- 1Ashoka Trust for Research in Ecology and the Environment, Bangalore, India
- 2Manipal Academy of Higher Education, Manipal, India
- 3Wildlife Conservation Trust, Mumbai, India
- 4School of Environment and Sustainability, Indian Institute for Human Settlements, Bangalore, India
- 5National Centre for Biological Sciences-Tata Institute of Fundamental Research (TIFR), Bangalore, India
- 6School of Biology, University of Leeds, Leeds, United Kingdom
Effects of land-cover change on insectivorous bat activity can be negative, neutral or positive, depending on foraging strategies of bats. In tropical agroforestry systems with high bat diversity, these effects can be complex to assess. We investigated foraging habitat use by three insectivorous bat guilds in forests and rubber plantations in the southern Western Ghats of India. Specifically, we monitored acoustic activity of bats in relation to (1) land-cover types and vegetation structure, and (2) plantation management practices. We hypothesized that activity of open-space aerial (OSA) and edge-space aerial (ESA) bat guilds would not differ; but narrow-space, flutter-detecting (NSFD) bat guild activity would be higher, in structurally heterogeneous forest habitats than monoculture rubber plantations. We found that bat activity of all guilds was highest in areas with high forest cover and lowest in rubber plantations. Higher bat activity was associated with understorey vegetation in forests and plantations, which was expected for NSFD bats, but was a surprise finding for OSA and ESA bats. Within land-cover types, open areas and edge-habitats had higher OSA and ESA activity respectively, while NSFD bats completely avoided open habitats. In terms of management practices, intensively managed rubber plantations with regular removal of understorey vegetation had the lowest bat activity for all guilds. Intensive management can undermine potential ecosystem services of insectivorous bats (e.g., insect pest-control in rubber plantations and surrounding agro-ecosystems), and magnify threats to bats from human disturbances. Low-intensity management and maintenance of forest buffers around plantations can enable persistence of insectivorous bats in tropical forest-plantation landscapes.
Introduction
Tropical forest fragmentation caused by agricultural intensification and expansion of commercial agroforestry plantations is a significant threat to biodiversity (Saunders et al., 1991; Raman, 2006; Gardner et al., 2009; Kumar et al., 2010; Canale et al., 2012). Generally irreversible or one-way conversion of primary forest habitat to monoculture plantations is among the main causes of forest fragmentation (FAO and UNEP, 2020). In forest-plantation mosaics, the spatial configuration of land-cover types is an important determinant of species persistence, movement, foraging, and reproductive success (Turner, 1996; Kumar et al., 2002; Henle et al., 2004; Canale et al., 2012). Within land-cover types, habitat use by different species of small mammals, such as insectivorous bats, may be driven by vegetation structure, habitat edges, human land-uses, and management practices (Dauber et al., 2003; Henle et al., 2004; Harvey and Villalobos, 2007). Landscape-scale habitat configuration and alterations in local habitat structure can thus affect the movement and dispersal of insectivorous bats (Erickson and West, 2003; Duchamp and Swihart, 2008; Meyer et al., 2008).
Depending on the dispersal ability and ranging behavior of insectivorous bats, their responses to habitat conversion, local habitat complexity, and landscape context can vary substantially (Gorresen and Willig, 2004; Kusch and Schotte, 2007). Jones et al. (2009) suggest that bats are potential bio-indicators due to their tractable population-level responses (in terms of activity) to land-cover change. Yet, studies on bat responses to land-cover change have yielded equivocal conclusions for different bat species (Estrada-Villegas et al., 2010; Frey-Ehrenbold et al., 2013; Ducci et al., 2015). Insectivorous bat responses to habitat conversion in terms of activity can range from negative (reduced foraging activity: Estrada-Villegas et al., 2010) to neutral (no difference in activity: Davy et al., 2007) to even positive (increased activity; Ethier and Fahrig, 2011). Responses may vary by the type of biome (temperate/tropical: Erickson and West, 2003; Heer et al., 2015), degree and nature of land conversion and agricultural intensification (Gorresen and Willig, 2004; Frey-Ehrenbold et al., 2013), sampling scale (local to landscape: Erickson and West, 2003; Gehrt and Chelsvig, 2003; Meyer et al., 2008), and regional species diversity and trait distributions (Duchamp and Swihart, 2008; Meyer et al., 2008; Ducci et al., 2015).
Understanding the influence of land-cover types on insectivorous bats needs an understanding of the diversity of their foraging strategies. Bat foraging strategies can be understood as summaries of functional trait diversity in the local species pool. Insectivorous bat “foraging guilds” can be categorized by echolocation characteristics across bat species (Siemers and Schnitzler, 2004; Denzinger and Schnitzler, 2013). Accounting for functional trait diversity (and not only species diversity) can help understand the responses of different bat guilds to land-cover change and habitat fragmentation (Siemers and Schnitzler, 2004; Ober and Hayes, 2008; Klingbeil and Willig, 2009; Cadotte et al., 2011; Frey-Ehrenbold et al., 2013). For example, Estrada-Villegas et al. (2010) showed that “forest-dependent” aerial insectivores were affected negatively by forest loss unlike “open-space” foragers that were largely unaffected. Guild-level responses can also be more intuitive to understand and provide an efficient way to monitor land-cover change impacts on ecosystem services from insectivorous bats (Cadotte et al., 2011).
In this study, we assessed the effects of (1) land-cover type (forests, rubber plantations, teak plantations, and settlements) and vegetation structure (stratification, height, and canopy cover) in forests and plantations, and (2) plantation management practices (e.g., clearing of understorey vegetation, pesticide use), on foraging habitat use by three insectivorous bat guilds in the Western Ghats biodiversity hotspot of India. These variables were expected to influence insect abundance, and the ability of bats to navigate, and in turn affect bat activity. We acoustically sampled bat activity in forests, rubber plantations, and adjacent land-cover types (teak woodlands and human settlements) in forest-plantation mosaics. We classified bat guilds as “Open-Space Aerial (OSA)” foragers, “Edge-Space Aerial (ESA)” foragers and “Narrow-Space Flutter Detecting (NSFD)” foragers, based on prey detection ranges and aerospace use (Denzinger and Schnitzler, 2013). OSA bats emit low-frequency echolocation calls with quasi-constant frequency modulations to detect flying insects in open air, ESA bats use mid-range, frequency-modulated calls to detect aerial insect prey mostly along habitat edges, and NSFD bats use mid- to high-range constant frequency calls to detect insects against cluttered vegetation (Schnitzler and Kalko, 2001; Siemers and Schnitzler, 2004; Denzinger and Schnitzler, 2013). As vegetation structure was expected to be more complex in tropical forests than rubber plantations, we expected differential activity of the three bat guilds. Accordingly, we hypothesized that the activity of OSA and ESA bat guilds would not differ between forests and rubber plantations. For NSFD bats, we expected higher activity in forest habitats than rubber plantations. Additionally, we predicted that human management of rubber plantations would alter vegetation structure and influence bat activity. Therefore, we also investigated the processes by which management practices might have affected bat activity within rubber plantations.
Materials and Methods
Study Area
In India's Western Ghats, a major driver of forest fragmentation has been expansion of commercial plantations (e.g., coffee, rubber, oil-palm; Jha et al., 2000; Kumar et al., 2002; Anand et al., 2010). Our study was conducted in a rubber plantation-dominated agroforestry landscape surrounding the Shenduruney Wildlife Sanctuary (Lat: 8°54.084′ N, Long: 77°08.162′ E), in the Agasthyamala hills, Kerala,in the Western Ghats. The state of Kerala accounts for the bulk of natural rubber production in India. Mass conversion of forests to rubber plantations in Kerala began about 70 years ago and rubber area expansion has been over 600% in this period (Kumar, 2005). Apart from the major land-cover classes of contiguous and fragmented semi-evergreen/moist-deciduous forests and rubber plantations, the landscape includes teak plantations, fruit and spice orchards, human settlements, and matchwood plantations (Figure 1). The forests of this landscape have a remarkable diversity of plants, fishes and amphibians, including many endemics. The study area covers the western slopes of the southern Western Ghats from 65 to 1,300 m above mean sea level, of a topographically complex and rugged horst-and-graben type mountain range. The region receives an average annual rainfall of c. 2,800 mm, with the Indian summer monsoon season duration from June to September (Nair, 1991).
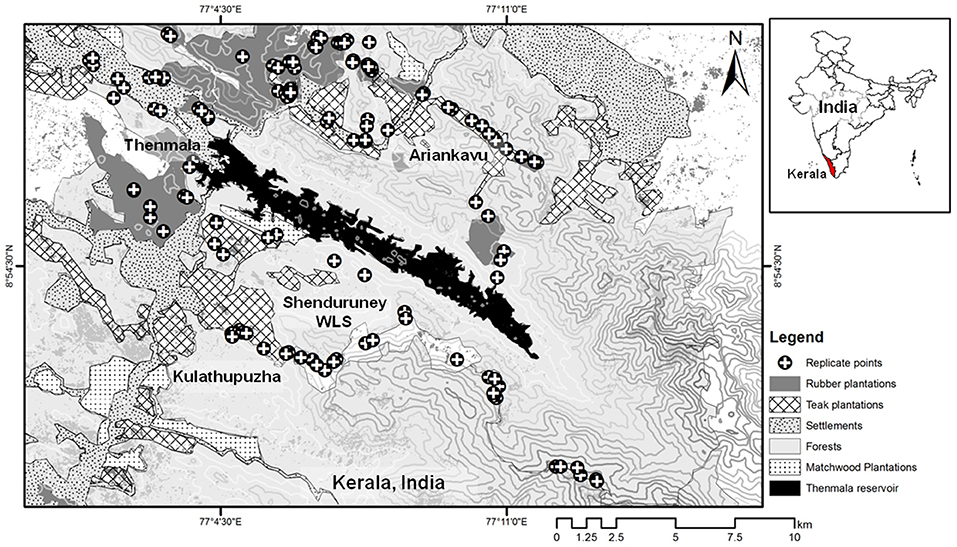
Figure 1. Map of the study area with land-cover types and sampling locations of bat foraging activity and ecological covariates.
Study Design
We selected sampling locations corresponding with four land-cover types: forests (n = 22 locations), rubber plantations (n = 20), teak woodlands (n = 13), and human settlements (n = 11). The numbers of locations were roughly proportional to the areal extents (in km2) of land-cover types in our effective sampling region (forests: 69.19 km2, rubber: 40.13 km2, teak: 28.63 km2, and settlements: 15.6 km2). Locations were also chosen based on accessibility due to safety-based restrictions, topographic variables (e.g., steep slope), rugged terrain conditions, and thorny vegetation (e.g., canebrakes). Hence, in many forested areas, we had to follow small paths and dirt roads for sampling (Figure 1). Location coordinates were logged in a Global Positioning System (Garmin eTrex Vista HCx). We sampled bat activity and habitat variables at replicate points within each location to increase spatial coverage of the land-cover types available there. Sampling was conducted during the dry-season (December to May) on clear evenings in 2011–12, with additional site visits in the dry-season in 2014 and 2019. The dry-season was defined according to the typical duration of the Indian summer monsoon season (see study area section).
Acoustic Sampling of Bat Activity
The echolocation call rate of insectivorous bats, calculated from recordings of bat ultrasound calls, indicates relative bat activity across different habitats (Vaughan et al., 1997; Davy et al., 2007; Williams-Guillén and Perfecto, 2011). Bat activity is considered a useful index of foraging habitat use as bats generally show site fidelity (Kapfer et al., 2008; Berthinussen and Altringham, 2012). At the selected sampling locations, we recorded bat echolocation calls with a handheld D240X detector (Pettersson Elektronik AB, Uppsala, Sweden1) with high-gain automatic detection and 10× time expansion factor, and stored in a recorder (Edirol R-09HR, Roland). The sampling rate of the detector was 307 kHz and detector frequency range from 10 to 120 kHz. This sampling rate was adequate in resolution for accurate estimation of characteristics of most bat species commonly encountered in the landscape (Brigham et al., 2004). D240X detectors are thought to be highly efficient compared to similar detector types in their detection range and directionality (Sprong et al., 2012; Kerbiriou et al., 2019). Given our financial constraints and for parity with other contemporaneous studies in this region (e.g., Wordley et al., 2014, 2015), this was the best available detector.
Our pilot sampling (at 15 points across land-cover types) with the D240X detector revealed that bat activity was substantially greater in recordings in the dusk and early night hours (1830–2000 h) as compared to later night hours (2000–2230 h) at all sites. Therefore, we focused our effort on the dusk activity peak, post bat emergence from roosts (as done by Hayes, 1997; Davy et al., 2007; Skalak et al., 2012) for sampling the majority of common species in the three guilds. Sampling began half an hour after sunset and was completed over 3 h after sunset (following Berthinussen and Altringham, 2012). Due to work safety issues, overnight sampling was not possible.
Overall, within the 66 locations across land-cover types, we sampled a total of 157 spatial replicate points. We varied the number of replicate points from 2 to 4 within each location, to avoid potential oversampling of bat calls in small areas and to adequately cover larger areas (Siemers and Schnitzler, 2004; Cel'uch and Zahn, 2008; MacSwiney et al., 2008; Frey-Ehrenbold et al., 2013). The spatial replicate points within each location were at least ~200 m apart for independence (Mullin et al., 2020; Yoh et al., 2020), at distances much more than the known detection distances for D240X detectors (Sprong et al., 2012; Kerbiriou et al., 2019). Typically, two land-cover types were sampled per night, by staggering the order of sampled points among land-cover types, to allocate similar effort to both. At each point, 10-min recordings were conducted, separated on average by 20-min intervals, which was the time taken to move between points, owing to terrain conditions. Several studies (e.g., Williams-Guillén and Perfecto, 2011; Hogue and McGowan, 2018; Mullin et al., 2020) have conducted replicated recordings of 10–12 min and confirmed this temporal duration to yield reliable estimates of bat activity in habitat mosaics. Thus, we made 367 echolocation call recordings at the 157 points (mean recording time per point = 21.5 min, range 20–40 min, total effort of c. 3380 min over 60 nights).
Bat Guild Assignments and Acoustic Analyses
We searched and visited bat roosts in the landscape to visually confirm bat species identification based on morphological keys (Bates and Harrison, 1997) and regional checklists (e.g., Korad et al., 2007). We then recorded bat resting frequencies and characteristic in-flight echolocation calls from roosting individuals and emerging bats at flyways near roost exits (Biscardi et al., 2004; Brigham et al., 2004; Fraser et al., 2020). We validated our recorded calls with available information on the peak, start, and end frequencies, inter-pulse intervals, call duration, etc. of Indian bats from previous studies (Neuweiler et al., 1984; Raghuram et al., 2014; Wordley et al., 2014; Deshpande and Kelkar, 2015).
Using acoustic parameters such as Frequency with maximum Energy (FmaxE) and call structure [Constant-Frequency (CF), Frequency-Modulation (FM), and Quasi-Constant-Frequency (QCF)], calls were identified as representative or reference calls of bat species confirmed at roosts (Schnitzler and Kalko, 2001; Siemers and Schnitzler, 2004; Denzinger and Schnitzler, 2013; Frey-Ehrenbold et al., 2013). Acoustic analyses of individual calls were carried out manually in the software BatSound Pro v.3.32 (Pettersson Elektronik AB, Uppsala, Sweden). All recordings were analyzed using sampling frequency of 44.1 kHz at FFT size of 1,024 samples with 95% overlap in a hanning window. We recorded a total of 25 insectivorous bat species in all sampled foraging locations. From our reference calls, data on 23 species (sample sizes of 51–114 bat calls each) could be used for unambiguous guild assignment. Ambiguous, unclear, or interrupted calls were omitted and only calls with high signal to noise ratio were considered for further analyses. Based on spectral signatures and acoustic detection ranges of different species, we assigned all usable calls to one of three foraging guilds of insectivorous bats: “Open-Space Aerial (OSA)” foragers, “Edge-Space Aerial (ESA)” foragers, and “Narrow-Space Flutter Detecting (NSFD)” foragers (Schnitzler and Kalko, 2001; Denzinger and Schnitzler, 2013). In our study, the OSA guild included the families Emballonuridae and Molossidae, ESA included Vespertilionidae and Miniopteridae, and NSFD included Rhinolophidae (details in Table 1). For all spatial replicate points in our sampling locations, we manually calculated bat activity (echolocation call rate or bat passes per unit time) for each guild, from the software BatSound Pro.
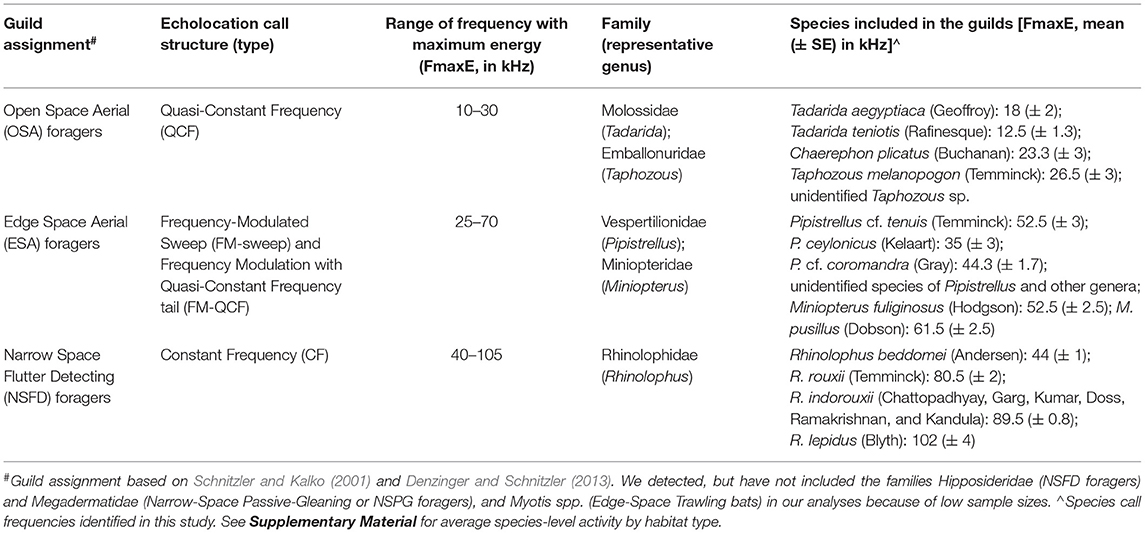
Table 1. Description of assigned bat foraging guilds according to echolocation call signatures and included species with frequencies recorded from the study area.
Habitat Variables
We defined four land-cover classes as (1) forests, (2) rubber plantations, (3) teak woodlands, and (4) village settlements, based on ground surveys and supervised classification of bands 2 (blue), 3 (green), and 4 (red) of Landsat OLI TIRS Level-1 images (30 m × 30 m spatial resolution) from April 2014, which was the dry-season after our main sampling period in 2011–12. The reason to not use Landsat images from 2011 to 12 was that they had a striping problem, which would have affected our classification. Our extensive ground surveys from 2011 to 2014 indicated negligible change in land-cover types since 2011 to 12, and helped us accurately classify smaller patches of land-cover types. We also used topographic maps to identify settlements and established rubber estates (1:25,000 cm; Survey of India, surveyed in 1976–77) in the region. For supervised classification, we used a Maximum Likelihood-based method from 50 training sites that were used to generate spectral signatures of forests, rubber plantations, teak woodlands, village settlements, and fruit orchards in Idrisi Selva software (ClarkLabs, 2012). Supervised classification resulted in a user accuracy rate of above 90% for the first four land-cover categories. Fruit orchards were very small in area and for analyses, thus, these locations were assigned mostly to settlements near fruit orchards. Around each acoustic sampling point (n = 157), the percent area under the above land-cover types within circular buffers of area 2 km2 (radius of 0.8 km) was extracted in Quantum GIS 2.4.0 (QGIS Development Team, 2018). The buffer area was chosen to represent approximate average daily foraging ranges of different bat guilds. No tagging studies were available for the three guilds from South or Southeast Asia, so we borrowed information on daily foraging ranges from other studies. We used studies from Europe and Australia on bats from the three guilds (NSFD: Bontadina et al., 2002; OSA: Marques et al., 2004; ESA: Gonsalves et al., 2013). Prakash et al. (2021) tagged insectivorous bats in the Western Ghats (Megaderma spasma, not included in our analyses), and found highly localized foraging activity.
Effects of percent land-cover types in surrounding buffers (representing the landscape context) were tested on bat activity recorded at each point in particular habitats (similar to Gili et al., 2020). Variables representing vegetation structure (e.g., overstorey canopy cover, tree height, presence of understorey, and lianas) and habitat edges (e.g., roads, streams, and open areas) were recorded at each acoustic sampling point (details of habitat variable measurements in Table 2). These variables were chosen in relation to our guild-specific predictions (see section Introduction). Vegetation measurements were conducted before we began acoustic recordings at dusk. The same observer conducted visual estimation of tree height and canopy cover. Visual estimates were initially calibrated with a clinometer and laser rangefinder for accuracy. Understorey vegetation, lianas, and habitat edges were recorded as categorical variables based on presence or absence, and size (e.g., for roads). At each point, we also extracted data on terrain, elevation, slope, and aspect from toposheets (1:25,000 cm; Survey of India), digital elevation models (ASTER 30 m), and satellite imagery (Landsat OLI TIRS2). We expected topographic variables to influence bat activity in interaction with local habitat conditions, so these were included later as additional covariates in analyses.
Statistical Analyses
We computed species accumulation curves with the Jack-knife II estimator from the cumulative recording effort (Moreno and Halffter, 2000). Species accumulation curves for forests and rubber plantations indicated adequate sampling effort, although teak plantations and settlements, which were smaller in extent, did not show a clear asymptote (Figure 2).
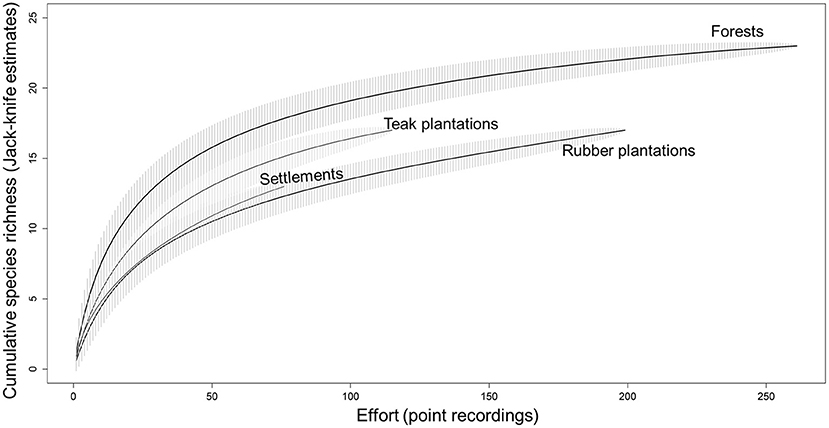
Figure 2. Species accumulation curve based on Jack-knife II estimation of species sampled across different land-cover types.
Prior to analyses, we first standardized all point-level data on guild-wise bat activity to 10 min. We also tested for spatial autocorrelation in guild-wise bat activity as a result of proximity of replicate points, by estimating sill and range parameters of omnidirectional variogram models (Fortin and Dale, 2005). Variograms poorly fit bat activity data in relation to distances between recording points, indicating no clear effects of spatial autocorrelation or suggesting spatially localized habitat use by bats. For the three guilds, variogram range was approximately near to or above 2 km (more than twice of our buffer radius). To avoid effects of spatial autocorrelation due to any overlapping buffers, we used a randomized subsampling procedure (generating 100 sub-samples with similar sample sizes from all land-cover types) by first thinning the data to 50% of the sample size by removing points <1 km apart, and running correlation and regression analyses (Segurado et al., 2006). The poor variogram fits could also be because of the detection range of D240X detectors, and variable detection distances of different species (Kerbiriou et al., 2019) or guilds. So, we separately analyzed guild-wise activity in relation to habitat variables, to avoid biases resulting from differential detection of species upon pooling bat activity across guilds (Kerbiriou et al., 2019).
Proportional or percent (%) cover is a simple but useful indicator that can represent effects of surrounding habitat on species (Watling et al., 2011). We analyzed acoustic activity of bat guilds in relation to percent of land-cover types within buffers (effect of landscape context). We estimated bat activity in (1) rubber plantation points at different % cover of forest in the buffer, and (2) in forest points with different % cover of rubber plantations in the buffer. We performed Spearman's rank-order correlation tests and evaluated correlation coefficients for univariate associations between bat activity and percent cover for consistency in sign, magnitude, and statistical significance. We then ran multiple regression models to estimate the effects of different covariates on guild-wise bat activity. Model covariates included landscape context (% cover in 2 km2 buffer), vegetation structure (canopy, understorey, and tree height), and habitat edge variables (roads, streams, and open areas) measured at recording locations within habitat types. Only combinations of uncorrelated covariates (correlation < 0.30) were included in the model. We found high occurrence of zeroes in bat activity data (50–90%), arising both from “absence” (structural zeroes) and “no detection” of bat calls at sites (He et al., 2014). Hence, we chose zero-inflated generalized linear models with Poisson errors (ZIP GLMs). ZIP GLMs achieved better model convergence and fit over other candidate models, e.g., Poisson, negative binomial, or zero-inflated negative binomial GLMs. Model fit was additionally calculated using the Cox-and-Snell Pseudo-R2 statistic (Williams, 2019), which provides an absolute measure of model improvement after adding covariates to a null model (i.e., model without covariates). ZIP GLMs were run in the package “pscl” in the software R 3.2.3 (R Core Team, 2018). Model selection was based on Akaike weights of models calculated from the Akaike Information Criterion (AIC; Burnham and Anderson, 2002). Models with lower AIC values were selected, if the difference between AICs of the two best comparable model AICs was >2. We combined the use of pseudo-R2 and AIC for careful model selection, so that we could compare among models for their absolute fit (as improvement over null models) as well as relative fit and parsimony.
Effects of Plantation Management on Vegetation Structure and Bat Activity
We surveyed 30 rubber plantations in 19 locations to compile qualitative categorical information on the varieties of rubber planted (new: high-yielding, and mixed: new + old), and management intensity. We recorded directly visible indicators of management intensity as: (1) presence or absence of understorey vegetation, indicating frequency of clearing or removal of natural vegetation and weeding, and (2) pesticide use, from direct field observations as well as through information provided by plantation managers and workers. Based on the above factors, we classified rubber plantations as low-management, moderate-management, or high-management, reflecting management intensity. A low-management plantation was typically one with relatively intact understorey vegetation and no pesticide use, medium-intensity plantations had any one of the two indicators or intermediate levels of both (e.g., thinner understorey and occasional pesticide use), and high-management plantations had both indicators of intensive management at high levels. Pesticide application frequency and rubber tapping frequency were both higher in high-management plantations. We then compared guild-wise bat activity between these plantation management categories, using non-parametric Kruskal–Wallis tests and Dunn tests.
Results
Effects of Land-Cover Type on Guild-Wise Bat Activity Within Buffers (Landscape Context)
Average activity of NSFD bats was almost 10 times greater in forests (10.06 passes/h) than in all other land-cover types (Table 3). Average ESA bat activity was higher in forests (34.1 passes/h) as compared to rubber (26.2), teak plantations (19.9), and settlements (18.7 passes/h; Table 3). Average OSA bat activity was highest in settlements and teak plantations (14 and 9.4 passes/h), followed by forests and rubber plantations (4.28 and 2.54 passes/h; Table 3). Activity of all bat guilds was positively correlated with percent forest cover and negatively with percent rubber in the surrounding buffers of 2 km2 (Table 4). Average OSA and ESA activity was highest (1.25 and 5.2 passes/h, respectively) in buffers with forest cover >25% and <75% (Figure 3). For NSFD bats, 97% of total activity was recorded in areas with >75% forest cover in buffers (Figure 3). NSFD activity was present in rubber plantations only if the buffer had over 80% forest cover (Figure 3). In contrast, if buffers had more than 50% rubber plantation cover, low bat activity was recorded (OSA: 1.1 passes/h, ESA: 14.7 passes/h, and NSFD: 0.23 passes/h).
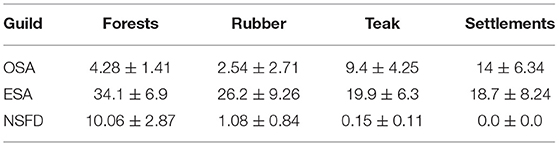
Table 3. Bat activity (mean ± SE of bat passes per hour) of the three guilds across the four land-cover types.
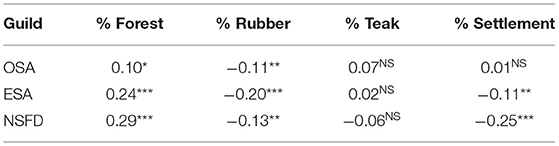
Table 4. Associations (Spearman's rank correlation coefficients) of bat guild activity with land-cover extent (***p < 0.001, **p < 0.01, *p < 0.05, and NS = Not Significant), indicating positive association with % forest cover and negative association with % rubber plantation and % settlement area in 2 km2 buffers.
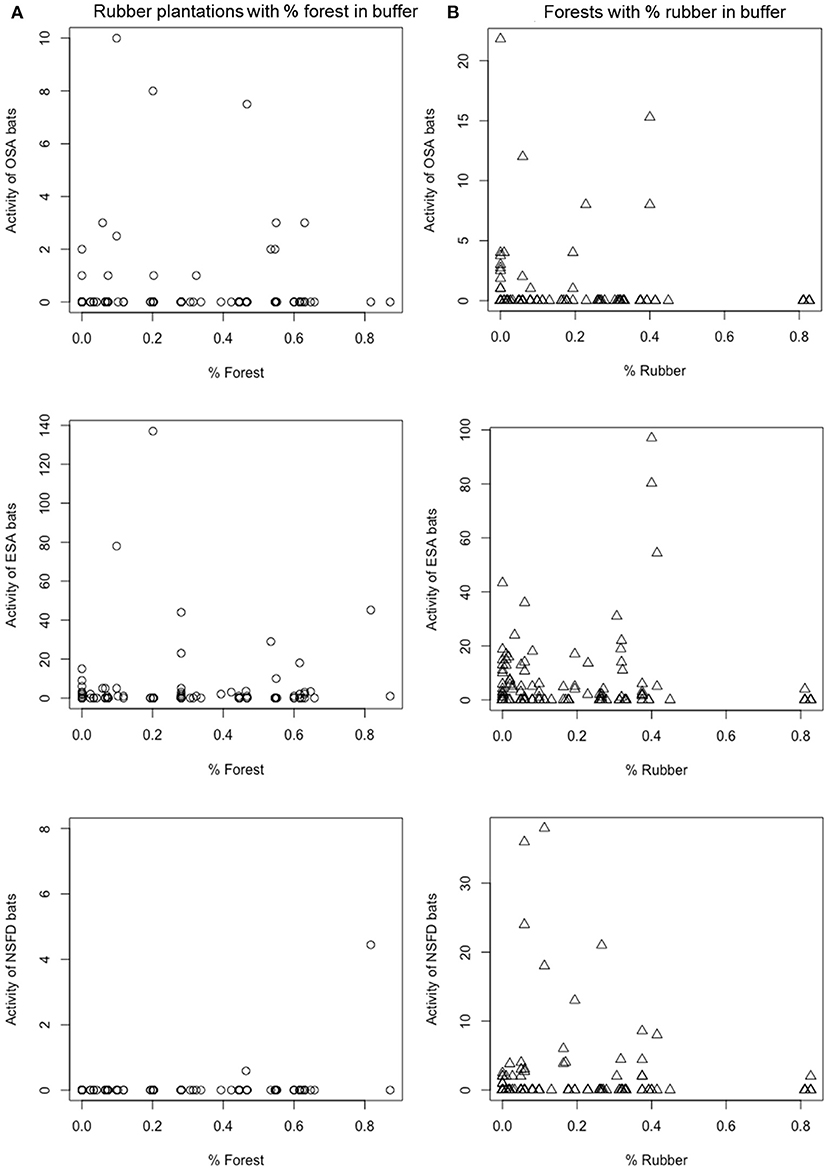
Figure 3. Time-standardized activity (bat passes per 10 min) of insectivorous bat guilds in rubber plantations (A) and forests (B) in relation to landscape context, i.e., % forest (A) and % rubber (B) cover in the surrounding buffer.
Effects of Vegetation Structure and Habitat Edges on Guild-Wise Bat Activity
Bat activity of all guilds, irrespective of the association with forest cover, was positively associated with the presence of understorey vegetation (Table 5; Figure 4). Across all land-cover types, OSA, ESA, and NSFD bat activity levels were thrice, twice, and 5.5 times higher in the presence of understorey vegetation (mean ± SE: 6.4 ± 1.5, 30.5 ± 5.6, and 5.5 ± 1.5 bat passes/h, respectively) than in its absence. Forest understorey was structurally heterogeneous (multi-layered) with mostly native plant species, whereas rubber understorey was homogenous (single-layered) and often composed of one or two invasive plant species like Lantana camara and Chromolaena odorata. The high activity of NSFD bats in forests with understorey vegetation was as expected, but the response of OSA and ESA bat activity to understorey vegetation was a surprise finding. Effect sizes of understorey vegetation on bat guilds varied as NSFD > ESA > OSA (Figure 4). NSFD bat activity was positively associated with old-growth forests with tall trees and dense canopy cover (Figure 4). NSFD bats were absent in open habitats such as settlements (Table 5; Figure 4), as expected. OSA and ESA bat activity were negatively associated with tree height (Table 5; Figure 4). ESA activity was positively associated with habitat edges, especially streams and small roads in forests and plantations (Table 5).
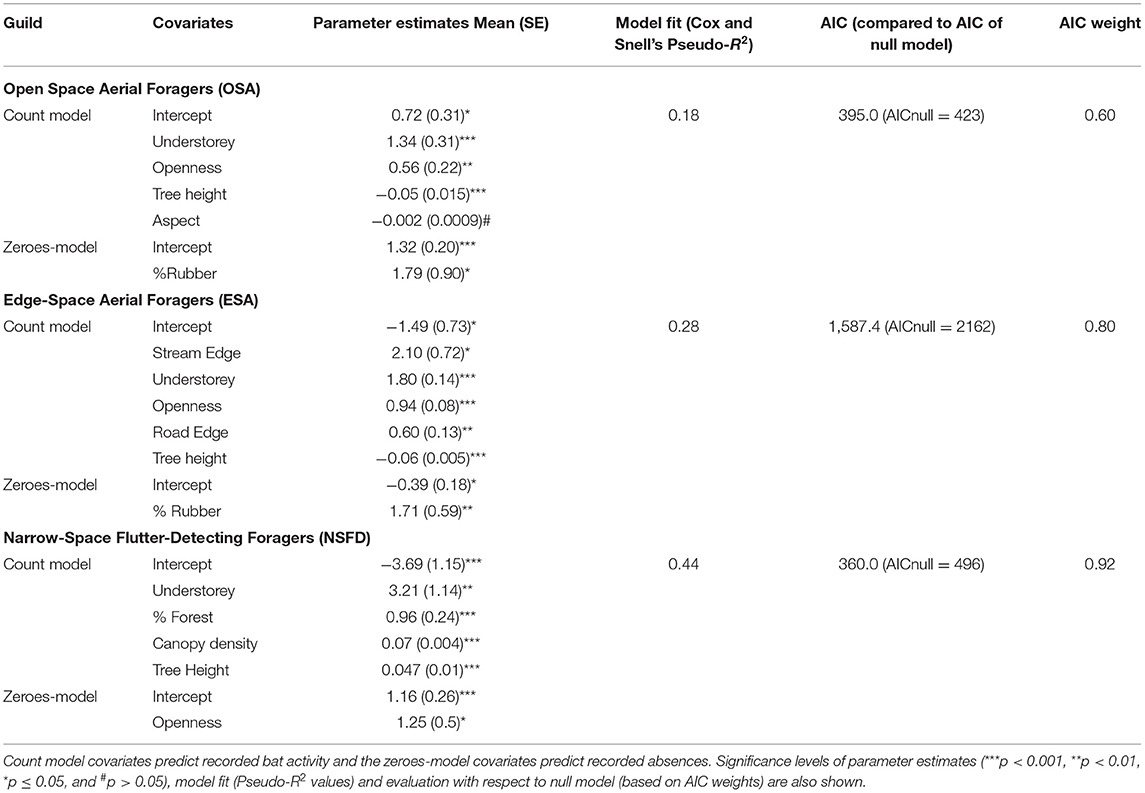
Table 5. Summaries of selected zero-inflated Poisson (ZIP) generalized linear models (GLMs) with effect sizes (parameter estimates) of habitat variables on bat activity of OSA, ESA, and NSFD bat guilds.
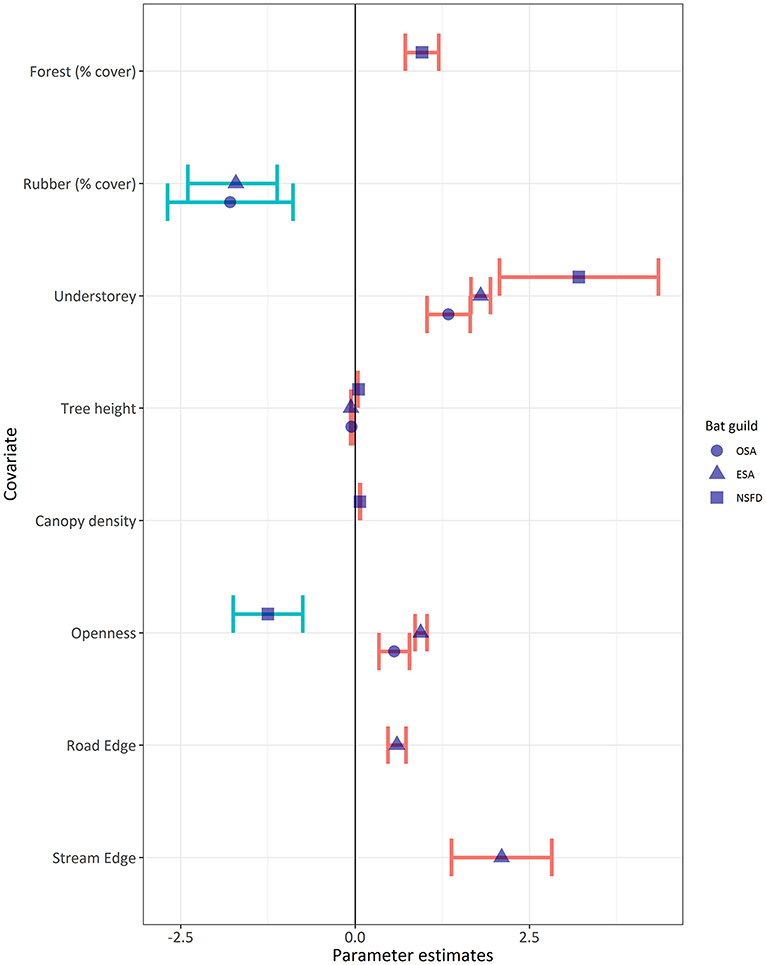
Figure 4. Comparison of effect sizes (mean ± SE of parameter estimates: Table 5) of habitat variables on bat activity of the OSA, ESA, and NSFD guilds. Understorey vegetation positively influenced activity of all bat guilds, but other variable effects differed. Variables explaining zero bat activity are denoted by blue error bars, and variables influencing non-zero bat activity are shown with red error bars.
Effects of Rubber Plantation Management Practices
Almost no activity of OSA bats (mean ± SE: 1 ± 0.16 bat pass/h) was recorded in the 30 rubber plantations selected to test the effects of management practices. Total ESA bat activity was higher in low- (144 ± 62 bat passes/h) and moderate-management rubber plantations (120 ± 30.5 passes/h) than those with high-management intensity (27 ± 10.6 passes/h). Here, the difference between moderate- and high-management intensity categories was statistically significant [Kruskal–Wallis (K–W) test: χ2 = 3.79, df = 2, and P = 0.15; Dunn's test: moderate vs. high, P = 0.03). Total NSFD bat activity was greater in low- (17.5 ± 4.1 passes/h) and moderate-management (11 ± 2.8) than high-management rubber plantations (0.75 ± 0.39), and differences were statistically significant (K–W test: χ2 = 5.94, df = 2, P = 0.05; Dunn test: low vs. high: P = 0.02; moderate vs. high: P = 0.01; low vs. moderate: P = 0.40). Importantly, NSFD bats showed activity in rubber plantations only when understorey vegetation was present. Total ESA bat activity was higher when understorey was present in rubber (138 ± 43.4 passes/h) than in its absence (60 ± 23.7), but differences were not statistically significant (K–W test: χ2 = 0.675, P = 0.41). About 61% of the rubber plantations removed understorey vegetation during weeding operations. Total ESA activity was also significantly higher (K–W test: χ2 = 1.74, P = 0.04) in rubber plantations without pesticide use (206.4 ± 49.5 passes/h) than those with regular pesticide use (65 ± 4.1). Total ESA and NSFD bat activity was on average much greater in plantations with mixed planting of old and new rubber varieties (162 and 18 passes/h), than in monocultures of new rubber varieties alone (97.8 and 9 passes/h).
Discussion
Consistent Positive Effects of Forest Cover on Activity of Bat Guilds
Activity of all bat guilds was consistently higher in forests than in rubber plantations in the Shenduruney Wildlife Sanctuary landscape of Kerala's Western Ghats. Similar positive effects of forest cover have also been reported on insectivorous bats in other plantation agroforestry regions of the Western Ghats. For instance, Molur and Singh (2009) reported higher bat activity in forest fragments than coffee plantations in the Western Ghats of Kodagu. In the Anamalai hills, Wordley et al. (2015) also reported lower bat activity in tea plantations as compared to shade-coffee plantations and natural forests. Tea plantations with forest fragments had higher activity than tea plantations without, in their study.
Commercial rubber plantations have been a major driver of forest loss in tropical Asia (Kumar, 2005; Tata, 2010; Warren-Thomas et al., 2015, 2020; Chakraborty et al., 2018). Studies from Southeast Asia have reported mostly negative effects of commercial rubber plantations (Tata, 2010; Phommexay et al., 2011; Warren-Thomas et al., 2015, 2020) and oil palm (Struebig et al., 2008, 2011; Mullin et al., 2020) on insectivorous bats. In Thailand, forest-dependent understorey-foraging bats (NSFD guild) were more diverse and abundant in forest fragments than rubber plantations (Furey et al., 2010; Phommexay et al., 2011; Warren-Thomas et al., 2015). Our findings for NSFD bats were very similar. But our hypothesis that activity of OSA and ESA bats would not differ between natural forests and plantations was not supported. This result contrasted with that of Heer et al. (2015), who reported indifferent or positive effects of rubber plantations on similar bat guilds in Brazil. This difference could be because of the much higher diversity of Neotropical bats, and also due to the structurally complex nature of secondary vegetation in “rubber-forest plantations” and mixed rubber-cacao plantations, in their study. In contrast, most rubber plantations in our study area were monocultures.
In the observed general tendency of preference for natural forests and avoidance of rubber plantations, the landscape context mattered. Different bat guilds showed variable activity across habitat types in relation to the percentage of forest cover in surrounding buffers. Responses of insectivorous bats to individual habitat types depend on whether the surrounding landscape context is bat-friendly or not (Harvey and Villalobos, 2007; Williams-Guillén and Perfecto, 2011). Faria et al. (2007) found that shade coffee and cocoa plantations in the neotropics, when adjacent to forests, supported greater bat foraging activity. Our results for NSFD and ESA bats were similar to those of Weier et al. (2021), who reported low activity of narrow-space foraging bats in macadamia monocultures of South Africa and more use of macadamia plantations with natural edges by edge-space foraging bats. In the same landscape, open-space foragers used more human-modified habitats. For rubber-dominated regions in Southeast Asia, preserving forest cover in the surrounding landscapes had positive effects on biodiversity (He and Martin, 2015; Sreekar et al., 2016).
Positive Effects of Understorey Vegetation on All Bat Guilds
Bat activity across the three guilds was generally higher when understorey vegetation was present in forests or plantations. This result is interesting and also surprising, because it highlights the importance of understorey vegetation for all bat guilds, irrespective of their foraging modes. Warren-Thomas et al. (2020) reported a strong positive effect of maintaining understorey in rubber plantations on multiple biodiversity elements. Such an overwhelming influence of understorey vegetation on bat activity across guilds could be due to higher insect abundance in undergrowth. Although, we could not sample insect abundance, understorey vegetation might significantly increase the abundance of insect prey for bats (Holloway et al., 1992; Phommexay et al., 2011). In Panama, in the dry-season, understorey vegetation had higher insect abundance than in clearings (Richards and Windsor, 2007). Rodríguez-San Pedro and Simonetti (2015) found that dense understorey in pine plantations supported higher bat activity along track edges. Understorey vegetation could lead to an aggregative effect on bats that may respond to concentration of insect prey in these habitats (Müller et al., 2012). Habitat types with understorey vegetation would thus support higher bat activity in turn. This was confirmed by our analysis of effects of rubber plantation management. Rainho et al. (2010) found that in spite of higher insect abundance in ground vegetation, dense vegetation cover might not allow bats to access insect prey in the undergrowth. Therefore, perhaps, rubber plantations that maintained moderate management levels and understorey shrub growth had higher bat activity than intensively managed plantations in our study.
Effects of Local Habitat Structure on Activity of Different Bat Guilds
OSA bats (families Emballonuridae and Molossidae) had higher activity in open habitat patches concurring with our hypothesis, but at the local scale. At the landscape scale, OSA activity was higher in buffers of high forest cover. This was not expected, although our result resonated with Frey-Ehrenbold et al. (2013), who found that even high-flying “long range echolocators” (similar to OSA) might be associated with vegetation structure in the landscape. We found that ESA bats (families Vespertilionidae and Miniopteridae) had the highest activity along stream or road edges at forest-plantation boundaries, vindicating their description of being edge-space foragers (Denzinger and Schnitzler, 2013). ESA bats prefer streams at plantation edges due to the high abundance of riparian insect prey (Fukui et al., 2006; Ober and Hayes, 2008). They also use thinned plantations and clearings along plantation edges (Humes et al., 1999; Hein et al., 2009; Obrist et al., 2011; Lentini et al., 2012). Ambivalent responses of the ESA bat guild to local habitat structure could also be due to the high species diversity within the Vespertilionidae. Future guild-based studies can benefit from more detailed analysis of species-level responses particularly for ESA bats (Siemers and Schnitzler, 2004). NSFD bats (family Rhinolophidae) totally avoided open habitats but used deciduous teak plantation belts along forest edges. NSFD bats are generally known to occur along wooded edges of forests (Davy et al., 2007; Goiti et al., 2008). These results together suggest variable effects of vegetation structure and habitat edges on different bat guilds (Gehrt and Chelsvig, 2003; Ducci et al., 2015), despite the consistent positive effects of forest cover at the landscape scale, and of understorey at the local scale (Figure 5).
Influence of Plantation Management Practices on Bat Activity in Fragmented Landscapes
Obrist et al. (2011) found insectivorous bat activity to be higher in managed orchards than unmanaged ones, indicating a favorable effect of plantation management. Wickramasinghe et al. (2003) reported higher bat activity in organically managed than conventional farms. Lentini et al. (2012) and Williams-Guillén and Perfecto (2011) found that lower levels of plantation management benefited bat activity, which our results concur with. Frequent removal of understorey might have contributed to low bat activity due to poor availability of insect prey in intensively managed rubber plantations (as reported by He and Martin, 2015). Intensive plantation management might have also accelerated forest fragmentation by expansion of roads and village settlements, causing disturbances to movement corridors of bats (Berthinussen and Altringham, 2012). Management practices prevalent in rubber plantations have been intensifying in our study area (authors' observations). This may negatively affect potential ecosystem services from insectivorous bats for rubber plantations. It is also likely that bats avoiding rubber plantations might forage more in adjacent forests and agro-ecosystems, resulting in positive effects in terms of insect pest control services to the latter habitats. Conversely, the spread of intensively managed rubber monocultures could also depress existing bat activity and potential for insect pest control in other habitats. Anecdotal reports from local villagers in our study area indicated higher mosquito abundances in rubber plantations. Bat activity might also be important to control mosquito abundances (Gonsalves et al., 2013) and have potential implications for associated disease risks to people. Our results thus indicate that maintaining low to moderate levels of management might enable reductions in pest activity, while allowing bats to use rubber plantations with overall low environmental impacts. Such management can maximize commercial benefits from rubber while securing insectivorous bat-generated ecosystem services (Häuser, 2016).
Implications for Bat Conservation in Forest-Plantation Landscapes
Our paper contributes evidence that forest habitats and understorey vegetation may enhance bat activity in agroforestry plantation landscapes dominated by rubber monocultures. Despite the fact that the bulk of our data was collected in 2011–12, our study remains relevant given the current state of knowledge on impacts of land-use change from forests to rubber plantations on insectivorous bats. At present there are no studies from India on this issue, even though rubber plantations are expanding in many regions (Chakraborty et al., 2018). Our study may be one among only a few to identify criteria that may allow bat guilds to use rubber plantation-dominated regions, in relation to percent of remnant forest cover in the landscape, and maintenance of understory vegetation in rubber plantations (Figure 5). By linking management practices to bat guild activity, our study provides an understanding of the conditions that may help sustain bat foraging and habitat use even in commercial rubber plantations. In Brazilian forest-rubber mosaics, intensively managed rubber-cacao plantations surprisingly had high bat diversity and abundance (Heer et al., 2015), possibly due to the mixed nature of these plantations. In our study area, plantations maintaining old rubber varieties along with new high-yielding varieties showed higher bat activity than rubber plantations with only new varieties (authors' field observations). To mitigate negative impacts of rubber plantations on bats, we suggest modification of management practices at multiple levels, including (1) protection of forest habitat buffers around rubber plantations, (2) maintenance of understorey vegetation in large, intensively managed rubber plantations, and (3) inter-cropping and organic management practices in plantations. Insectivorous bats could also benefit if their ecosystem services toward controlling insect pests are valued in eco-certification for rubber plantations (Gouyon, 2003; Tata, 2010; Warren-Thomas et al., 2020). Hence, quantifying insect pest control (especially of beetle and moth pests) by insectivorous bat guilds in rubber plantations will be important in future studies. In conclusion, our findings emphasize that ecologically sensitive management of commercial agroforestry plantations can help conserve insectivorous bats and secure bat-generated ecosystem services to tropical agroforestry landscapes (Fitzherbert et al., 2008; Häuser, 2016).
Data Availability Statement
The raw data supporting the conclusions of this article will be made available by the authors, without undue reservation.
Ethics Statement
Ethical review and approval was not required for the animal study because the study was based on non-invasive acoustic sampling of insectivorous bats. To do this study, we had obtained research permits from the state Forest Department where we worked.
Author Contributions
KD: conceptualization, methodology, formal analysis, investigation, data curation, writing – original draft, writing – review and editing, visualization, project administration, and funding acquisition. NK: methodology, formal analysis, writing – review and editing, and visualization. JK: writing – review and editing, visualization, supervision, and resources. MS: methodology, writing – review and editing, visualization, supervision, and resources. All authors contributed to the article and approved the submitted version.
Conflict of Interest
The authors declare that the research was conducted in the absence of any commercial or financial relationships that could be construed as a potential conflict of interest.
Publisher's Note
All claims expressed in this article are solely those of the authors and do not necessarily represent those of their affiliated organizations, or those of the publisher, the editors and the reviewers. Any product that may be evaluated in this article, or claim that may be made by its manufacturer, is not guaranteed or endorsed by the publisher.
Acknowledgments
We thank the Kerala Forest Department for granting research permissions and support to work in the Shenduruney Wildlife Sanctuary and surrounding areas. Thanks are due to Divisional Forest Officers K. I. Pradeep Kumar and R. Lekshmi (Kerala Forest Dept.) for their help and support, to Shinu Jacob for field assistance, and to field-based key informants. KD's fieldwork was supported by the Rufford Small Grants Foundation (grant number 9686-1) (2011–12), the WWF-India Small Grants Program (2014), and the Ravi Sankaran Inlaks Small Grant (2017–18). Neil Furey and Ajith Kumar provided valuable support through the study. Aniruddha Marathe and Aditya Joshi helped with statistical analyses and GIS. This paper is in partial fulfillment of the PhD of KD at the Manipal Academy of Higher Education.
Supplementary Material
The Supplementary Material for this article can be found online at: https://www.frontiersin.org/articles/10.3389/fcosc.2021.751694/full#supplementary-material
Abbreviations
OSA, “Open-Space Aerial” foraging bats; ESA, “Edge-Space Aerial” foraging bats; NSFD, “Narrow-Space Flutter Detecting” foraging bats; FmaxE, Frequency with maximum Energy; CF, Constant Frequency; FM, Frequency Modulation; QCF, Quasi-Constant Frequency; GIS, Geographical Information System; OLI, Operational Land Imager; TIRS, Thermal Infrared Sensor; AIC, Akaike Information Criterion; ZIP GLMs, Zero-inflated Poisson Generalized Linear Models.
Footnotes
References
Anand, M. O., Krishnaswamy, J., Kumar, A., and Bali, A. (2010). Sustaining biodiversity conservation in human-modified landscapes in the Western Ghats: remnant forests matter. Biol. Conserv. 143, 2363–2374. doi: 10.1016/j.biocon.2010.01.013
Bates, P. J. J., and Harrison, D. L. (1997). Bats of the Indian Subcontinent. Kent: Harrison Zoological Museum.
Berthinussen, A., and Altringham, J. (2012). The effect of a major road on bat activity and diversity. J. Appl. Ecol. 49, 82–89. doi: 10.1111/j.1365-2664.2011.02068.x
Biscardi, S., Orprecio, J., Fenton, M. B., Tsoar, A., and Ratcliffe, J. M. (2004). Data, sample sizes and statistics affect the recognition of species of bats by their echolocation calls. Acta Chiropterol. 6, 347–363. doi: 10.3161/001.006.0212
Bontadina, F., Schofield, H., and Naef-Daenzer, B. (2002). Radio-tracking reveals that lesser horseshoe bats (Rhinolophus hipposideros) forage in woodland. J. Zool. Soc. London 258, 281–290. doi: 10.1017/S0952836902001401
Brigham, R. M., Kalko, E. K. V., Jones, G., Parsons, S., and Limpens, H. J. G. A. (2004). Bat Echolocation Research: Tools, Techniques and Analysis. Austin, TX: Bat Conservation International, 11 + 95.
Burnham, K. P., and Anderson, D. R. (2002). Model Selection and Multimodel Inference: A Practical Information-Theoretic Approach, 2nd Edn. New York, NY: Springer-Verlag.
Cadotte, M. W., Carscadden, K., and Mirotchnick, N. (2011). Beyond species: functional diversity and the maintenance of ecological processes and services. J. Appl. Ecol. 48, 1079–1087. doi: 10.1111/j.1365-2664.2011.02048.x
Canale, G. R., Peres, C. A., Guidorizzi, C. E., Gatto, C. A. F., and Kierulff, C. M. (2012). Pervasive defaunation of forest remnants in a tropical biodiversity hotspot. PLoS One 7:e41671. doi: 10.1371/journal.pone.0041671
Cel'uch, M., and Zahn, A. (2008). Foraging habitats preferences of bats: new question in interpretation of bat detector data. Vespertilio 12, 3–9.
Chakraborty, K., Sudhakar, S., Sarma, K. K., Raju, P. L. N., and Das, A. K. (2018). Recognizing the rapid expansion of rubber plantations - a threat to native forest in parts of northeast India. Curr. Sci. 114, 207–213. doi: 10.18520/cs/v114/i01/207-213
Dauber, J., Hirsch, M., Simmering, D., Waldhardt, R., Otte, A., and Wolters, V. (2003). Landscape structure as an indicator of biodiversity: matrix effects on species richness. Agric. Ecosyst. Environ. 98, 321–329. doi: 10.1016/S0167-8809(03)00092-6
Davy, C., Russo, D., and Fenton, M. (2007). Use of native woodlands and traditional olive groves by foraging bats on a Mediterranean island: consequences for conservation. J. Zool. 273, 397–405. doi: 10.1111/j.1469-7998.2007.00343.x
Denzinger, A., and Schnitzler, H.-U. (2013). Bat guilds, a concept to classify the highly diverse foraging and echolocation behaviors of microchiropteran bats. Front. Physiol. 4:164. doi: 10.3389/fphys.2013.00164
Deshpande, K., and Kelkar, N. (2015). Acoustic identification of Otomops wroughtoni and other free-tailed bat species (Chiroptera: Molossidae) from India. Acta Chiropterol. 17, 419–428. doi: 10.3161/15081109ACC2015.17.2.018
Ducci, L., Agnelli, P., Di Febbraro, M., Frate, L., Russo, D., Loy, A., et al. (2015). Different bat guilds perceive their habitat in different ways: a multiscale landscape approach for variable selection in species distribution modelling. Landscape Ecol. 30, 2147–2159. doi: 10.1007/s10980-015-0237-x
Duchamp, J. E., and Swihart, R. K. (2008). Shifts in bat community structure related to evolved traits and features of human-altered landscapes. Landscape Ecol. 23, 849–860. doi: 10.1007/s10980-008-9241-8
Erickson, J. L., and West, S. D. (2003). Associations of bats with local structures and landscape features of forested stands in western Oregon and Washington. Biol. Conserv. 109, 95–102. doi: 10.1016/S0006-3207(02)00141-6
Estrada-Villegas, S., Meyer, C. F. J., and Kalko, E. K. V. (2010). Effects of tropical forest fragmentation on aerial insectivorous bats in a land-bridge island system. Biol. Conserv. 143, 597–608. doi: 10.1016/j.biocon.2009.11.009
Ethier, K., and Fahrig, L. (2011). Positive effects of forest fragmentation, independent of forest amount, on bat abundance in eastern Ontario, Canada. Landscape Ecol. 26, 865–876. doi: 10.1007/s10980-011-9614-2
FAO and UNEP (2020). The State of the World's Forests 2020 Forests, Biodiversity and People. Rome: FAO.
Faria, D., Paciencia, M. L. B., Dixo, M., Laps, R. R., and Baumgarten, J. (2007). Ferns, frogs, lizards, birds and bats in forest fragments and shade cacao plantations in two contrasting landscapes in the Atlantic forest, Brazil. Biodivers. Conserv. 16, 2335–2357. doi: 10.1007/s10531-007-9189-z
Fitzherbert, E. B., Struebig, M. J., Morel, A., Danielsen, F., Brühl, C. A., Donald, P. F., et al. (2008). How will oil palm expansion affect biodiversity? Trends Ecol. Evol. 23, 538–545. doi: 10.1016/j.tree.2008.06.012
Fortin, M. J., and Dale, M. R. T. (2005). Spatial Analysis: A Guide for Ecologists. Cambridge: Cambridge University Press.
Fraser, E. E., Silvis, A., Brigham, R. M., and Czenze, Z. J. (2020). Bat Echolocation Research: A Handbook for Planning and Conducting Acoustic Studies, 2nd Edn. Austin, TX: Bat Conservation International.
Frey-Ehrenbold, A., Bontadina, F., Arlettaz, R., and Obrist, M. K. (2013). Landscape connectivity, habitat structure and activity of bat guilds in farmland-dominated matrices. J. Appl. Ecol. 50, 252–261. doi: 10.1111/1365-2664.12034
Fukui, D., Murakami, M., Nakano, S., and Aoi, T. (2006). Effect of emergent aquatic insects on bat foraging in a riparian forest. J. Anim. Ecol. 75, 1252–1258. doi: 10.1111/j.1365-2656.2006.01146.x
Furey, N. M., Mackie, I. J., and Racey, P. A. (2010). Bat diversity in Vietnamese limestone karst areas and the implications of forest degradation. Biodivers. Conserv. 19, 1821–1838. doi: 10.1007/s10531-010-9806-0
Gardner, T. A., Barlow, J., Chazdon, R., Ewers, R. M., Harvey, C. A., Peres, C. A., et al. (2009). Prospects for tropical forest biodiversity in a human-modified world. Ecol. Lett. 12, 561–582. doi: 10.1111/j.1461-0248.2009.01294.x
Gehrt, S. D., and Chelsvig, J. E. (2003). Bat activity in an urban landscape: patterns at the landscape and microhabitat scale. Ecol. Appl. 13, 939–950. doi: 10.1890/02-5188
Gili, F., Newson, S. E., Gillings, S., Chamberlain, D. E., and Border, J. A. (2020). Bats in urbanising landscapes: habitat selection and recommendations for a sustainable future. Biol. Conserv. 241:108343. doi: 10.1016/j.biocon.2019.108343
Goiti, U., Garin, I., Almenar, D., Salsamendi, E., and Aihartza, J. (2008). Foraging by Mediterranean horseshoe bats (Rhinolophus euryale) in relation to prey distribution and edge habitat. J. Mammal. 89, 493–502. doi: 10.1644/07-MAMM-A-054R2.1
Gonsalves, L., Law, B., Webb, C., and Monamy, V. (2013). Foraging ranges of insectivorous bats shift relative to changes to changes in mosquito abundance. PLoS One 8:e64081. doi: 10.1371/journal.pone.0064081
Gorresen, P. M., and Willig, M. R. (2004). Landscape responses of bats to habitat fragmentation in Atlantic Forest of Paraguay. J. Mammal. 85, 688–697. doi: 10.1644/BWG-125
Gouyon, A. (2003). Eco-certification as an Incentive to Conserve Biodiversity in Rubber Smallholder Agroforestry Systems: A Preliminary Study. Indonesia: World Agroforestry Centre (ICRAF), 61.
Harvey, C. A., and Villalobos, J. A. G. (2007). Agroforestry systems conserve species-rich but modified assemblages of tropical birds and bats. Biodivers. Conserv. 16, 2257–2292.
Häuser, I. (2016). Impact of Rubber Tree Dominated Land-Use on Biodiversity and Ecosystem Services in the Greater Mekong Subregion. PhD thesis, University of Hohenheim, 168+vi.
Hayes, J. P. (1997). Temporal variation in activity of bats and the design of echolocation-monitoring studies. J. Mammal. 78, 514–524. doi: 10.2307/1382902
He, H., Tang, W., Wang, W., and Crits-Christoph, P. (2014). Structural zeroes and zero-inflated models. Shanghai Arch. Psychiatry 26, 236–242. doi: 10.3969/j.issn.1002-0829.2014.04.008
He, P., and Martin, K. (2015). Effects of rubber cultivation on biodiversity in the Mekong Region. CAB Rev. 10:1–7. doi: 10.1079/PAVSNNR201510044
Heer, K., Helbig-Bonitz, M., Fernandes, R. G., Mello, M. A. R., and Kalko, E. K. V. (2015). Effects of land use on bat diversity in a complex plantation–forest landscape in northeastern Brazil. J. Mammal. 96, 720–731. doi: 10.1093/jmammal/gyv068
Hein, C. D., Castleberry, S. B., and Miller, K. V. (2009). Site-occupancy of bats in relation to forested corridors. Forest Ecol. Manag. 257, 1200–1207. doi: 10.1016/j.foreco.2008.09.054
Henle, K., Davies, K. F., Kleyer, M., Margules, C., and Settele, J. (2004). Predictors of species sensitivity to fragmentation. Biodivers. Conserv. 13, 207–251. doi: 10.1023/B:BIOC.0000004319.91643.9e
Hogue, A. S., and McGowan, A. T. (2018). Comparison of Driving Transect Methods for Acoustic Monitoring of Bats, Bats. ed H. Mikkola (IntechOpen). doi: 10.5772/intechopen.75834. Available online at: https://www.intechopen.com/chapters/60155 (accessed October 15, 2020).
Holloway, J. D., Kirk-Spriggs, A. H., and Khen, C. V. (1992). The response of some rain-forest insect groups to logging and conversion to plantation. Philos. Trans. R. Soc. London B 335, 425–436. doi: 10.1098/rstb.1992.0034
Humes, M. L., Hayes, J. P., and Collopy, M. W. (1999). Bat activity in thinned, unthinned, and old-growth forests in western Oregon. J. Wildl. Manag. 63, 553–561. doi: 10.2307/3802642
Jha, C. S., Dutt, C. B. S., and Bawa, K. S. (2000). Deforestation and land use changes in Western Ghats, India. Curr. Sci. 79, 231–238.
Jones, G., Jacobs, D. S., Kunz, T. H., Willig, M. R., and Racey, P. A. (2009). Carpe noctem: the importance of bats as bioindicators. Endangered Species Res. 8, 93–115. doi: 10.3354/esr00182
Kapfer, G., Rigot, T., Holsbeek, L., and Aron, S. (2008). Roost and hunting site fidelity of female and juvenile Daubenton's bat Myotis daubentonii (Kuhl, 1817) (Chiroptera: Vespertilionidae). Mammal. Biol. 73, 267–275. doi: 10.1016/j.mambio.2007.01.001
Kerbiriou, C., Bas, Y., Le Viol, I., Lorrillière, R., Mougnot, J., and Julien, J. F. (2019). Bat pass duration measurement: an indirect measure of distance of detection. Diversity 11:47. doi: 10.3390/d11030047
Klingbeil, B. T., and Willig, M. R. (2009). Guild-specific responses of bats to landscape composition and configuration in fragmented Amazonian rainforest. J. Appl. Ecol. 46, 203–213. doi: 10.1111/j.1365-2664.2008.01594.x
Korad, V., Yardi, K., and Raut, R. (2007). Diversity and distribution of bats in the Western Ghats of India. Zoos Print J. 22, 2752–2758. doi: 10.11609/JoTT.ZPJ.1563.2752-8
Kumar, A., Chellam, R., Choudhury, B. C., Mudappa, D., Vasudevan, K., Ishwar, N. M., et al. (2002). Impact of Rainforest Fragmentation on Small Mammals and Herpetofauna in the Western Ghats, South India. Final Report submitted to the US Fish and Wildlife Service, USA, 159.
Kumar, B. M. (2005). Land use in Kerala: changing scenarios and shifting paradigms. J. Trop. Agric. 42, 1–12.
Kumar, M. A., Mudappa, D., and Raman, T. R. S. (2010). Asian elephant Elephas maximus habitat use and ranging in fragmented rainforest and plantations in the Anamalai hills, India. Trop. Conserv. Sci. 3, 143–158. doi: 10.1177/194008291000300203
Kusch, J., and Schotte, F. (2007). Effects of fine-scale foraging habitat selection on bat community structure and diversity in a temperate low mountain range forest. Folia Zool. 56, 263–276.
Landsat Operational Land Imager and Thermal Infrared Sensor (OLI TIRS). (2014). Available online at: www.earthexplorer.usgs.gov (accessed April, 2014).
Lentini, P. E., Gibbons, P., Fischer, J., Law, B., Hanspach, J., and Martin, T. G. (2012). Bats in a farming landscape benefit from linear remnants and unimproved pastures. PLoS One 7:e48201. doi: 10.1371/journal.pone.0048201
MacSwiney, G., M. C., Clarke, F. M., and Racey, P. A. (2008). What you see is not what you get: the role of ultrasonic detectors in increasing inventory completeness in Neotropical bat assemblages. J. Appl. Ecol. 45, 1364–1371. doi: 10.1111/j.1365-2664.2008.01531.x
Marques, J. T., Rainho, A., Carapuso, M., Oliveira, P., and Palmeirim, J. M. (2004). Foraging behaviour and habitat use by the European free-tailed bat Tadarida teniotis. Acta Chiropterol. 6, 99–110. doi: 10.3161/001.006.0108
Meyer, J., Fründ, J., Lizano, W. P., and Kalko, E. K. V. (2008). Ecological correlates of vulnerability to fragmentation in Neotropical bats. J. Appl. Ecol. 45, 381–391. doi: 10.1111/j.1365-2664.2007.01389.x
Molur, S., and Singh, M. (2009). Diversity and abundance of bats in different habitats of Coorg, Western Ghats, India. Biosystematica 3, 65–90.
Moreno, C. E., and Halffter, G. (2000). Assessing the completeness of bat biodiversity inventories using species accumulation curves. J. Appl. Ecol. 37, 149–158. doi: 10.1046/j.1365-2664.2000.00483.x
Müller, J., Mehr, M., Bässler, C., Fenton, M. B., Hothorn, T., Pretzsch, H., et al. (2012). Aggregative response in bats: prey abundance versus habitat. Oecologia 169, 673–684. doi: 10.1007/s00442-011-2247-y
Mullin, K. E., Yoh, N., Mitchell, S. L., Basrur, S., Seaman, D. J., Bernard, H., et al. (2020). Riparian reserves promote insectivorous bat activity in oil palm dominated landscapes. Front. For. Global Change 3:73. doi: 10.3389/ffgc.2020.00073
Nair, S. C. (1991). The Southern Western Ghats: A Biodiversity Conservation Plan. New Delhi: INTACH, 92.
Neuweiler, G., Singh, S., and Sripathi, K. (1984). Audiograms of a south Indian bat community. J. Comp. Physiol. 154, 133–142. doi: 10.1007/BF00605398
Ober, H. K., and Hayes, J. P. (2008). Influence of vegetation on bat use of riparian areas at multiple spatial scales. J. Wildl. Manag. 72, 396–404. doi: 10.2193/2007-193
Obrist, M. K., Rathey, E., Bontadina, F., Martinoli, A., Conedera, M., Christe, P., et al. (2011). Response of bat species to sylvo-pastoral abandonment. For. Ecol. Manag. 261, 789–798. doi: 10.1016/j.foreco.2010.12.010
Phommexay, P., Satasook, C., Bates, P., Pearch, M., and Bumrungsri, S. (2011). The impact of rubber plantations on the diversity and activity of understorey insectivorous bats in southern Thailand. Biodivers. Conserv. 20, 1441–1456. doi: 10.1007/s10531-011-0036-x
Prakash, H., Saha, K., Sahu, S., and Balakrishnan, R. (2021). Ecological drivers of selection for remnant forest habitats by an insectivorous bat in a tropical, human-modified landscape. For. Ecol. Manag. 496:119451. doi: 10.1016/j.foreco.2021.119451
QGIS Development Team (2018). QGIS Geographic Information System. Open Source Geospatial Foundation Project. Available online at: http://qgis.osgeo.org (accessed April 20, 2020).
R Core Team (2018). R: A Language and Environment for Statistical Computing [Computer Software]. Vienna: R Foundation for Statistical Computing. Available online at http://www.R-project.org (accessed June 20, 2018).
Raghuram, H., Jain, M., and Balakrishnan, R. (2014). Species and acoustic diversity of bats in a palaeotropical wet evergreen forest in southern India. Curr. Sci. 107, 631–642.
Rainho, A., Augusto, A. M., and Palmeirim, J. M. (2010). Influence of vegetation clutter on the capacity of ground foraging bats to capture prey. J. Appl. Ecol. 47, 850–858. doi: 10.1111/j.1365-2664.2010.01820.x
Raman, T. R. S. (2006). Effects of habitat structure and adjacent habitats on birds in tropical rainforest fragments and shaded plantations in the Western Ghats, India. Biodivers. Conserv. 15, 1577–1607. doi: 10.1007/s10531-005-2352-5
Richards, L. A., and Windsor, D. M. (2007). Seasonal variation of arthropod abundance in gaps and the understorey of a lowland moist forest in Panama. J. Trop. Ecol. 23, 169–176. doi: 10.1017/S0266467406003907
Rodríguez-San Pedro, A., and Simonetti, J. A. (2015). Does understory clutter reduce bat activity in forestry pine plantations? Eur. J. Wildl. Res. 61, 177–179. doi: 10.1007/s10344-014-0871-7
Saunders, D. A., Hobbs, R. J., and Margules, C. R. (1991). Biological consequences of ecosystem fragmentation: a review. Conserv. Biol. 5, 18–32. doi: 10.1111/j.1523-1739.1991.tb00384.x
Schnitzler, H.-U., and Kalko, E. K. V. (2001). Echolocation by insect-eating bats. BioScience 51, 557–569. doi: 10.1641/0006-3568(2001)051[0557:EBIEB]2.0.CO;2
Segurado, P., Araújo, M. B., and Kunin, W. E. (2006). Consequences of spatial autocorrelation for niche-based models. J. Appl. Ecol. 43, 433–444. doi: 10.1111/j.1365-2664.2006.01162.x
Siemers, B. M., and Schnitzler, H.-U. (2004). Echolocation signals reflect niche differentiation in five sympatric congeneric bat species. Nature 429, 657–661. doi: 10.1038/nature02547
Skalak, S. L., Sherwin, R. E., and Brigham, R. M. (2012). Sampling period, size and duration influence measures of bat species richness from acoustic surveys. Methods Ecol. Evol. 3, 490–502. doi: 10.1111/j.2041-210X.2011.00177.x
Sprong, L., Keith, M., and Seamark, E. C. J. (2012). Assessing the effect of waterproofing on three different bat detectors. Afr. Bat Conserv. News 28, 4–14.
Sreekar, R., Huang, G., Yasuda, M., Quan, R.-C., Goodale, E., Corlett, R. T., et al. (2016). Effects of forests, roads and mistletoe on bird diversity in monoculture rubber plantations. Sci. Rep. 6:21822. doi: 10.1038/srep21822
Struebig, M. J., Kingston, T., Petit, E. J., Le Comber, S. C., Zubaid, A., Mohd.-Adnan, A., et al. (2011). Parallel declines in species and genetic diversity in tropical forest fragments. Ecol. Lett. 14, 582–590. doi: 10.1111/j.1461-0248.2011.01623.x
Struebig, M. J., Kingston, T., Zubaid, A., Mohd.-Adnan, A., and Rossiter, S. J. (2008). Conservation value of forest fragments to palaeotropical bats. Biol. Conserv. 141, 2112–2126. doi: 10.1016/j.biocon.2008.06.009
Tata, H. L. (2010). Recognising Biodiversity in Rubber Plantations. Bogor: World Agroforestry Centre (ICRAF) Southeast Asia Regional Program, 111.
Turner, I. M. (1996). Species loss in fragments of tropical rain forest: a review of the evidence. J. Appl. Ecol. 33, 200–209. doi: 10.2307/2404743
Vaughan, N., Jones, G., and Harris, S. (1997). Habitat use by bats (Chiroptera) assessed by means of a broad-band acoustic method. J. Appl. Ecol. 34, 716–730. doi: 10.2307/2404918
Warren-Thomas, E., Dolman, P. M., and Edwards, D. P. (2015). Increasing demand for natural rubber necessitates a robust sustainability initiative to mitigate impacts on tropical biodiversity. Conserv. Lett. 8, 230–241. doi: 10.1111/conl.12170
Warren-Thomas, E., Nelson, L., Juthong, W., Bumrungsri, S., Brattström, O., Stroesser, L., et al. (2020). Rubber agroforestry in Thailand provides some biodiversity benefits without reducing yields. J. Appl. Ecol. 57, 17–30. doi: 10.1111/1365-2664.13530
Watling, J. I., Nowakowski, A. J., Donnelly, M. A., and Orrock, J. L. (2011). Meta-analysis reveals the importance of matrix composition for animals in fragmented habitat. Glob. Ecol. Biogeogr. 20, 209–217. doi: 10.1111/j.1466-8238.2010.00586.x
Weier, S. M., Linden, V. M., Hammer, A., Grass, I., Tscharntke, T., and Taylor, P. J. (2021). Bat guilds respond differently to habitat loss and fragmentation at different scales in macadamia orchards in South Africa. Agric. Ecosyst. Environ. 320:107588. doi: 10.1016/j.agee.2021.107588
Wickramasinghe, L. P., Harris, S., Jones, G., and Vaughan, N. (2003). Bat activity and species richness on organic and conventional farms: impact of agricultural intensification. J. Appl. Ecol. 40, 984–993. doi: 10.1111/j.1365-2664.2003.00856.x
Williams, R. (2019). Scalar Measures of Fit: Pseudo-R2 and Information Measures (AIC and BIC). University of Notre Dame. Available online at: https://www3.nd.edu/$\sim$rwilliam/stats3 (accessed December 02, 2019).
Williams-Guillén, K., and Perfecto, I. (2011). Ensemble composition and activity levels of insectivorous bats in response to management intensification in coffee agroforestry systems. PLoS One 6:e16502. doi: 10.1371/journal.pone.0016502
Wordley, C., Foui, E., Mudappa, D., Sankaran, M., and Altringham, J. (2014). Acoustic identification of bats in the southern Western Ghats, India. Acta Chiropterol. 16, 213–222. doi: 10.3161/150811014X683408
Wordley, C. F. R., Sankaran, M., Mudappa, D., and Altringham, J. D. (2015). Landscape scale habitat suitability modelling of bats in the Western Ghats of India: bats like something in their tea. Biol. Conserv. 191, 529–536. doi: 10.1016/j.biocon.2015.08.005
Keywords: insectivorous bat guilds, bat activity, forest cover, rubber plantations, management practices, understorey vegetation, Western Ghats, ecosystem services
Citation: Deshpande K, Kelkar N, Krishnaswamy J and Sankaran M (2021) Stretching the Habitat Envelope: Insectivorous Bat Guilds Can Use Rubber Plantations, but Need Understorey Vegetation and Forest Buffers. Front. Conserv. Sci. 2:751694. doi: 10.3389/fcosc.2021.751694
Received: 01 August 2021; Accepted: 17 November 2021;
Published: 09 December 2021.
Edited by:
Paulo Estefano Bobrowiec, National Institute of Amazonian Research (INPA), BrazilReviewed by:
Wenke Bai, China West Normal University, ChinaDunwu Qi, Chengdu Research Base of Giant Panda Breeding, China
Copyright © 2021 Deshpande, Kelkar, Krishnaswamy and Sankaran. This is an open-access article distributed under the terms of the Creative Commons Attribution License (CC BY). The use, distribution or reproduction in other forums is permitted, provided the original author(s) and the copyright owner(s) are credited and that the original publication in this journal is cited, in accordance with accepted academic practice. No use, distribution or reproduction is permitted which does not comply with these terms.
*Correspondence: Kadambari Deshpande, a2FkYW1iYXJpLmRlc2hwYW5kZUBhdHJlZS5vcmc=; a3ZkLm5vdmVsQGdtYWlsLmNvbQ==