- Department of Biology, Virginia Commonwealth University, Richmond, VA, United States
Plants are particularly vulnerable to physical disturbance in low productivity areas, due to the high energetic cost of replacing lost tissue. In the eastern United States, serpentine savannahs are fragmented ecosystems with high concentrations of rare endemic plant species, low concentrations of soil nutrients, and severe deer overpopulation. This study assessed the recovery of flowering plants in a serpentine savannah when deer were excluded. Plant count, flower count, vegetative area, and plant height of 10 serpentine plant species were compared inside and outside of deer exclusion structures throughout an entire growing season. Throughout the growing season and across the plant community, deer exclusion consistently increased values for all plant response traits measured. Species that responded most strongly to deer exclusion included Arabis lyrata (Brassicaceae, the wide ranging lyre-leaf rockcress) and the serpentine near-endemic Symphyotrichum depauperatum (a serpentine aster known only in the eastern US). The slender knotweed, Polygonum tenue performed worse in excluded areas, which may indicate exclusion by more competitive species, or, alternatively, local scarcity. Overall, species richness did not increase in excluded plots, which may indicate that years of deer overbrowsing have depleted the local seed banks. While longer term studies might reveal different results, this study showed significant differences in vegetation response traits between excluded and unexcluded areas in just one year. We recommend that further restoration efforts should include reintroductions of locally extirpated species, in combination with deer exclusion to allow rare serpentine plant communities and their seedbanks to recover from intense overbrowsing pressure.
Introduction
Severe ecosystem disturbances including species invasion, anthropogenic land use change, and extinction of carnivores have resulted in dramatic changes in ecosystem composition and losses of global biodiversity (Rambo and Faeth, 1999; Myers et al., 2000; Raghubanshi and Tripathi, 2009; Strong and Frank, 2010). Especially when disturbances directly impact the abundance and distribution of vegetation, their effects can propagate across ecosystems, reducing foodweb stability and resilience (Nakamura et al., 2000; Raghubanshi and Tripathi, 2009; Strong and Frank, 2010; Ripple and Betschta, 2012). Understanding both the scale and cascading impacts of disturbance across ecosystems, and exploring their mitigation is imperative to prevent the loss of unique species (Raghubanshi and Tripathi, 2009). Species loss, even of uncommon and weakly interacting species, can degrade an ecosystem's resiliency to disturbance and invasion, increase variability of ecosystem processes, and result in the loss of additional unique species (O'Gorman et al., 2010).
In the past few decades, white-tailed deer (Odocoileus virginianus) populations have expanded across the eastern United States, due to local extinction of natural predators, landscape and ecological changes, reduction in hunting pressure, and the ability of deer to adapt to human-modified ecosystems (Côté et al., 2004; Latham et al., 2005; Creacy, 2006; Rawinski, 2008; Strong and Frank, 2010). At high densities, deer alter community dynamics and ecosystem processes through trampling and selective browsing, especially of reproductive plant parts (Augustine and Frelich, 1998; Latham et al., 2005; Geddes and Mopper, 2006; Averill et al., 2017). Strong browsing pressure limits the reproductive potential of preferred plants (Nakahama et al., 2020) and can reduce the density and diversity of herbaceous plants over time, causing local extinctions (Augustine and Frelich, 1998; Strong and Frank, 2010). As a result, white-tailed deer are considered a keystone species, because their browsing has such strong direct and indirect impacts on the ecosystems they live in (Rooney, 2001; Rooney and Waller, 2003).
The effects of deer browsing on plant communities may be magnified in low productivity areas (Olff and Ritchie, 1998; Proulx and Mazumder, 1998; Bakker et al., 2006) where low soil nutrient availability increases the energetic costs of replacing lost plant tissue (Janzen, 1974). This may be especially true for serpentine barrens, which are globally rare, heavily fragmented habitats that occur on resource-poor soils derived from ultramafic rock (Latham and McGeehin, 2012). Due to the increased cost of regrowth in resource-poor environments, serpentine plant species have reduced resilience to herbivory (Coley et al., 1985; Lau et al., 2008; Strauss and Boyd, 2011).
In the eastern United States, serpentine barrens are biodiversity hotspots for rare endemic serpentine plant species, as well as many locally or regionally endangered species (Rajakaruna et al., 2009; Flinn et al., 2017). In recent decades, these ecosystems have been overwhelmed by increasing browsing pressure from white tailed deer (Prince et al., 2004; Floyd, 2006). Several endangered flowering forbs, including downy lobelia (Lobelia puberula) and white heath aster (Symphyotrichum ericoides) have been locally extirpated from eastern serpentine barrens in the past decades (Latham and McGeehin, 2012). While it is unclear that deer overbrowsing is directly responsible for these declines, it is clear that rare and endangered species highly preferred by deer are vulnerable. Latham and McGeehin (2012) suggest that any attempt to restore rare eastern serpentine grasslands without limiting the deer population is a “lesson in futility” and “the only practical method” to protect vulnerable endemic species is the complete exclusion of deer.
Herbivore exclusion, particularly deer exclusion, has been a common technique to restore rare plant communities worldwide (Rambo and Faeth, 1999; Ruhren and Handel, 2003; Stephan et al., 2017). This method of restoration has been widely successful at increasing herbaceous plant diversity for ecosystems that were overbrowsed by deer (Ruhren and Handel, 2003; Stephan et al., 2017; Nakahama et al., 2020). However, herbivore exclusion as a method of restoration may not always improve plant diversity. The efficacy of restoration by herbivore exclusion is dependent on the length and severity of herbivory disturbance (Nakahama et al., 2020). Areas that have experienced chronic browsing disturbance for decades may have depleted seedbanks and dwindling soil nutrients, and may not recover from disturbance, even when herbivores are excluded. Additionally, certain species, particularly those with energetically costly adaptations, are sensitive to disturbance and may not recover when herbivores are excluded (Tamura, 2010). Alternatively, herbivore exclusion may decrease biodiversity (Mortenson et al., 2017). If deer preferentially feed on highly competitive or exotic species, browsing may provide less competitive species an advantage. In this scenario, herbivore removal could destabilize plant communities by allowing dominance of one or a few competitive plant species (Mortenson et al., 2017). This may be particularly true in low productivity environments, characterized by plants with limited competitive abilities.
In order to assess serpentine ecosystem recovery from deer browsing, we deployed deer exclusion plots to quantify differences in flowering plant community composition and key response traits (total plant number per plot, total flower number per plot, average blossom area per plot, average vegetative area per plot, and average height per plant) between areas of deer presence and absence. Response traits describe how plants respond to changes in their environments (Violle et al., 2007). Plant community response to deer exclusion was assessed over the course of one growing season. In a recovery scenario, we expected increases in diversity and response trait values where deer were excluded. In a depleted seedbank scenario, we expected no difference between treatments. In a scenario where deer played a role in increasing plant diversity through indirect effects on plant competition, we expected lower plant diversity in excluded areas, and the proliferation and dominance of one or a few plant species. This research was conducted in an eastern serpentine savannah, a globally rare and highly fragmented habitat for which restoration from deer overbrowsing has not been attempted.
Methods
Study Site
Serpentine savannas, defined as serpentine areas with 10–25% tree cover (Flinn et al., 2017), are considered to have an especially high conservation value, and house nearly all of the rare endemic plant and pollinator species characteristic of serpentine barrens (Floyd, 2006; Smith, 2010; Flinn et al., 2017). Up to 96% of eastern serpentine savannahs are found in the Northern Piedmont of Maryland and Pennsylvania, covering only 3,400 acres in total (about 5 square miles) (Latham and McGeehin, 2012). Located in suburban Baltimore, Soldiers Delight Natural Environmental Area includes the largest remaining serpentine savannah ecosystem in the eastern United States, covering 1,900 acres (Tyndall, 1992; Tyndall and Hull, 1999; Floyd, 2006).
The soil at Soldiers Delight is a sandy loam with a low concentration of calcium and a high concentration of magnesium and nickel (Tyndall, 2012). Dominant savannah species include true prairie grasses, such as little bluestem (Schizachyrium scoparium) and Indian grass (Sorghastrum nutans) (Tyndall, 1994; Tyndall and Hull, 1999). Over 39 rare, threatened, or endangered plant species also occur at Soldiers Delight, including the fringed gentian flower (Gentianopsis crinita), serpentine aster (Symphyotrichum depauperatum), serpentine chickweed (Cerastium arvense L. var. villosum) and the ten lobe false foxglove (Agalinis decemloba) (Tyndall, 1994, 2005; Tyndall and Hull, 1999; Floyd, 2006; Flinn et al., 2017). White tailed deer density around Soldiers Delight has increased dramatically (from 10/km2 to 40/km2) since the 1970s (Porter, 1991; Floyd, 2006; Walters et al., 2016), and has had an uncharacterized impact on the unique flora and fauna of this rare ecosystem.
Deer Exclusion Plot Construction
Exclusion plots are frequently used to restore plant communities that have experienced overbrowsing pressures (Rambo and Faeth, 1999; Pasari et al., 2014; Averill et al., 2017; Stephan et al., 2017; Nakahama et al., 2020). We identified two sites 0.27 km apart within Soldiers Delight with similar physical, topographic, and edaphic characteristics (39.41 °N, −76.83 °W). Between both sites, a total of 10 plots (five fenced plots and five adjacent unfenced plots) were established. Plots were established February 2019. Due to shallow soil depth at the study site, exclosures were constructed using 19-liter buckets of cement anchoring 2 m tall wooden posts, and 2 m tall polypropylene fence with 5 cm mesh openings, to permit movement of pollinators and birds. Two holes (10 × 12 cm) were cut in the bottom of each 5 m length of fence in order to allow small mammals access (a total of eight holes per exclusion structure). Additionally, 1 cm thick wire was wrapped around the bottom of each structure to prevent fawns from accessing the plots.
Plant Sampling
In ecosystems with low diversity, measuring changes in species abundance is more informative than measuring biodiversity alone (Mendenhall et al., 2011; Murphy and Romanuk, 2014), thus both were quantified here. To quantify the effect of deer exclusion on the diversity and abundance of flowering plant species, monthly plant inventories were conducted. Differences in plant response traits between excluded and unexcluded areas can indicate the efficacy of restoration treatments and their comprehensiveness across plant communities (Sandel et al., 2011; Piqueray et al., 2015). At the center of experimental plot, two 1 × 1 m quadrats were established diagonally from one another, leaving a minimum distance of 1.5 m to the fence. Percent cover of plant species was recorded for each sampling event. Additionally, for each plot, the number of flowering plants, the number of flowers on each plant, and the height of each plant was recorded. Plant height and percent cover of plants in grassland ecosystems can indicate competitive ability to procure water, sunlight, and soil nutrients (Craine and Dybzinski, 2013; Funk et al., 2016). Abundance and density of flowering plants and flowers on plants is positively correlated with the number of visitations by pollinators, and may represent a greater chance of reproductive success (Vasquez et al., 2009; Peuker et al., 2020). Sampling occurred every 2–3 weeks for a total of ten sampling events (two sampling sessions per season) in order to capture peak bloom for all species present.
Statistical Analyses
To quantify patterns in community composition data over the growing season, we used a non-metric multidimensional scaling (NMDS) ordination to divide sampling points throughout the year into distinct season groups based on their unique plant community compositions (NMDS; McCune and Grace, 2002). The input data were total counts for plant species that were flowering at each sampling date that were relativized prior to NMDS analysis. The NMDS was performed using the package vegan in R, using the Bray-Curtis dissimilarity index as a distance measure (Oksanen et al., 2015). Based on their distinct groupings, sampling dates were divided into five significantly different seasons: spring, early summer, mid-summer, late summer, and fall [F(4, 9) = 18.535 p = 0.005, dimensions = 2, stress = 0.04]. Community composition differences between these seasons were compared using a permutational multivariate analysis of variance (PERMANOVA).
Counts of flowering plant number per plot, total flower number per plot, and flower number per plant for each species were compared between control and exclusion treatments for each unique combination of date, plot, and treatment using a negative binomial regression with repeated measures. Treatment and season were treated as fixed explanatory variables. All negative binomial regressions were run using the package MASS in R (Venables and Ripley, 2002). To assess differences in plant diversity between treatments, the package vegan was used to calculate Shannon's Diversity Index for each unique combination of date, plot, and treatment (Oksanen et al., 2015). Diversity values were compared between treatments over time using linear models.
Mean herbaceous plant cover, and mean plant height per species for each unique combination of date, plot, and treatment were analyzed using general linear mixed effects model with repeated measures (GLMMs). In all models, “plot” and “quadrat” were treated as random factors. The GLMMs were validated visually (Zuur et al., 2010) leading to Box-Cox transformations for plant cover and plant height variables. Diversity values were compared between treatments over time using GLMMs. All GLMMs were run using the package lme4 in R (Bates et al., 2015), and best models were determined based on AIC values.
To determine which flowering species were driving differences in flower counts between exclusion and control plots, average values for each species in control and exclusion plots were calculated for five plant functional responses: total plant number per plot, total flower number per plot, average blossom area per plot, average vegetative area per plot, and average height per plant. To determine the magnitude of difference between exclusion and control plant responses, the ratio of the response between exclusion and control for each species and each functional response was calculated. Responses were weighted equally by adding all ratio values for each vegetation response and dividing each individual ratio value by the sum. An NMDS was performed comparing ratios of plant functional responses between exclusion and control plots across species, to determine which species showed the greatest differences in plant response between control and exclusion treatments. NMDS analysis was performed using the package vegan in R, using the Euclidean distance as a dissimilarity index (Oksanen et al., 2015).
To assess community response to exclusion treatments, community weighted means (CWMs, Garnier et al., 2004; Violle et al., 2007) were calculated for four plant functional responses: total flower number per plot and number of flowers per plant (metrics of reproductive capacity), and average vegetative area per plot and average height per plant (metrics of competition). An NMDS was performed comparing plant response trait responses on the CWMs between exclusion and control plots using the package vegan in R, using the Euclidean distance as a dissimilarity index (Oksanen et al., 2015). The functional trait responses between control and exclusion treatments were compared using a PERMANOVA.
Results
The total number of flowering plants on the landscape fluctuated over the course of the growing season, peaking during the late summer (Figure 1). NMDS was used to assess how plant community functional responses differed between control and exclusion treatments. Both the magnitude and variability of all plant response traits were higher in the exclusion treatment compared to the control treatment (dimensions = 2, stress = 0.02; Figure 2). Permanova results showed that plant functional responses were significantly different in exclusion plots relative to control plots [F(1, 8) = 5.6, p = 0.02], and pairwise analysis showed that all plant response variables differed significantly between the two treatments (Supplementary Table 1).
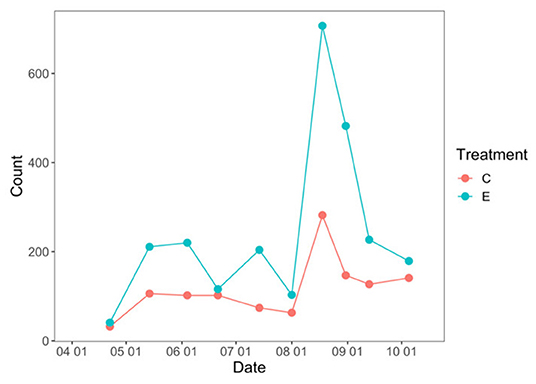
Figure 1. Total flowering plant count between deer exclusion (E) and control plots (C) over the course of the growing season.
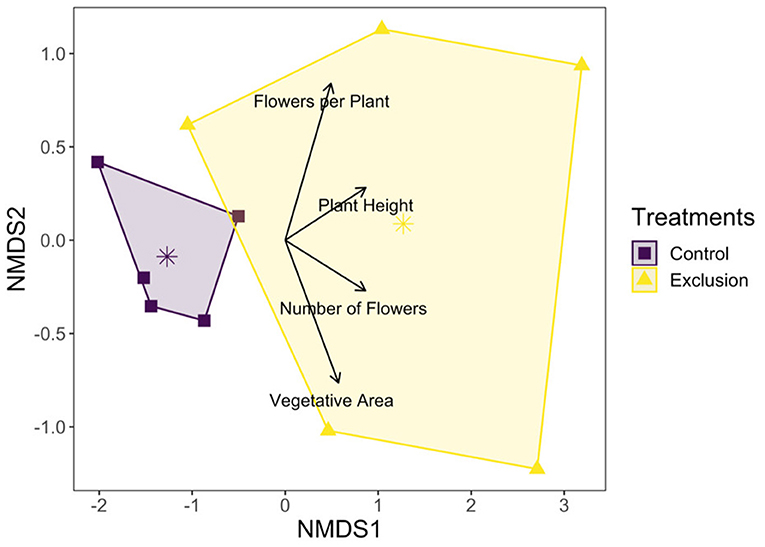
Figure 2. NMDS plot showing net vegetation response based on community weighted means of plant response variables to deer presence and absence. Points represent net average community response values for each control and exclusion plot, and arrow length reflects the relative contribution of each plant response variable to the magnitude of community response. The larger area for the exclusion treatment polygon reflects greater overall variability in plant response values compared to the control treatment.
Flowering plant count in exclusion plots was consistently greater than in control plots ( = 12.79, p < 0.001). Deer presence had a significant negative impact on all measured plant response variables: species and floral counts, vegetative area per plot, number of flowers per plant, and average plant height per species (Table 1, Supplementary Table 2). Based on NMDS analysis, flower species that responded most positively to deer exclusion were lyrate rockcress (Arabis lyrata) and serpentine aster (S. depauperatum) (dimensions = 2, stress <0.001; Figure 3, Supplementary Table 3). Shannon's Diversity did not vary between control plots and exclusion plots [F(1, 40) = 0.38, p = 0.82].
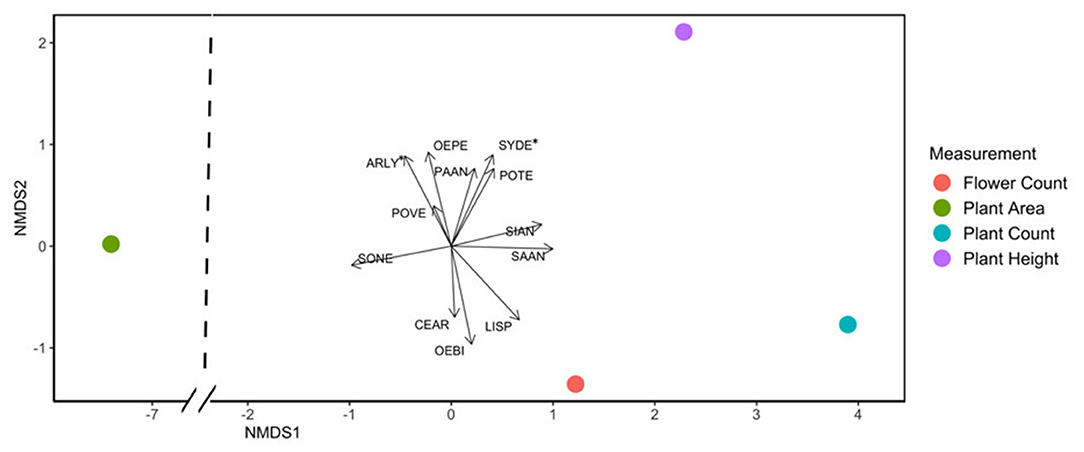
Figure 3. NMDS plot showing that the species Arabis lyrata (ARLY) and Symphyotrichum depauperatum (SYDE) were most affected by deer presence, using an integrated combination of all plant response variables. Points represent average plant response values across species. Length of arrows corresponds to magnitude of the ratio in species response between disturbed and control plots, with longer length indicating greater differences between control and exclusion. Asterisks indicate p values <0.05.
Discussion
This study examined serpentine plant community recovery after one year of deer exclusion. If deer exclusion promoted community recovery, we predicted excluded plots would exhibit increased diversity and response trait values in excluded plots. If deer browsing caused an increase in diversity by limiting the abundance of competitive species, we expected to find lower diversity in excluded areas, the proliferation of one or a few plant species and their trait values, and a decrease in trait values for all other species. If however, decades of deer browsing resulted in irreversible degradation of the seedbank, we expected no changes between treatments. Rather than finding strong support for one scenario, after only one year of data collection, we found evidence to support all three.
No differences in overall herbaceous plant Shannon's Diversity Index were observed between control and exclusion treatments. These results could be explained by a number of factors, including exclusion of annual seedlings by established perennials, changes in soil composition due to deer trampling, or a depleted seedbank at Soldiers Delight. Tamura (2010) reports that if deer overbrowsing remains unchecked for over 10 years, recovery may be a long and difficult process. Deer have existed at high densities (approximately 40/km2 since the 1990s) around Soldiers Delight for several decades (Porter, 1991; Walters et al., 2016). Serpentine barrens are already vulnerable to herbivory pressures, due to their low plant productivity, low plant species diversity, and nutrient poor soils (Safford and Mallek, 2011; Strauss and Boyd, 2011; Flinn et al., 2017). As a result, chronic browsing pressure could have driven preferentially consumed species to extinction (Anderson et al., 2001; Latham and McGeehin, 2012). These results are consistent with studies of Midwest prairies, that have attributed low floral diversity to decades of intense deer browsing (Anderson et al., 2001). However, this research was only conducted for one year, and a continuation of this study might reveal different results.
Although plant diversity was unaffected by deer exclusion, flowering plant functional responses and abundances dramatically increased in exclusion plots. Throughout the growing season (Figure 2) and across the plant community (Figure 3), deer exclusion consistently increased values for all plant response traits measured (Table 1), including plant and flower count, vegetative area, flowers per plant, and plant height for herbaceous species. In other words, deer exclusion resulted in plant communities with traits indicative of greater competitive (increased height and percent cover) and reproductive ability (increased species and floral counts). These results are consistent with numerous studies that found deer exclusion dramatically increases the abundance and response trait values of flowering plants in prairie (Anderson et al., 2017) and temperate forest ecosystems (Ruhren and Handel, 2003; Wang and Mopper, 2008; Sakata and Yamasaki, 2015; Nakahama et al., 2020). Stephan et al. (2017) suggests that in addition to increases in height, size and abundance, plants can allocate more resources to defense against other herbivores in the absence of significant biomass loss from deer.
Although deer exclusion caused higher response values at the community level, not all plant species responded in the same way. Species most impacted by deer herbivory included A. lyrata and S. depauperatum. Exclusion had a strong positive impact on the height of S. depauperatum plants, and both the height and plant area of A. lyrata plants. At our sites, both species were perennials with long flowering times of up to 2 months. Plant area increased most for S. rugosa, and flower count increased most for O. biennis (Figure 3). The only species that did not follow the community trend of increasing abundance or trait values in the absence of deer was slender knotweed (Polygonum tenue). In control plots this species grew taller and was more abundant. The unexpected results from this species may reflect overall plant scarcity, or competitive exclusion of P. tenue when deer were absent. During the late summer when P. tenue was flowering, Liatris spicata was hyper-abundant in exclusion plots, at a density as high as 41 flowers per square meter. In control plots, L. spicata was never more abundant than 11 flowers per square meter. The dominance of L. spicata in exclusion plots may result in limited nutrients or space for P. tenue to thrive.
Serpentine barrens are considered to be zones of high conservation concern (Latham and McGeehin, 2012; Flinn et al., 2017). Of the flowering plants observed in this study, L. spicata, C. arvense and S. depauperatum are ranked as critically imperiled and highly rare in the state of Maryland (Maryland Natural Heritage Program, 2021). S. depauperatum is additionally classified as critically endangered and near-endemic to eastern serpentine barrens (Rajakaruna et al., 2009; Latham and McGeehin, 2012; Maryland Natural Heritage Program, 2021). If left unprotected, eastern serpentine grasslands may lose these unique rare species, and be permanently degraded by continued intense browsing pressure (Latham and McGeehin, 2012; Flinn et al., 2017). Although plant diversity did not increase in exclusion plots, and P. tenue performed worse in exclusion plots than control plots, we observed dramatic recovery in the abundance and response traits of rare imperiled species in a single growing season. If fences were maintained and vegetation was monitored over additional years, we expect the herbaceous plant community would continue to recover biomass, increase reproductive potential, and possibly increase species diversity. However, due to the long legacy of deer overbrowsing, and possible seed bank depletion, we recommend that effective restoration include reintroductions of extirpated species, and additional planting of competitively excluded species, in combination with deer exclusion (Ruhren and Handel, 2003; Nakahama et al., 2020). Constructing fences to exclude deer may be a simple yet effective strategy to protect endemic species and restore ecosystem functionality to historically degraded eastern serpentine habitats.
Data Availability Statement
The raw data supporting the conclusions of this article will be made available by the authors, without undue reservation.
Author Contributions
AR contributed to the conceptualization, methodology, investigation, formal analysis, visualization, and writing of this manuscript. CH contributed to the conceptualization, methodology, reviewing and editing, and funding acquisition for this manuscript. Both authors contributed to the article and approved the submitted version.
Conflict of Interest
The authors declare that the research was conducted in the absence of any commercial or financial relationships that could be construed as a potential conflict of interest.
Publisher's Note
All claims expressed in this article are solely those of the authors and do not necessarily represent those of their affiliated organizations, or those of the publisher, the editors and the reviewers. Any product that may be evaluated in this article, or claim that may be made by its manufacturer, is not guaranteed or endorsed by the publisher.
Acknowledgments
We thank Emelia Kusi, Tor Bertin, Kayla Cosner, Belinda Vu, Angela Hong, Ria Rathod, Kareem Woods, Caitlin Bishop, and Charlotte Hartle for field and lab assistance. We are especially grateful to the Friends of Soldiers Delight and Wayne Tyndall for providing site access and additional resources. Research was supported by the Rice River Center Graduate Research Scholarship awarded to AR and start-up funding from Virginia Commonwealth University awarded to CH.
Supplementary Material
The Supplementary Material for this article can be found online at: https://www.frontiersin.org/articles/10.3389/fcosc.2022.874304/full#supplementary-material
References
Anderson, R. C., Anderson, R. A., and Corbett, E. A. (2017). High White-Tailed Deer (Odocoileus virginianus) and fire effects on flowering diversity of tallgrass forbs. J. Torrey Botan. Soc. 144, 243–253. doi: 10.3159/TORREY-D-15-00024.1
Anderson, R. C., Corbett, E. A., Anderson, R. A., Corbett, G. A., and Kelley, T. M. (2001). High white-tailed deer density has negative impact on tallgrass prairie forbs. J. Torrey Botan. Soc. 128, 381–392. doi: 10.2307/3088670
Augustine, D. J., and Frelich, L. E. (1998). Effects of white-tailed deer on populations of an under- story forb in fragmented deciduous forests. Conserv. Biol. 12, 995–1004. doi: 10.1046/j.1523-1739.1998.97248.x
Averill, K. M., Mortensen, D. A., Smithwick, E. A., Kalisz, S., McShea, W. J., Bourg, N. A., et al. (2017). A regional assessment of white-tailed deer effects on plant invasion. AoB PLANTS. 101, 1–22. doi: 10.1093/aobpla/plx047
Bakker, E. S., Ritchie, M. E., Olff, H., Milchunas, D. G., and Knops, J. M. H. (2006). Herbivore impact on grassland plant diversity depends on habitat productivity and herbivore size. Ecol. Lett. 9, 780–788. doi: 10.1111/j.1461-0248.2006.00925.x
Bates, D., Mächler, M., Bolker, B., and Walker, S. (2015). Fitting linear mixed-effects models using lme4. J. Stat. Softw. 671, 1–48. doi: 10.18637/jss.v067.i01
Coley, P. D., Bryant, J. P., and Chapin, F. S. (1985). Resource availability and plant antiherbivore defense. Science. 230, 895–899. doi: 10.1126/science.230.4728.895
Côté, S. D., Rooney, T. P., Tremblay, J., Dussault, C., and Waller, D. M. (2004). Ecological Impacts of Deer Overabundance. Ann. Rev. Ecol. Evol. System. 35, 113–147. doi: 10.1146/annurev.ecolsys.35.021103.105725
Craine, J. M., and Dybzinski, R. (2013). Mechanisms of plant competition for nutrients, water and light. Funct. Ecol 27, 833–840. doi: 10.1111/1365-2435.12081
Flinn, K. M., Mikes, J. L., and Kuhns, H. A. D. (2017). Plant diversity and community composition in eastern North American serpentine barrens. J. Torrey Botan. Soc. 1442, 25–138. doi: 10.3159/TORREY-D-16-00030
Floyd, C. J. (2006). Soldiers Delight Barrens: Preservation of a Rare Ecosystem. Soldiers Delight Conservation Inc. Friends of Soldiers Delight.
Funk, J., Larson, J., Ames, G., Butterfield, B., Cavender-Bares, J., Firn, J., et al. (2016). Revisiting the Holy Grail: using plant functional traits to understand ecological processes. Biol. Rev. Cambr. Philosoph. Soc. 92, 1156–1173. doi: 10.1111/brv.12275
Garnier, E., Cortez, J., Billes, G., Navas, M. L., Roumet, C., and Debussche, M. (2004). Plant functional markers capture ecosystem properties during secondary succession. Ecology. 85, 2630–2637. doi: 10.1890/03-0799
Geddes, N. A., and Mopper, S. (2006). Effects of environmental salinity on vertebrate florivory and wetland communities. Nat. Areas J. 261, 31–37. doi: 10.3375/0885-8608(2006)26[31:EOESOV]2.0.CO;2
Latham, R. E., Beyea, J., Benner, M., Benner, M., Dunn, C. A., and Fajvan, M. A. (2005). Managing White-Tailed Deer in Forest Habitat From an Ecosystem Perspective: Pennsylvania Case Study. Report by the Deer Management Forum. Harrisburg, PA: Audubon Pennsylvania and Pennsylvania Habitat Alliance.
Latham, R. E., and McGeehin, M. (2012). Unionville Serpentine Barrens Restoration and Management Plan. Natural Lands Trust. Media, Pennsylvania.
Lau, J. A., McCall, A. C., Davies, K. F., McKay, J. K., and Wright, J. W. (2008). Herbivores and edaphic factors constrain the realized niche of a native plant. Ecology. 89, 754–762. doi: 10.1890/07-0591.1
Maryland Natural Heritage Program (2021). Rare, Threatened and Endangered Plants of Maryland. Maryland: Maryland Department of Natural Resources.
McCune, B., and Grace, J. B. (2002). Analysis of Ecological Communities. MjM Software Design. Gleneden Beach, OR.
Mendenhall, C. D., Sekercioglu, C. H., Oviedo, B. F., and Ehrlich, P. R. (2011). Predictive model for sustaining biodiversity in the tropical countryside. PNAS. 108, 16313–16316. doi: 10.1073/pnas.1111687108
Mortenson, B., Danielson, B., Harpole, W. S., Alberti, J., Arnillas, C. A., Biederman, L., et al. (2017). Herbivores safeguard plant diversity by reducing variability in dominance. J. Ecol. 106, 101–112. doi: 10.1111/1365-2745.12821
Murphy, G. E. P., and Romanuk, T. N. (2014). A meta-analysis of declines in local species richness from human disturbances. Ecol. Evol. 41, 91–103. doi: 10.1002/ece3.909
Myers, N., Mittermeier, R., Mittermeier, C., da Fonseca, G., and Kent, J. (2000). Biodiversity hotspots for conservation priorities. Nature. 403, 853–858. doi: 10.1038/35002501
Nakahama, N., Uchida, K., Koyama, A., Iwasaki, T., Ozeki, M., and Suka, T. (2020). Construction of deer fences restores the diversity of butterflies and bumblebees as well as flowering plants in semi-natural grassland. Biodiv. Conserv. 29, 2201–2215. doi: 10.1007/s10531-020-01969-9
Nakamura, F., Swanson, F. J., and Wondzell, S. M. (2000). Disturbance regimes of stream and riparian systems – a disturbance-cascade perspective. Hydrol. Proc. 1416, 2849–2860. doi: 10.1002/1099-1085(200011/12)14:16/17<2849::AID-HYP123>3.0.CO;2-X
O'Gorman, E. J., Yearsley, J. M., Crowe, T. P., Emmerson, M. C., Jacob, U., and Petchey, O. L. (2010). Loss of functionally unique species may gradually undermine ecosystems. Proc. R. Soc. B: Biol. Sci. 278, 1886–1893. doi: 10.1098/rspb.2010.2036
Oksanen, J. F., Blanchet, F. G., Kindt, R., Legendre, P., Minchin, P. R., O'Hara, R. B., et al. (2015). Package ‘vegan'. Community ecology package. R Cran Repository.
Olff, H., and Ritchie, M. E. (1998). Importance of herbivore type and scale. Trends Ecol. Evol. 137, 261–265. doi: 10.1016/S0169-5347(98)01364-0
Pasari, J. R., Hernández, D. L., and Zavaleta, E. S. (2014). Interactive effects of nitrogen deposition and grazing on plant species composition in a serpentine grassland. Rangeland Ecol. Manage. 676, 693–700. doi: 10.2111/REM-D-13-00116.1
Peuker, M. A., Burger, H., Krausch, S., Neumuller, U., Ayasse, M., and Kuppler, J. (2020). Floral traits are associated with the quality but not quantity of heterospecific stigmatic pollen loads. BMC Ecol. 20, 54. doi: 10.1186/s12898-020-00323-5
Piqueray, J., Ferroni, L., Delescaille, L. M., Speranza, M., Mahy, G., and Poschlod, P. (2015). Response of plant functional traits during the restoration of calcareous grasslands from forest stands. Ecol. Indic. 48, 408–416. doi: 10.1016/j.ecolind.2014.08.039
Porter, W. F. (1991). White-Tailed Deer in Eastern Ecosystems: Implications for Management and Research in National Parks. Natural Resources Report. NPS/NRSUNY/NRR-91/05. United States Department of Interior, Washington, DC, United States. Available online at: http://npshistory.com/publications/wildlife/NRR-91-5.pdf
Prince, A., Thoms, J., and Prince, J. (2004). Natural Communities – Serpentine Grasslands. Maryland Dept. of Natural Resources. Learn More About It. Wildlife and Heritage Service. The Nature Conservancy.
Proulx, M., and Mazumder, A. (1998). Reversal of grazing impact on plant species richness in nutrient-poor vs. nutrient-rich ecosystems. Ecology. 79, 2581–2592. doi: 10.1890/0012-9658(1998)079[2581:ROGIOP]2.0.CO;2
Raghubanshi, A. S., and Tripathi, A. (2009). Effect of disturbance, habitat fragmentation and alien invasive plants on floral diversity in dry tropical forests of Vindhyan highland: a review. Trop. Ecol. 501, 57–69. doi: 10.1155/2011/297097
Rajakaruna, N., Harris, T. B., and Alexander, E. B. (2009). Serpentine geoecology of eastern North America: a review. Rhodora. 111, 21–108. doi: 10.3119/07-23.1
Rambo, J., and Faeth, S. (1999). Effect of vertebrate grazing on plant and insect community structure. Conserv. Biol. 135, 1047–1054. doi: 10.1046/j.1523-1739.1999.98504.x
Rawinski, T. J. (2008). Impacts of White-Tailed Deer Overabundance in Forest Ecosystems: An Overview. Northeastern Area State and Private Forestry Forest Service, U.S. Department of Agriculture Newtown Square, PA.
Ripple, W. J., and Betschta, R. L. (2012). Trophic cascades in Yellowstone: the first 15 years after wolf reintroduction. Biol. Conserv. 1451, 205–213. doi: 10.1016/j.biocon.2011.11.005
Rooney, T. P. (2001). Deer impacts on forest ecosystems: a North American perspective. Forestry. 743, 201–208. doi: 10.1093/forestry/74.3.201
Rooney, T. P., and Waller, D. M. (2003). Direct and indirect effects of white-tailed deer in forest ecosystems. Forest Ecol. Manage. 181, 165–176. doi: 10.1016/S0378-1127(03)00130-0
Ruhren, S., and Handel, S. N. (2003). Herbivory constrains survival, reproduction and mutualisms when restoring nine temperate forest herbs. J. Torrey Botan. Soc. 1301, 34–42. doi: 10.2307/3557524
Safford, H., and Mallek, C. (2011). “Disturbance and Diversity in Low-Productivity Ecosystems” in Serpentine: The Evolution and Ecology of a Model System. S. Harrison and N. Rajakaruna (University of California Press). 249–274. doi: 10.1525/california/9780520268357.003.0012
Sakata, Y., and Yamasaki, M. (2015). Deer overbrowsing on autumn-flowering plants causes bumblebee decline and impairs pollination service. Ecosphere. 6, 1–13. doi: 10.1890/ES15-00401.1
Sandel, B., Corbin, J., and Krupa, M. (2011). Using plant functional traits to guide restoration: A case study in California coastal grassland. Ecosphere. 2, 1–16. doi: 10.1890/ES10-00175.1
Smith, R. H. (2010). Butterflies of Soldiers Delight NEA. Soldiers Delight Conservation Inc. Friends of Soldiers Delight.
Stephan, J. G., Pourazari, F., Tattersdill, K., Kobayashi, T., Nishizawa, K., and De Long, J. R. (2017). Long-term deer exclosure alters soil properties, plant traits, understory plant community and insect herbivory, but not the functional relationships among them. Oecologia. 1843, 685–699. doi: 10.1007/s00442-017-3895-3
Strauss, S., and Boyd, R. (2011). “Herbivory and Other Cross-Kingdom Interactions on Harsh Soils” in Serpentine: The Evolution and Ecology of a Model System. eds S. Harrison and N. Rajakaruna (University of California Press) 181–200. doi: 10.1525/9780520948457-011
Strong, D. R., and Frank, K. T. (2010). Human Involvement in Food Webs. Ann. Rev. Environ. Resour. 35, 1–23. doi: 10.1146/annurev-environ-031809-133103
Tamura, A. (2010). Effect of time lag of establishment of deer-proof fences on the recovery of perennial herbs in a cool temperate deciduous forest diminished by sika deer browsing in the Tanzawa Mountains, central Japan. Japan J. Conserv. Ecol. 15, 255–264. doi: 10.1007/s10310-015-0509-y
Tyndall, R. W. (1992). Historical considerations of conifer expansion in Maryland Serpentine “Barrens”. Castanea. 572, 123–131.
Tyndall, R. W. (1994). Conifer clearing and prescribed burning effects to herbaceous layer vegetation on a maryland serpentine “Barren”. Castanea. 593, 255–273.
Tyndall, R. W. (2005). Twelve years of vegetation change in oak savanna habitat on a Maryland serpentine barren after Virginia pine removal. Castanea. 70, 287–297. doi: 10.2179/0008-7475(2005)070[0287:TYOHVC]2.0.CO;2
Tyndall, R. W. (2012). Soil differences between extant serpentine oad savanna and grassland in Soldiers Delight Natural Area. Castanea. 77, 224–224. doi: 10.2179/11-020
Tyndall, R. W., and Hull, J. C. (1999). “Vegetation, flora, and plant physiological ecology of serpentine barrens of eastern North America” in Savannas, barrens, and rock outcrop plant communities of North America. eds R. C. Anderson, J. S. Fralish, and J. M., Baskin (Cambridge University Press) 67–82. doi: 10.1017/CBO9780511574627.005
Vasquez, D. P., Bluthgen, N., Cagnolo., L, and Chacoff, N. P. (2009). Uniting pattern and process in plant-animal mutualistic networks: A review. Ann. Bot. 103, 1445–1457. doi: 10.1093/aob/mcp057
Venables, W. N., and Ripley, B. D. (2002). Modern Applied Statistics with S, Fourth edition. New York: Springer. doi: 10.1007/978-0-387-21706-2
Violle, C., Navas, M., Vile, D., Kazakou, E., Fortunel, C., Hummel, I., et al. (2007). Let the concept of trait be functional!. Oikos 116, 882–892. doi: 10.1111/j.0030-1299.2007.15559.x
Walters, B. F., Woodall, C. W., and Russell, M. B. (2016). White-Tailed Deer Density Estimates Across the Eastern United States, 2008. Bogart, GA: Data Repository for the University of Minnesota. doi: 10.13020/D6G014
Wang, H., and Mopper, S. (2008). Separate and Interacting Effects of Deer Florivory and Salinity Stress on Iris Herbivores. Oikos. 1174, 564–570. doi: 10.1111/j.0030-1299.2008.16335.x
Keywords: overbrowsing: deer, deer overabundance, serpentine grassland, rare and endemic plants, restoration treatments, plant community regeneration, plant response traits
Citation: Richins AE and Hulshof CM (2022) Deer Exclusion Regenerates Native Plant Functional Responses, but Not Species Richness in an Eastern Serpentine Savannah. Front. Conserv. Sci. 3:874304. doi: 10.3389/fcosc.2022.874304
Received: 11 February 2022; Accepted: 05 April 2022;
Published: 10 May 2022.
Edited by:
Colleen Lynda Seymour, South African National Botanical Institute, South AfricaReviewed by:
Bernd Blossey, Cornell University, United StatesAndrea T. Kramer, Chicago Botanic Garden, United States
Copyright © 2022 Richins and Hulshof. This is an open-access article distributed under the terms of the Creative Commons Attribution License (CC BY). The use, distribution or reproduction in other forums is permitted, provided the original author(s) and the copyright owner(s) are credited and that the original publication in this journal is cited, in accordance with accepted academic practice. No use, distribution or reproduction is permitted which does not comply with these terms.
*Correspondence: Allyson E. Richins, YWVyaWNoaW5zOTNAZ21haWwuY29t