- 1Ecology and Evolutionary Biology Program, Texas A&M University, College Station, TX, United States
- 2Department of Ecology and Conservation Biology, Texas A&M University, College Station, TX, United States
The concept of ecological resilience is widely used to assess how species and ecosystems respond to external stressors but is applied infrequently at the level of the community or to chronic, ongoing disturbances. In this review, we first discuss the concept of ecological resilience and methods for quantifying resilience in ecological studies. We then synthesize existing evidence for the resilience of avian communities to climate change and urbanization, two chronic disturbances that are driving global biodiversity loss, and conclude with recommendations for future directions. We only briefly discuss the theoretical framework behind ecological resilience and species-specific responses to these two major disturbances, because numerous reviews already exist on these topics. Current research suggests strong heterogeneity in the responses and resilience of bird communities to urbanization and climate change, although community disassembly and reassembly is high following both disturbances. To advance our understanding of community resilience to these disturbances, we recommend five areas of future study (1) the development of a standardized, comprehensive community resilience index that incorporates both adaptive capacity and measures of functional diversity, (2) measurement/modeling of both community resistance and recovery in response to disturbance, (3) multi-scale and/or multi-taxa studies that include three-way interactions between plants, animals, and climate, (4) studies that incorporate interactions between disturbances, and (5) increased understanding of interactions between ecological resilience and socio-ecological dynamics. Advancement in these areas will enhance our ability to predict and respond to the rapidly accelerating effects of climate change and urbanization.
1 Introduction
1.1 Overview
A major conservation priority in the field of modern ecology is predicting and ameliorating the effects of climate change and their synergistic interactions with land-use change (Jetz et al., 2007; Northrup et al., 2019), particularly in regions of high biological diversity and/or endemism (Myers et al., 2000). To effectively address this priority, it is critical for scientists and conservation practitioners to have a thorough understanding of: (1) the current manifestation and projected future effects of major anthropogenic factors on ecosystems and natural communities, and (2) methods of quantifying and conceptually understanding how ecosystems, communities, and populations are responding to these changes. Ecological resilience (Holling, 1973) is the ability of a system to resist (Karp et al., 2011; Scheffer et al., 2015), and recover from (Harrison, 1979; Selwood et al., 2015) perturbations, thus encompassing both the process of resistance, the magnitude of disturbance that causes a change in structure, and recovery, the speed of return to the original community function (Table 1) (Tilman and Downing, 1994; Côté and Darling, 2010). Ecological resilience provides a framework for quantifying and conceptualizing ecological response to anthropogenic change at multiple ecological scales (Allen et al., 2005), but reviews at the level of the ecological community are lacking.
Here, we synthesize existing literature on the topic of ecological resilience in birds, and examine how it can be more effectively applied at the level of the community, especially regarding chronic disturbance. Additionally, we discuss how further integration of the concept of resilience with other conservation-oriented frameworks (i.e., adaptive capacity and vulnerability) can improve conservation assessments. Bird communities provide excellent case studies for ecological resilience because they are well-studied, perform a diverse array of ecological functions, and are important providers of ecosystem services (Sekercioglu, 2006; Bregman et al., 2016). Birds are also conspicuous to the general public, leading to an abundance of community science data that can be paired with climate and land-use data to assess the effects of these drivers on avian populations and community structure (Neate-Clegg et al., 2020; Binley et al., 2021). Because birds occupy a range of ecological functions (Sekercioglu, 2006), their community-level resilience may be indicative of ecosystem resilience (Fischer et al., 2007); and birds drive conservation efforts in many regions, particularly in biodiversity hotspots (Myers et al., 2000; Larsen et al., 2012).
Resilience can be defined as the capacity of a community to absorb and recover from disturbance without affecting the function and stability of the community or broader ecosystem (Table 1) (Ives and Carpenter, 2007; Côté and Darling, 2010). This is consistent with the working definition adopted by the majority of recent (post-2015) ecological literature (Fremier et al., 2015; Scheffer et al., 2015; Levine et al., 2016; Falk et al., 2019; Morelli et al., 2020). When community resistance is overcome, community disassembly, or nonrandom loss of species from within a community, can occur (Zavaleta et al., 2009). Following disassembly, recovery does not necessitate a return to the original community composition and structure. Instead, recovery can involve turnover of species within a community (i.e., community reassembly, Table 1; Schaefer et al., 2008) that leads to a resumption of original community functionality. Figure 1 illustrates the resilience of a community to climate change and urbanization through a variety of different mechanisms and outcomes.
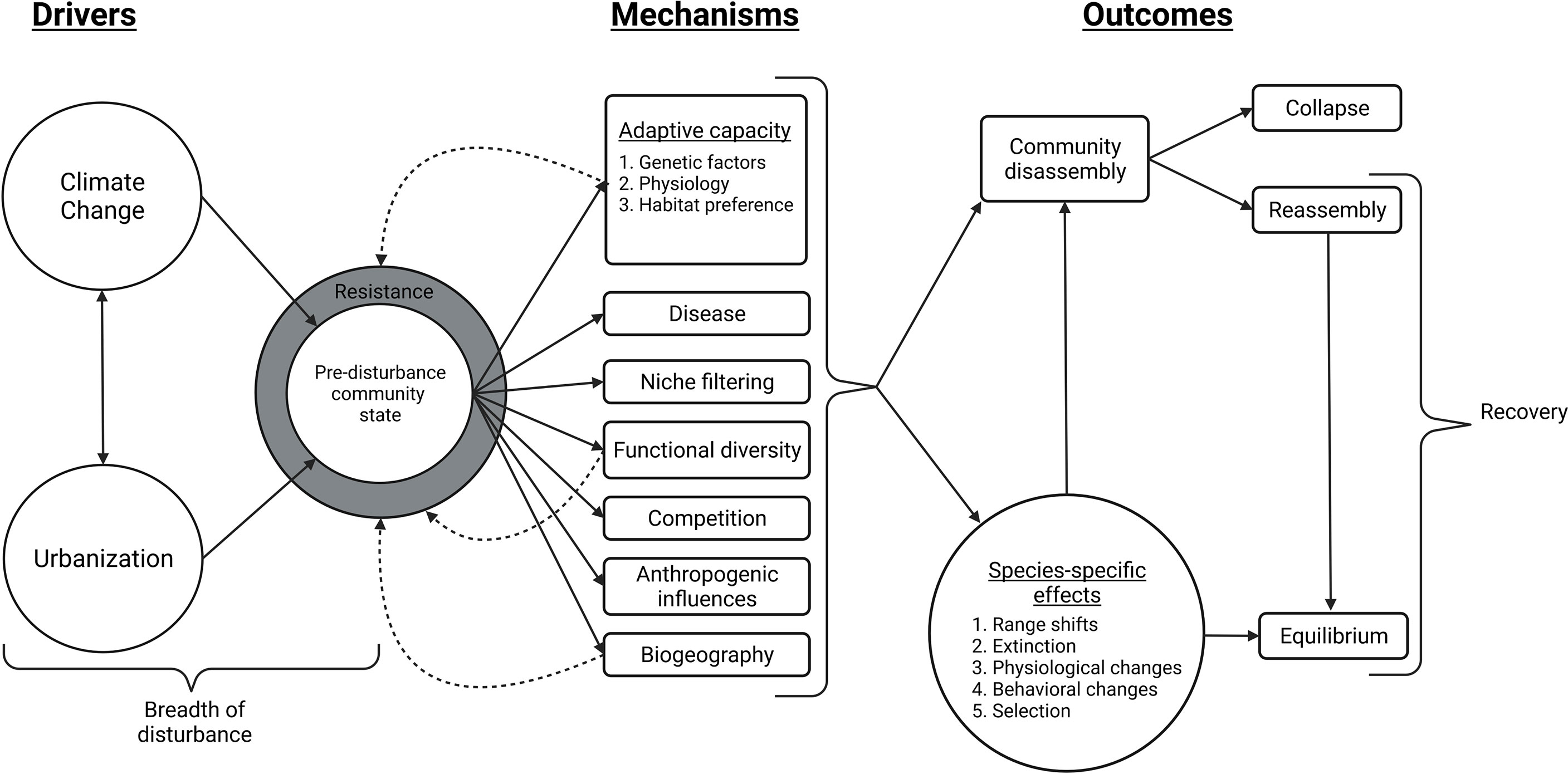
Figure 1 Influence diagram representing community resilience, via the mechanisms by which different community-level outcomes may result from the chronic disturbances of climate change and urbanization, and their interactions. In this diagram, resistance is depicted through the breadth of disturbance and the underlying mechanisms of community response, while recovery occurs through community reassembly and/or when a given community reaches a state of equilibrium. Resistance is a buffer against disturbance that allows for community persistence until it is overcome by the breadth of disturbance. Mechanisms such as adaptive capacity, functional diversity, and biogeography can contribute to resistance and/or recovery by returning a community to its pre-disturbance state when resistance is overcome (illustrated by dotted arrows). This diagram is not a comprehensive illustration of mechanisms and outcomes, but instead depicts those that are commonly associated with climate change and urbanization. These outcomes are also not finite, climate change and urbanization will continue to operate on reassembled and alternative communities through these mechanisms, producing later community disassembly, reassembly, and alternative stable states (Created with BioRender.com).
In this review we focus on avian community resilience in response to the independent and interactive effects of climate change and urbanization, two chronic anthropogenic drivers of global biodiversity loss and community disassembly (Travis, 2003; Jetz et al., 2007; Kampichler et al., 2012; Yalcin and Leroux, 2018). Resilience is typically measured following acute disturbances, where recovery can be easily observed. However, both acute and chronic disturbances have the capacity to shape ecological communities (May, 1977; Johnstone et al., 2016) and many chronic disturbances, such as climate change and urbanization, drive global conservation priorities. An abundance of published literature exists around the broad topics of avian responses to climate change and urbanization, and numerous reviews have synthesized various aspects ranging from species-specific responses (e.g., Marzluff and Ewing, 2008; Reif, 2013; Scridel et al., 2018), to ecosystem-level effects (Garrett et al., 2006; Doney et al., 2012; Aronson et al., 2014), to economic effects (Tol, 2009), among others. We only briefly discuss the theoretical framework behind ecological resilience and species-specific responses to urbanization and climate change, because numerous thorough reviews of these topics already exist (e.g., Holling, 1973; Gunderson, 2000; Crick, 2004; Chace and Walsh, 2006; Jiguet et al., 2006; Leech and Crick, 2007; Bonier, 2012; Reif, 2013; Scheffer et al., 2015; Seress and Liker, 2015; Scridel et al., 2018; Falk et al., 2019; Maxwell et al., 2019). Instead, we synthesize current literature that apply the concept of ecological resilience to avian responses to climate change and urbanization at the community level, and provide recommendations for future studies, including improved methods for quantifying resilience across ecosystem scales.
1.2 Quantifying Resilience
Empirical methods for quantifying resilience in avian communities range from species-specific measures to functional diversity indices, with associated trade-offs in ease of calculation, efficacy, complexity, and relevance (Fischer et al., 2007). Species abundance, occupancy probability, richness, and diversity (e.g., Shannon Index) have been widely used as straightforward proxies for resilience (Clergeau et al., 1998; Johnson and Winker, 2010; Irizarry et al., 2021). For example, Selwood et al. (2015) used species occupancy data generated by community scientists in BirdLife Australia’s Atlas Program to estimate resistance and recovery of Australian birds following a 13-year drought. Abundance, occupancy, richness, and diversity are popular methods for estimating resilience because they are easy to calculate and are adequate for analyzing localized resistance and recovery of species, guilds, or communities (Johnson and Winker, 2010; Karp et al., 2011; Selwood et al., 2015; Irizarry et al., 2021). However, these metrics alone convey little information regarding niche overlap or redundancy, which are important aspects of community resilience. They also do not account for temporal and spatial heterogeneity in the niche space, which may change as a community undergoes a chronic disturbance. Thus, they provide an incomplete (and in some cases, misleading) picture of community resilience, especially at broader temporal or spatial scales.
At the community level, functional diversity indices (Table 1) offer a more complex picture of community resilience (Allen et al., 2005; Mason et al., 2005; Ricotta et al., 2014; Morelli et al., 2020), and are frequently used in assessments of ecosystem services (Sekercioglu, 2012; Lavorel et al., 2013; Song et al., 2014). In contrast to species diversity, functional diversity is concerned with the range of functional traits of species within a community, or those components of the phenotype that influence ecosystem functioning (Petchey and Gaston, 2006) and is calculated using functional groups, or groupings of species based on similarity in ecological functions (Blondel, 2003), as opposed to taxonomic groups (Hooper et al., 2005). Functional diversity indices can be broadly grouped into those that describe how much of the functional niche space is filled by species in the community (i.e., functional richness), or how that space is filled (i.e., functional evenness, functional divergence/variance) (Schleuter et al., 2010). Thus, functional diversity indices consider redundancy and complementarity of species within a community (Schleuter et al., 2010) and provide insights to ecosystem functions (Bremner et al., 2003; Mason et al., 2005), but may not respond to disturbance in the same way as species diversity (Lee and Martin, 2017). Functional diversity indices may act as indicators of community resilience, with higher diversity correlating with higher resilience (Allen et al., 2005).
Functional evenness is a functional diversity index that has garnered particular attention in the resilience literature. It represents the distribution of species abundances across functional space (Villéger et al., 2008), providing an indication of functional redundancies of species within a community and thus the capacity for a community to sustain species loss without suffering declines in function (Morelli et al., 2020). This metric has found wide application in quantifying community responses to disturbance, such as studying how avian communities are responding to forest change in the Amazon (Bregman et al., 2016) and how plant communities respond to various types of disturbance (Fontaine et al., 2006; Biswas and Mallik, 2010; Schneider et al., 2017). However, measuring functional evenness has proven to be difficult since the traditional FEve Index (Villéger et al., 2008) is problematic for detecting community composition in cases where multiple species occupy the same functional space (Mouchet et al., 2010; Ricotta et al., 2014; Van der Linden et al., 2016). Despite these technical issues, functional evenness and other diversity indices are the preferred measures of resilience over phylogenetically-focused metrics because of their emphasis on ecosystem and community functions, and should be used in place of, or in conjunction with these when possible (Mouchet et al., 2010).
1.3 Adaptive Capacity and Resilience
Adaptive capacity is the ability of a system to cope with or adjust to changes in external circumstances or internal demands (Smit et al., 2001; Carpenter and Brock, 2008; Engle, 2011; Thurman et al., 2020), and is a central deterministic component of resilience that affects both resistance and recovery (Gallopin, 2006; Carpenter and Brock, 2008). Although adaptive capacity can be applied to entire ecosystems (Angeler et al., 2019), ecological adaptive capacity is most often applied at the species level. There are three generally recognized components of adaptive capacity: phenotypic plasticity, dispersal ability, and genetic diversity (Beever et al., 2016). These components can be further divided into individual attributes and life history traits, which allows researchers, policymakers, and practitioners to easily calculate the adaptive capacity of individual species (Thurman et al., 2020). At the community-level, adaptive capacity can be estimated for communities indirectly by generalizing from the adaptive capacity of multiple species within that community (Nicotra et al., 2015; Siders, 2019; Thurman et al., 2020), or from representative species from different functional groups (Thurman et al., 2020). For example, if many species within a community have a low adaptive capacity, then that community as a whole may be assumed to also have a low adaptive capacity.
Adaptive capacity is a critical component of resilience (Gallopin, 2006; Carpenter and Brock, 2008), however, there remains a disconnect between the application of adaptive capacity at the species level and the resilience of entire ecological communities (subsequently referred to simply as, “communities”). We suggest that these concepts be considered together in a systems-based approach to analyzing community dynamics in the face of global change. Community ecologists may benefit from looking at the sociological literature, for which adaptive capacity is often used to calculate resilience of human populations (Smit and Wandel, 2006). These methods may be transferable to some extent to ecological communities, although the specific mechanisms driving adaptive capacity and resilience in human systems often differ from those of ecological systems (Thurman et al., 2020). For example, the resilience of human communities can be calculated by averaging the adaptive capacity of individual households for various indicators, then adding additional community-level features (e.g., community organization, dependence on resources) (Maldonado and Moreno-Sanchez, 2014). In an analogous manner, an index for resilience of ecological communities could include the average adaptive capacities of species within the community (weighted, for example, by relative abundance or biomass), and community-level metrics such as functional diversity. However, assessing the determinants of adaptive capacity across multiple species can be difficult (Siders, 2019) and consensus is needed on community traits influencing resilience, including and in addition to measures of functional diversity. The ability to assess and quantify adaptive capacity across avian communities will therefore greatly aid in understanding the interplay between adaptive capacity and avian resilience in the face of urbanization and climate change.
2 Urbanization and Avian Community Resilience
As the global human population grows, widespread losses of habitat and biodiversity are increasingly attributed to urbanization and land use change- in particular, conversion to agriculture. Here we focus on urbanization instead of agricultural conversion because of the rapid growth of urban land area. By 2030, urban land area is projected to increase by around 1.5 million square km, worldwide (Seto et al., 2011; Seto et al., 2012) with 70% of the global human population predicted to live in urban areas by 2050 (Seto et al., 2011). This urban land conversion is occurring at the highest rates in global biodiversity hotspots (Seto et al., 2012), which provide critical avian habitat. Here, we focus on avian community-level responses to urbanization and not species-specific responses because other reviews already exist on these topics (e.g., Chace and Walsh, 2006; Bonier, 2012; Seress and Liker, 2015). We discuss the impacts of urban conversion on avian communities and the subsequent effects on community resilience.
2.1 Impacts of Urban Conversion on Avian Communities
2.1.1 Intermediate Disturbance and Ecosystem Stress-Gradient Hypotheses
Urbanization is widely considered to be a primary driver of biodiversity loss, biotic and seasonal homogenization (McKinney, 2006; Leveau et al., 2021), and community disassembly and destabilization, particularly for specialist species (Biamonte et al., 2011). There are two primary hypotheses that predict the response of avian communities to urbanization: the intermediate disturbance hypothesis and the ecosystem stress-gradient hypothesis. Both of these predictive hypotheses provide insights to how avian communities respond to disturbance events in terms of diversity and richness, and therefore may help predict their resilience and adaptive capacity. Under the intermediate disturbance hypothesis, communities are expected to be more functionally diverse and speciose when disturbance is at an intermediate level, neither too rare nor too common (Grimes, 1973; Horn, 1975; Connell, 1978). This hypothesis is typically applied over a temporal scale, but within the context of urbanization it is often examined along a spatial scale, with intermediate urbanization conditions (e.g., suburban habitats, urban habitats with green spaces, etc.) predicted to be more diverse, and thus resilient, than low or high levels of urbanization. In contrast to the intermediate disturbance hypothesis, the ecosystem stress-gradient hypothesis predicts a decrease in resilience, as measured by species richness and/or functional diversity, as stress increases within a habitat (Bertness and Callaway, 1994). Within the context of urbanization, this hypothesis predicts that richness and diversity will be highest in non-urban, intact habitats and lowest in urban habitats (Lepczyk et al., 2008; Tomasevic, 2017; Evans et al., 2018).
The ecosystem stress-gradient hypothesis is widely supported by the literature on avian diversity, with diversity (and thus, resilience) steadily declining as urbanization increases (McKinney, 2008). However, the intermediate disturbance hypothesis has also found support from studies examining species richness (another measure of resilience), with richness highest at intermediate levels of urbanization (Blair, 1996; Marzluff, 2001; Leveau and Leveau, 2005; and Lepczyk et al., 2008). For example, in the early stages of urbanization (i.e., when cities are small, and distance from city center to habitats of interest is short) both avian and hymenopteran communities increase in species richness (Jokimaki and Suhonen, 1993; Christie and Hochuli, 2009), potentially due to species-specific preferences for edge habitat (Sisk and Battin, 2002). However, this increase is temporary and as urbanization continues communities typically decline in species richness (Jokimaki and Suhonen, 1993), although long-term changes to urban edge effects are understudied. Thus, current evidence suggests that species richness patterns may follow the intermediate disturbance hypothesis, while diversity patterns are most closely associated with the ecosystem-stress gradient hypothesis. Given these conflicting results, it is unclear how community resilience changes with urbanization. Further investigations that incorporate city-specific effects (e.g., human population dynamics, greenspace abundance and arrangement, city age) (Leveau et al., 2017), seasonal and latitudinal changes in richness and diversity (Clergeau et al., 1998; Leveau and Leveau, 2016; Leveau et al., 2017), along with functional diversity indices and adaptive capacity metrics would be of great benefit to understanding the resilience dynamics of urban avian communities and may increase the accuracy of these hypotheses in predictive models.
2.1.2 Biotic Homogenization and Niche Filtering
Under either disturbance hypothesis, high levels of urbanization result in community disassembly through biotic homogenization (i.e., the process by which species within a given habitat become genetically, taxonomically, and functionally similar over time) (Olden et al., 2004; McKinney, 2006) and loss of species richness and functional diversity (Figure 2) (McKinney, 2002; MicKinney, 2006; Ortega-Álvarez and MacGregor-Fors, 2009; Sol et al., 2014) which can fundamentally change the resilience and composition of communities across seasonal and long-term scales (Olden et al., 2004; Leveau et al., 2021). Biotic homogenization is often linked to niche-filtering, the process by which abiotic factors act as a filter on biotic organisms, allowing only certain traits to persist and resulting in coexisting species being more similar than would be expected by chance (Figure 2) (Mouchet et al., 2010). Many studies have highlighted the role of niche-filtering on urban avian communities (McKinney, 2006), with strong, homogenizing selection on foraging and nesting life history traits. In general, foraging strategies including granivory, omnivory, aerial insectivory, and ground-foraging are favored by urban habitats (Emlen, 1974; Allen and O’Connor, 2000; Chace and Walsh, 2006; Croci et al., 2008; Guetté et al., 2017), while surface-foraging insectivory and carnivory are selected against (Lim and Sodhi, 2004; Croci et al., 2008). Regarding nesting behaviors, both cavity-nesting and ground-nesting species are selected against in urban habitats (Tomasevic and Marzluff, 2017), due to the lack of available nest excavation sites (e.g., snags, Tomasevic and Marzluff, 2017) and appropriate, undisturbed ground-nesting habitat (Blewett and Marzluff, 2005; Evans et al., 2011). However, secondary cavity nesting species such as the European starling (Sturnus vulgaris) and black-capped chickadee (Poecile atricapillus) may be more resistant to extirpation in urban habitats than primary cavity nesting species due to their use of anthropogenic cavities (i.e., nest boxes, cavities in human-built structures) (Tomasevic and Marzluff, 2017). Furthermore, habitat specialists across taxa tend to be extirpated or have reduced abundances throughout urban environments (Donnelly and Marzluff, 2006; Devictor et al., 2007), likely due to habitat loss and resource limitations in homogenous urban habitats (Schneiberg et al., 2020).
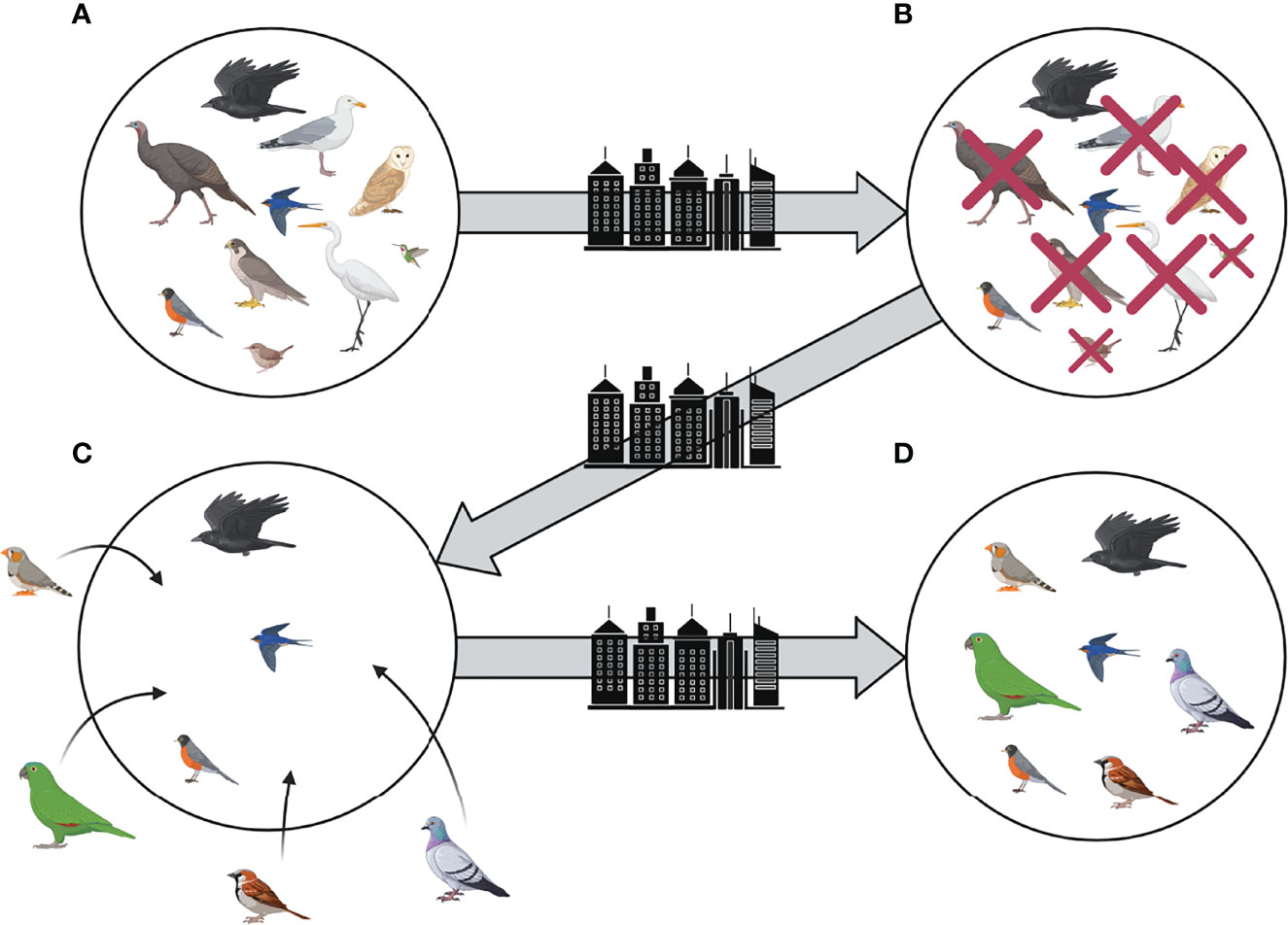
Figure 2 Species loss, replacement, and subsequent shifts in community composition during the chronic disturbance of urbanization, as illustrated by an avian community. The original pre-disturbance community is represented by (A), while a stable post-disturbance community is represented by (D) where the community is more functionally similar than the original state (e.g., predominantly omnivorous species) and non-native species predominate (e.g., house sparrow, rock pigeon, and monk parakeet in the United States). Transitional stages of avian community composition are represented by (B, C), which demonstrate species loss and replacement, respectively, through the process of niche filtering (Created with BioRender.com).
2.2 Avian Community Resilience in Response to and Following Urban Conversion
Traditional resilience metrics (i.e., resistance and recovery as typically applied to acute disturbances) are infrequently applied to urban conversion because the disturbance induced by urbanization is relatively permanent, making it difficult or impossible to measure community recovery (Marzluff and Ewing, 2008). In heavily altered systems such as urban environments, measuring resilience of avian communities is complicated by the multifaceted biophysical (i.e., climate, species-interactions, physical processes, etc.) and socioeconomic (i.e., economic development, human demography, policies and regulation, etc.) influences on systems (Alberti and Marzluff, 2004).
Resilience dynamics under urbanization change as the habitat is converted from natural to suburban to urban (Figure 3). First, a primary disturbance occurs when urban conversion begins. Initial urban conversion is highly disruptive and natural communities have little ability to resist or recover from such extensive habitat destruction, resulting in significant permanent destructuring effects on communities (McKinney, 2006) and converting natural communities to alternative stable suburban communities. Subsequent continued urbanization events can then convert the suburban community to a stable urban community (Figure 3). Suburban avian communities may have greater resistance to continued urban conversion because niche filtering has already begun, but this has not been explicitly investigated to our knowledge. Once urbanization is complete, communities typically reassemble to an alternative stable state (i.e., a novel community) that differs in composition and function from the original state (Figures 2, 4) (e.g., a stable urban system replacing a stable grassland system; Côté and Darling, 2010; Lugo et al., 2012), with little, if any opportunity to recover to the original state. Changes in species assemblages have been noted by Lugo et al. (2012) in Puerto Rican ecosystems, where communities of plants, birds, and reptiles shift to novel assemblages following extensive land-use change for urban or agricultural development. Following this reassembly, urban avian communities may differ in their resilience capacity and subsequent response to future disturbances (Alberti and Marzluff, 2004). As urbanization and the strength of niche filtering increases, avian communities become less diverse in regard to life-history characteristics (Clergeau et al., 2006; Ortega-Álvarez and MacGregor-Fors, 2009; Sol et al., 2014), resulting in a loss of functional groups and correlated community resilience (Figure 2) (Allen et al., 2005). These effects can be compounded by interactions between urbanization and other anthropogenic factors, such as environmental toxins (Møller, 2019). Consequently, the resilience of avian communities to subsequent disturbance is predicted to decrease as urbanization increases.
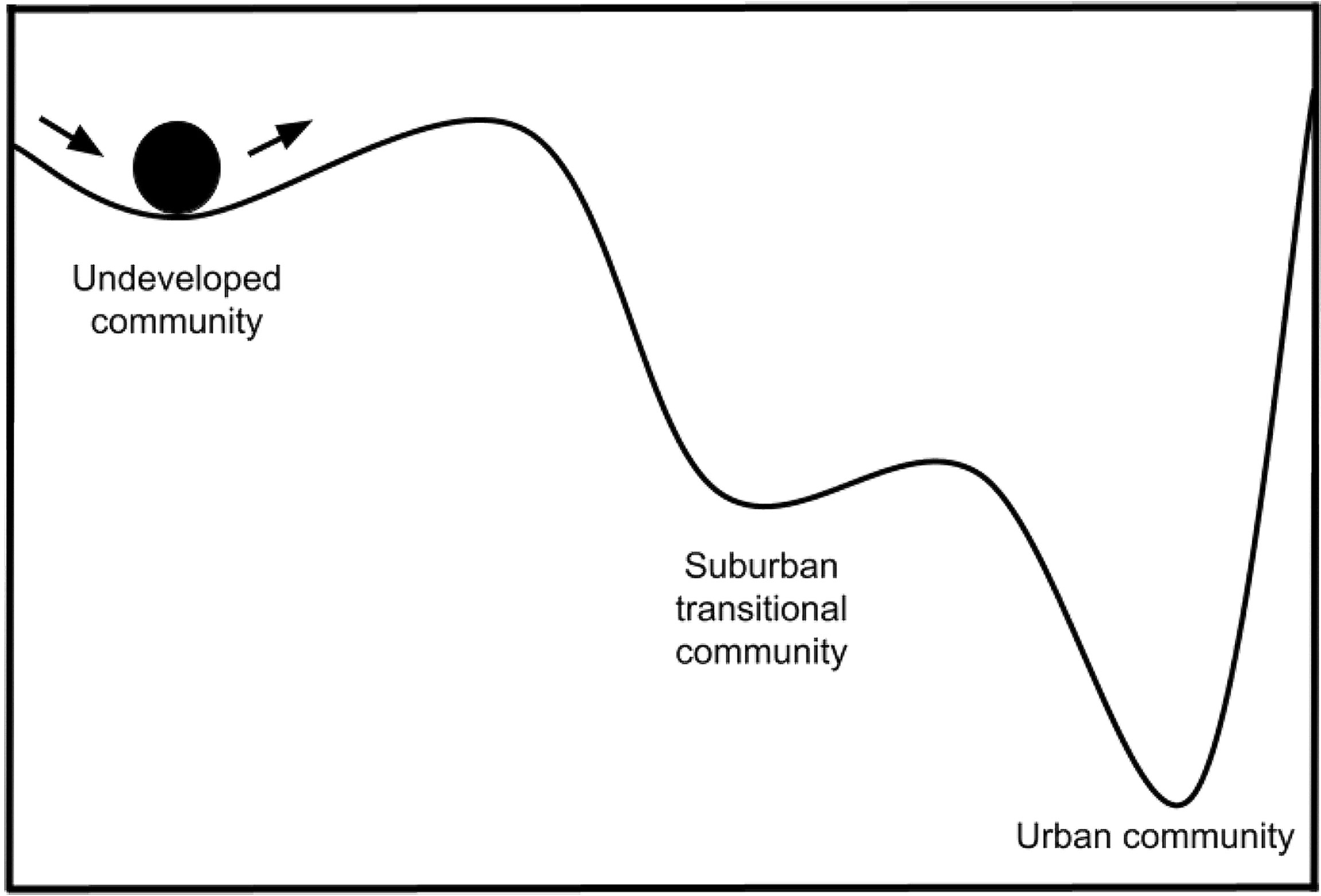
Figure 3 Conceptual diagram of resilience in urban habitats, illustrated by a ball-cup analogy and loosely modeled after Figure 1 of Gunderson (2000). Communities in undeveloped habitats have little resistance to urban conversion and are quickly converted to suburban, then urban communities (although the suburban state can be skipped). Urban communities then require significant energy input to transition to another community state (e.g., through habitat restoration, shown by the steep rightward slope) or to recover to the original, pre-urbanization community (i.e., the steep leftward slope). In most instances recovery to the original community is not observed because urban habitats are rarely reverted to natural, undeveloped states. The steepness of these slopes may decrease with the use of green infrastructure and other habitat improvements, allowing an urban community to transition to a suburban state or a new, semi-urban state.
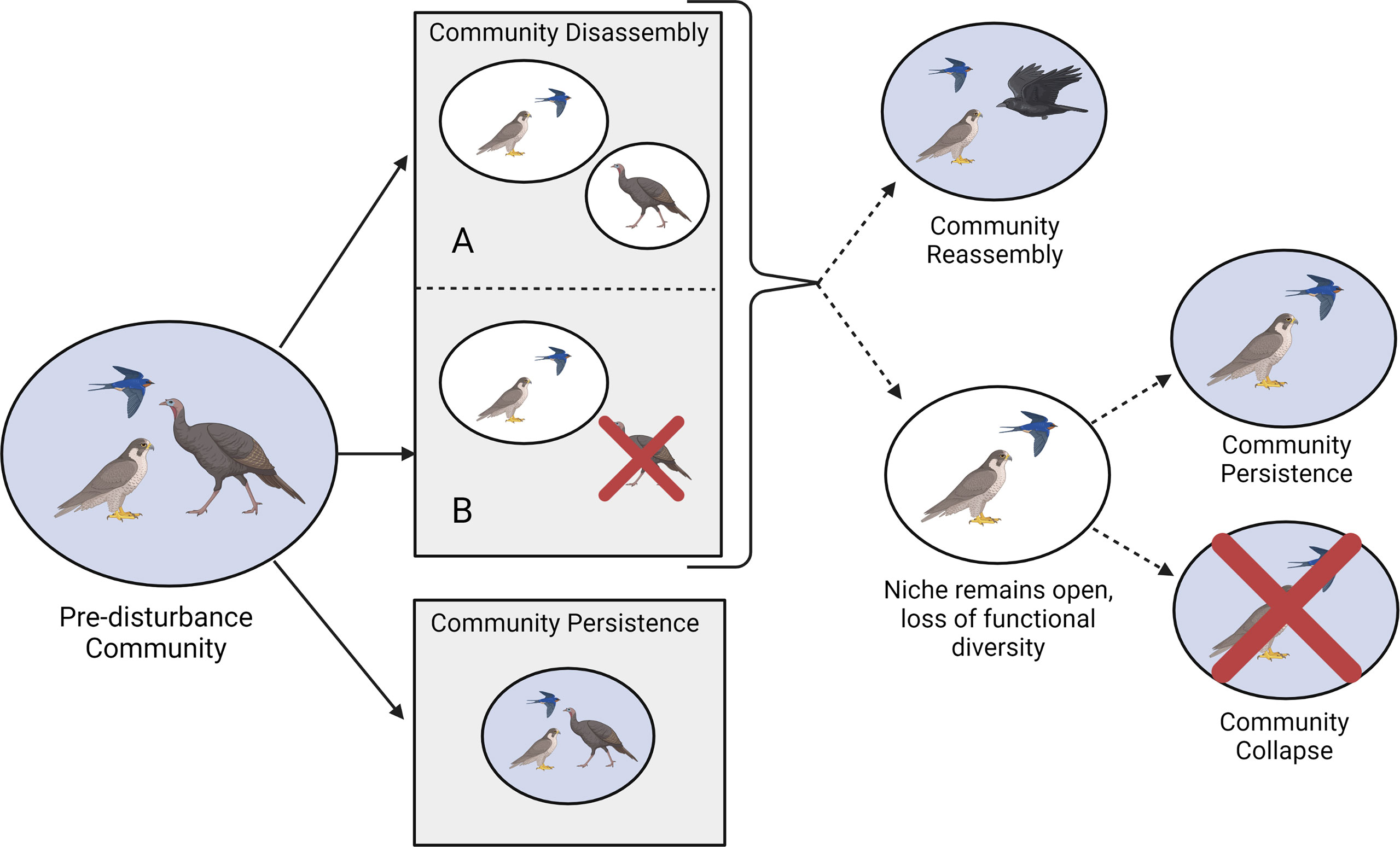
Figure 4 Community shifts as a result of chronic disturbances such as climate change and urbanization. Here, distinct communities are represented by circles, where blue circles represent stable community states and white circles represent transitional community states. Arrows represent temporal progression, where the leftmost set of solid arrows coincides with the initial response to a chronic disturbance event and dotted arrows represent transitional shifts. Under community disassembly, state A represents geographic shifts by some community members and adaptation/plasticity by others, while state B represents adaptation/plasticity or geographic shifts by some community members and partial extinction of others. Alternatively, communities that exhibit adaptation, plasticity, or geographic shifts of all community members may persist, unchanged; these communities would exhibit high resistance. Communities that exhibit later reassembly or persistence following a loss of diversity would exhibit high recovery (Created with BioRender.com).
Species-specific vulnerability to urban conversion also probably plays a role in determining community resilience in urban conversion but is relatively untested in urban resilience assessments. In birds and bats, vulnerability and adaptability to urban habitats are likely trait-mediated (Jung and Kalko, 2011; Jung and Threlfall, 2018; Paton et al., 2019; Tai et al., 2022), but interplay between reproductive, migratory, physiological, and foraging traits determine risk of extirpation from urban habitats (Brown and Graham, 2015). Trait-vulnerability relationships vary among geographic regions (González-Oreja, 2011), and mechanisms underlying these relationships are unknown. More specifically, experimental studies are necessary across species and geographic areas before generalizations regarding trait-mediated vulnerabilities to urban conversion can be formed (Seress and Liker, 2015; Rega-Brodsky et al., 2022).
2.3 Complexities in Predicting the Effects of Urban Conversion on Avian Communities and Resilience
There are numerous complexities in urban conversion that make predicting community-level resilience and outcomes difficult. Many of these topics are understudied or discussed inconsistently in the existing avian literature, s and we echo the calls of other researchers (i.e., Rega-Brodsky et al., 2022) to encourage future research in these areas. Better accounting for these complexities in future studies will be essential for thoroughly understanding the resilience dynamics of avian communities in urban systems, and we suggest the following areas for future study:
1. Incorporation of the concept of recovery into studies of urbanization. This can be difficult, because effects are heterogeneous and the conversion to urban habitats is relatively permanent. However, the restoration of community function following urbanization is relatively unexplored.
2. Multi-scale studies (Hostetler and Holling, 2000; Melles et al., 2003) that incorporate temporal (e.g., time of sampling, age of urban habitats), socioeconomic and land-use factors (Kang et al., 2015; Schütz and Schulze, 2015) into ecological studies of urbanization (Leveau, 2018).
3. Investigations into the multi-trophic effects on communities of exotic/invasive expansion in urban habitats, and their implications for community resilience. Human mediated dispersal of exotic species can strongly affect avian community structure in urban habitats (Donnelly and Marzluff, 2006; Schneiberg et al., 2020). Dominance of exotic plants and birds in urban habitats is well-documented (Green and Baker, 2003; Banerjee and Dewanji, 2017) and these species can negatively impact native avifauna, through resource competition, predation, or other disturbance (Yap and Sodhi, 2004). Invasive species also disrupt avian community reassembly through limitations on prey species (e.g., invasive plants often outcompete native plants, leading to population declines in both invertebrates and their avian predators (Litt et al., 2014; Møller, 2019), nesting habitat, or foraging habitat (Litt et al., 2014; Schneider and Miller, 2014). Although some invasive plants may provide refugia for forest species or understory-nesting birds in urban habitats, these effects are species-specific and vary seasonally (McCusker et al., 2010).
4. Increased studies occurring in the global south and non-English speaking countries. There is extensive geographic bias in the existing urban ecology literature (Rega-Brodsky et al., 2022), with studies occurring in the global north (Nearctic and Paleartic), and English speaking countries being overrepresented, despite many biodiversity hotspots and larger human populations being located in tropical, southern regions (Rega-Brodsky et al., 2022).
3 Climate Change and Avian Community Resilience
The current climate is warming at a rate unprecedented in the last 2000 years (Masson-Delmotte et al., 2021), producing a plethora of climatic changes, including an increase in average precipitation over land, more frequent and intense precipitation events, more frequent concurrent heatwaves and droughts, sea level rise, shifts in storm tracks, and poleward shifts in climate zones (Masson-Delmotte et al., 2021). Over the next century climatic changes are expected to continue and intensify as temperatures increase 1.0 - 5.7°C by 2100 (Masson-Delmotte et al., 2021), resulting in the disappearance of some present climates and the creation of “novel” climates not currently experienced (Williams et al., 2007). Many studies have investigated species-specific responses to climatic changes due to global warming, the results of which are already reviewed by numerous others (e.g., Crick, 2004; Jiguet et al., 2006; Leech and Crick, 2007; Reif, 2013; Scridel et al., 2018; Maxwell et al., 2019). Here, we provide a brief overview of vulnerability and resilience at the species level, then discuss these within the context of avian community impacts and resilience.
3.1 Vulnerability of Bird Species to Climate Change: Exposure and Sensitivity
Vulnerability assessments are a key method for evaluating extinction risk under climate change scenarios and prioritizing conservation actions (Foden et al., 2019). Various definitions of vulnerability exist (Williams et al., 2007), but the Intergovernmental Panel on Climate Change (IPCC) defines vulnerability as “the predisposition to be adversely affected” (Oppenheimer et al., 2014). The vulnerability of species to climate change is typically viewed as a combination of exposure to changing climatic means and extremes (e.g., geographic distribution of the species, climatic niche) and the intrinsic sensitivity of the species (e.g., physiological limits, life history, specialization, and obligate species interactions) (Reif, 2013).
3.1.1 Exposure of Avian Species to Changing Climatic Means and Extremes
Regarding exposure, birds inhabiting poleward portions of continents and tropical montane regions are projected to experience disappearing climates over the next century (Williams et al., 2007), and are at high risk of extinction associated with climate change (Sekercioglu et al., 2012; Reif, 2013; Freeman et al., 2018). Tropical montane species are expected to be vulnerable to the effects of climate change due to the historic stability of temperatures, smaller range sizes near the equator (Williams et al., 2007), and limited extents of occurrence at high elevations (Sekercioglu et al., 2008), which create more narrow endemism in climatic and geographic space (Willams et al., 2007). While exposure is typically estimated broadly for all species within a geographic area, microhabitat and physiological buffering strategies can also influence exposure (Riddell et al., 2021). For example, small mammals are predicted to be less vulnerable to warming and drying due to climate change than sympatric birds because of their lower thermoregulatory cooling costs and the buffering effects of underground burrowing (Riddell et al., 2021).
3.1.2 Sensitivity of Avian Species to Changing Climatic Means and Extremes
Regarding sensitivity, species with broad thermal tolerance and higher thermal maximums appear to be less sensitive to dramatic changes in temperature and steady increases in average temperature than those with narrow thermal tolerance and lower thermal maximums (Jiguet et al., 2006; Jiguet et al., 2007). For example, during an extreme heatwave in France, bird species with small thermal ranges exhibited the sharpest decreases in population growth rate in correspondence with highest temperatures (Jiguet et al., 2006). Of course, climate change effects are not limited to changes in temperature, but also include increases in extreme weather events with most studies reporting negative effects on bird populations (Maxwell et al., 2019). Sensitivity of bird species and guilds to extreme weather will depend on timing and type of event (e.g., drought, flooding, cyclone, fire) and species-specific characteristics (e.g., habitat, foraging guild, life history traits).
Life history traits are also critical components of sensitivity to the impacts of climate change (Foden et al., 2013; Pacifici et al., 2015). Many bird species that are habitat specialists, have fast life histories, or are single brooded are currently in decline and climate change impacts can explain a significant portion of these declines (Reif, 2013), but habitat type and migration strategy have little impact on projected future responses to climate change (Langham et al., 2015). Habitat specialists are limited in their ability to adjust to climatic changes by immigrating to new habitats or exploiting novel resource bases, and competition induced by differential responses to these changes may favor generalist species (Warren et al., 2001; Clavel et al., 2011). Regarding life history speed, population growth rates of birds with fast life histories are more sensitive to climate-induced changes to demographic rates, such as growth, survival, and reproduction (Morris et al., 2008; Sekercioglu et al., 2012), and their shorter lifespans allow for fewer opportunities to learn and adjust to directional environmental changes (Jiguet et al., 2007), such as shifts in peak food availability (Visser et al., 2004). For example, blue tits can learn the best breeding date through experience of mistiming (Grieco et al., 2002); but for short-lived birds such as these, most breeders are young without previous experience to learn from (Visser et al., 2004). However, species with fast life histories are also frequently multi-brooded with larger clutches, traits that increase their adaptive capacity and thus, the probability of recovery from mortality associated with extreme weather events and other climate change effects (Angert et al., 2011; Sekercioglu et al., 2012). Thus, although species with fast life histories may be more sensitive to climate change impacts they may also recover more quickly.
3.2 Responses of Avian Species to Climate Change: Adaptive Capacity and Extinction
The combination of exposure and sensitivity to climate change is highly species-specific, and thus, species’ responses to climate change are predicted to be idiosyncratic (Williams et al., 2007). Faced with novel climatic conditions, a species can respond with tolerance in situ through behavioral and physiological adaptation or plasticity, shifts in phenology, habitat, and/or geographic distributions, and/or extinction if these mechanisms fail (Jackson and Overpeck, 2000; Moritz and Agudo, 2013). Tolerance depends in large part on the adaptive capacity of the species (Gallopin, 2006; Carpenter and Brock, 2008; Thurman et al., 2020), which is primarily determined by the propensity of a species for genetic adaptation, phenotypic plasticity, and dispersal (Beever et al., 2016).
3.2.1 Genetic Adaptation in Response to Climate Change
Genetic adaptation is predicted to be a critical determinant of species’ resilience to climate change (Vedder et al., 2013; Merila and Hendry, 2014; Meester et al., 2018). Direct evidence of genetic adaptation to climate change is scarce (Charmantier and Gienapp, 2014; Merila and Hendry, 2014), but has been observed in at least one wild bird species, the tawny owl, Strix aluco (Karell et al., 2011). The capacity to genetically track climate change is expected to depend on the species’ generation time, population size, genetic variation, and genetic correlations between traits (Meester et al., 2018). All else being equal, smaller birds with relatively short generation times, large populations, and large pools of genetic variation will have a higher capacity for rapid genetic response to climate change than those with long generation times and/or small populations (Vedder et al., 2013; Meester et al., 2018). Although adaptation to climate change effects may rescue some vulnerable species from extinction, adaptation also has costs at both the population and individual levels. For example, selection can reduce population sizes and genetic variation, decreasing capacity for response to future stressors and increasing risk of species extinction. Evolutionary trait change can also have costs associated with tradeoffs, pleiotropy, or linkage. Thus, while genetic adaptation may provide a buffer against climate change and is a critical component of adaptive capacity, it will not guarantee survival (Meester et al., 2018) and may contribute only weakly to rapid responses in multicellular organisms (Merila and Hendry, 2014).
3.2.2 Phenotypic Plasticity in Response to Climate Change
Rapid responses may be more strongly affected by phenotypic plasticity, which can occur over a shorter time period than genetic adaptation and is also predicted to be a critical determinant of species’ resilience to climate change (Vedder et al., 2013; Merila and Hendry, 2014; Meester et al., 2018). Selection can increase or decrease phenotypic plasticity through genetic change. Thus, phenotypic plasticity and genetic adaptation are intrinsically related, but often operate on different temporal scales (Meester et al., 2018), with phenotypic plasticity being the more rapid of the two. Phenotypic plasticity may be sufficient for some birds to persist in situ in the face of climate change (Vedder et al., 2013), although not sufficient for all organisms (e.g., trees, Duputié et al., 2015; ectotherms, Gunderson and Stillman, 2015). However, plastic responses will only remain adaptive if environmental cues remain reliable and informative. Climate change may disrupt the detection of cues, or their reliability through a variety of mechanisms. If cue reliability decreases, selection can drive accompanying decreases in plasticity, or mismatches with the optimum phenotype in a fluctuating environment could lead to population extinction (Bonamour et al., 2019). Evolution of adaptive phenotypically plastic shifts in optimal reproductive timing have been reported in at least one wild bird (the great tit, Parus major) (Nussey et al., 2005; Charmantier et al., 2008). Overwhelmingly, however, direct evidence of both phenotypic plasticity and microevolution in avian responses to the effects of climate change are lacking, despite widespread discussion of their importance (Charmantier and Gienapp, 2014).
3.2.3 Dispersal in Response to Climate Change
Dispersal propensity also influences the adaptive capacity of a species to a changing climate, by affecting its ability to track shifting climate envelopes (Leech and Crick, 2007; Beever et al., 2016). Current climate change is already producing observable systemic shifts in plant and animal phenology (Root et al., 2003; Crick, 2004; Root et al., 2005; Leech and Crick, 2007) and species distributions (e.g., Parmesan and Yohe, 2003; Hickling et al., 2006; Leech and Crick, 2007; Freeman et al., 2018), with range shifts averaging 6.1km per decade toward the poles for birds, butterflies, and alpine herbs (Parmesan and Yohe, 2003). At least some bird species are shifting their distributions and community compositions to track moving climate envelopes, although not quickly enough to match the rate of climate change (Devictor et al., 2008; Santangeli and Lehikoinen, 2017). Dispersal ability in response to climate change appears to be highly idiosyncratic across bird species, depending on region, climatic constraints, and species-specific traits (Brotons et al., 2019). Migratory species may have greater dispersal potential, because of their greater mobility (Sekercioglu et al., 2008), and indeed, migratory bird species are currently at a lower risk of extinction than sedentary species (Sekercioglu, 2007). However, migratory species may also be restricted in their capacity for phenological shifts in response to climate change (Leech and Crick, 2007). Because climatic changes are not spatially or temporally monotonic (Burkett et al., 2005), mismatches between the relative timing of cues for spring migration and periods of peak food availability on the breeding grounds may occur.
3.3 Methods to Predict Impacts of Climate Change on Avian Communities
Two approaches are predominantly used to predict the impacts of climate change on bird communities. The first utilizes single-species studies and extrapolates to communities, assuming that the response of single species can be applied across all species (Jenouvrier, 2013). Secondly, modeling methods can be used to predict impacts for entire communities (e.g., joint species distribution models) (Brotons et al., 2019). Various modeling techniques have been used to predict changes in community structure and function under future climate change scenarios, including space-for-time substitution and the community temperature index (CTI) approach. CTI reflects the dominance of warm- and cold-dwelling species in a community and has been used to investigate changes in community structure in relation to shifting climate (Devictor et al., 2008; Santangeli and Lehikoinen, 2017).
3.4 Impacts of Climate Change on Avian Communities
Climate constrains the fundamental niche of each species, and multivariate changes in climate are expected to result in community disassembly and reassembly because of species-specific shifts in distribution, abundance, and extinction (Figure 4) (Root et al., 2003; Williams et al., 2007). This conceptual framework is supported by evidence from the last deglaciation in which climates with no modern analogs produced plant associations and vegetation biomes with no modern analogs (Overpeck et al., 1992; Williams et al., 2001; Bush et al., 2004). Given that temperature is currently increasing at a rate greater than that of the last 2000 years (Masson-Delmotte et al., 2021), species extinction rates and community reorganization are predicted to increase accordingly (Pimm, 2009). Here, we examine the predicted and observed impacts of climate change on avian species richness, turnover (i.e., community reassembly), and function.
Many studies have examined the projected change in number of species (e.g., extinction rate) and changes to species’ geographic distributions under different climate scenarios, which can be used to estimate changes in species richness (e.g., Stralberg et al., 2015; Massimino et al., 2017). Most predict an increase in the proportion of high-temperature dwelling species (Devictor et al., 2008; Bowler et al., 2018), a reduction in species ranges, and a decrease in species richness (Brotons et al., 2019). However, the ability of birds to disperse to new suitable habitat is a crucial factor of these models, and assumptions of no dispersal or total dispersal can completely reverse the outcome regarding species richness (Barbet-Massin et al., 2009; Brotons et al., 2019). For vegetation specialists, immigration will be limited by the often much slower dispersal rates of associated vegetation (Root and Schneider, 2002). In contrast, vegetation generalists will have much greater dispersal ability. Where avian communities include both vegetation-specialists and generalists, immigration rate (Root and Schneider, 2002) and changes in abundances (Bowler et al., 2018) will differ greatly among community members and the potential for community turnover (i.e., disassembly and reassembly) is high (Figure 4) (Root and Schneider, 2002). Still other species may persist in place through phenotypic plasticity or genetic adaptation (Brotons et al., 2019), and where communities are composed of species with varying degrees of phenotypic plasticity and capacity for genetic adaptation, species turnover may also be high (Figure 4). Such independent shifts in species distributions are predicted to result in the rapid development of novel species assemblages, with potentially dramatic consequences for species interactions and ecosystem function (Brotons et al., 2019).
Bird assemblages fulfill critical sets of ecological functions for ecosystems, which can be altered following community disassembly and/or reassembly (Barbet-Massin and Jetz, 2015; Brotons et al., 2019). Under the IPCC’s Fourth Assessment Report emission scenario A2 (IPCC, 2007), avian assemblage functional structure is projected to change highly unevenly across space, sometimes resulting in substantial loss of functional diversity (Barbet-Massin and Jetz, 2015). Tropical and subtropical biomes are predicted to be especially hard hit by losses in functional diversity, while range expansions may compensate in high latitude regions (Barbet-Massin and Jetz, 2015). Dietary guilds are also projected to change heterogeneously under continued current greenhouse gas emissions, with decreases predominantly in primary and mixed consumer guilds, and increases in high-level consumers (Ko et al., 2014), although these projections are dependent on geographic region and assumptions regarding dispersal distance (Ko et al., 2014).
3.5 Resilience of Avian Communities to Climate Change: Resistance and Recovery
Most studies on the resilience of avian communities to climate change have focused on resistance over recovery, because (1) the window between extreme climatic events may become so short that recovery is unlikely, and (2) many climate disturbances will not be acute, providing little role for recovery (Côté and Darling, 2010). The resistance of avian communities will depend on the interactive effects of species vulnerability (i.e., sensitivity and exposure) and adaptive capacity (e.g., plasticity, genetic diversity, dispersal abilities), the ability of the vegetative community to shift phenology/geography in concert with the avian community, and the ability of the community to compensate for lost species and open niches (e.g., functional evenness, diversity, and redundancy) (Figure 4). The probability of community disassembly will naturally be higher where species’ vulnerability to climate change is also high, such as in poleward and tropical montane regions (Williams et al., 2007), thus species exposure and sensitivity are critical to determining community resiliency. Novel communities are predicted to develop in response to novel climates (Hobbs et al., 2006), which are projected to be concentrated in the tropics and subtropics (Williams et al., 2007). For species with high vulnerability, resistance to climate change may be low, but adaptive capacity and human intervention can provide a road to recovery. Appropriate adaptive and plastic responses may occur over a longer time scale than climate change (Jackson and Overpeck, 2000; Williams et al., 2007), leading to temporary community disassembly and decreases in species’ population sizes, followed by recovery once appropriate responses occur.
3.6 Complexities in Predicting Avian Community Resilience to Climate Change
A number of issues are in need of increased study to improve predictions of avian community response and resilience to climate change:
1. The majority of current research efforts on plant and animal responses to climate change focus on first order effects of global warming, especially temperature and precipitation changes. However, second and higher order effects also directly influence plant and animal community composition and function (Burkett et al., 2005). For example, as the global temperature rises, so too does sea level, leading to increases in coastal sediment and water salinity and selection for species with higher salinity tolerance (Burkett et al., 2005).
2. Climate change is often non-linear (Burkett et al., 2005) and acts on bird communities via both direct effects and indirect ecological cascade effects (Brotons et al., 2019). Increased research is needed on these indirect effects of climate change.
3. Finally, responses of species and communities to climate change will depend on local biotic and abiotic factors, including chemical pollution (Noyes and Lema, 2015), disease and pest outbreaks (Harvell et al., 2009), invasive species, land-use changes, and alterations in disturbance regimes (Brotons et al., 2019). Research on the interactions between these factors at local scales, and modeling and larger geographic scales is needed to accurately predict community response.
4 Urbanization and Climate Change Interact to Affect Avian Community Resilience
Many studies fail to consider the interactive effects of climate change and urban conversion (Felton et al., 2009) and disentangling their relative impacts and importance can be difficult (Hockey et al., 2011; Brotons et al., 2019). For many species, interactions between these two drivers of community change are expected to amplify the effects of each on temperature change and habitat loss, creating a deadly “anthropogenic cocktail” (Travis, 2003), although positive or balancing effects are also possible (Clavero et al., 2011).
4.1 Negative Interactive Effects of Urbanization and Climate Change: Temperature and Habitat Loss
Habitat loss, such as through urban conversion, can induce de facto climate change effects by driving relocation of species to less suitable habitats, causing subsequent niche restriction, increased susceptibility to climate change (White et al., 2014), and altering species assemblages. Communities that are forced into or choose to move into urban environments typically experience higher temperatures than those in undeveloped habitats, which can exacerbate the warming effects of climate change (Leveau et al., 2021), and induce species turnover/loss in the community. For example, urban and agricultural avian communities have proportionally more warm-adapted species than forest dwelling avian communities in the European Mediterranean (Clavero et al., 2011). We see this also in England, where avian communities have suffered a steep loss of cold-adapted species, in part due to thermal effects of both land-use change and climate change (Oliver et al., 2017). Anthropogenic land use changes can also decrease resilience to climate change by fragmenting or destroying remaining habitat for communities that are predicted to experience diminishing ranges under climate change (Jetz et al., 2007), and restricting the ability of communities to track moving climate envelopes (Roberts et al., 2019). These effects will be most evident for habitat specialist communities that are limited by vegetation dispersal. Coastal prairie communities in North America, for example, rely on unique sandy soils and appear to be restricted in shifting northward because of coastal landscape fragmentation and urban conversion (Roberts et al., 2019). Similarly, saltmarsh specialists could theoretically exhibit high resilience to rising sea levels by moving inland, but are often limited by anthropogenic coastal development, leading to saltmarsh reduction and simultaneous reduction of saltmarsh specialist communities (Thorne et al., 2012; Rosencranz et al., 2018).
4.2 Positive and Balancing Interactive Effects of Urbanization and Climate Change
Although the interactive effects of climate change and human induced disturbance such as urbanization are widely considered to have strongly negative effects for most avian communities, positive effects are possible. For example, evidence in coral reef communities suggests that anthropogenic disturbance can increase resilience to climate change by selecting for the most resilient phenotypes (Côté and Darling, 2010). Additionally, urban habitats may provide relatively stable refugia for urban exploiters to replace or subsidize habitat made unsuitable by climate change. Purple martins (Progne subis), for example, are increasing their use of urban areas for nesting and migratory staging (Bridge et al., 2016) and displaying phenotypically plastic migration timing in association with rising global temperatures (Fraser et al., 2019). The simultaneous effects of land use change and climate change can also be balancing; for example, in Danish avian communities, agricultural land use change decreases species abundances, while warmer winters increase species abundances (Bowler et al., 2018). Thus, land use changes can increase, decrease, or maintain the resilience of avian communities in the face of climate change (Clavero et al., 2011) in species-specific (Yalcin and Leroux, 2018) and habitat-specific manners (Kampichler et al., 2012).
5 Discussion
Ecological resilience has the potential to be a useful metric for assessing the current and predicted future state of an ecological community in response to disturbances, such as urbanization and climate change. Resilience has direct, oftentimes complex, interactions with other concepts such as adaptive capacity, the ecosystem stress-gradient hypothesis, and the intermediate disturbance hypothesis, which are in need of increased examination. In this review, we focus on avian communities because birds are sensitive to environmental change (Foden et al., 2013) and resilience studies at the scale of the ecological community are generally lacking (Allen et al., 2005; Fischer et al., 2007). Existing research suggests strong heterogeneity in the responses and resilience of bird communities to urban conversion and climate change, depending on a variety of species-specific and community-level mechanisms that interact to determine outcomes (Figure 1). Moving forward, we see three primary issues hindering forward momentum in this field: (1) implementation of standardized methods of quantifying resilience, (2) unknowns surrounding responses of communities to chronic disturbance across ecological (e.g., species to communities) and geographic (e.g., local to regional) scales, and (3) unknowns surrounding interactions between community resilience and socio-ecological factors. Considering these factors, we recommend five directions for future studies:
(1) Incorporation of both adaptive capacity and measures of functional diversity in definitions of community resilience. The development of a more comprehensive community resilience index would allow for synchronization across studies that would facilitate replication and meta-analyses.
(2) Measurement and/or modeling of both resistance and recovery in urbanization and climate change studies that focus on ecological communities. Recovery is especially understudied, specifically whether avian communities can reassemble and resume original ecosystem functions, and factors that facilitate recovery. This will most likely be accomplished through long-term ecological studies of communities.
(3) Multiscale and/or multitaxa studies including three-way interactions between plants, animals, and climate are needed. For example, coastal vegetation community structure is shifting in response to sea level rise and changes in salinity (Burkett et al., 2005), but we have little understanding of how coastal marsh bird communities are responding to climate change-associated drivers (Woodrey et al., 2012), or how these responses interact with changes in vegetation and competitive dynamics. Resilience measurements (incorporating both adaptive capacity and functional diversity) for both plant and animal species in a defined spatial extent alongside relevant climate and/or land-use variables may provide a more thorough understanding of the effects and interplay of these factors.
(4) Additional evaluations and predictions of ecological resilience that incorporate interactions between anthropogenic changes (e.g., climate change, urbanization, pollution). Section 4 of this review provides several examples of such studies.
(5) Increased understanding of the interactions between ecological resilience and measurable socio-ecological dynamics (e.g., regional socioeconomics, ecotourism, and human use of greenspace, see Ostrum, 2009 and McGinnis and Ostrom, 2014 for an extensive list of factors and socio-ecological system framework explanations) (Cote and Nightingale, 2012). For example, regional socio-economics can interact with species life histories to affect abundances in birds (Chaudhary and Gutzwiller, 2020). Research like this could be ecologically scaled up to the community level to make broader predictions of community resilience and recovery to anthropogenic change and socio-ecological dynamics, and/or geographically scaled down to provide specific predictions for local communities.
Author Contributions
MM spearheaded the development of the concepts presented in this review. MM, JG, and RA all contributed to literature review and manuscript preparation, with MM responsible for Section I (introduction), RA responsible for Section II (urbanization), JG responsible for Section III (climate change), RA and JG responsible for Section IV (urbanization and climate change interactions), and MM responsible for Section V (discussion). MM, RA, and JG all contributed equally to the editing process and to the development of figures and tables. MM acted as corresponding author and was responsible for the submission process. All authors contributed to the article and approved the submitted version.
Funding
Funding for this review was made possible by MM’s graduate research fellowship through the Rob & Bessie Welder Wildlife Foundation.
Conflict of Interest
The authors declare that the research was conducted in the absence of any commercial or financial relationships that could be construed as a potential conflict of interest.
Publisher’s Note
All claims expressed in this article are solely those of the authors and do not necessarily represent those of their affiliated organizations, or those of the publisher, the editors and the reviewers. Any product that may be evaluated in this article, or claim that may be made by its manufacturer, is not guaranteed or endorsed by the publisher.
Acknowledgments
We would like to thank the Rob and Bessie Welder Wildlife Foundation for fellowship support to MM, and the Texas A&M Office of Graduate Studies for fellowship support to RA. This manuscript is Rob and Bessie Welder Wildlife Foundation Contribution number 729. We would also like to thank Dr. Mary Ann Ottinger, Dr. J. Kenneth Grace, and members of the Grace Lab for their feedback on this manuscript, and the anonymous reviewers for their constructive comments and suggestions.
References
Alberti M., Marzluff J. M. (2004). Ecological Resilience in Urban Ecosystems: Linking Urban Patterns to Human and Ecological Functions. Urban Ecosyst. 7, 241–265. doi: 10.1023/B:UECO.0000044038.90173.c6
Allen C. R., Gunderson L., Johnson A. R. (2005). The Use of Discontinuities and Functional Groups to Assess Relative Resilience in Complex Systems. Ecosystems 8, 958–966. doi: 10.1007/s10021-005-0147-x
Allen A. P., O’Connor R. J. (2000). Hierarchical Correlates of Bird Assemblage Structure on Northeastern U.S.A. Lakes. Environ. Monit. Assess. 62, 15–37. doi: 10.1023/A:1006244932033
Angeler D. G., Fried-Petersen H. B., Allen C. R., Garmestani A., Twidwell D., Chuang W. C., et al. (2019). ““Adaptive Capacity in Ecosystems,”,” in Advances in Ecological Research (Cambridge, MA: Academic Press Inc), 1–24. doi: 10.1016/bs.aecr.2019.02.001
Angert A. L., Crozier L. G., Rissler L. J., Gilman S. E., Tewksbury J. J., Chunco A. J. (2011). Do Species’ Traits Predict Recent Shifts at Expanding Range Edges? Ecol. Lett. 14, 677–689. doi: 10.1111/j.1461-0248.2011.01620.x
Aronson M. F. J., la Sorte F. A., Nilon C. H., Katti M., Goddard M. A., Lepczyk C. A., et al. (2014). A Global Analysis of the Impacts of Urbanization on Bird and Plant Diversity Reveals Key Anthropogenic Drivers. Proc. R. Soc. B: Biol. Sci. 281, 1–8. doi: 10.1098/rspb.2013.3330
Banerjee A. K., Dewanji A. (2017). Native Exotic Relationships in Plant Communities: The Role of Exotic Dominance in Framing Community Composition. Ecol. Res. 32, 653–665. doi: 10.1007/s11284-017-1476-x
Barbet-Massin M., Jetz W. (2015). The Effect of Range Changes on the Functional Turnover, Structure and Diversity of Bird Assemblages Under Future Climate Scenarios. Global Change Biol. 21, 2917–2928. doi: 10.1111/gcb.12905
Barbet-Massin M., Walther B. A., Thuiller W., Rahbek C., Jiguet F. (2009). Potential Impacts of Climate Change on the Winter Distribution of Afro-Palaearctic Migrant Passerines. Biol. Lett. 5, 248–251. doi: 10.1098/rsbl.2008.0715
Beever E. A., O’Leary J., Mengelt C., West J. M., Julius S., Green N., et al. (2016). Improving Conservation Outcomes With a New Paradigm for Understanding Species’ Fundamental and Realized Adaptive Capacity. Conserv. Lett. 9, 131–137. doi: 10.1111/conl.12190
Bertness M. D., Callaway R. (1994). Positive Interactions in Communities. Trends Ecol. Evol. 9, 191–193. doi: 10.1016/0169-5347(94)90088-4
Biamonte E., Sandoval L., Chacón E., Barrantes G. (2011). Effect of Urbanization on the Avifauna in a Tropical Metropolitan Area. Landscape Ecol. 26, 183–194. doi: 10.1007/s10980-010-9564-0
Binley A. D., Proctor C. A., Pither R., Davis S. A., Bennett J. R. (2021). The Unrealized Potential of Community Science to Support Research on the Resilience of Protected Areas. Conserv. Sci. Pract. 3. doi: 10.1111/csp2.376
Biswas S. R., Mallik A. U. (2010). Disturbance Effects on Species Diversity and Functional Diversity in Riparian and Upland Plant Communities. Ecology 91, 28–35. doi: 10.1890/08-0887.1
Blair R. B. (1996). Land Use and Avian Species Diversity Along an Urban Gradient. Ecol. Appl. 6, 506–519. doi: 10.2307/2269387
Blewett C. M., Marzluff J. M. (2005). Effects of Urban Sprawl on Snags and the Abundance and Productivity of Cavity-Nesting Birds. Condor 107, 678–693. doi: 10.1093/condor/107.3.678
Blondel J. (2003). Guilds or Functional Groups: Does it Matter? Oikos 100, 223–231. doi: 10.1034/j.1600-0706.2003.12152.x
Bonamour S., Chevin L. M., Charmantier A., Teplitsky C. (2019). Phenotypic Plasticity in Response to Climate Change: The Importance of Cue Variation. Philos. Trans. R. Soc. B: Biol. Sci. 374. doi: 10.1098/rstb.2018.0178
Bonier F. (2012). Hormones in the City: Endocrine Ecology of Urban Birds. Hormones Behav. 61, 763–772. doi: 10.1016/j.yhbeh.2012.03.016
Bowler D. E., Heldbjerg H., Fox A. D., O’Hara R. B., Böhning-Gaese K. (2018). Disentangling the Effects of Multiple Environmental Drivers on Population Changes Within Communities. J. Anim. Ecol. 87, 1034–1045. doi: 10.1111/1365-2656.12829
Bregman T. P., Lees A. C., MacGregor H. E. A., Darski B., de Moura N. G., Aleixo A., et al. (2016). Using Avian Functional Traits to Assess the Impact of Land-Cover Change on Ecosystem Processes Linked to Resilience in Tropical Forests. Proc. R. Soc. B: Biol. Sci. 283. doi: 10.1098/rspb.2016.1289
Bremner J., Rogers S., Frid C. (2003). Assessing Functional Diversity in Marine Benthic Ecosystems: A Comparison of Approaches. Mar. Ecol. Prog. Ser. 254, 11–25. doi: 10.3354/meps254011
Bridge E. S., Pletschet S. M., Fagin T., Chilson P. B., Horton K. G., Broadfoot K. R., et al. (2016). Persistence and Habitat Associations of Purple Martin Roosts Quantified via Weather Surveillance Radar. Landscape Ecol. 31, 43–53. doi: 10.1007/s10980-015-0279-0
Brotons L., Herrando S., Jiguet F., Lehikoinen A. (2019). ““Bird Communities and Climate Change,”,” in Effects of Climate Change on Birds. Eds. Dunn P. O., Moller A. P. (Oxford, England: Oxford University Press), 221–235. doi: 10.1093/oso/9780198824268.003.0016
Brown L. M., Graham C. H. (2015). Demography, Traits and Vulnerability to Urbanization: Can We Make Generalizations? J. Appl. Ecol. 52, 1455–1464. doi: 10.1111/1365-2664.12521
Burkett V. R., Wilcox D. A., Stottlemyer R., Barrow W., Fagre D., Baron J., et al. (2005). Nonlinear Dynamics in Ecosystem Response to Climatic Change: Case Studies and Policy Implications. Ecol. Complexity 2, 357–394. doi: 10.1016/j.ecocom.2005.04.010
Bush M. B., de Oliveira P. E., Colinvaux P. A., Miller M. C., Moreno J. E. (2004). Amazonian Paleoecological Histories: One Hill, Three Watersheds. Palaeogeography Palaeoclimatol. Palaeoecol. 214, 359–393. doi: 10.1016/j.palaeo.2004.07.031
Carpenter S. R., Brock W. A. (2008). Adaptive Capacity and Traps. Ecology and Society 13(2):40. doi: 10.5751/ES-02716-130240
Chace J. F., Walsh J. J. (2006). Urban Effects on Native Avifauna: A Review. Landscape Urban Plann. 74, 46–69. doi: 10.1016/j.landurbplan.2004.08.007
Charmantier A., Gienapp P. (2014). Climate Change and Timing of Avian Breeding and Migration: Evolutionary Versus Plastic Changes. Evolutionary Appl. 7, 15–28. doi: 10.1111/eva.12126
Charmantier A., McCleery R. H., Cole L. R., Perrins C., Kruuk L. E. B., Sheldon, B. C (2008). Adaptive Phenotypic Plasticity in Response to Climate Change in a Wild Bird Population. Science 320, 800–803. doi: 10.1126/science.1157174
Chaudhary A., Gutzwiller K. J. (2020). Within-Scale and Cross-Scale Interaction Effects of Temperature and Human Socioeconomic Conditions on Avian Abundance. Avian Conserv. Ecol. 15, 1–64. doi: 10.5751/ACE-01622-150208
Christie F. J., Hochuli D. F. (2009). Responses of Wasp Communities to Urbanization: Effects on Community Resilience and Species Diversity. J. Insect Conserv. 13, 213–221. doi: 10.1007/s10841-008-9146-5
Clavel J., Julliard R., Devictor V. (2011). Worldwide Decline of Specialist Species: Toward a Global Functional Homogenization? Front. Ecol. Environ. 9, 222–228. doi: 10.1890/080216
Clavero M., Villero D., Brotons L. (2011). Climate Change or Land Use Dynamics: Do We Know What Climate Change Indicators Indicate? PLoS One 6. doi: 10.1371/journal.pone.0018581
Clergeau P., Croci S., Jokimäki J., Kaisanlahti-Jokimäki M.-L., Dinetti M. (2006). Avifauna Homogenisation by Urbanisation: Analysis at Different European Latitudes. Biol. Conserv. 127, 336–344. doi: 10.1016/j.biocon.2005.06.035
Clergeau P., Savard J.-P. L., Mennechez G., Falardeau G. (1998). Bird Abundance and Diversity Along an Urban-Rural Gradient: A Comparative Study Between Two Cities on Different Continents. Condor 100, 413–425. doi: 10.2307/1369707
Connell J. H. (1978). Diversity in Tropical Rain Forests and Coral Reefs. Science 199, 1302–1310. doi: 10.1126/science.199.4335.1302
Côté I. M., Darling E. S. (2010). Rethinking Ecosystem Resilience in the Face of Climate Change. PLoS Biol. 8. doi: 10.1371/journal.pbio.1000438
Cote M., Nightingale A. J. (2012). Resilience Thinking Meets Social Theory: Situating Social Change in Socio-Ecological Systems (SES) Research. Prog. Hum. Geogr. 36, 475–489. doi: 10.1177/0309132511425708
Crick H. Q. P. (2004). The Impact of Climate Change on Birds. Ibis 146, 48–56. doi: 10.1111/j.1474-919X.2004.00327.x
Croci S., Butet A., Clergeau P. (2008). Does Urbanization Filter Birds on the Basis of Their Biological Traits? Condor 110, 223–240. doi: 10.1525/cond.2008.8409
Devictor V., Julliard R., Couvet D., Jiguet F. (2008). Birds are Tracking Climate Warming, But Not Fast Enough. Proc. R. Soc. B: Biol. Sci. 275, 2743–2748. doi: 10.1098/rspb.2008.0878
Devictor V., Julliard R., Couvet D., Lee A., Jiguet F. (2007). Functional Homogenization Effect of Urbanization on Bird Communities. Conserv. Biol. 21, 741–751. doi: 10.1111/j.1523-1739.2007.00671.x
Doney S. C., Ruckelshaus M., Emmett Duffy J., Barry J. P., Chan F., English C. A., et al. (2012). Climate Change Impacts on Marine Ecosystems. Annu. Rev. Mar. Sci. 4, 11–37. doi: 10.1146/annurev-marine-041911-111611
Donnelly R., Marzluff J. M. (2006). Relative Importance of Habitat Quantity, Structure, and Spatial Pattern to Birds in Urbanizing Environments. Urban Ecosyst. 9, 99–117. doi: 10.1007/s11252-006-7904-2
Duputié A., Rutschmann A., Ronce O., Chuine I. (2015). Phenological Plasticity Will Not Help All Species Adapt to Climate Change. Global Change Biol. 21, 3062–3073. doi: 10.1111/gcb.12914
Emlen J. T. (1974). An Urban Bird Community in Tucson, Arizona: Derivation, Structure, Regulation. Condor 76, 184–197. doi: 10.2307/1366729
Engle N. L. (2011). Adaptive Capacity and its Assessment. Global Environ. Change 21, 647–656. doi: 10.1016/j.gloenvcha.2011.01.019
Evans K. L., Chamberlain D. E., Hatchwell B. J., Gregory R. D., Gaston K. J. (2011). What Makes an Urban Bird? Global Change Biol. 17, 32–44. doi: 10.1111/j.1365-2486.2010.02247.x
Evans B. S., Reitsma R., Hurlbert A. H., Marra P. P. (2018). Environmental Filtering of Avian Communities Along a Rural-to-Urban Gradient in Greater Washington, D.C., USA. Ecosphere 9, e02402. doi: 10.1002/ecs2.2402
Falk D. A., Watts A. C., Thode A. E. (2019). Scaling Ecological Resilience. Front. Ecol. Evol. 7. doi: 10.3389/fevo.2019.00275
Felton A., Fischer J., Lindenmayer D. B., Montague-Drake R., Lowe A. R., Saunders D., et al. (2009). Climate Change, Conservation and Management: An Assessment of the Peer-Reviewed Scientific Journal Literature. Biodiversity Conserv. 18, 2243–2253. doi: 10.1007/s10531-009-9652-0
Fischer J., Lindenmayer D. B., Blomberg S. P., Montague-Drake R., Felton A., Stein J. A. (2007). Functional Richness and Relative Resilience of Bird Communities in Regions With Different Land Use Intensities. Ecosystems 10, 964–974. doi: 10.1007/s10021-007-9071-6
Foden W. B., Butchart S. H. M., Stuart S. N., Vié J. C., Akçakaya H. R., Angulo A., et al. (2013). Identifying the World’s Most Climate Change Vulnerable Species: A Systematic Trait-Based Assessment of All Birds, Amphibians and Corals. PLoS One 8. doi: 10.1371/journal.pone.0065427
Foden W. B., Young B. E., Akçakaya H. R., Garcia R. A., Hoffmann A. A., Stein B. A., et al. (2019). Climate Change Vulnerability Assessment of Species. Wiley Interdiscip. Rev.: Climate Change 10. doi: 10.1002/wcc.551
Fontaine C., Dajoz I., Meriguet J., Loreau M. (2006). Functional Diversity of Plant-Pollinator Interaction Webs Enhances the Persistence of Plant Communities. PLoS Biol. 4, 0129–0135. doi: 10.1371/journal.pbio.0040001
Fraser K. C., Shave A., de Greef E., Siegrist J., Garroway C. J. (2019). Individual Variability in Migration Timing can Explain Long-Term, Population-Level Advances in a Songbird. Front. Ecol. Evol. 7. doi: 10.3389/fevo.2019.00324
Freeman B. G., Scholer M. N., Ruiz-Gutierrez V., Fitzpatrick J. W. (2018). Climate Change Causes Upslope Shifts and Mountaintop Extirpations in a Tropical Bird Community. Proc. Natl. Acad. Sci. U.S.A. 115, 11982–11987. doi: 10.1073/pnas.1804224115
Fremier A. K., Kiparsky M., Gmur S., Aycrigg J., Craig R. K., Svancara L. K., et al. (2015). A Riparian Conservation Network for Ecological Resilience. Biol. Conserv. 191, 29–37. doi: 10.1016/j.biocon.2015.06.029
Gallopín G. C. (2006). Linkages Between Vulnerability, Resilience, and Adaptive Capacity. Global Environ. Change 16, 293–303. doi: 10.1016/j.gloenvcha.2006.02.004
Garrett K. A., Dendy S. P., Frank E. E., Rouse M. N., Travers S. E. (2006). Climate Change Effects on Plant Disease: Genomes to Ecosystems. Annu. Rev. Phytopathol. 44, 489–509. doi: 10.1146/annurev.phyto.44.070505.143420
González-Oreja J. A. (2011). Birds of Different Biogeographic Origins Respond in Contrasting Ways to Urbanization. Biol. Conserv. 144, 234–242. doi: 10.1016/j.biocon.2010.08.021
Green D. M., Baker M. G. (2003). Urbanization Impacts on Habitat and Bird Communities in a Sonoran Desert Ecosystem. Landscape Urban Plann. 63, 225–239. doi: 10.1016/S0169-2046(02)00195-0
Grieco F., van Noordwijk A. J., Visser M. E. (2002). Evidence for the Effect of Learning on Timing of Reproduction in Blue Tits. Science 296, 136–138. doi: 10.1126/science.1068287
Grime J. P. (1973). Control of Species Density in Herbaceous Vegetation. J. Environ. Manage 1, 151–167.
Guetté A., Gaüzère P., Devictor V., Jiguet F., Godet L. (2017). Measuring the Synanthropy of Species and Communities to Monitor the Effects of Urbanization on Biodiversity. Ecol. Indic. 79, 139–154. doi: 10.1016/j.ecolind.2017.04.018
Gunderson L. H. (2000). Ecological Resilience—In Theory and Application. Annu. Rev. Ecol. Systematics 31, 425–439. doi: 10.1146/annurev.ecolsys.31.1.425
Gunderson A. R., Stillman J. H. (2015). Plasticity in Thermal Tolerance has Limited Potential to Buffer Ectotherms From Global Warming. Proc. R. Soc. B: Biol. Sci. 282. doi: 10.1098/rspb.2015.0401
Harrison G. W. (1979). Stability Under Environmental Stress: Resistance, Resilience, Persistence, and Variability. Am. Nat. 113, 659–669. doi: 10.1086/283424
Harvell D., Altizer S., Cattadori I. M., Harrington L., Weil E. (2009). Climate Change and Wildlife Diseases: When Does the Host Matter the Most? Ecology 90, 912–920. doi: 10.1890/08-0616.1
Hickling R., Roy D. B., Hill J. K., Fox R., Thomas C. D. (2006). The Distributions of a Wide Range of Taxonomic Groups are Expanding Polewards. Global Change Biol. 12, 450–455. doi: 10.1111/j.1365-2486.2006.01116.x
Hobbs R. J., Arico S., Aronson J., Baron J. S., Bridgewater P., Cramer V. A., et al. (2006). Novel Ecosystems: Theoretical and Management Aspects of the New Ecological World Order. Global Ecol. Biogeography 15, 1–7. doi: 10.1111/j.1466-822X.2006.00212.x
Hockey P. A. R., Wanless R. M., von Brandis R. (2011). Demographic Resilience of Territorial Island Birds to Extinction: The Flightless Aldabra Rail Dryolimnas (Cuvieri) Aldabranus as an Example. Ostrich 82, 1–9. doi: 10.2989/00306525.2011.556794
Holling C. S. (1973). Resilience and Stability of Ecological Systems. Annu. Rev. Ecology Evol. Systematics 4, 1–23. doi: 10.1146/annurev.es.04.110173.000245
Hooper D. U., Chapin F. S., Ewel J. J., Hector A., Inchausti P., Lavorel S., et al. (2005). Effects of Biodiversity on Ecosystem Functioning: A Consensus of Current Knowledge. Ecol. Monogr. 75, 3–35. doi: 10.1890/04-0922
Horn H. S. (1975). ”Markovian Properties of Forest Succession”. in Ecology and Evolution of Communities, eds. Cody M. L., Diamond J. M. (Cambridge, MA: Belknap Press) 196–211.
Hostetler M., Holling C. S. (2000). Detecting the Scales at Which Birds Respond to Structure in Urban Landscapes. Urban Ecosyst. 4, 25–54. doi: 10.1023/A:1009587719462
IPCC, Core Writing Team (2007). Climate Change 2007: Synthesis Report. Eds. Pachauri R. K., Reisinger A. (Geneva: IPCC).
Irizarry A. D., Collazo J. A., Vandermeer J., Perfecto I. (2021). Coffee Plantations, Hurricanes and Avian Resiliency: Insights From Occupancy, and Local Colonization and Extinction Rates in Puerto Rico. Global Ecol. Conserv. 27. doi: 10.1016/j.gecco.2021.e01579
Ives A. R., Carpenter S. R. (2007). Stability and Diversity of Ecosystems. Science 317, 58–62. doi: 10.1126/science.1133258
Jackson S. T., Overpeck J. T. (2000). Responses of Plant Populations and Communities to Environmental Changes of the Late Quaternary. Paleobiology 26, 194–220. doi: 10.1017/s0094837300026932
Jenouvrier S. (2013). Impacts of Climate Change on Avian Populations. Global Change Biol. 19, 2036–2057. doi: 10.1111/gcb.12195
Jetz W., Wilcove D. S., Dobson A. P. (2007). Projected Impacts of Climate and Land-Use Change on the Global Diversity of Birds. PLoS Biol. 5, e157. doi: 10.1371/journal.pbio.0050157
Jiguet F., Gadot A. S., Julliard R., Newson S. E., Couvet D. (2007). Climate Envelope, Life History Traits and the Resilience of Birds Facing Global Change. Global Change Biol. 13, 1672–1684. doi: 10.1111/j.1365-2486.2007.01386.x
Jiguet F., Julliard R., Thomas C. D., Dehorter O., Newson S. E., Couvet D. (2006). Thermal Range Predicts Bird Population Resilience to Extreme High Temperatures. Ecol. Lett. 9, 1321–1330. doi: 10.1111/j.1461-0248.2006.00986.x
Johnson A. B., Winker K. (2010). Short-Term Hurricane Impacts on a Neotropical Community of Marked Birds and Implications for Early- Stage Community Resilience. PLoS One 5. doi: 10.1371/journal.pone.0015109
Johnstone J. F., Allen C. D., Franklin J. F., Frelich L. E., Harvey B. J., Higuera P. E., et al. (2016). Changing Disturbance Regimes, Ecological Memory, and Forest Resilience. Front. Ecol. Environ. 14, 369–378. doi: 10.1002/fee.1311
Jokimäki J., Suhonen J. (1993). Effects of Urbanization on the Breeding Bird Species Richness in Finland: A Biogeographical Comparison. Ornis Fennica 70, 71.
Jung K., Kalko E. (2011). Adaptability and Vulnerability of High Flying Neotropical Aerial Insectivorous Bats to Urbanization. Diversity Distributions 17, 262–274. doi: 10.1111/j.1472-4642.2010.00738.x
Jung K., Threlfall C. G. (2018). Trait-Dependent Tolerance of Bats to Urbanization: A Global Meta-Analysis. Proc. R. Soc. B: Biol. Sci. 285, 20181222. doi: 10.1098/rspb.2018.1222
Kampichler C., van Turnhout C. A. M., Devictor V., van der Jeugd H. P. (2012). Large-Scale Changes in Community Composition: Determining Land Use and Climate Change Signals. PLoS One 7. doi: 10.1371/journal.pone.0035272
Kang W., Minor E. S., Park C.-R., Lee D. (2015). Effects of Habitat Structure, Human Disturbance, and Habitat Connectivity on Urban Forest Bird Communities. Urban Ecosyst. 18, 857–870. doi: 10.1007/s11252-014-0433-5
Karell P., Ahola K., Karstinen T., Valkama J., Brommer J. E. (2011). Climate Change Drives Microevolution in a Wild Bird. Nat. Commun. 2. doi: 10.1038/ncomms1213
Karp D. S., Ziv G., Zook J., Ehrlich P. R., Daily G. C. (2011). Resilience and Stability in Bird Guilds Across Tropical Countryside. Proc. Natl. Acad. Sci. U.S.A. 108, 21134–21139. doi: 10.1073/pnas.1118276108
Ko C. Y., Schmitz O. J., Barbet-Massin M., Jetz W. (2014). Dietary Guild Composition and Disaggregation of Avian Assemblages Under Climate Change. Global Change Biol. 20, 790–802. doi: 10.1111/gcb.12419
Langham G. M., Schuetz J. G., Distler T., Soykan C. U., Wilsey C. (2015). Conservation Status of North American Birds in the Face of Future Climate Change. PLoS One 10. doi: 10.1371/journal.pone.0135350
Larsen F. W., Bladt J., Balmford A., Rahbek C. (2012). Birds as Biodiversity Surrogates: Will Supplementing Birds With Other Taxa Improve Effectiveness? J. Appl. Ecol. 49, 349–356. doi: 10.1111/j.1365-2664.2011.02094.x
Lavorel S., Storkey J., Bardgett R. D., de Bello F., Berg M. P., le Roux X., et al. (2013). A Novel Framework for Linking Functional Diversity of Plants With Other Trophic Levels for the Quantification of Ecosystem Services. J. Vegetation Sci. 24 (5), 942–948. doi: 10.1111/jvs.12083
Leech D. I., Crick H. Q. P. (2007). Influence of Climate Change on the Abundance, Distribution and Phenology of Woodland Bird Species in Temperate Regions. Ibis 149, 128–145. doi: 10.1111/j.1474-919X.2007.00729.x
Lee M. B., Martin J. A. (2017). Avian Species and Functional Diversity in Agricultural Landscapes: Does Landscape Heterogeneity Matter? PLoS One 12. doi: 10.1371/journal.pone.0170540
Lepcyzyk C. A., Flather C. H., Radeloff V. C., Pidgeon A. M., Hammer R. B., Liu J. (2008). Human Impacts on Regional Avian Diversity and Abundance. Conserv. Biol. 22, 405–416. doi: 10.1111/j.1523-1739.2008.00881.x
Leveau L. M. (2018). Urbanization, Environmental Stabilization and Temporal Persistence of Bird Species: A View From Latin America. PeerJ 6, e6056. doi: 10.7717/peerj.6056
Leveau L. M., Jokimäki J., Kaisanlahti-Jokimäki M., Villalobos F. (2021). Urbanization Buffers Seasonal Change in Composition of Bird Communities: A Multi-Continental Meta-Analysis. J. Biogeography 48, 2391–2401. doi: 10.1111/jbi.14236
Leveau C. M., Leveau L. M. (2005). Avian Community Response to Urbanization in the Pampean Region, Argentina. Ornitol Neotrop 16, 503–510.
Leveau L. M., Leveau C. M. (2016). Does Urbanization Affect the Seasonal Dynamics of Bird Communities in Urban Parks? Urban Ecosyst. 19, 631–647. doi: 10.1007/s11252-016-0525-5
Leveau L. M., Leveau C. M., Villegas M., Cursach J. A., Suazo C. G. (2017). Bird Communities Along Urbanization Gradients: A Comparative Analysis Among Three Neotropical Cities. Ornitología Neotropical 28, 77–87.
Levine N. M., Zhang K., Longo M., Baccini A., Phillips O. L., Lewis S. L., et al. (2016). Ecosystem Heterogeneity Determines the Ecological Resilience of the Amazon to Climate Change. Proc. Natl. Acad. Sci. U.S.A. 113, 793–797. doi: 10.1073/pnas.1511344112
Lim H. C., Sodhi N. S. (2004). Responses of Avian Guilds to Urbanisation in a Tropical City. Landscape Urban Plann. 66, 199–215. doi: 10.1016/S0169-2046(03)00111-7
Litt A., Cord E., Fulbright T., Schuster G. L. (2014). Effects of Invasive Plants on Arthropods. Conserv. Biol. 28, 1532–1549. doi: 10.1111/cobi.12350
Lugo A. E., Carlo T. A., Wunderle J. M. (2012). Natural Mixing of Species: Novel Plant-Animal Communities on Caribbean Islands. Anim. Conserv. 15 (3), 233–241. doi: 10.1111/j.1469-1795.2012.00523.x
Møller A. P. (2019). Parallel Declines in Abundance of Insects and Insectivorous Birds in Denmark Over 22 Years. Ecol. Evol. 9, 6581–6587. doi: 10.1002/ece3.5236
Maldonado J. H., Moreno-Sánchez d. P. R. (2014). Estimating the Adaptive Capacity of Local Communities at Marine Protected Areas in Latin America: A Practical Approach. Ecol. Soc. 19. doi: 10.5751/ES-05962-190116
Marzluff J. M. (2001). ““Worldwide Urbanization and its Effects on Birds,”,” in Avian Ecology and Conservation in an Urbanizing World (Boston, MA: Springer US), 19–47. doi: 10.1007/978-1-4615-1531-9_2
Marzluff J. M., Ewing K. (2008). ““Restoration of Fragmented Landscapes for the Conservation of Birds: A General Framework and Specific Recommendations for Urbanizing Landscapes,”,” in Urban Ecology (Boston, MA: Springer US), 739–755. doi: 10.1007/978-0-387-73412-5_48
Mason N. W. H., Mouillot D., Lee W. G., Wilson J. B. (2005). Functional Richness, Functional Evenness and Functional Divergence: The Primary Components of Functional Diversity. Oikos 111, 112–118. doi: 10.1111/j.0030-1299.2005.13886.x
Massimino D., Johnston A., Gillings S., Jiguet F., Pearce-Higgins J. W. (2017). Projected Reductions in Climatic Suitability for Vulnerable British Birds. Climatic Change 145, 117–130. doi: 10.1007/s10584-017-2081-2
Masson-Delmotte V., Zhai A., Pirani A., Connors S. L., Péan C., Berger S., et al. (2021). ““IpcC 2021: Summary for Policy Makers,”,” in Climate Change 2021: The Physical Basis. Contribution of Working Group I to the Sixth Assessment Report of the Intergovernmental Panel on Climate Change (Cambridge, MA: Cambridge University Press).
Maxwell S. L., Butt N., Maron M., McAlpine C. A., Chapman S., Ullmann A., et al. (2019). Conservation Implications of Ecological Responses to Extreme Weather and Climate Events. Diversity Distributions 25, 613–625. doi: 10.1111/ddi.12878
May R. M. (1977). Thresholds and Breakpoints in Ecosystems With a Multiplicity of Stable States. Nature 269, 471–477. doi: 10.1038/269471a0
McCusker C. E., Ward M. P., Brawn J. D. (2010). Seasonal Responses of Avian Communities to Invasive Bush Honeysuckles (Lonicera Spp.). Biol. Invasions 12, 2459–2470. doi: 10.1007/s10530-009-9655-5
McGinnis M. D., Ostrom E. (2014). Social-Ecological System Framework: Initial Changes and Continuing Challenges. Ecol. Soc. 19. doi: 10.5751/ES-06387-190230
McKinney M. L. (2002). Urbanization, Biodiversity, and Conservation. BioScience 52, 883–890. doi: 10.1641/0006-3568(2002)052[0883:UBAC]2.0.CO;2
McKinney M. L. (2006). Urbanization as a Major Cause of Biotic Homogenization. Biol. Conserv. 127, 247–260. doi: 10.1016/j.biocon.2005.09.005
McKinney M. L. (2008). Effects of Urbanization on Species Richness: A Review of Plants and Animals. Urban Ecosyst. 11, 161–176. doi: 10.1007/s11252-007-0045-4
Meester L. D., Stoks R., Brans K. I. (2018). Genetic Adaptation as a Biological Buffer Against Climate Change: Potential and Limitations. Integr. Zool. 13, 372–391. doi: 10.1111/1749-4877.12298
Melles S., Glenn S., Martin K. (2003). Species–environment Associations Along a Multiscale Habitat Gradient. Conserv. Ecol. 7. doi: 10.5751/es-00478-070105
Merilä J., Hendry A. P. (2014). Climate Change, Adaptation, and Phenotypic Plasticity: The Problem and the Evidence. Evolutionary Appl. 7, 1–14. doi: 10.1111/eva.12137
Morelli F., Benedetti Y., Jerzak L., Kubecka J., Delgado J. D. (2020). Combining the Potential Resilience of Avian Communities With Climate Change Scenarios to Identify Areas of Conservation Concern. Ecol. Indic. 116, 106509. doi: 10.1016/j.ecolind.2020.106509
Moritz C., Agudo R. (2013). The Future of Species Under Climate Change: Resilience or Decline? Science 341, 504–508. doi: 10.1126/science.1237190
Morris W. F., Pfister C. A., Tuljapurkar S., Haridas C., Boggs C. L., Boyce M. S., et al. (2008). Longevity can Buffer Plant and Animal Populations Against Changing Climatic Variability. Ecology 89, 19–25. doi: 10.1890/07-0774.1
Mouchet M. A., Villéger S., Mason N. W. H., Mouillot D. (2010). Functional Diversity Measures: An Overview of Their Redundancy and Their Ability to Discriminate Community Assembly Rules. Funct. Ecol. 24, 867–876. doi: 10.1111/j.1365-2435.2010.01695.x
Myers N., Mittermeier R. A., Mittermeier C. G., da Fonseca G. A. B., Kent J. (2000). Biodiversity Hotspots for Conservation Priorities. Nature 403, 853–858. doi: 10.1038/35002501
Neate-Clegg M. H. C., Horns J. J., Adler F. R., Kemahlı Aytekin M.Ç., Şekercioğlu Ç.H. (2020). Monitoring the World’s Bird Populations With Community Science Data. Biol. Conserv. 248. doi: 10.1016/j.biocon.2020.108653
Nicotra A. B., Beever E. A., Robertson A. L., Hofmann G. E., O’Leary J. (2015). Assessing the Components of Adaptive Capacity to Improve Conservation and Management Efforts Under Global Change. Conserv. Biol. 29, 1268–1278. doi: 10.1111/cobi.12522
Northrup J. M., Rivers J. W., Yang Z., Betts M. G. (2019). Synergistic Effects of Climate and Land-Use Change Influence Broad-Scale Avian Population Declines. Global Change Biol. 25, 1561–1575. doi: 10.1111/gcb.14571
Noyes P. D., Lema S. C. (2015). Forecasting the Impacts of Chemical Pollution and Climate Change Interactions on the Health of Wildlife. Curr. Zool. 61, 669–689. doi: 10.1093/czoolo/61.4.669
Nussey D. H., Postma E., Gienapp P., Visser M. E. (2005). Selection on Heritable Phenotypic Plasticity in a Wild Bird Population. Science 310, 304–306. doi: 10.1126/science.1117004
Olden J. D., LeRoy Poff N., Douglas M. R., Douglas M. E., Fausch K. D. (2004). Ecological and Evolutionary Consequences of Biotic Homogenization. Trends Ecol. Evol. 19, 18–24. doi: 10.1016/j.tree.2003.09.010
Oliver T. H., Gillings S., Pearce-Higgins J. W., Brereton T., Crick H. Q. P., Duffield S. J., et al. (2017). Large Extents of Intensive Land Use Limit Community Reorganization During Climate Warming. Global Change Biol. 23, 2272–2283. doi: 10.1111/gcb.13587
Oppenheimer M., Campos M., Warren R., Birkmann J., Luber G., O’Neill B., et al. (2014). ““Emergent Risks and Key Vulnerabilities,”,” in Climate Change 2014 Impacts, Adaptation, and Vulnerability. Eds. Field C. B., Barros V. R., Dokken D. J., Mach K. J., Mastrandrea M. D. (Cambridge: Cambridge University Press), 1039–1100. doi: 10.1017/CBO9781107415379.024
Ortega-Álvarez R., MacGregor-Fors I. (2009). Living in the Big City: Effects of Urban Land-Use on Bird Community Structure, Diversity, and Composition. Landscape Urban Plann. 90, 189–195. doi: 10.1016/j.landurbplan.2008.11.003
Ostrom E. (2009). A General Framework for Analyzing Sustainability of Social-Ecological Systems. Science 325, 419–422. doi: 10.1126/science.1172133
Overpeck J. T., Webb R. S., Webb III, T. (1992). Mapping Eastern North American Vegetation Change of the Past 18 Ka: No-Analogs and the Future. Geology 20, 1071. doi: 10.1130/0091-7613(1992)020<1071:MENAVC>2.3.CO;2
Pacifici M., Foden W. B., Visconti P., Watson J. E. M., Butchart S. H. M., Kovacs K. M., et al. (2015). Assessing Species Vulnerability to Climate Change. Nat. Climate Change 5, 215–225. doi: 10.1038/nclimate2448
Parmesan C., Yohe G. (2003). A Globally Coherent Fingerprint of Climate Change Impacts Across Natural Systems. Nature 421, 37–42. doi: 10.1038/nature01286
Paton G. D., Shoffner A., Wilson A. M., Gagné S. A. (2019). The Traits That Predict the Magnitude and Spatial Scale of Forest Bird Responses to Urbanization Intensity. PLoS One 14, e0220120. doi: 10.1371/journal.pone.0220120
Petchey O. L., Gaston K. J. (2006). Functional Diversity: Back to Basics and Looking Forward. Ecol. Lett. 9, 741–758. doi: 10.1111/j.1461-0248.2006.00924.x
Pimm S. L. (2009). Climate Disruption and Biodiversity. Curr. Biol. 19, R595–R601. doi: 10.1016/j.cub.2009.05.055
Rega-Brodsky C. C., Aronson M. F. J., Piana M. R., Carpenter E. S., Hahs A. K., Herrera-Montes A., et al. (2022). Urban Biodiversity: State of the Science and Future Directions. Urban Ecosystems. doi: 10.1007/s11252-022-01207-w
Reif J. (2013). Long-Term Trends in Bird Populations: A Review of Patterns and Potential Drivers in North America and Europe. Acta Ornithologica 48, 1–16. doi: 10.3161/000164513x669955
Ricotta C., Bacaro G., Moretti M. (2014). A New Measure of Functional Evenness and Some of its Properties. PLoS One 9, e104060. doi: 10.1371/journal.pone.0104060
Riddell E. A., Iknayan K. J., Hargrove L., Tremor S., Patton J. L., Ramirez R., et al. (2021). Exposure to Climate Change Drives Stability or Collapse of Desert Mammal and Bird Communities. Science 371, 633–638. doi: 10.1126/science.abd4605
Roberts C. P., Allen C. R., Angeler D. G., Twidwell D. (2019). Shifting Avian Spatial Regimes in a Changing Climate. Nat. Climate Change 9, 562–566. doi: 10.1038/s41558-019-0517-6
Root T. L., MacMynowski D. P., Mastrandrea M. D., Schneider S. H. (2005). Human-Modified Temperatures Induce Species Changes: Joint Attribution. Proc. Natl. Acad. Sci. 102, 7465–7469. doi: 10.1073/pnas.0502286102
Root T. L., Price J. T., Hall K. R., Schneider S. H., Rosenzweig C., Pounds J. A. (2003). Fingerprints of Global Warming on Wild Animals and Plants. Nature 421, 57–60. doi: 10.1038/nature01333
Root T. L., Schneider S. H. (2002). ““Climate Change: Overview and Implications for Wildlife,”,” in Wildlife Responses to Climate Change: North American Case Studies. Eds. Schneider S. H., Root T. L. (Washington D.C: Island Press).
Rosencranz J. A., Thorne K. M., Buffington K. J., Takekawa J. Y., Hechinger R. F., Stewart T. E., et al. (2018). Sea-Level Rise, Habitat Loss, and Potential Extirpation of a Salt Marsh Specialist Bird in Urbanized Landscapes. Int. J. Business Innovation Res. 8, 8115–8125. doi: 10.1002/ece3.4196
Santangeli A., Lehikoinen A. (2017). Are Winter and Breeding Bird Communities Able to Track Rapid Climate Change? Lessons From the High North. Diversity Distributions 23, 308–316. doi: 10.1111/ddi.12529
Schaefer H. C., Jetz W., Böhning-Gaese K. (2008). Impact of Climate Change on Migratory Birds: Community Reassembly Versus Adaptation. Global Ecol. Biogeography 17, 38–49. doi: 10.1111/j.1466-8238.2007.00341.x
Scheffer M., Carpenter S. R., Dakos V., van Nes E. H. (2015). Generic Indicators of Ecological Resilience: Inferring the Chance of a Critical Transition. Annu. Rev. Ecology Evol. Systematics 46, 145–167. doi: 10.1146/annurev-ecolsys-112414-054242
Schleuter D., Daufresne M., Massol F., Argillier C. (2010). A User’s Guide to Functional Diversity Indices. Ecol. Monogr. 80, 469–484. doi: 10.1890/08-2225.1
Schneiberg I., Boscolo D., Devoto M., Marcilio-Silva V., Dalmaso C. A., Ribeiro J. W., et al. (2020). Urbanization Homogenizes the Interactions of Plant-Frugivore Bird Networks. Urban Ecosyst. 23, 457–470. doi: 10.1007/s11252-020-00927-1
Schneider S. C., Miller J. R. (2014). Response of Avian Communities to Invasive Vegetation in Urban Forest Fragments. Condor 116, 459–471. doi: 10.1650/CONDOR-13-009R1.1
Schneider F. D., Morsdorf F., Schmid B., Petchey O. L., Hueni A., Schimel D. S., et al. (2017). Mapping Functional Diversity From Remotely Sensed Morphological and Physiological Forest Traits. Nat. Commun. 8. doi: 10.1038/s41467-017-01530-3
Schütz C., Schulze C. H. (2015). Functional Diversity of Urban Bird Communities: Effects of Landscape Composition, Green Space Area and Vegetation Cover. Ecol. Evol. 5, 5230–5239. doi: 10.1002/ece3.1778
Scridel D., Brambilla M., Martin K., Lehikoinen A., Iemma A., Matteo A., et al. (2018). A Review and Meta-Analysis of the Effects of Climate Change on Holarctic Mountain and Upland Bird Populations. Ibis 160, 489–515. doi: 10.1111/ibi.12585
Sekercioglu C. H. (2006). Increasing Awareness of Avian Ecological Function. Trends Ecol. Evol. 21, 464–471. doi: 10.1016/j.tree.2006.05.007
Sekercioglu C. H. (2007). Conservation Ecology: Area Trumps Mobility in Fragment Bird Extinctions. Curr. Biol. 17, R283–R286. doi: 10.1016/j.cub.2007.02.019
Sekercioglu C. H. (2012). Bird Functional Diversity and Ecosystem Services in Tropical Forests, Agroforests and Agricultural Areas. J. Ornithol. 153 (1), 153–161. doi: 10.1007/s10336-012-0869-4
Sekercioglu C. H., Primack R. B., Wormworth J. (2012). The Effects of Climate Change on Tropical Birds. Biol. Conserv. 148, 1–18. doi: 10.1016/j.biocon.2011.10.019
Sekercioglu C. H., Schneider S. H., Fay J. P., Loarie S. R. (2008). Climate Change, Elevational Range Shifts, and Bird Extinctions. Conserv. Biol. 22, 140–150. doi: 10.1111/j.1523-1739.2007.00852.x
Selwood K. E., Thomson J. R., Clarke R. H., Mcgeoch M. A., mac Nally R. (2015). Resistance and Resilience of Terrestrial Birds in Drying Climates: Do Floodplains Provide Drought Refugia? Global Ecol. Biogeography 24, 838–848. doi: 10.1111/geb.12305
Seress G., Liker A. (2015). Habitat Urbanization and its Effects on Birds. Acta Zoologica Academiae Scientiarum Hungaricae 61, 373–408. doi: 10.17109/AZH.61.4.373.2015
Seto K. C., Fragkias M., Güneralp B., Reilly M. K. (2011). A Meta-Analysis of Global Urban Land Expansion. PLoS One 6, e23777. doi: 10.1371/journal.pone.0023777
Seto K. C., Guneralp B., Hutyra L. R. (2012). Global Forecasts of Urban Expansion to 2030 and Direct Impacts on Biodiversity and Carbon Pools. Proc. Natl. Acad. Sci. 109, 16083–16088. doi: 10.1073/pnas.1211658109
Siders A. R. (2019). Adaptive Capacity to Climate Change: A Synthesis of Concepts, Methods, and Findings in a Fragmented Field. WIREs Climate Change 10. doi: 10.1002/wcc.573
Sisk T. D., Battin J. (2002). Habitat Edges and Avian Ecology: Geographic Patterns and Insights for Western Landscapes. Stud. Avian Biol. 25, 30–48.
Smit B., Pilifosova O. v., Burton I., Challenger B., Huq S., Klein R. J. T., et al. (2001). ““Adaptation to Climate Change in the Context of Sustainable Development and Equity,”,” in Climate Change 2001: Impacts, Adaptation, and Vulnerability. Eds. McCarthy J. J., Canziani O. F., Leary N. A., Dokken D. J., White K. S. (Cambridge, UK: Press Syndicate of the University of Cambridge), 877–911.
Smit B., Wandel J. (2006). Adaptation, Adaptive Capacity and Vulnerability. Global Environ. Change 16, 282–292. doi: 10.1016/j.gloenvcha.2006.03.008
Sol D., González-Lagos C., Moreira D., Maspons J., Lapiedra O. (2014). Urbanisation Tolerance and the Loss of Avian Diversity. Ecol. Lett. 17, 942–950. doi: 10.1111/ele.12297
Song Y., Wang P., Li G., Zhou D. (2014). Relationships Between Functional Diversity and Ecosystem Functioning: A Review. Acta Ecologica Sin. 34 (2), 85–91. doi: 10.1016/j.chnaes.2014.01.001
Stralberg D., Matsuoka S. M., Hamann A., Bayne E. M., Sólymos P., Schmiegelow F. K. A., et al. (2015). Projecting Boreal Bird Responses to Climate Change: The Signal Exceeds the Noise. Ecol. Appl. 25, 52–69. doi: 10.1890/13-2289.1
Tai D., Chen C., Song Y., Tan X., Yang X., Wang Y. (2022). Ecological Traits and Landscape Characteristics Predicting Bird Sensitivity to Urbanization in City Parks. Basic Appl. Ecol. 58, 110–120. doi: 10.1016/j.baae.2021.12.004
Thorne K. M., Takekawa J. Y., Elliott-Fisk D. L. (2012). Ecological Effects of Climate Change on Salt Marsh Wildlife: A Case Study From a Highly Urbanized Estuary. J. Coast. Res. 28, 1477–1487. doi: 10.2112/JCOASTRES-D-11-00136.1
Thurman L. L., Stein B. A., Beever E. A., Foden W., Geange S. R., Green N., et al. (2020). Persist in Place or Shift in Space? Evaluating the Adaptive Capacity of Species to Climate Change. Front. Ecol. Environ. 18, 520–528. doi: 10.1002/fee.2253
Tilman D., Downing J. (1994). Biodiversity and Stability in Grasslands. Nature 367, 363–365. doi: 10.1038/367363a0
Tol R. S. J. (2009). The Economic Effects of Climate Change. J. Economic Perspect. 23, 29–51. doi: 10.1257/jep.23.2.29
Tomasevic J. A. (2017). Cavity-Nesting Bird interactionsiIn the Urban-Suburban Gradient. [PhD thesis]. [Seattle (WA)]: University of Washington.
Tomasevic J. A., Marzluff J. M. (2017). Cavity Nesting Birds Along an Urban-Wildland Gradient: Is Human Facilitation Structuring the Bird Community? Urban Ecosyst. 20 (2), 435–448. doi: 10.1007/s11252-016-0605-6
Travis J. M. J. (2003). Climate Change and Habitat Destruction: A Deadly Anthropogenic Cocktail. Proc. R. Soc. B: Biol. Sci. 270, 467–473. doi: 10.1098/rspb.2002.2246
van der Linden P., Marchini A., Dolbeth M., Patrício J., Veríssimo H., Marques J. C. (2016). The Performance of Trait-Based Indices in an Estuarine Environment. Ecol. Indic. 61, 378–389. doi: 10.1016/j.ecolind.2015.09.039
Vedder O., Bouwhuis S., Sheldon B. C. (2013). Quantitative Assessment of the Importance of Phenotypic Plasticity in Adaptation to Climate Change in Wild Bird Populations. PLoS Biol. 11. doi: 10.1371/journal.pbio.1001605
Villéger S., Mason N. W. H., Mouillot D. (2008). New Multidimensional Functional Diversity Indices for a Multifaceted Framework in Functional Ecology. Ecology 89, 2290–2301. doi: 10.1890/07-1206.1
Visser M. E., Both C., Lambrechts M. M. (2004). Global Climate Change Leads to Mistimed Avian Reproduction. Adv. Ecol. Res. 35, 89–110. doi: 10.1016/S0065-2504(04)35005-1
Warren M. S., Hill J. K., Thomas J. A., Asher J., Fox R., Huntley B., et al. (2001). Rapid Responses of British Butterflies to Opposing Forces of Climate and Habitat Change. Nature 414, 65–69. doi: 10.1038/35102054
White J. T.H., Collazo J. A., Dinsmore S. J., Llerandi-Román I. (2014). “Niche Restriction and Conservatism in a Neotropical Psittacine: The Case of the Puerto Rican Parrot,” in Habitat Loss: Causes, Effects on Biodiversity and Reduction Strategies (New York: Nova Science), 1–83.
Williams J. W., Jackson S. T., Kutzbach J. E. (2007). Projected Distributions of Novel and Disappearing Climates by 2100 AD. Proc. Natl. Acad. Sci. 104, 5738–5742. doi: 10.1073/pnas.0606292104
Williams J. W., Shuman B. N., Iii W. (2001). Dissimilarity Analyses of Late-Quaternary Vegetation and Climate in Eastern North America. Ecology 82, 3346–3362. doi: 10.1890/0012-9658(2001)082[3346:DAOLQV]2.0.CO;2
Woodrey M. S., Rush S. A., Cherry J. A., Nuse B. L., Cooper R. J., Lehmicke A. J. J. (2012). Understanding the Potential Impacts of Global Climate Change on Marsh Birds in the Gulf of Mexico Region. Wetlands 32, 35–49. doi: 10.1007/s13157-011-0264-6
Yalcin S., Leroux S. J. (2018). An Empirical Test of the Relative and Combined Effects of Land-Cover and Climate Change on Local Colonization and Extinction. Global Change Biol. 24, 3849–3861. doi: 10.1111/gcb.14169
Yap C. A. M., Sodhi N. S. (2004). Southeast Asian Invasive Birds: Ecology, Impact and Management. Ornithological Sci. 3, 57–67. doi: 10.2326/osj.3.57
Keywords: bird, adaptive capacity, vulnerability, biodiversity, conservation, resistance, recovery, land use change
Citation: McCloy MWD, Andringa RK and Grace JK (2022) Resilience of Avian Communities to Urbanization and Climate Change: an Integrative Review. Front. Conserv. Sci. 3:918873. doi: 10.3389/fcosc.2022.918873
Received: 12 April 2022; Accepted: 20 June 2022;
Published: 12 July 2022.
Edited by:
Daniel Steven Cooper, Natural History Museum of Los Angeles County, United StatesReviewed by:
Tanja M. Straka, Technical University of Berlin, GermanyThomas H. White, United States Fish and Wildlife Service, Puerto Rico
Copyright © 2022 McCloy, Andringa and Grace. This is an open-access article distributed under the terms of the Creative Commons Attribution License (CC BY). The use, distribution or reproduction in other forums is permitted, provided the original author(s) and the copyright owner(s) are credited and that the original publication in this journal is cited, in accordance with accepted academic practice. No use, distribution or reproduction is permitted which does not comply with these terms.
*Correspondence: Michael W. D. McCloy, mwdmccloy@tamu.edu