considered problematic, either because the animal returned to their capture site, died prematurely, or contributed to HCC (Athreya et al., 2011; Houser et al., 2011; Morapedi et al., 2021).
Apex carnivores, such as leopards (Panthera pardus), have large spatial requirements and can exist in diverse environments where they can occur at various densities depending on resource availability and HCC (Bailey, 1993; Hunter et al., 2013; Ripple et al., 2014; Devens et al., 2018; Devens et al., 2021). Their wide-range behavior increases the interface with anthropogenic landscapes, reducing their survival. Habitat loss and fragmentation, pray depletion, conflicts with livestock or game owners, edge effects, and poorly managed hunting practices contribute to the significant decline of leopard populations in Asia and Africa (Swanepoel et al., 2013; Jacobson et al., 2016; Swanepoel et al., 2016). With merely 25% of their historic distribution remaining (Jacobson et al., 2016), leopards are listed as vulnerable by the IUCN Red List (Stein et al., 2020). In South Africa, most leopard habitats exist outside of protected areas, where HCC is high (Swanepoel et al., 2013; McManus et al., 2021; McManus et al., 2022). The lack of connectivity between isolated, low-density populations of South Africa’s Eastern and Western Cape provinces contributes to reduced gene flow (McManus et al., 2014; Devens et al., 2018; Mann et al., 2020; Devens et al., 2021; Müller et al., 2022).
Although leopards are commonly translocated as a management tool to mitigate HCC or as a conservation strategy to restore genetic diversity in isolated populations (Mondal et al., 2013; Briers-Louw et al., 2019), the acrimony towards leopards in livestock production landscapes and legislative controls make leopard translocations controversial. As a result, many translocations have taken place in a clandestine manner, adding to the unreported nature of these interventions and the lack of monitoring to evaluate translocation outcomes. The divergent anecdotal reports and conflicting literature make translocations an important topic to better understand the potential as a mitigation tool to conflict and species management (Hayward et al., 2006; Weilenmann et al., 2010; Weise et al., 2015a; Power et al., 2021). Since most studies focused on local to regional extents (Hayward et al., 2006; Weilenmann et al., 2010; Power et al., 2021), with only one national (Weise et al., 2015a), we attempted to upscale to the whole of South Africa and considered the contribution of multiple agencies, whether private, non-government (NGO), or government. This study evaluated (1) the availability of leopard translocation data in South Africa, (2) the contexts and mechanisms driving the intentional removal of leopards, (3) three potential factors associated with successful and failed leopard translocation events, and (4) recommendations towards standardized pre- and post-translocation efforts to encourage an evidence-driven approach for future leopard translocations.
We defined a successful translocation event to include four criteria. First, the leopard displayed a home range stabilization behavior away from the site of capture (Weise et al., 2015a). Therefore, if the animal was moved and relocated within its original home range, it was considered a relocation (Power et al., 2021). Second, success was also evaluated on the survival of the individual (Weise et al., 2015a; Briers-Louw et al., 2019; Power et al., 2021). If mortality was a direct result of the translocation effort, such as intra- or inter-specific competition within 6 months post-translocation, it was considered a failed translocation. However, if mortality occurred after 6 months or was unrelated to the translocation effort (i.e., poaching and natural causes), it was considered a non-translocation-related death (unrelated death). This assumes that the individual is more vulnerable in the months following the translocation event. Finally, if habitual livestock losses were linked to the translocated individual within the first 6 months post-release, it was considered a failure. Where post-translocation monitoring through collaring failed early on, as unreliable technology of animal tracking does demonstrate on occasions, the outcome was listed as unknown.
We obtained two types of data for analyses: first, metadata from South African governmental departments, parastatals, NGOs, and private entities relating to any intentional movement of leopard in their jurisdiction or part of their efforts as far back as records were available and, second, we obtained post-monitoring GPS data from 13 translocation events from leopards that were captured, collared, and translocated.
The metadata included information on the individuals’ demographics, capture location, reason for capture, release site, type of release (soft or hard release), and if monitoring efforts pre- and post-release were employed. We included all translocation events when individuals were considered DCA (known or perceived) and rescue events (i.e., reported as non-target captures where landowners would otherwise kill the animal). We did not consider orphaned animals, confiscated animals (from illegal trade), or any captive-held sourced animals in this study, as the conditions regarding the translocation were different in that these cases involved animals from unknown locations and were placed in captivity for some period (Power et al., 2021).
Leopard management is governed by South Africa’s National Environmental Management: Biodiversity Act (Act 10 of 2004), Threatened or Protected Species Regulations, as well as provincial legislation. Currently, there are no overarching national or provincial policies on leopard management nor leopard translocations in South Africa. Each province is governed by a relevant statutory conservation body with varying approaches dealing with HCC incidences and the translocation of DCA incidents. In all events, the animals were translocated under the regulatory oversight and direction of the relevant statutory conservation agency in each province.
Leopards were captured using walk-in, fall-door cages specifically designed by local conservation authorities to capture leopards with least risk of injury to individuals. In some cases, animals were rescued from devices set due to HCC and poaching, including leg-hold traps (gin-traps) and wire snares. None of the animals suffered severe injuries in the cages; however, two rescued individuals (Table 1) suffered toe amputations due to gin-trap devices. Once an animal was captured, it was immobilized by a veterinarian using a drug combination of zoletol-medetomidine at a standard dosage (1 to 2 mg/kg) or ketamine (4–6 mg/kg) with xylazine (1 to 2 mg/kg). The animal was immobilized for approximately 60 min before the reversal drug was administered. During the immobilization, the animal was fitted with a tracking collar (African Wildlife Tracking Pretoria, South Africa; Hotgroup, Pretoria, South Africa; Tellus, Lindesberg, Sweden; or Vectronics-aerospace, Berlin, Germany; Table 1). The collars recorded location data between four and six hourly intervals. We used these tracks to evaluate home range stabilization. All statistical analysis were conducted in the R environment, version 4.1.2 (R Core Team, 2021), using the R Studio interface (R Studio 2021, Boston, MA, U.S.A.). We evaluated home range stabilization using the continuous-time movement modeling “ctmm” package (Calabrese et al., 2016; Fleming and Calabrese, 2016). We plotted semivariograms to establish if translocated leopards displayed home range stabilization behavior. Semivariograms plot the variance in GPS locations as a function of the time lag between locations (Noonan et al., 2019). Stabilization in a home range establishment was a factor of reaching the asymptotic smoothing of semi-variance. Finally, we tested if the outcome of the translocation (i.e., success or failure) was correlated to the distance the animals were moved, using a simple regression model. Prior to the analysis, we visually inspected the normality of the residuals and the homogeneity of the variance.
Table 1 Biological and technical details relevant to the 60 translocation events across South Africa.
We obtained information relating to the cost of translocation events from the first-hand capture and translocation events, where costs were known. Costs included veterinary, staff, travel, tracking collar, and satellite data transfer costs.
We obtained information on 60 translocation and relocation events that took place between April 1994 and December 2021 from five provinces in South Africa (Eastern Cape, n = 18; Limpopo, n = 10; Mpumalanga, n = 5; Northwest Province, n = 7; and Western Cape, n = 20; Table 1; Figure 1). Most original capture sites of leopards were located outside formal protected areas, except in five cases (n = 5) in Kruger National Park where animals were removed via cage traps and one (n = 1) instance where the animal was caught in a gin-trap along the boundary of the Greater Addo Elephant National Park (Table 1). All release sites occurred within protected areas (i.e., national park, provincial nature reserve, or eco-tourism-based private game reserve) based on habitat suitability for leopards (e.g., slope, terrain, vegetation type: Gavashelishvili and Lukarevskiy, 2008; McManus et al., 2022) and land uses considered to have a reduced likelihood of HCC.
Overall, the mean Euclidian distance leopards were moved was 63.3 ± 51.7 km (range, 2.5–196.3 km). In the Limpopo, Mpumalanga, and Western Cape provinces, leopards were moved similar distances on average (44.8, 46.9, and 50.2 km, respectively), while in the North West and Eastern Cape provinces, leopards were moved farthest on average (75.7 and 90.1 km, respectively).
Forty-five (75%) of the translocation events included adult leopards (19 female and 26 male), six (10%) of which were sub-adults (5 female and 1 male), and age category was unknown in nine cases (15%). Overall, 25 (42%) female leopards and 31 (52%) male leopards were translocated, while in four (7%) cases, the sex or age category of the animals was not reported.
Of the 60 events, 49 (82%) were reported to be associated with perceived or known DCA incidents. Of these, leopard killed game animals that made up natural prey in three instances but were reported as DCAs due to the hunting value of the game. In eight events (13%), the reason for the animal being moved was unknown. Of the 60 events, 36 (60%) events had no pre- or post-monitoring efforts. Overall, 40 (67%) cases resulted in unknown outcomes (collar failure, no information provided, and no/unsuccessful post-monitoring), seven (12%) were considered successful, three (5%) failed, and four (7%) were relocation events (short distance relocations: 5.4–18 km), resulting in these animals being released within their original home ranges—therefore, the individuals were not translocated away from the site of capture—and six (10%) ended in unrelated deaths (Table 1).
The probability of a successful translocation increased as a result of translocation distance (ß = 139; r² = 0.51, t = 3.2, p = 0.01), and failed outcomes were also strongly correlated to distance (ß = 62; t = 3.1, p = 0.02) (Figure 2).
Figure 2 Outcome of translocated leopards in relation to the distance moved, with the average distance and 95% confidence interval.
From the 22 leopards fitted with tracking collars (Table 1), location data was available for 13 of these events (3 female and 10 male, Table 2). This discrepancy is due to some collars being audio-tracking devices that do not store GPS data or, in some cases, GPS-capable collars that failed, or there were limitations in acquiring the GPS data from various entities.
Table 2 Details of post-release monitoring of 13 translocated leopards fitted with tracking collars.
Leopard identities L111 and L2344 were the same male moved on two unrelated occasions (18 and 80.5 km, respectively), and leopard L6849 and L6897 were the same male moved 68 and 196 km on two unrelated occasions, respectively (Table 2). These two individuals were moved due to consistent damage caused to livestock and would otherwise have been killed. All post-release monitored leopards were reported as DCAs. We analyzed each translocation event separately.
Leopard L111 was first moved a short distance (18 km) within its original home range (Figure 3) and continued to predate on livestock. L111 was later captured and re-collared as L2344 where it was translocated outside of its original home range (80.5 km) but was killed 16 days post-translocation via poaching and died prematurely in a gin-trap set along the boundary of a formally protected area (the Greater Addo Elephant National Park; Table 2). Similarly, LM14 and LM17 were translocated and died via poaching (wire snare and unrelated death) after 13 and 87 days post-translocation, respectively (Table 2). Leopard LM07 (moved 8 km) and L6773 (moved 5.4 km) were relocated within their respective original home range where LM07 survived and L6773 died of natural causes 148 days after the relocation event. Leopard L6849 was translocated outside of its original home range (68 km) but returned to its original home range approximately 5 weeks post-release (Figure 3) and continued to cause livestock damage, resulting in a failed translocation (Table 2). The collar of leopard L1038 malfunctioned (20 days post-translocation), resulting in an unknown outcome. L2996, L6897, and LF16 displayed home range stabilization (Table 2; Figure 3), and no livestock losses were associated post-release, resulting in successful translocation events. LM06 and LM08 were translocated outside of their original home ranges, and home range stabilization was reached, but both died via intraspecific competition at 86 and 188 days post-translocation, respectively. Home range stabilization occurred within 3 months for six individuals (Figure 3).
Figure 3 Variograms of translocated leopard home range stabilization regimes (A–L). The gray shading indicates pointwise 95% CI, and the red shading represents fitted model 95% CI to indicate home range stabilization. Leopards A, B and H home ranges stabilized; however, these were considered relocated individuals moved within their home ranges. Leopards C, E and J indicates non-stabilization. Leopards D, F, G, I, K, and L indicate stabilization at different locations to their original home ranges.
We used information on 7 translocation events where specific costs were known to infer the costs of translocation events (see also Weise et al., 2014; Power et al., 2021). For each event, tracking collars used to monitor leopards cost 3,400 USD, veterinarian cost varied between 300 and 1,000 USD, travel and accommodation cost 500 USD, and satellite data cost 400 USD per collar. On average, each individual cost 4,700 USD to be translocated.
Translocation is a commonly employed management tool to mitigate HCC (Fontúrbel and Simonetti, 2011). While increased scientific evaluations of translocations have been undertaken in the African context (see Weise et al., 2015a; Briers-Louw et al., 2019; Power et al., 2021), sampling remains low, especially for wild felids. This continues to limit our understanding of translocations. In efforts to contribute to these samples and better understand the nuances of translocation as a management tool, we assessed data from 60 translocation events obtained from various conservation entities from five South African provinces.
While not all reported DCAs were confirmed to be occasional or habitual culprits, most translocation events (82%) were attributed to DCAs because of known or perceived livestock depredation. Conflicting reports of successfully translocating DCAs exist, with some studies suggesting that damage-causing leopards are not suitable to be translocated because the animals returned to their original site of capture and continued to cause damage around the release site (Weilenmann et al., 2010; Athreya et al., 2011; Power et al., 2021), while other studies showed success (Hayward et al., 2006; Weise et al., 2015a; Briers-Louw et al., 2019). This can be related to variations on how success is gauged (Weilenmann et al., 2010; Briers-Louw et al., 2019; Power et al., 2021). Our definition was similar to the one of Weise et al. (2015a), where a leopard (1) is moved outside of its original home range, (2) can establish a home range anywhere away from the original home range, (3) does not contribute substantively to livestock depredation, and (4) survives at least 6 months post-translocation. The seven translocation events considered successes in our study were DCAs, which support findings that DCA can be successfully translocated under specific conditions (Hayward et al., 2006; Weise et al., 2015a; Briers-Louw et al., 2019). Of the relocated animals (moved within their home ranges), two continued to contribute to livestock losses and were ultimately recaptured and translocated to farther distances. We found that translocation success was significantly correlated to the distance the animals were moved. This supports results from other studies where translocations were successful when animals were translocated to farther distances, including leopards (Weise et al., 2015a; Briers-Louw et al., 2019), foxes (Vulpes arabica and V. ruppelli sabea: Lenain and Warrington, 2001), gray wolves (Bradley et al., 2005), black bears (Landriault et al., 2009; Bauder et al., 2020), and grizzly bears (Ursus arctos: Milligan et al., 2018). We report on a small sample size; however, the failed events (n = 3) were statistically inversely correlated to the distance moved, and relocating wide-range species over short distances often resulted in the animal being replaced within its original range (n = 4). One failed event included a male leopard that re-homed 5 weeks after a 68-km translocation distance. Therefore, the wide-range nature of leopards contributes to the outcome of translocations. This is relevant to the home range sizes of leopards which vary greatly across various bio-regions, depending on pray resources, land cover types, topography, and social structures, with some leopards requiring small home ranges of <20 km², while others require >1,000 km² (e.g., le Roux and Skinner, 1989; Bailey, 1993; Mondal et al., 2013; Weise et al., 2015a; Devens et al., 2018; Müller et al., 2022). As such, regional variations of leopard spatial requirements should be considered when planning an appropriate translocation distance. Some authors have recommended moving leopards at least 200 km from the capture site to prevent capture site fidelity and homing instinct (Weise et al., 2015a; Briers-Louw et al., 2019). It would seem prudent to lean towards developing translocation distances of greater than four diameters of the regionally known localized leopard home ranges—for instance, based on the leopard home range size in the southern provinces of South Africa (Devens et al., 2018; Müller et al., 2022), our findings support the consideration of translocation distances between 200 and 400 km in these provinces.
The definition of translocation success or failure rarely include the impact on source and receiving populations (but see Weise et al., 2015a). Translocations could be a detriment to leopard populations if poorly implemented, such as releasing individuals into a high-density area (e.g., Hamilton, 1981; Athreya et al., 2011; Lorand et al., 2022). Weise et al. (2015a) mitigated these challenges and demonstrated success in the repeated release of individuals into the same geographic region by releasing individuals into a receiving population with low to moderate densities of conspecifics and by ensuring at least 6 months of inter-release period for individuals to assimilate the additional leopards. Further considerations should include the asymmetrical dispersal behavior of leopards, whereby male individuals are primarily the dispersing sex, moving vast distances compared to female leopards (Fattebert et al., 2013; Elbroch et al., 2016; Sunquist and Sunquist, 2017; Müller et al., 2022). Female individuals are generally philopatric and influence the local structure of populations and their dynamics (Elbroch et al., 2016; McManus et al., 2021). The removal of female leopards from a system could cause far-reaching changes in the structure of the population (Elbroch et al., 2016; McManus et al., 2021). Almost half (42%) of the reported translocations were those of female leopards, further emphasizing the need to reduce HCC. Furthermore, the removal of established male leopards from a stable population also has disruptive and potentially negative impacts on leopard populations. Adult male leopards occupy larger home ranges which typically encompass multiple female ones and often a combination of land use types (Balme et al., 2009; Devens et al., 2018; McManus et al., 2021). Territorial and dominant male leopards may keep the younger ones at bay, and the removal of these territorial male individuals from their home range could (1) exacerbate livestock depredations as multiple younger male leopards could compete for the same range (previously occupied by a dominant male; Rabinowitz, 1986), (2) elicit infanticide (Balme et al., 2009; Balme and Hunter, 2013; Fattebert et al., 2015), potentially resulting in population declines (Balme et al., 2009), and (3) lead to inbreeding when off-take is dramatic (Naude et al., 2020). Therefore, sex-related behavioral differences should be considered when translocating animals and particular attention should be made to avoid translocating leopards into areas with saturated leopard populations (Athreya et al., 2011; Weise et al., 2015a).
Leopards in South Africa are part of a single genetic species, Panthera pardus pardus (Jacobson et al., 2016), and their wide-range behavior should allow them to interchange genes over vast areas. However, modified landscapes and human-caused mortality impede connectivity among separated populations (McRae et al., 2005; Dutta et al., 2013; Roques et al., 2016; McManus et al., 2022). Such genetic restrictions have been observed in the Eastern and Western Cape provinces where three sub-populations have been identified as with moderate to low gene flow among them (McManus et al., 2014). Low population densities, low gene flow, increased isolation due to loss of habitat, and human-caused mortality are all major conservation concerns that contribute to inbreeding and local population extinctions (Keyghobadi, 2007; Dutta et al., 2013; Ripple et al., 2014; Swanepoel et al., 2016; Lino et al., 2019). The translocation of leopards that would conventionally be culled in DCA scenarios can reduce these threats and have been used to successfully re-establish locally extinct wildlife populations (Clark et al., 2002; Mowry et al., 2015; Briers-Louw et al., 2019; Mueller et al., 2020). This would facilitate gene flow among isolated, low-density, and declining populations, making it an important conservation tool (Johnson et al., 2010; Houser et al., 2011; Tensen et al., 2019; Miller et al., 2020; Morapedi et al., 2021). However, for translocations to contribute constructively to conservation, increased monitoring efforts and improved implementation are key.
Unfortunately, the majority (67%) of translocation events reported in our study had unknown outcomes, largely due to the lack of monitoring efforts—a clear deficiency in the employment of this management intervention. The limitation of evaluating translocations therefore continues, contributing to the challenge of effectively employing these tools in practice (Mills, 1991; Grimbeek, 1992; Athreya et al., 2011; but see Weise et al., 2015a; Power et al., 2021). We echo the need to promote transparency and a structured monitoring approach both before and after the translocation events, of the source and potential resident populations at the release site (Mills, 1991; Hayward et al., 2006; Briers-Louw et al., 2019). However, this may not always be possible for one organization to achieve—for example, the financial cost of translocation can be high (Weise et al., 2014; Power et al., 2021), and resources into monitoring translocated animals might fall outside the scope and budget of conservation bodies. Furthermore, the objectives among stakeholders involved in large carnivore management are often divergent. This misalignment of objectives is an important factor impeding the knowledge of leopard translocation outcomes as was observed in the current study with the high number of unknown outcomes. Therefore, reporting on translocations and promoting transparency and collaborations among stakeholders can improve the monitoring and evaluation efforts of translocations.
We suggest the development of basic standardized operational translocation protocols (see the Box 1) as a starting point to address this challenge. First, the use of non-lethal techniques mitigates HCC by effectively reducing livestock losses, increasing tolerance and co-existence (McManus et al., 2015; Treves et al., 2016; Khorozyan and Waltert, 2021; Boronyak et al., 2022), and should always be advocated for and employed prior to any translocations. Increased tolerance, awareness, and building trust with the local community are key to mitigating HCC (Bennett et al., 2017; Boronyak et al., 2022; Consorte-McCrea et al., 2022). Second, to avoid translocating non-culprit animals, the specific individual should be identified and confirmed to be a DCA (i.e., via a camera trap survey, carcass evaluations, and/or GPS tracking). This could be determined using a standard operational guideline on best practice at local governmental scales. Finally, pre- and post-translocation monitoring efforts, including identifying and surveying suitable release sites, and ensuring that materials are available to monitor the animal post-release are required to better understand the drivers of failed or successful translocations (IUCN/SSC, 2013). The use of GPS collars, camera traps, and genetic analyses are required to monitor aspects such as home range stabilization, record mating behavior, population genetic dynamics, and survival (Weise et al., 2015a; Briers-Louw et al., 2019; Power et al., 2021). We found that GPS-tagged leopards displayed home range stabilization within 3 months after a translocation event. Monitoring should take place for as long as possible post-release, recognizing financial, technical, and practical constraints, but at least for a 6-month period to improve the evaluation and success of translocations (Armstrong and Seddon, 2008; Weise et al., 2015a; Briers-Louw et al., 2019; Power et al., 2021).
Furthermore, employing techniques such as soft release using a boma infrastructure is considered to promote release site fidelity and reduce the stress of the translocated animal (Linnell et al., 1997; Weise et al., 2015a; Briers-Louw et al., 2019). However, soft releases are not synonymous with translocation success, as many authors have reported successful translocations using hard releases (Houser et al., 2011; Mondal et al., 2013; Weise et al., 2015a). Translocation efforts are expensive, and these costs increase with soft release efforts (Weise et al., 2014; Power et al., 2021). While our findings indicate that translocation distances of four times the diameter of home ranges (200 – 400km) will likely increase success without soft release efforts, we were unable to test the effect of release type on the likelihood of translocation success due to the small sample size.
Finally, clearly defining criteria of success and failure will improve the comparability among translocation evaluations. Incorporating monitoring efforts on source and receiving populations can reduce the dangers of translocation (Weise et al., 2015a). In addition to the parameters considered in our study (i.e., stabilization of home range, survival, and no livestock depredation at the release site), reproductive success was previously considered a barometer of translocation success (Weise et al., 2015a; Power et al., 2021). However, this requires a long post-monitoring survey (1 to 2 years) and is not always possible for practical, financial, and technical reasons. Importantly, there is a need for reporting the findings of all translocation attempts, as these findings, along with other scientific literature, would probably improve the success rates. The lack of transparency hinders the efforts to understand the scope of the problem, reduces the possibility of finding solutions, and limits our understanding of the effects that translocations have on leopard populations (Athreya et al., 2011; Lorand et al., 2022).
Therefore, there is a need for standardized protocols for pre- and post-release monitoring to promote successful translocation efforts while respecting the legislation of each South African province. Standardizing the translocation protocols can contribute to managing DCAs and inform conservation strategy that can restore vulnerable populations or re-establish an extinct population within their historical range, if implemented responsibly. Moreover, formalizing data gathering prescripts and maintaining a rigorous centralized database for all translocation events will contribute to evaluating the effectiveness of each event. Without such efforts, translocation actions may remain inconclusive and controversial as a management tool, which limits its potential to contribute towards the conservation management of species.
Box 1. Proposed protocols for leopard translocations.
Conditions required for consideration for the translocation of damage-causing leopards
1. Leopard causes validated, repeated, and regular losses to livestock where several non-lethal mitigation actions have failed.
2. All mitigation measures proposed by conservation authorities have been exhausted.
3. Leopard causes validated, repeated, regular, and excessive losses to wild game or domestic specimens managed in breeding camps, which is inconsistent with the animal’s natural behavior and is ecologically or excessively commercially damaging in areas with certificates of adequate enclosures for the species affected.
4. Leopard presents an imminent and realistic threat to human life.
Pre-conditions for translocation to be undertaken
1. Ensuring that the release site is large enough to support leopards in a wild, free-roaming territory.
2. Release site habitat has depleted presence or absent leopard activity, as is reasonably possible to determine, or areas where such releases would be tolerated and provide opportunities for ecological dispersal.
3. Encourage ecologically compatible release site habitat to the original capture site habitat.
4. Receiving habitat has adequate suitable natural prey availability.
5. Release site is considered to have no or very low chance of post-release human–carnivore conflict present.
6. Release site is not surrounded by intensive livestock farming or intensive game breeding camps with rare, very-high-value game which is also natural prey for leopards.
7. Released leopards must be GPS satellite and monitored and form part of a scientific analysis of the efficacy of such translocations.
8. Data on basic morphology, sex of the animal, and reason for translocation should be collected.
9. Genetic analysis of all leopards will be undertaken to understand the genetics of local populations, and best available information must be consulted, i.e., ecotypes, subspecies.
10. 10. Consideration should be given to local institutional arrangements and variations of different leopard conservation imperatives in each region by considering variations in leopard biological responses within their respective areas.
Post-translocation objectives
1. Leopards are not to be habituated or kept captive beyond the release period deemed appropriate by the conservation authorities and veterinarians caring for the animals.
2. Leopards must be GPS satellite-collared and monitored for as long as possible, but at least 6 months post-release, and form part of the scientific analysis of the efficacity of translocations.
3. Preferentially, leopards are to be released into state/or collaborating private protected areas wherefrom they had been extirpated.
4. Private landowners or entities receiving the translocated leopards must be encouraged to contribute towards the costs of translocation, collars, and any costs incurred in monitoring or analysis of the translocation effort.
5. Private protected area entities receiving the leopards must enter a legal contract with the authorities to take all legal and financial responsibility for any damage that may result from the relocated animal.
6. Any subsequent interference with the leopard must be subject to relevant permitting.
7. The entities receiving the leopards will comply with all permit requirements and conditions.
8. Translocated leopards may not be hunted.
9. Entities that want to receive leopards may not canvas for leopards to be labelled as DCA, any such actions will disqualify applicants from participating in translocations.
10. Leopards will be randomly allocated to receiving entities by the authorities if competing private applications exist, but ecological considerations will more than likely be able to discriminate the most suitable release sites.
11. The authorities may elect to serially send opposite sexes sequentially to the same location should attempts be supported to re-establish leopards in former areas of distribution (i.e., reintroduction).
The original contributions presented in the study are included in the article/supplementary material. Further inquiries can be directed to the corresponding author.
This research was reviewed and approved by Rhodes University ethics authorization (2007/11/1), Witwatersrand University (2011/05/04), University of the Western Cape ethics authorization (AREC19/2/1) and provincial research permits as issued by the Eastern Cape Department of Economic Development, Environmental Affairs (24503), and Western Cape: Cape Nature (0035-AAA004-00797).
All authors contributed to draft editing. All authors contributed to the article and approved the submitted version.
We would like to extend our gratitude to the funders of this study: Hans Hoheisen Conservation Trust, Mary Oppenheimer and Daughters Foundation, National Department of Forestry, Fisheries and Environment, Development Bank of Southern Africa, Global Environmental Facility project managed by the United Nations Environmental Program: GEF Project identity number: 9382; Umoja No. 01333, Green Fund, United Nations Environmental Program, United Nations Development Program, GEF Small Grants Programme, National Lotteries Distribution Trust Fund, now called National Lotteries Commission, Project Number 73027, Landmark Foundation Trust, Henry and Iris Englund Foundation, Abax Foundation, Mones Michaels Trust, Felix Schneier Foundation, JDI, Brad Banducci and Arne Hanson.
We thank Dr. Ernst Baard, Dr. Charlene Bissett, Dr. Judith Botha, Corne Claassen, Barend Le Roux, and John Power for their assistance with data collection and for comments that improved the manuscript. JP also provided us with high-quality GPS data and provided valuable inputs to earlier drafts that improved the manuscript. We sincerely thank the landowners who participated in this study. We acknowledge CapeNature, South African National Parks, Eastern Cape Department of Economic Development and Environmental Affairs and Parks and Tourism Agency, and Northern Cape Department of Agriculture, Environmental Affairs, Rural Development and Land Reform, Sanbona Wildlife Reserve, Kazuko Lodge, North West Provincial Government’s Department of Economic Development, Environment, Conservation and Tourism, and Cheetah Outreach Trust for access to their data and managed areas and access to the leopards we studied. David Gaynor assisted with the study’s development and data processing, and Matthew Schurch and Stefan Goets are thanked for their input to some data capture.
The authors declare that the research was conducted in the absence of any commercial or financial relationships that could be construed as a potential conflict of interest.
All claims expressed in this article are solely those of the authors and do not necessarily represent those of their affiliated organizations, or those of the publisher, the editors and the reviewers. Any product that may be evaluated in this article, or claim that may be made by its manufacturer, is not guaranteed or endorsed by the publisher.
Armstrong D. P., Seddon P. J. (2008). Directions in reintroduction biology. Trends Ecol. Evol. 23 (1), 20–25. doi: 10.1016/j.tree.2007.10.003
Athreya V., Odden M., Linnell J. D. C., Karanth K. U. (2011). Translocation as a tool for mitigating conflict with leopards in human-dominated landscapes of India. Conserv. Biol. 25 (1), 133–141. doi: 10.1111/j.1523-1739.2010.01599.x
Bailey T. N. (1993). The African leopard: a study of the ecology and behaviour of a solitary felid (New York: Columbia University Press).
Balme G. A., Hunter L. T. B. (2013). Why leopards commit infanticide. Anim. Behav. 86 (4), 791–799. doi: 10.1016/j.anbehav.2013.07.019
Balme G. A., Slotow R., Hunter L. T. B. (2009). Impact of conservation interventions on the dynamics and persistence of a persecuted leopard (Panthera pardus) population. Biol. Conserv. 142 (11), 2681–2690. doi: 10.1016/j.biocon.2009.06.020
Bauder J. M., Roberts N. M., Ruid D., Kohn B., Allen ,. M. L. (2020). Black bear translocations in response to nuisance behaviour indicate increased effectiveness by translocation distance and landscape context. Wildlife Res. 47 (5), 426–435. doi: 10.1071/WR19161
Bauder J. M., Ruid D., Roberts N. M., Kohn B., Allen M. L. (2021). Effects of translocation on survival of nuisance bears. Anim. Conserv. 24 (5), 820–831. doi: 10.1111/acv.12684
Bennett N. J., Roth R., Klain S. C., Chan K., Christie P., Clark D. A., et al. (2017). Conservation social science: Understanding and integrating human dimensions to improve conservation. Biol. Conserv. 205, 93–108. doi: 10.1016/j.biocon.2016.10.006
Berger-Tal O., Blumstein D. T., Swaisgood R. R. (2020). Conservation translocations: a review of common difficulties and promising directions. Anim. Conserv. 23 (2), 121–131. doi: 10.1111/acv.12534
Boronyak L., Jacobs B., Wallach A., McManus J., Stone S., Stevenson S., et al. (2022). Pathways towards coexistence with large carnivores in production systems. Agric. Hum. Values 39 (1), 47–64. doi: 10.1007/s10460-021-10224-y
Bradley E. H., Pletscher D. H., Bangs E. E., Kunkel K. E., Smith D. W., Mack C. M., et al. (2005). Evaluating wolf translocation as a nonlethal method to reduce livestock conflicts in the northwestern united states. Conserv. Biol. 19 (5), 1498–1508. doi: 10.1111/j.1523-1739.2005.00102.x
Briers-Louw W. D., Verschueren S., Leslie A. J. (2019). Big cats return to majete wildlife reserve, malawi: Evaluating reintroduction success. Afr. J. Wildlife Res. 49 (1), 34–50. doi: 10.3957/056.049.0034
Calabrese J. M., Fleming C. H., Gurarie E. (2016). Ctmm: an r package for analyzing animal relocation data as a continuous-time stochastic process. Methods Ecol. Evol. 7 (9), 1124–1132. doi: 10.1111/2041-210X.12559
Clark J. D., Huber D., Servheen C. (2002). Bear reintroductions: lessons and challenges. Ursus 13, 335–345.
Consorte-McCrea A., Kolipaka S., Owens J. R., Ruiz-Miranda C. R., Waters S. (2022). Guidelines to facilitate human-wildlife interactions in conservation translocations. Front. Conserv. Sci. 3, 1–18. doi: 10.3389/fcosc.2022.788520
Devens C. H., Hayward M. W., Tshabalala T., Dickman A., McManus J. S., Smuts B., et al. (2021). Estimating leopard density across the highly modified human-dominated landscape of the Western cape, south Africa. ORYX 55 (1), 34–45. doi: 10.1017/S0030605318001473
Devens C., Tshabalala T., McManus J., Smuts B. (2018). Counting the spots: The use of a spatially explicit capture–recapture technique and GPS data to estimate leopard (Panthera pardus) density in the Eastern and Western cape, south Africa. Afr. J. Ecol. 56 (4), 850–859. doi: 10.1111/aje.12512
Dutta T., Sharma S., Maldonado J. E., Wood T. C., Panwar H. S., Seidensticker J. (2013). Fine-scale population genetic structure in a wide-ranging carnivore, the leopard (Panthera pardus fusca) in central India. Diversity Distributions 19 (7), 760–771. doi: 10.1111/ddi.12024
Elbroch L. M., Lendrum P. E., Quigley H., Caragiulo A. (2016). Spatial overlap in a solitary carnivore: Support for the land tenure, kinship or resource dispersion hypotheses? J. Anim. Ecol. 85 (2), 487–496. doi: 10.1111/1365-2656.12447
Fattebert J., Balme G., Dickerson T., Slotow R., Hunter L. (2015). Density-dependent natal dispersal patterns in a leopard population recovering from over-harvest. PloS One 10 (4), e0122355. doi: 10.1371/journal.pone.0122355
Fattebert J., Dickerson T., Balme G., Slotow R., Hunter L. (2013). Long-distance natal dispersal in leopard reveals potential for a three-country metapopulation. South Afr. J. Wildlife Res. 43 (1), 61–67. doi: 10.3957/056.043.0108
Fleming C. H., Calabrese J. M. (2016). “Ctmm: Continuous-time movement modeling,” in R package version 0.3. 2. Available at: https://CRAN.R-project.org/package=ctmm.
Fontúrbel F. E., Simonetti J. A. (2011). Translocations and human-carnivore conflicts: Problem solving or problem creating? Wildlife Biol. 17 (2), 217–224. doi: 10.2981/10-091
Gavashelishvili A., Lukarevskiy V. (2008). Modelling the habitat requirements of leopard panthera pardus in west and central Asia. J. Appl. Ecol. 45 (2), 579–588. doi: 10.1111/j.1365-2664.2007.01432.x
Goodrich J. M., Miquelle D. G. (2005). Translocation of problem amur tigers panthera tigris altaica to alleviate tiger-human conflicts. ORYX 39 (4), 454–457. doi: 10.1017/S0030605305001146
Grimbeek A. M. (1992). The ecology of the leopard (Panthera pardus) in the waterberg – PhD thesis (South Africa: University of Pretoria).
Hamilton P. H. (1981). The leopard Panthera paradus and the cheetah Acinonyx jubatus in Kenya. Nairobi: Report for the U.S. Fish & Wildlife Service and The Government of Kenya, Nairobi.
Hayward M. W., Henschel P., O’Brien J., Hofmeyr M., Balme, Kerley G. I. H. (2006). The successful reintroduction of leopard panthera pardus to the addo elephant national park. J. Zool. 270 (2), 298–313.
Houser A., Gusset M., Bragg C. J., Boast L. K., Somers M. J. (2011). Pre-release hunting training and post-release monitoring are key components in the rehabilitation of orphaned large felids. South Afr. J. Wildlife Res. 41 (1), 11–20. doi: 10.3957/056.041.0111
Hunter L., Henschel P., Ray J. (2013). “Panthera pardus,” in The mammals of Africa.Vol. V: Carnivores, pangolins, equids and rhinoceroses. Eds. Kingdon J., Hoffmann M.(Bloomsbury, London), 159–168.
Inskip C., Zimmermann A. (2009). Human-felid conflict: A review of patterns and priorities worldwide. ORYX 43 (1), 18–34. doi: 10.1017/S003060530899030X
IUCN/SSC (2013). Guidelines for reintroductions and other conservation translocations (Gland, Switzerland).
Jacobson A. P., Gerngross P., Lemeris J. R., Schoonover R. F., Anco C., Breitenmoser-Würsten C., et al. (2016). Leopard (Panthera pardus) status, distribution, and the research efforts across its range. PeerJ 4, e1974. doi: 10.7717/peerj.1974
Johnson W. E., Onorato D. P., Roelke M. E., Land E. D., Cunningham M., Belden R. C., et al. (2010). Genetic restoration of the Florida panther. Science 329(5999), 1641–1645. doi: 10.1126/science.1192891
Keyghobadi N. (2007). The genetic implications of habitat fragmentation for animals. Can. J. Zool. 85 (10), 1049–1064. doi: 10.1139/Z07-095
Khorozyan I., Waltert M. (2021). A global view on evidence-based effectiveness of interventions used to protect livestock from wild cats. Conserv. Sci. Pract. 3 (2), 1–13. doi: 10.1111/csp2.317
Landriault L. J., Brown G. S., Hamr J., Mallory F. F. (2009). Age, sex and relocation distance as predictors of return for relocated nuisance black bears ursus americanus in Ontario, Canada. Wildlife Biol. 15 (2), 155–164. doi: 10.2981/07-084
Lenain D. M., Warrington S. (2001). Is translocation an effective tool to remove predatory foxes from a desert protected area? J. Arid Environments 48 (2), 205–209. doi: 10.1006/jare.2000.0754
Linnell J. D. C., Aanes R., Swenson J. E., Odden J., Smith M. E. (1997). Translocation of carnivores as a method for managing problem animals: A review. Biodiversity Conserv. 6 (9), 1245–1257. doi: 10.1023/B:BIOC.0000034011.05412.cd
Lino A., Fonseca C., Rojas D., Fischer E., Ramos Pereira M. J. (2019). A meta-analysis of the effects of habitat loss and fragmentation on genetic diversity in mammals. Mamm. Biol. 94 (1), 69–76. doi: 10.1016/j.mambio.2018.09.006
Lorand C., Robert A., Gastineau A., Mihoub J.-B., Bessa-Gomes C. (2022). Effectiveness of interventions for managing human-large carnivore conflicts worldwide. Sci. Total Environ. 838 (2), 156195. doi: 10.1016/j.scitotenv.2022.156195
Mann G. K. H., O’Riain M. J., Parker D. M. (2020). A leopard’s favourite spots: Habitat preference and population density of leopards in a semi-arid biodiversity hotspot. J. Arid Environments 181, 104218. doi: 10.1016/j.jaridenv.202.104218
McManus J. S., Dalton D. L., Kotzé A., Smuts B., Dickman A., Marshal J. P., et al. (2014). Gene flow and population structure of a solitary top carnivore in a human-dominated landscape. Ecol. Evol. 5 (2), 335–344. doi: 10.1002/ece3.1322
McManus J. S., Dickman A. J., Gaynor D., Smuts B. H., Macdonald D. W. (2015). Dead or alive? comparing costs and benefits of lethal and non-lethal human-wildlife conflict mitigation on livestock farms. ORYX 49 (4), 687–695. doi: 10.1017/S0030605313001610
McManus J., Marshal J. P., Keith M., Tshabalala T., Smuts B., Treves A. (2021). Factors predicting habitat use by leopards in human-altered landscapes. J. Mammalogy 102 (6), 1473–1483. doi: 10.1093/jmammal/gyab110
McManus J., Schurch M. P. E., Goets S., Faraut L., Couldridge V., Smuts B. (2022). Delineating functional corridors linking leopard habitat in the Eastern and Western cape, south Africa. Conservation 2 (1), 99–122. doi: 10.3390/conservation2010009
McRae B. H., Beier P., Dewald L. E., Huynh L. Y., Keim P. (2005). Habitat barriers limit gene flow and illuminate historical events in a wide-ranging carnivore, the American puma. Mol. Ecol. 14 (7), 1965–1977. doi: 10.1111/j.1365-294x.2005.02571.x
Miller S. M., Druce D. J., Dalton D. L., Harper C. K., Kotze A., Packer C., et al. (2020). Genetic rescue of an isolated African lion population. Conserv. Genet. 21 (1), 41–53. doi: 10.1007/s10592-019-01231-y
Milligan S., Brown L., Hobson D., Frame P., Stenhouse G. (2018). Factors affecting the success of grizzly bear translocations. J. Wildlife Manage. 82 (3), 519–530. doi: 10.1002/jwmg.21410
Mills M. G. L. (1991). Conservation management of large carnivores in africa. Koedoe 34 (1), 81–90. doi: 10.4102/koedoe.v34i1.417
Mondal K., Bhattacharjee S., Gupta S., Sankar K., Qureshi Q. (2013). Home range and resource selection of problem leopards translocated to forested habitat. Curr. Sci. 105 (3), 338–345.
Morapedi M., Reuben M., Gadimang P., Bradley J., Given W., Reading R. P., et al. (2021). Outcomes of lion, panthera leo, translocations to reduce conflict with farmers in Botswana. Afr. J. Wildlife Res. 51 (1), 6–12. doi: 10.3957/056.051.0006
Moreira-Arce D., Ugarte C. S., Zorondo-Rodríguez F., Simonetti J. A. (2018). Management tools to reduce carnivore-livestock conflicts: Current gap and future challenges. Rangeland Ecol. Manage. 71 (3), 389–394. doi: 10.1016/j.rama.2018.02.005
Mowry R. A., Schneider T. M., Latch E. K., Gompper M. E., Beringer J., Eggert L. S. (2015). Genetics and the successful reintroduction of the Missouri river otter. Anim. Conserv. 18 (2), 196–206. doi: 10.1111/acv.12159
Mueller S. A., Reiners T. E., Middelhoff T. L., Anders O., Kasperkiewicz A., Nowak C. (2020). The rise of a large carnivore population in central Europe: genetic evaluation of lynx reintroduction in the harz mountains. Conserv. Genet. 21 (3), 577–587. doi: 10.1007/s10592-020-01270-w
Müller L., Briers-Louw W. D., Seele B. C., Stefanus Lochner C., Amin R. (2022). Population size, density, and ranging behaviour in a key leopard population in the Western cape, south Africa. PloS One 17 (5), p.e0254507. doi: 10.1371/journal.pone.0254507
Naude V. N., Balme G. A., O’Riain J., Hunter L. T. B., Fattebert J., Dickerson T., et al. (2020). Unsustainable anthropogenic mortality disrupts natal dispersal and promotes inbreeding in leopards. Ecol. Evol. 10 (8), 3605–3619. doi: 10.1002/ece3.6089
Noonan M. J., Tucker M. A., Fleming C. H., Akre T., Alberts S. C., Ali A. H., et al. (2019). A comprehensive analysis of autocorrelation and bias in home range estimation. Ecol. Monogr. 89 (2), e01344. doi: 10.1002/ecm.1344
Pooley S., Bhatia S., asava A. (2021). Rethinking the study of human–wildlife coexistence. Conserv. Biol. 35 (3), 784–793. doi: 10.1111/cobi.13653
Power R. J., Venter L., Botha M., Bartels P. (2021). Repatriating leopards into novel landscapes of a south African province. Ecol. Solutions Evidence 2 (1), 1–19. doi: 10.1002/2688-8319.12046
Rabinowitz A. R. (1986). Jaguar predation on domestic livestock in belize. Wildlife Soc. Bull. 14 (2), 170–174.
R Core Team (2021). R: A language and environment for statistical computing (Vienna, Austria: R Foundation for Statistical Computing). Available at: https://www.r-project.org/.
Ripple W. J., Estes J. A., Beschta R. L., Wilmers C. C., Ritchie E. G., Hebblewhite M., et al. (2014). Status and ecological effects of the world’s largest carnivores. Science 343 (6167), 124184. doi: 10.1126/science.1241484
Roques S., Sollman R., Jacomo A., Torres N., Silveira L., Chavez C., et al. (2016). Effects of habitat deterioration on the population genetics and conservation of the jaguar. Conserv. Genet. 17 (1), 125–139. doi: 10.1007/s10592-015-0766-5
Roux P. G. le, Skinner ,. J. D. (1989). A note on the ecology of the leopard (Panthera pardus linnaeus) in the londolozi game reserve, south Africa. Afr. J. Ecol. 27 (2), 167–171. doi: 10.1111/j.1365-2028.1989.tb00940.x
Rust N. A., Whitehouse-Tedd K. M., MacMillan D. C. (2013). Perceived efficacy of livestock guarding dogs in south africa: implications for cheetah conservation. Wildlife Soc. Bull. 37 (4), 690–697. doi: 10.1002/wsb.352
Seoraj-Pillai N., Pillay N. (2017). A meta-analysis of human-wildlife conflict: South African and global perspectives. Sustainability (Switzerland) 9 (34), 1–21. doi: 10.3390/su9010034
Stahl P., Vandel J. M., Herrenschmidt V., Migot P. (2001). The effect of removing lynx in reducing attacks on sheep in the French jura mountains. Biol. Conserv. 101 (1), 15–22. doi: 10.1016/S0006-3207(01)00054-4
Stein A. B., Athreya V., Gerngross P., Balme G., Henschel P., Karanth U., et al. (2020). “Panthera pardus (amended version of 2019 assessment),” in The IUCN red list of threatened species. Gland, Switzerland.
Stenhouse G. B., Larsen T. A., McClelland C. J. R., Wilson A. E., Graham K., Wismer D., et al. (2022). Grizzly bear response to translocation into a novel environment. Wildlife Res. doi: 10.1071/WR21060
Steyaert S. M. J. G., Endrestøl A., Hackländer K., Swenson J. E., Zedrosser A. (2012). The mating system of the brown bear Ursus arctos. Mammal Rev. 42 (1), 12–34. doi: 10.1111/j.1365-2907.2011.00184.x
Swanepoel L. H., Lindsey P., Somers M. J., Hoven W.v., Dalerum (2013). Extent and fragmentation of suitable leopard habitat in south Africa. Anim. Conserv. 16 (1), 41–50. & F. doi: 10.1111/j.1469-1795.2012.00566.x
Swanepoel L. H., Williams S., Power R. J. (2016). “A conservation assessment of panthera pardus,” in The red list of mammals of south Africa, Lesotho and Swaziland. Eds. Child M. F., Roxburgh L., do Linh San E., Raimondo D., Davies-Mostert H. T. (South Africa: South African National Biodiversity Institute and Endangered Wildlife Trust).
Teichman K. J., Cristescu B., Darimont C. T. (2016). Hunting as a management tool? cougar-human conflict is positively related to trophy hunting. BMC Ecol. 16 (1), 1–8. doi: 10.1186/s12898-016-0098-4
Tensen L., Jansen Van Vuuren B., Plessis C., Marneweck D. G. (2019). African Wild dogs: Genetic viability of translocated populations across south Africa. Biol. Conserv. 234, 131–139. du. doi: 10.1016/j.biocon.2019.03.033
Treves A., Krofel M., McManus J. (2016). Predator control should not be a shot in the dark. Front. Ecol. Environ. 14 (7), 380–388. doi: 10.1002/fee.1312
Weilenmann M., Gusset M., Mills D. R., Gabanapelo T., Schiess-Meier M. (2010). Is translocation of stock-raiding leopards into a protected area with resident conspecifics an effective management tool? Wildlife Res. 37 (8), 702–707. doi: 10.1071/WR10013
Weise F. J., Lemeris J., Stratford K. J., Vuuren R. J. v., Munro S. J., Crawford S. J., et al. (2015a). A home away from home: insights from successful leopard (Panthera pardus) translocations. Biodiversity Conserv. 24 (7), 1755–1774. doi: 10.1007/s10531-015-0895-7
Weise F. J., Stratford K. J., Vuuren R. J. v. (2014). Financial costs of large carnivore translocations - accounting for conservation. PloS One 9 (8), e105042. doi: 10.1371/journal.pone.0105042
Keywords: carnivore conservation, damage-causing animal, human–carnivore conflict, leopard, Panthera pardus, translocation, conservation management
Citation: McManus J, Faraut L, Couldridge V, van Deventer J, Samuels I, Cilliers D, Devens C, Vorster P and Smuts B (2022) Assessment of leopard translocations in South Africa. Front. Conserv. Sci. 3:943078. doi: 10.3389/fcosc.2022.943078
Received: 13 May 2022; Accepted: 27 June 2022;
Published: 29 July 2022.
Edited by:
Carlos R. Ruiz-Miranda, State University of the North Fluminense Darcy Ribeiro, BrazilReviewed by:
Willem Briers-Louw, Zambeze Delta Conservation, MozambiqueCopyright © 2022 McManus, Faraut, Couldridge, van Deventer, Samuels, Cilliers, Devens, Vorster and Smuts. This is an open-access article distributed under the terms of the Creative Commons Attribution License (CC BY). The use, distribution or reproduction in other forums is permitted, provided the original author(s) and the copyright owner(s) are credited and that the original publication in this journal is cited, in accordance with accepted academic practice. No use, distribution or reproduction is permitted which does not comply with these terms.
*Correspondence: Jeannine McManus, amVhbm5pbmVAbGFuZG1hcmtmb3VuZGF0aW9uLm9yZy56YQ==
†These authors have contributed equally to this work
Disclaimer: All claims expressed in this article are solely those of the authors and do not necessarily represent those of their affiliated organizations, or those of the publisher, the editors and the reviewers. Any product that may be evaluated in this article or claim that may be made by its manufacturer is not guaranteed or endorsed by the publisher.
Research integrity at Frontiers
Learn more about the work of our research integrity team to safeguard the quality of each article we publish.