- 1U.S. Geological Survey, Western Geographic Science Center, Tucson, AZ, United States
- 2United States Geological Survey, Western Geographic Science Center, Moffett Field, CA, United States
- 3Wildlands Network, Hermosillo, Sonora, Mexico
- 4Wildlands Network, Tucson, AZ, United States
The construction of a wall at the United States-Mexico border is known to impede and deter movement of terrestrial wildlife between the two countries. One such species is the jaguar, in its northernmost range in the borderlands of Arizona and Sonora. We developed an anisotropic cost distance model for jaguar in a binational crossing area of the Madrean Sky Islands at the United States-Mexico border in Southern Arizona as a case study by using previously collected GPS tracking data for jaguars, bioenergetic calculations for pumas, and a digital elevation model. This model describes projected energy expenditure for jaguar to reach key water sources north of the international border. These desert springs and the broader study region provide vital habitat for jaguar conservation and reintroduction efforts in the United States. An emerging impediment to jaguar conservation and reintroduction is border infrastructure including border wall. By comparing walled and un-walled border sections, and three remediation scenarios, we demonstrate that existing border infrastructure significantly increases energy expenditure by jaguars and that some partial remediation scenarios are more beneficial than others. Our results demonstrate opportunities for remediation. Improved understanding of how border infrastructure impacts physiological requirements and resulting impacts to jaguar and other terrestrial wildlife in the United States-Mexico borderlands may inform conservation management.
Introduction
Transboundary landscapes often coincide with biodiversity hotspots and hence provide important habitat for threatened species, but face major threats from habitat fragmentation by walls and other types of security infrastructure (Erg et al., 2015). This makes such regions increasingly important to conservation, especially as physical barriers are increasing globally in number and extent (Titley et al., 2021). By limiting movement, these fences and walls threaten to reduce access to water, food, and mates for wide-ranging and migratory species and those with naturally or anthropogenically fragmented habitats (Liu et al., 2020). In the case of the United States-Mexico border, wall construction has directly reduced the area, quality, and connectivity of available habitat by physically limiting wildlife movement, and dispersal of species that cannot climb or move through existing walls or that avoid light and noise from human activity and vegetation openings (Flesch et al., 2010; Peters et al., 2018). A continuous border-wide wall could fragment an estimated34% of U.S. nonflying native terrestrial animal species (Peters et al., 2018). One region of the borderlands at particular risk are the Madrean Sky Islands of southeastern Arizona in the U.S. and northeastern Sonora in Mexico, with high biodiversity and home of low-density populations near the margins of species’ ranges (Lasky et al., 2011). One such animal is the jaguar (Panthera onca), a species of concern in both the U.S. and Mexico, which is the target of binational recovery efforts that may fail in the absence of transboundary connectivity (Rodríguez-Soto et al., 2013). The Madrean Sky Islands serve as critical habitat and dispersal routes but face threats from fragmentation by border infrastructure (Patrick-Birdwell et al., 2013).
The jaguar is listed as endangered by both Mexico’s Ministry of Environment and Natural Resources and the United States Fish and Wildlife Service (USFWS, 2014; González-Gallina et al., 2018). Near the species’ northern limits, Sonora supports the northernmost breeding population of jaguars from which individual males sometimes disperse into its historical range in the southwestern U.S. states of Arizona and New Mexico. International cooperation and transboundary connectivity are vital for the conservation and recovery of this northern population (Svancara et al., 2015; Cassaigne et al., 2016). For conservation efforts to be successful, landscapes that support habitat in the borderlands must foster sufficient landscape connectivity to allow natural movement and dispersal. Structures such as road crossings and remediation of habitat altered by humans may benefit movement and dispersal (Manteca-Rodríguez et al., 2021). In the case of the United States-Mexico border, potential impediments to movement include built wall and fence structures and a 60-100-foot-wide parallel road with flood lights, fixed-location surveillance towers with access roads, and frequent vehicle traffic that may obstruct or divert movement and increase energetic costs. Minimizing impediments is especially critical in the case of reproduction. Female jaguars disperse much shorter distances than males, and hence use a smaller amount of energy for movement (Sadowski-Smith, 2013; Povilitis, 2015). However, females require significantly more energy than males when gestating, when lactating, and when feeding cubs, all events required for effective jaguar recovery within the U.S. (Oftedal and Gittleman, 1989; Conde et al., 2010). Currently, only male jaguars have been documented in the U.S. in recent decades, and conservation planners are working to design effective corridors linking populations that minimize energy expenditure. Similarly, proposed jaguar reintroduction in the U.S. (Sanderson et al., 2021) would show greater success with such considerations in remediation, especially as recovery will likely require both the reintroduction of female jaguars to Arizona (Babb et al., 2022) and migration of jaguars from Sonora (Sanderson et al., 2022).
Energy expenditure
Animals minimize energy expenditure by altering behavior to conserve energy stores (Iodice et al., 2017). One such behavior change is patrolling of barriers to search for gaps and the decision to leave the barrier (McInturff et al., 2020). When a wide-ranging species must maintain connectivity, any impediment to movement can increase individual energy expenditure over time by a cumulative effect of moving past human-built barriers (Jakes et al., 2018). In order to better understand energy expenditure outside the lab setting, Shepard et al. (2013) recommended modeling energy landscapes to “provide a framework with which variation in animal movements can be understood or predicted over varying spatial and temporal scales.” Such a model can consider both terrain and direction of locomotion, requiring an anisotropic measure of energy expenditure (Vosper, 2003). Anisotropic modeling assigns different cost values depending on the direction of travel, which is especially useful in predicting energy expenditure (Etherington, 2016). As animals may deviate movement to avoid areas of high energy cost or to exploit the potential of saving energy (Shepard et al., 2013), cost distance modeling can serve as a both predictor for movement and a measure of cumulative bodily cost over time and space (Chambers et al., 2022a). With such modeling, conservation planning could better account for survival and reproduction, which are both dependent on energy efficiency, balancing intake and expenditure (Kinnison et al., 2003). Measurements are compared by regression analysis to environmental or other physiological factors such as step length and mass (Gold, 1973; Wilson et al., 2013). In the case of wildlife, energy expenditure has most often been inferred from other models (Rohweder et al., 2012; Porter et al., 2015) rather than cost distance. Such bioenergetic models can identify and map hot-spots of physiological stress, and there is growing interest in mapping such ‘stress-scapes’ (Cooke and O’Connor, 2010; Madliger et al., 2016; Ames et al., 2020).
Jaguar movement and modeling
Movement and dispersal of large carnivores is dependent on energy reserves and an ample supply of prey and water (Garland, 1983; McNab, 1989; Stander et al., 1997; Boratyński, 2021). This is especially prevalent in heterogeneous landscapes, particularly those altered by humans (Rosas-Rosas et al., 2010). Energy is a limiting factor for individual range size and dispersal (Foster et al., 2013). To successfully access water, jaguar and their prey must maintain homeostasis and minimize increases in body temperature (O’Farrill et al., 2014). Water is most necessary for the indirect hydration of jaguars through prey (Pietsch et al., 2011) and in addition, temperature regulation is dependent on minimizing energy expenditure and ample hydration (Fuller et al., 2016 This requires limiting energy expenditure (McNab, 2000) and maintaining hydration (Strauss et al., 2017). To assure efficiency and survival, big-cats minimize energy expenditure by limiting distance traveled and travel even in rugged terrain to avoid walking perpendicular to slope (Harmsen et al., 2011; Wilmers et al., 2017; Dunford et al., 2020). It appears that apex predators are undertaking long journeys along the border wall. Evidence of this can be anecdotal, but jaguar journeys ranging from 3 to 1102 km in Mexico and Central America (Rabinowitz and Zeller, 2010) have been documented. More recently, a Mexican gray wolf in New Mexico, followed the U.S.-Mexico border wall for 23 miles before turning back north (CBD, 2021). Similarly, jaguars have been shown to look for and use openings in other linear barriers such as fences and roads (González-Gallina et al., 2018). Springs could serve as transient refugia and “stepping-stones”, for a range shift to habitat with greater water availability than that of Sonora or southern Arizona (Cartwright et al., 2020). Although species like jaguar could theoretically move their home ranges in response to a border barrier (McCallum et al., 2014), limiting factors such as energy expenditure and resource availability could limit the effectiveness and overall distance of such movement.
Flesch et al. (2010) used topography and distance based least-cost paths to assess impacts of the United States-Mexico border fence on bighorn sheep and show opportunities for mitigation. Similarly, Thompson and Velilla (2017) used a combination of least-cost modeling and circuit theory with landscape characteristics and expert opinion to map and rate multi-regional corridors for jaguar in South America. Although animal energetics are inferred in creating such cost surfaces, analyses are typically isotropic (i.e., not considering the direction of slope; Green et al., 2020) and do not consider bodily cost, which is less realistic for individual-based and local models (Rabinowitz and Zeller, 2010).
The objective of this study is to measure the effects of border barriers on the required energy expenditure of individual jaguars moving north to the U.S. from Mexico. We hypothesized increases in total energy expenditure for northbound movements and those to reach key water sources required for successful dispersal. In particular, we address the following questions:
i. Is there a significant increase in energy expenditure to the water sources which are required for maintaining viable breeding populations by hydration and prey availability?
ii. What border barrier remediation scenario best reduces required energy expenditure to reach water sources?
Materials and methods
Critical habitat crossings
In 2014, the U.S. Fish and Wildlife Service (USFWS) identified areas of critical jaguar habitat, based on current knowledge of the species’ habitat needs, including prey species, water, canopy cover, terrain characteristics, and human population densities (USFWS, 2014). Much of these areas were within the Madrean Sky Island Ecoregion (Stoner et al., 2015; Villarreal et al., 2019) where the U.S. Border Patrol maintains infrastructure at the United States-Mexico border that include various types of permanent barriers, i.e., walls and fences that can limit wildlife movement and transboundary landscape connectivity (McCallum et al., 2014). Stoner et al. (2015) identified how roads impact habitat connectivity in this same region, referred to as the Northwestern Recovery Unit in the USFWS Jaguar Recovery Plan (USFWS, 2018). Based on this knowledge, we modeled unfragmented habitat in the Madrean Sky Islands, to identify corridors connecting jaguar habitat patches from Mexico to the United States using the geospatial tool Corridor Designer (Majka et al., 2007). This model assumed existing pedestrian fencing at the United States-Mexico border had impacted crossings in similar fashion as roads and identified the most permeable corridors for jaguars in southern Arizona and New Mexico in the U.S. and northern Sonora and Chihuahua in Mexico.
Study area
Using area identified by the critical habitat crossing model described above, we selected the the Tumacacori Highlands near Nogales, Arizona and Sonora as a case study. This area is a proposed wilderness area (Hoover et al., 2014) that includes land managed by the U.S. Forest Service (Coronado National Forest), Arizona State Land Department, and private landowners and is designated as part of the Atacosa critical habitat area by the USFWS (2014). Because there have been multiple jaguars identified in this region in the past (Babb et al., 2022), information to guide conservation is important. This study takes into account wall construction as of 2021 (Traphagen, 2021).
Speed estimates
To estimate average speed of jaguar locomotion, we first extracted elevation values from 30-meter digital elevation models (DEM) (Farr et al., 2007) and assigned values to 134,690 time-coded GPS data points for 117 individual jaguars (Morato et al., 2018) in Mexico, Guatemala, Costa Rica, Brazil, Paraguay, and Argentina. We then derived degree slope and direction (ascending or descending) from consecutive points and used the time between these points to derive speed. We removed all sedentary data (<2 km/hr or 0.56 m/s) and those speeds impossible for jaguar (>22.2 m/s) (Harmsen et al., 2009). All remaining data with greater than a day of travel were also removed from analysis. To preserve accuracy of slope estimates, we also removed all points with greater than three raster cell lengths between them.
Using transformed linear regression (R Core Team, 2022), we modeled the relationship of jaguar speed to slope for both inclines (Si) and declines (Sd) (p-value< 0.001).
Predicted energy expenditure
To predict energy expenditure (EE) for a 60 kg jaguar, a value used in previous analysis to represent typical body mass over the entire distribution of the species, (Hayward et al., 2016) by slope and distance, we used these calculations in combination with the formulas developed for cougar (Puma concolor) by Dunford et al. (2020). As there were no available similar analyses for jaguar, we assumed this method was suitable due to felid energy expenditure correlating across species and being dependent on body mass, food, and climate, irrespective of body shape (McNab, 2000). Figure 1 shows the results of our regression analyses and the resulting function of EE by slope for inclines (EEi) and declines (EEd).
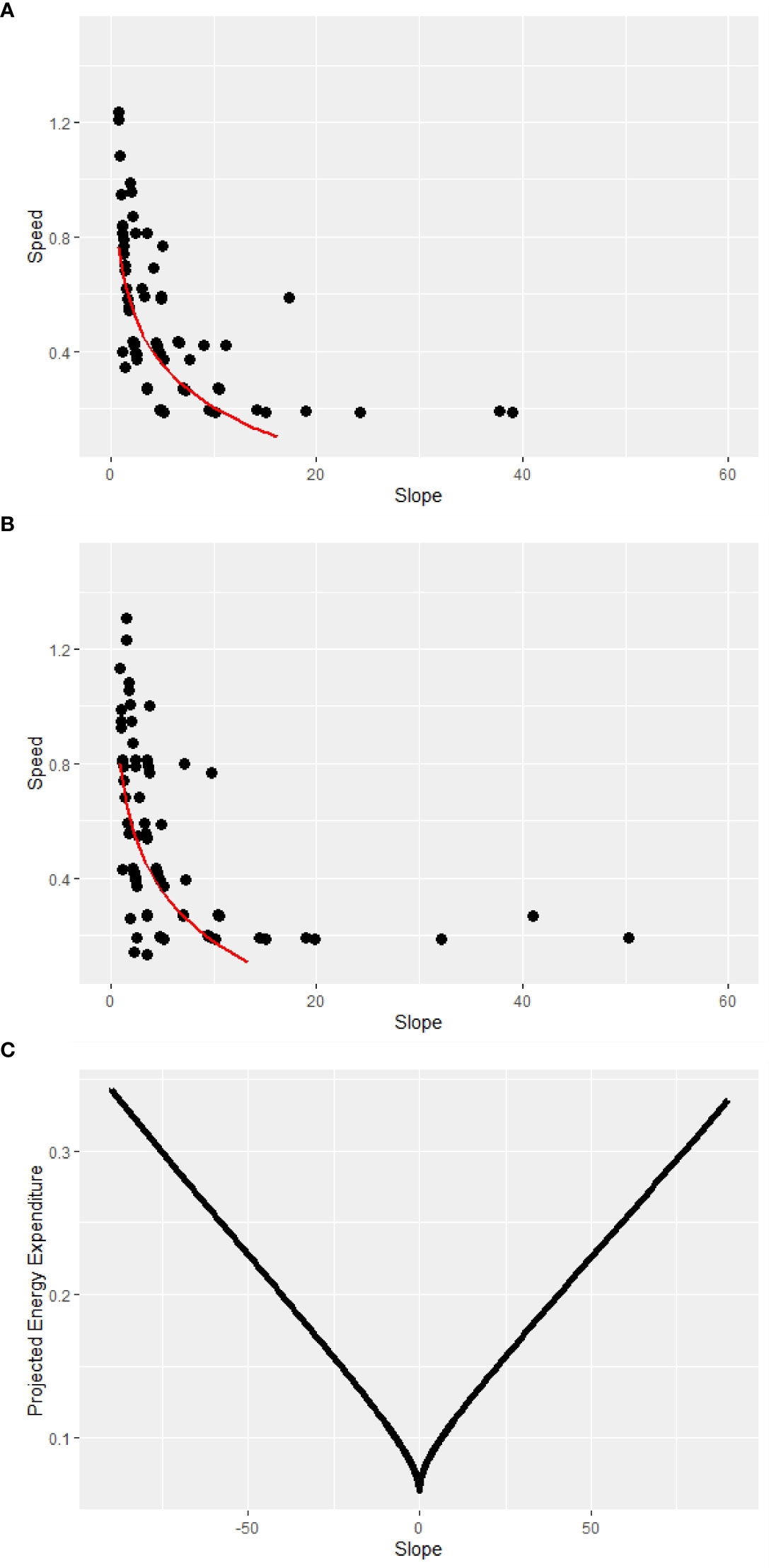
Figure 1 Regression scatter plots of incline (A) and decline degree slope (B) and jaguar speed (meters per second) and resulting energy expenditure, in kilocalories per meter walked, by slope (C).
With the regression results, we created an anisotropic EE cost distance model using the ArcGIS Path Distance Tool (Esri, 2020) and a DEM for the binational crossing in southern Arizona (USGS, 2017). We ran this model from the Mexico border moving north for an unwalled and a walled EE as of the year 2021 (Chambers et al., 2022b). We extracted the values for the unwalled and walled EE to the locations of documented springs (Ledbetter et al., 2018) within the binational crossing in order to test for change specific to water sources. To test for changes in EE, we performed t-tests for total walled and unwalled costs and for walled and unwalled costs at water sources.
Remediation scenario development
Following this initial analysis, we created remediation scenarios where we added gaps (Saxena and Habib, 2022) to walled portions of the border and calculated EE for each (Chambers et al., 2022b). These scenarios would allow jaguars to cross either in rugged terrain west of the existing wall (Scenario A), a dry wash, also in relatively rugged terrain, running north from the existing wall (Scenario B), and a less rugged terrain (Scenario C) in the east. Each gap was 30 meters wide, the minimum possible with our raster analysis. We then extracted these values to the spring locations and ran additional t-tests for each against the original walled scenario of 2021.
Results
Wall impacts
The mean EE to reach water from the border increased significantly by 90.94 kcal between the unwalled model and that with walls (Table 1), which is approximately 4% of the daily energy required for a 60 kg jaguar (Rueda et al., 2013). EE increased over the entire area but was greatest in areas directly north of the wall and for those springs adjacent to the wall (Figure 2). In the presence of the border wall EE had a maximum value of 3,724.15 whereas without the wall it was 3,680.7. The surplus distance required of a jaguar to walk with this caloric increase ranges from 3 to 9 km.

Table 1 Results of t-test comparing energy expenditures (kcal) for jaguar, unwalled and walled 2021 and t-tests comparing remediation scenarios A, B, and C to walled 2021.
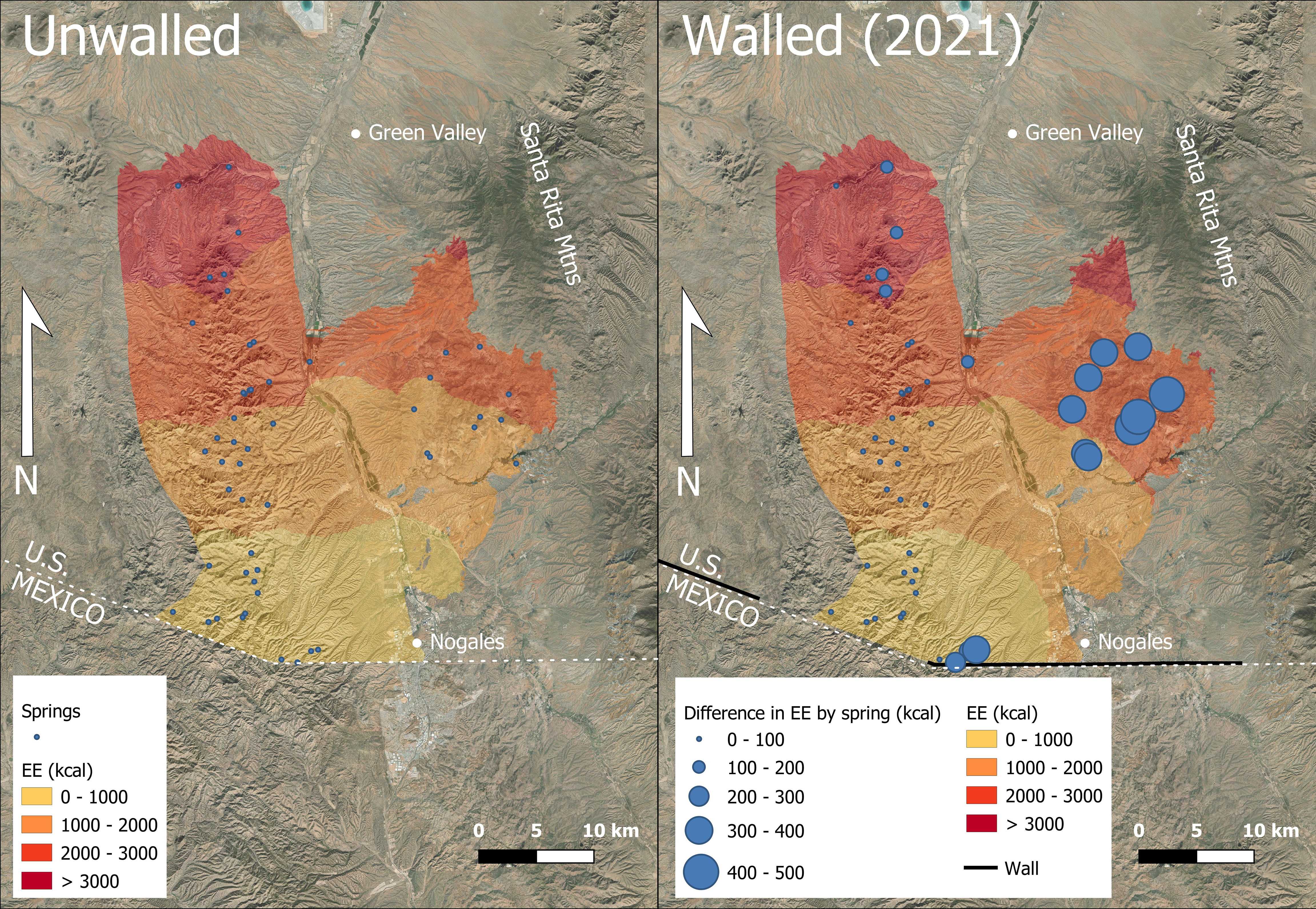
Figure 2 Cumulative jaguar energy expenditure (kcal) from the border north pre-wall (left) and with walls as of 2021 (right) in the United States portion of the Critical habitat crossings corridor for our case study around Nogales, Arizona. Spring locations post-wall are symbolized by difference in EE from pre-wall scenario (Chambers et al., 2022b).
Remediation scenarios
Scenario A, in the more rugged terrain, showed no significant change from the 2021 baseline for EE to reach springs in any part of the binational crossing, whereas scenario B in the wash and C in the less rugged terrain both reduced EE but differed little as the mean change for scenario B was 1,368.86 kcal verses 1,366.28 kcal for C. Neither returned to the 1,342.92 kcal of the unwalled scenario. Scenario A, like 2021, had a maximum of 3,724.15 kcal while both B and C had a maximum of 3,680.7 kcal. Figure 3 shows how EE decreased on the eastern side of the binational crossing for those gaps in scenarios B and C but remained the same in the case of scenario A.
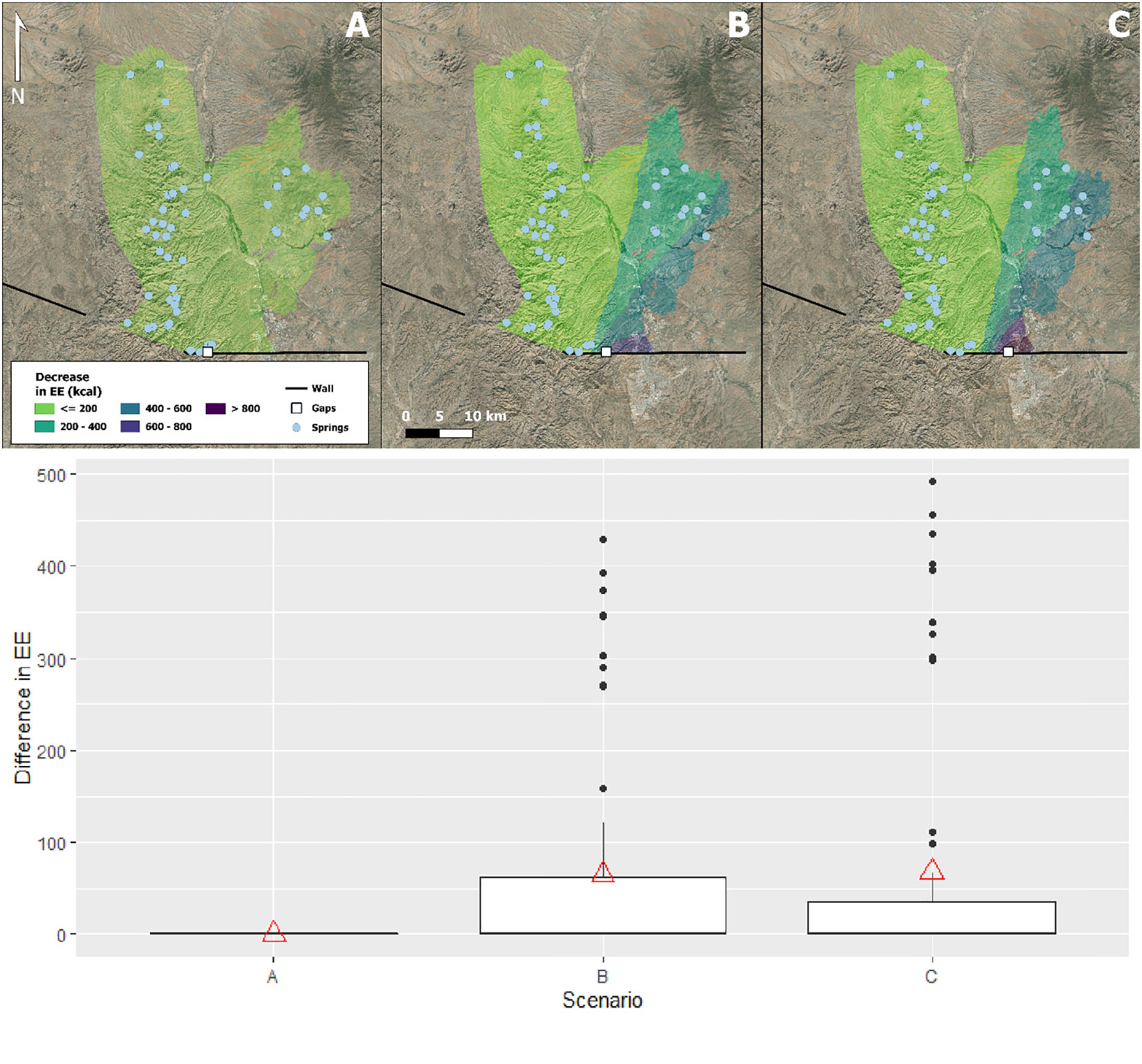
Figure 3 Maps showing decrease in energy expenditure (kcal) from 2021 walled scenario for remediation scenarios (A–C) and box and whisker plots of difference in energy expenditure, from 2021 walled energy expenditure (y-axis), for each remediation scenario (x-axis), (A–C). Greatest change is seen in scenario (B) while scenario (A) shows no difference between 2021 and remediation. Triangles mark mean energy expenditure for each difference in scenario.
Discussion
Border wall construction has increased the energy required for jaguars to reach key water sources in our binational study area in the U.S.-Mexico borderlands. This increase was greatest in springs due north of the border wall and springs adjacent to the wall. This suggests the wall has an immediate and compounding effect when jaguars cannot enter the U.S. in the eastern part of the study region. Energy expenditure required to reach those springs increases because a jaguar must circumvent the wall rather than walking directly across the border to the springs. Remediation scenario A focused in rugged terrain at the western end of the wall did not decrease EE to reach any springs, but both scenario B and C focused at a central dry wash and less rugged terrain in the east significantly decreased EE. Management that considers energy expenditure and associated water requirements could support decision making to augment landscape connectivity for jaguars.
Wall and fence infrastructure are expected to divert dispersing jaguars and are already known to divert movement of other species in the region (e.g., puma and coati; McCallum et al., 2014) and affect energy expenditures (EE). The results of our analysis support this by demonstrating that border barriers have immediate impacts on local habitat connectivity and drive potentially compounding effects of increasing EE of jaguars to reach areas farther from the border. Jaguar populations depend on caloric reserves and ample supply of water and prey (Anile et al., 2020), needs which are greater in semi-arid regions and areas altered by humans (Rosas-Rosas et al., 2010). Hence, minimization of energy expenditures may improve the probability of reproductive success and survival and support maintenance or increase of the jaguar population (Foster et al., 2013). With the increase of EE to reach springs, we recommend remediation scenarios that best limit EE by providing gaps like those in large dry washes for rugged habitat and near the center of our study area the wall and less rugged spaces like that of the eastern portion of the crossing. Analyses focused on other regions of the borderlands and potential wall locations could support gap placement decisions dependent on the influence of terrain ruggedness on EE.
Data availability statement
Raster layers of cumulative energy expenditure models and metadata are available as a U.S. Geological Survey (USGS) 451 Data Release at the USGS data repository ScienceBase (Chambers et al. 2022; doi: 10.5066/P9DSSV2Q).
Author contributions
SC: Conceptualization, Methodology, Data curation, Writing, Visualization. MV: Supervision, Conceptualization, Writing. LN: Data Curation, Writing. JB: Advising, Writing. MT: Data collection. All authors contributed to the article and approved the submitted version.
Acknowledgments
We would like thank Aaron Flesch for reviewing and commenting on a draft of this manuscript. We would also like to thank Alex Vanko of Wildlands Network for helping with the provision of maps of the existing border wall in Arizona. Funding for this research was provided by the U.S. Geological Survey Land Change Science Program. Any use of trade, firm, or product names in this article is for descriptive purposes only and does not imply endorsement by the U.S. government.
Conflict of interest
The authors declare that the research was conducted in the absence of any commercial or financial relationships that could be construed as a potential conflict of interest.
Publisher’s note
All claims expressed in this article are solely those of the authors and do not necessarily represent those of their affiliated organizations, or those of the publisher, the editors and the reviewers. Any product that may be evaluated in this article, or claim that may be made by its manufacturer, is not guaranteed or endorsed by the publisher.
References
Ames E. M., Gade M. R., Nieman C. L., Wright J. R., Tonra C. M., Marroquin C. M., et al. (2020). Striving for population-level conservation: integrating physiology across the biological hierarchy. Conserv. Physiol. 8 (1), coaa019. doi: 10.1093/conphys/coaa019
Anile S., Greenspan E., Nielsen C. K. (2020). Determinants of jaguar occupancy at the northern range edge. Mamm. Res. 65 (4), 667–677. doi: 10.1007/s13364-020-00511-0
Babb R. D., Brown D. E., Culver M., Childs J., Thompson R., Kohls R. L., et al. (2022). Updates of historic and contemporary records of jaguars (Panthera onca) from Arizona. J. Arizona-Nevada Acad. Sci. 49 (2), 65–91. doi: 10.2181/036.049.0205
Boratyński Z. (2021). Energetic constraints on mammalian distribution areas. J. Anim. Ecol. 90 (8), 1854–1863.4. doi: 10.1111/1365-2656.13501
Cartwright J. M., Dwire K. A., Freed Z., Hammer S. J., McLaughlin B., Misztal L. W., et al. (2020). Oases of the future? springs as potential hydrologic refugia in drying climates. Front. Ecol. Environ. 18 (5), 245–253. doi: 10.1002/fee.2191
Cassaigne I., Medellín R. A., Thompson R. W., Culver M., Ochoa A., Vargas K., et al. (2016). Diet of pumas (Puma concolor) in Sonora, Mexico, as determined by GPS kill sites and molecular identified scat, with comments on jaguar (Panthera onca) diet. Southwest. Nat. 61 (2), 125–132. doi: 10.1894/0038-4909-61.2.125
Center for Biological Diversity (CBD) (2021) Wandering Mexican gray wolf in new Mexico blocked by border wall. Available at: https://biologicaldiversity.org/w/news/press-releases/wandering-mexican-gray-wolf-in-new-mexico-blocked-by-border-wall-2021-12-13/ (Accessed April 29, 2022).
Chambers S., Villarreal M. L., Duane O., Seth M. Munson S. M., Stuber E., Gayle T., et al. (2022a). Conflict of energies: Spatially modeling mule deer caloric expenditure in response to oil and gas development. Landscape Ecol. doi: 10.1007/s10980-022-01521-w
Chambers S. N., Villarreal M. L., Norman L., Bravo J. C. (2022b). Maps of cumulative energy expenditure models for jaguar in southern Arizona: U.S (Geological Survey data release). doi: 10.5066/P9DSSV2Q
Conde D. A., Colchero F., Zarza H., Christensen N. L. Jr., Sexton J. O., Manterola C., et al. (2010). Sex matters: Modeling male and female habitat differences for jaguar conservation. Biol. Conserv. 143 (9), 1980–1988. doi: 10.1016/j.biocon.2010.04.049
Cooke S. J., O’Connor C. M. (2010). Making conservation physiology relevant to policy makers and conservation practitioners. Conserv. Lett. 3 (3), 159–166. doi: 10.1111/j.1755-263X.2010.00109.x
Dunford C. E., Marks N. J., Wilmers C. C., Bryce C. M., Nickel B., Wolfe L. L., et al. (2020). Surviving in steep terrain: a lab-to-field assessment of locomotor costs for wild mountain lions (Puma concolor). Mov. Ecol. 8 (1), 1–12. doi: 10.1186/s40462-020-00215-9
Erg B., Groves C., McKinney M., Michel T. R., Phillips A., Schoon M. L., et al. (2015). Transboundary conservation: A systematic and integrated approach, Vol. 2015(23) (Gland, Switzerland: The World Conservation Union (IUCN). xii + 107.
Esri (2020) How the path distance tools work. Available at: https://pro.arcgis.com/en/pro-app/latest/tool-reference/spatial-analyst/how-the-path-distance-tools-work.htm.
Etherington T. R. (2016). Least-cost modelling and landscape ecology: concepts, applications, and opportunities. Curr. Landscape Ecol. Rep. 1 (1), 40–53. doi: 10.1007/s40823-016-0006-9
Farr T. G., Rosen P. A., Caro E., Crippen R., Duren R., Hensley S., et al. (2007). The shuttle radar topography mission. Rev. Geophys. 45 (2), 1–33. doi: 10.1029/2005RG000183
Flesch A. D., Epps C. W., Cain J. W. III, Clark M., Krausman P. R., Morgart J. R. (2010). Potential effects of the united states-Mexico border fence on wildlife. Conserv. Biol. 24 (1), 171–181. doi: 10.1111/j.1523-1739.2009.01277.x
Foster V. C., Sarmento P., Sollmann R., Tôrres N., Jácomo A. T., Negrões N., et al. (2013). Jaguar and puma activity patterns and predator-prey interactions in four Brazilian biomes. Biotropica 45 (3), 373–379. doi: 10.1111/btp.12021
Fuller A., Mitchell D., Maloney S. K., Hetem R. S. (2016). Towards a mechanistic understanding of the responses of large terrestrial mammals to heat and aridity associated with climate change. Climate Change Response. 3 (1), 1–19. doi: 10.1186/s40665-016-0024-1
Garland T. Jr. (1983). Scaling the ecological cost of transport to body mass in terrestrial mammals. Am. Nat. 121 (4), 571–587. doi: 10.1086/284084
Gold A. (1973). Energy expenditure in animal locomotion. Science 181 (4096), 275–276. doi: 10.1126/science.181.4096.275
González-Gallina A., Hidalgo-Mihart M. G., Castelazo-Calva V. (2018). Conservation implications for jaguars and other neotropical mammals using highway underpasses. PloS One 13 (11), e0206614. doi: 10.1371/journal.pone.0206614
González-Solís J., Felicísimo A., Fox J. W., Afanasyev V., Kolbeinsson Y., Muñoz J. (2009). Influence of sea surface winds on shearwater migration detours. Mar. Ecol. Prog. Ser. 391, 221–230. doi: 10.3354/meps08128
Green S. J., Boruff B. J., Bonnell T. R., Grueter C. C. (2020). Chimpanzees use least-cost routes to out-of-sight goals. Curr. Biol. 30 (22), 4528–4533. doi: 10.1016/j.cub.2020.08.076
Harmsen B. J., Foster R. J., Silver S. C., Ostro L. E., Doncaster C. P. (2009). Spatial and temporal interactions of sympatric jaguars (Panthera onca) and pumas (Puma concolor) in a neotropical forest. J. Mamm. 90 (3), 612–620. doi: 10.1644/08-MAMM-A-140R.1
Harmsen B. J., Foster R. J., Silver S. C., Ostro L. E., Doncaster C. P. (2011). Jaguar and puma activity patterns in relation to their main prey. Mamm. Biol. 76 (3), 320–324. doi: 10.1016/j.mambio.2010.08.007
Hayward M. W., Kamler J. F., Montgomery R. A., Newlove A., Rostro-García S., Sales L. P., et al. (2016). Prey preferences of the jaguar panthera onca reflect the post-pleistocene demise of large prey. Front. Ecol. Evol. 3, 148. doi: 10.3389/fevo.2015.00148
Hoover K., Alexander K., Johnson S. (2014). ‘Wilderness: Legislation and Issues in the 113th Congress’, Congressional Research Service Report R41610.
Iodice P., Ferrante C., Brunetti L., Cabib S., Protasi F., Walton M. E., et al. (2017). Fatigue modulates dopamine availability and promotes flexible choice reversals during decision making. Sci. Rep. 7 (1), pp.1–pp11. doi: 10.1038/s41598-017-00561-6
Jakes A. F., Jones P. F., Paige L. C., Seidler R. G., Huijser M. P. (2018). A fence runs through it: A call for greater attention to the influence of fences on wildlife and ecosystems. Biol. Conserv. 227, 310–318. doi: 10.1016/j.biocon.2018.09.026
Kinnison M. T., Unwin M. J., Quinn T. P. (2003). Migratory costs and contemporary evolution of reproductive allocation in male chinook salmon. J. Evol. Biol. 16 (6), 1257–1269. doi: 10.1046/j.1420-9101.2003.00631.x
Lasky J. R., Jetz W., Keitt T. H. (2011). Conservation biogeography of the US–Mexico border: a transcontinental risk assessment of barriers to animal dispersal. Diversity Distrib. 17 (4), 673–687. doi: 10.1111/j.1472-4642.2011.00765.x
Ledbetter J. D., Stevens L. E., Springer A. E., Brandt B. (2018) Springs online: Springs and springs-dependent species database. version 1.0 (Flagstaff: Springs Stewardship Institute, Museum of Northern Arizona) (Accessed January 12, 2022).
Liu J., Yong D. L., Choi C. Y., Gibson L. (2020). Transboundary frontiers: an emerging priority for biodiversity conservation. Trends Ecol. Evol. 35 (8), 679–690. doi: 10.1016/j.tree.2020.03.004
Madliger C. L., Cooke S. J., Crespi E. J., Funk J. L., Hultine K. R., Hunt K. E., et al. (2016). Success stories and emerging themes in conservation physiology. Conserv. Physiol. 4 (1), cov057. doi: 10.1093/conphys/cov057
Majka D., Jenness J., Beier P. (2007). CorridorDesigner: ArcGIS tools for designing and evaluating corridors. Available at http://corridordesign.org.
Manteca-Rodríguez M., Félix-Burruel R. E., Aguilar-Morales C., Bravo J. C., Traphagen M., Larios E. (2021). Wildlife use of drainage structures under 2 sections of federal highway 2 in the sky island region of northeastern Sonora, Mexico. Air Soil Water Res. 14, 1178622120988721. doi: 10.1177/1178622120988721
McCallum J. W., Rowcliffe J. M., Cuthill I. C. (2014). Conservation on international boundaries: The impact of security barriers on selected terrestrial mammals in four protected areas in Arizona, USA. PloS One 9 (4), e93679. doi: 10.1371/journal.pone.0093679
McInturff A., Xu W., Wilkinson C. E., Dejid N., Brashares J. S. (2020). Fence ecology: Frameworks for understanding the ecological effects of fences. BioScience 70 (11), 971–985. doi: 10.1093/biosci/biaa103
McNab B. K. (1989). Basal rate of metabolism, body size, and food habits in the order CarnivoraCarnivore behavior, ecology, and evolution (Boston, MA: Springer), 335–354.
McNab B. K. (2000). “The standard energetics of mammalian carnivores: Felidae and hyaenidae”. Can. J. Zool. 78 (12), 2227–2239. doi: 10.1139/z00-167
Morato R. G., Thompson J. J., Paviolo A., de la Torre J. A., Lima F., McBride R. T. Jr., et al. (2018). Jaguar movement database: a GPS-based movement dataset of an apex predator in the neotropics. Ecology 99, 1691. doi: 10.1002/ecy.2379
O’Farrill G., Gauthier Schampaert K., Rayfield B., Bodin Ö., Calme S., Sengupta R., et al. (2014). The potential connectivity of waterhole networks and the effectiveness of a protected area under various drought scenarios. PloS One 9 (5), e95049. doi: 10.1371/journal.pone.0095049
Oftedal O. T., Gittleman J. L. (1989). “Patterns of energy output during reproduction in carnivores,” in Carnivore behavior, ecology, and evolution (Boston, MA: Springer), 355–378.
Patrick-Birdwell C., Avila-Villegas S., Neeley J., Misztal L. (2013). “Mapping and assessing the environmental impacts of border tactical infrastructure in the sky island region,” in Merging science and management in a rapidly changing world: Biodiversity and management of the madrean archipelago III and 7th conference on research and resource management in the southwestern deserts; 2012 may 1-5; Tucson, AZ. Eds. Gottfried G. J., Ffolliott P. F., Gebow B. S., Eskew L. G., Collins L. C. (Fort Collins, CO: US Department of Agriculture, Forest Service, Rocky Mountain Research Station), 365–369.
Peters R., Ripple W. J., Wolf C., Moskwik M., Carreón-Arroyo G., Ceballos G., et al. (2018). Nature divided, scientists united: US–Mexico border wall threatens biodiversity and binational conservation. BioScience 68 (10), 740–743. doi: 10.1093/biosci/biy063
Pietsch S. J., Hobson K. A., Wassenaar L. I., Tütken T. (2011). Tracking cats: problems with placing feline carnivores on δ18O, δD isoscapes. PloS One 6 (9), e24601. doi: 10.1371/journal.pone.0024601
Porter J. H., Dueser R. D., Moncrief N. D. (2015). Cost-distance analysis of mesopredators as a tool for avian habitat remediation on a naturally fragmented landscape. J. Wildl. Manage. 79 (2), 220–234. doi: 10.1002/jwmg.829
Povilitis T. (2015). Recovering the jaguar panthera onca in peripheral range: a challenge to conservation policy. Oryx 49 (4), 626–631. doi: 10.1017/S0030605313001361
Rabinowitz A., Zeller K. A. (2010). A range-wide model of landscape connectivity and conservation for the jaguar, panthera onca. Biol. Conserv. 143 (4), 939–945. doi: 10.1016/j.biocon.2010.01.002
R Core Team (2022). R: A language and environment for statistical computing (Vienna, Austria: R Foundation for Statistical Computing). Available at https://www.R-project.org/.
Rodríguez-Soto C., Monroy-Vilchis O., Zarco-González M. M. (2013). Corridors for jaguar (Panthera onca) in Mexico: conservation strategies. J. Nat. Conserv. 21 (6), 438–443. doi: 10.1016/j.jnc.2013.07.002
Rohweder J. J., De Jager N. R., Guntenspergen G. R. (2012). Anticipated effects of development on habitat fragmentation and movement of mammals into and out of the schoodic district, Acadia national park, Maine. US Geol. Surv. Sci. Invest. Rep. 5149, 30. doi: 10.3133/sir20125149
Rosas-Rosas O. C., Bender L. C., Valdez R. (2010). Habitat correlates of jaguar kill-sites of cattle in northeastern Sonora, Mexico. Human-Wildl. Interact. 4 (1), 103–111.
Rueda P., Mendoza G. D., Martínez D., Rosas-Rosas O. C. (2013). Determination of the jaguar (Panthera onca) and puma (Puma concolor) diet in a tropical forest in San Luis potosi, Mexico. J. Appl. Anim. Res. 41 (4), 484–489. doi: 10.1080/09712119.2013.787362
Sadowski-Smith C. (2013). “US Border ecologies, environmental criticism, and transnational American studies,” in American Studies, ecocriticism, and citizenship(Abingdon-on-Thames, Oxfordshire, U.K:, Routledge), 164–177.
Sanderson E. W., Beckmann J. P., Beier P., Bird B., Bravo J. C., Fisher K., et al. (2021). The case for reintroduction: The jaguar (Panthera onca) in the united states as a model. Conserv. Sci. Pract. 3 (6), e392. doi: 10.1111/csp2.392
Sanderson E. W., Fisher K., Peters R., Beckmann J. P., Bird B., Bradley C. M., et al. (2022). A systematic review of potential habitat suitability for the jaguar panthera onca in central Arizona and new Mexico, USA. Oryx 56 (1), 116–127. doi: 10.1017/S0030605320000459
Saxena A., Habib B. (2022). Crossing structure use in a tiger landscape, and implications for multi-species mitigation. Transportation Research Part D: Transport and Environment, 109, 103380 doi: 10.2139/ssrn.4032623
Shepard E. L., Wilson R. P., Rees W. G., Grundy E., Lambertucci S. A., Vosper S. B. (2013). Energy landscapes shape animal movement ecology. Am. Nat. 182 (3), 298–312. doi: 10.1086/671257
Stander P. E., Haden P. J., Kaqece I. I., Ghau I. I. (1997). The ecology of asociality in Namibian leopards. J. Zool. 242 (2), 343–364. doi: 10.1111/j.1469-7998.1997.tb05806.x
Stoner K. J., Hardy A. R., Fisher K., Sanderson E. W. (2015). “Jaguar habitat connectivity and identification of potential road mitigation locations in the northwestern recovery unit for the jaguar,” in Fish and wildlife service in response to solicitation (New York, NY, USA: Wildlife Conservation Society final draft report to the U.S), 29. Available at: https://databasin.org/datasets/ac5dae675c604589a3f310f9458f33b5/.
Strauss W. M., Hetem R. S., Mitchell D., Maloney S. K., O'Brien H. D., Meyer L. C., et al. (2017). Body water conservation through selective brain cooling by the carotid rete: a physiological feature for surviving climate change? Conserv. Physiol. 5 (1), 1–15. doi: 10.1093/conphys/cow078
Svancara C. M., Lien A. M., Vanasco W. T., Lopez-Hoffman L., Bonar S. A., Ruyle G. B. (2015). Jaguar critical habitat designation causes concern for southwestern ranchers. Rangelands 37 (4), 144–151. doi: 10.1016/j.rala.2015.05.003
Thompson J. J., Velilla M. (2017). Modeling the effects of deforestation on the connectivity of jaguar panthera onca populations at the southern extent of the species’ range. Endang. Species Res. 34, 109–121. doi: 10.3354/esr00840
Titley M. A., Butchart S. H., Jones V. R., Whittingham M. J., Willis S. G. (2021). Global inequities and political borders challenge nature conservation under climate change. Proc. Natl. Acad. Sci. 118 (7), e2011204118. doi: 10.1073/pnas.2011204118
Traphagen M. B. (2021). “The border wall in Arizona and NewMexico-July 2021,” in Wildlands network story map. (Tucson, AZ, USA: Wildlands Network) Available at: https://storymaps.arcgis.com/stories/8532c503c2084293bb8847407245228d.
U.S. Fish and Wildlife Service (USFWS) (2014). Endangered and threatened wildlife and plants; designation of critical habitat for jaguar: final rule. Fed. Regist. 79, 12572–12654.
U.S. Fish and Wildlife Service (USFWS) (2018). Jaguar recovery plan (Panthera onca) (Albuquerque, New Mexico: U.S. Fish and Wildlife Service, Southwest Region).
U.S. Geological Survey (USGS) (2017). 1/3rd arc-second digital elevation models (DEMs) - USGS national map 3DEP downloadable data collection: U.S. Geol. Surv.
Villarreal M. L., Haire S. L., Bravo J. C., Norman L. M. (2019). A mosaic of land tenure and ownership creates challenges and opportunities for transboundary conservation in the US-Mexico borderlands. Case Stud. Environ. 3 (1), 1–10. doi: 10.1525/cse.2019.002113
Vosper S. (2003). Development and testing of a high resolution mountain-wave forecasting system. Meteorol. Appl.: A J. Forecast. Pract. Appl Training Tech Model. 10 (1), 75–86. doi: 10.1017/S1350482703005085
Wilmers C. C., Isbell L. A., Suraci J. P., Williams T. M. (2017). Energetics-informed behavioral states reveal the drive to kill in African leopards. Ecosphere 8 (6), e01850. doi: 10.1002/ecs2.1850
Keywords: cost distance, jaguar, ecophysiology, natural recolonization, international border, bioenergetics, GIScience, habitat restoration
Citation: Chambers SN, Villarreal ML, Norman LM, Bravo J C and Traphagen MB (2022) Spatial models of jaguar energy expenditure in response to border wall construction and remediation. Front. Conserv. Sci. 3:1012010. doi: 10.3389/fcosc.2022.1012010
Received: 04 August 2022; Accepted: 10 October 2022;
Published: 28 October 2022.
Edited by:
Mohammad Farhadinia, University of Oxford, United KingdomReviewed by:
Stella De La Torre, Universidad San Francisco de Quito, EcuadorJohn Polisar, Zamorano, Honduras
Copyright © 2022 Chambers, Villarreal, Norman, Bravo and Traphagen. This is an open-access article distributed under the terms of the Creative Commons Attribution License (CC BY). The use, distribution or reproduction in other forums is permitted, provided the original author(s) and the copyright owner(s) are credited and that the original publication in this journal is cited, in accordance with accepted academic practice. No use, distribution or reproduction is permitted which does not comply with these terms.
*Correspondence: Samuel N. Chambers, schambers@usgs.gov