- 1James Watt School of Engineering, University of Glasgow, Glasgow, United Kingdom
- 2School of Engineering and Physical Science, Heriot-Watt University, Edinburgh, United Kingdom
By 2050, experts estimate that the agricultural produce must increase by 60%–70% to meet the needs of the ever increasing population of the world. To this aim, the concept of precision agriculture or smart farming has recently been coined. The idea of precision agriculture is well represented as a smart management system, having the ability to monitor, observe, sense, measure and control the health and water contents in plants at nano-scale and crops at macro-scale. The goal is to maximise the production while preserving the vital resources. The combination of terahertz (THz) based sensing technology to estimate plant health at a cellular level, and wireless sensor networks deployed within crops to monitor different variables while making intelligent decisions is far reaching. The integration and operation of such a macro-nano-sensor system requires a sustainable communication infrastructure that considers the demands of remote and agile agricultural environments. In this paper, an integrated sensing and communication system for plant health monitoring that utilises THz signals, is presented as a 6G use case. The joint architecture is outlined and various challenges including energy harvesting, practical implementation among others, followed by recommendations for future research are presented.
1 Introduction
According to a United Nations (UN) report, by 2050 there will be an additional two billion people living on our planet Earth (Nations, 2015). It is estimated that the food production must increase by 60%–70% to meet the nourishment needs of the population living at that time (Schönfeld et al., 2018). The current agriculture methods are incapable of achieving this production target. To achieve this ambition, the concept of precision agriculture has recently been introduced that works to precisely identify the health status of a particular part in a farm, i.e., a plant or even a leaf. Precision agriculture can be regarded as a smart agriculture management system that keeps a track of the plants’ health and needs, providing concrete information of tailoring soils and crops by deploying various sensors into the agricultural field. Furthermore, the concept of precision agriculture aims to maximise production whilst minimising the use of fertilisers, pesticides, and herbicides, which can be achieved by monitoring the plants and the soil for water and moisture. To this aim, different types of sensors are proposed in the literature for measuring various bio markers in the agricultural sector. For instance, temperature sensors, humidity sensors, soil moisture sensors, pH sensors, light sensors, colour sensors, and other bio sensors are already being used in the field (Mukhopadhyay, 2014).
However, these macro sensor systems alone are unable to provide long-lasting and reliable solutions for sustained agriculture productivity. The need is to have a system that can monitor the nutrition contents of a plant at nano-scale for an early detection of agriculture diseases or malnutrition. In this regard, Terahertz (THz) sensing is proposed in the literature (Zahid et al., 2020). THz technology has distinctive features to explore the molecular changes of, for example, water contents in leaves due to its high penetration features (Song et al., 2018).
Studies have emerged that put the 5th generation of mobile networks (5G) as a key driver of smart agriculture (Khelifi et al., 2021). The main benefits of 5G can be summarised into high data rates, low latency, and higher connection density which makes it ideal for various smart agriculture applications such as autonomous tractors, real-time crop status, fertilization using drones, and others (Said Mohamed et al., 2021; Tang et al., 2021). On the other hand, the sixth generation (6G) of mobile networks are envisioned to support even higher data rates and ultra-massive connected things, compared to 5G, alongside the use of higher frequencies (Abdullahi and Al-Turjman, 2020; Barakat et al., 2021). This also includes bio and nano-internet of things (IoT) tele-operated driving, unmanned mobility, haptic communications, unmanned aerial vehicles, and many more (Alsharif et al., 2021). Where Nano-IoT is a recent concept which connects millions of nano-sensors to the Internet present in various industrial, in-body and in-plants systems. Given the size of nano-sensors, THz frequency is proposed to do various sensing activities at this scale. However, it will be ideal to use the same radio frequency for communications as well. Furthermore, THz is also proposed as an enabler of extremely high data rate applications in 6G communications (Alsharif et al., 2021). Hence it is highly desirable to exploit THz for both sensing and communications in future 6G networks, especially in the domain of precision agriculture (Zhang et al., 2019; Chaccour et al., 2021; Li et al., 2021; Wedage et al., 2021).
This paper presents a 6G-enabled precision agriculture architecture exploiting THz for joint communication and sensing applications. We envision that future precision agriculture will include nano-sensors to enable early disorders in plants. The information collected through these sensors will be reflected into a remote cloud server via communication infrastructure. As its predecessors, future 6G networks will have an ability to provide ubiquitous coverage to any location around the globe. Hence, such a network should have the capabilities to support both sensing and communications while harvesting power at the same time.
The rest of the paper is structured as follows: In Sections 2 and 3 THz-based use cases are presented. In Section 4 a vision and challenges of a combined THz-based communication and sensing framework for precision agriculture as a 6G use case is included. Finally, Section 5 concludes the paper with our recommendations for future research.
2 Sensing at Terahertz for Agricultural Applications
The research community has shown great interest in the concept of wireless sensor networks for agricultural applications with some looking at a higher level architecture (Ojha et al., 2015) and others focusing on the wireless sensing and monitoring aspect (Tagarakis et al., 2021). With focus on the sensing application, this section presents a use-case on the utilisation of THz wireless signals to monitor health-related parameters in plants. In this regard, Figure 1 presents the architecture of a THz-based sensing system that combines THz sensing and data transfer for analytics to monitor plants’ health.
2.1 Estimation of Water Content Using THz-Based Extraction of Plants Electromagnetic Properties
In recent times, there has been significant progress of THz in diverse disciplines due to its distinctive characteristics and viable opportunities (Zahid et al., 2019a,b). However, considering ongoing climate transformations, researchers are of a strong view that its utility in the field of plant science has yet to be fully studied, particularly in terms of monitoring the plants health and their biological status to sustain crops productivity. Plant leaves comprise of composite biological structure and other synthesis compounds such as protein and carbohydrates (Zahid et al., 2019b). THz technology can provide prolific recommendations and insights about sensory characteristics of plants leaves by determining their electromagnetic parameters such as permittivity and refractive index through the scattered parameters. Through this study (Zahid et al., 2019b), it is aimed to establish an understanding of die-electric properties of various plant leaves and observed their reactions in different environmental condition. The findings of this research can provide valuable information to horticulturists, and cultivators to perform proactive actions in relations to plants health monitoring and boost the agricultural production (Zahid et al., 2019b).
In this regard, significant contributions have been proposed that address the estimation of water contents in leaves. However, these techniques are limited by the resolution and cannot provide any information about physiological characteristics of leaves (Zahid et al., 2018). Later, non-invasive technique is proposed to closely observe the behaviour and water contents of plant leaves using scattering parameters of THz pulse (Zahid et al., 2019b). For this purpose, various different leaves have been investigated and observed for their electromagnetic parameters at THz frequency in non-invasive manner, providing substantial information about the internal morphological characteristics of leaves at molecular level, including the presence of water contents in leaves. The study revealed an-isotropic behaviour of plant leaves and determined significant relationship between electromagnetic parameters and leaf water contents, indicating that variations in leaves water contents are reflected in electromagnetic parameters at specific frequencies (Zahid et al., 2019a,b). This concept shows great promise in the future of precision agriculture, especially with the research community starting to actively investigate THz for communication in 6G. Hence, this use case makes a compelling case for the development of a combined THz-based sensing and communication system for agricultural production (Zahid et al., 2019a,b).
It is important to mention that along with THz waves, millimeter waves are also studied in the literature for their applications in sensing. However, millimeter waves have lower estimation resolution than THz waves. This is generally due to their lesser frequency range of millimeter waves where it is difficult to monitor and observe water molecules with high resolution. A detailed comparison between THz and millimeter waves in sensing is presented in (Brown, 2003; Taylor et al., 2015).
3 In-Vivo Nano-Network Communication With Simultaneous Energy Harvesting at Terahertz
A promising application of THz communication is to enable active communication link between nano-sensors. In this regard, a pioneering work in proposing THz-based in-vivo networks at nano-scale is presented in (Abbasi et al., 2017). Although the communication at nano-scale can be performed in different ways, such as molecular, acoustic and electromagnetic (EM) communications at THz frequencies, which is considered as one of the most suitable approaches to enable communication amongst nano-sensors. This is because biological tissues remain non-ionised at THz frequencies. Furthermore, THz frequencies are more resistant to scattering phenomenon (Alomainy et al., 2019).
Another reason of utilising THz frequencies for nano-scale communication is the property of molecular resonance at these frequencies. The underlying concepts of THz from physical to network layer are explained in (Abbasi et al., 2016), where a visionary architecture of THz based nano-communications is presented. Based on that work, Figure 2 envisions an architecture of THz enabled in-vivo networks incorporated in 6G communications (Abbasi et al., 2017).
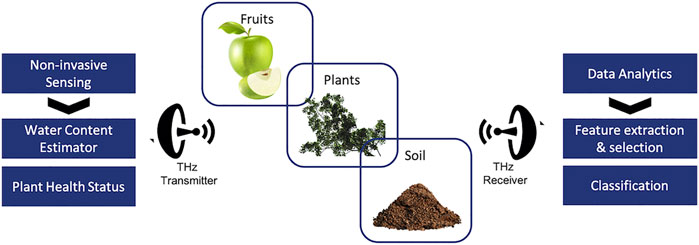
FIGURE 2. The envisioned architecture of in-vivo nano-networks connected with 6G communications (Abbasi et al., 2017).
Nano-sensors depicted in Figure 2 are small devices with highly limited communication, memory and energy resources. They would perform the simplest tasks of communication and sensing. Contrarily, nano-routers are slightly larger than nano-sensors in terms of the resources they have and the actions they can perform. They act as the controller of nano-sensors by sending simple commands such as on, off, and sleep etc. Nano-micro interface are comparatively larger devices that can control the information passed to nano-routers. They have the ability to communicate over a nano-paradigm at the southbound interface and a classical communication paradigm at the northbound interface. The gateway is the central entity that connects the entire system to the Internet. The data collected through nano-sensors is transferred to a remote data analytics platform using 6G communications network. The data analytics platform sends the instruction back to the nano-network over the same 6G network.
In-vivo nano-networks are proposed to be an integral part of future remote healthcare monitoring systems, due to their ability to reach deep inside human body (Usman et al., 2018). It is assumed that in-vivo nano-networks will play a crucial role in realising precision medicine, where each individual can be treated as per their specialised needs.
In addition to the nano-network communications, we envisage these nodes to also simultaneously transfer power wirelessly. Harvesting energies in this fashion to make the networks more sustainable is investigated by authors in (Rong et al., 2017), where they proposed a nano-network that uses the same THz band to transfer power to other nano-nodes using amplify and forward technique while also transferring useful data.
4 TERAHERTZ IN 6G AND BEYOND
Although precision agriculture and in-vivo networks are important use-cases of THz, 6G communications aims to utilise THz frequencies to enable high data rate communications required for future applications such as holographic communication, massive bio/nano-IoT, haptic communications and tele-operated driving. These use-cases will need high data rates requiring an operating bandwidth in several gigahertz. This high bandwidth is readily available in higher electromagnetic spectrum, such as in THz frequency bands. THz communications is considered as one of the most relevant enabling technologies of 6G networks, envisioned to provide extremely high data rates, low latency and ubiquitous coverage (Dang et al., 2020).
With a number of inherited benefits of THz frequencies, it is expected that THz frequencies will be deployed at various levels throughout 6G networks, ranging from backhaul, data centers, access networks and at the device level for nano-communications and simultaneous sensing.
Keeping the well investigated characteristics of THz frequency, an architecture covering deployment of THz in 6G mobile networks for precision agriculture is envisioned in Figure 3. We foresee a single THz information carrier signal for multiple purposes. For instance, signal attenuation alongside machine learning techniques can be used to sense the plant’s vitals and subsequently transfer power to the neighbouring nano-nodes wirelessly. The contemplated THz-based system can then forward messages out of the nano-network through the micro IoT framework using various backhaul options such as periodic flights of drones, satellite coverage or even terrestrial mobile networks for transfer of information to the internet for data inference and analysis, finally providing useful insights to the stakeholders such as farmers, transporters and consumers.
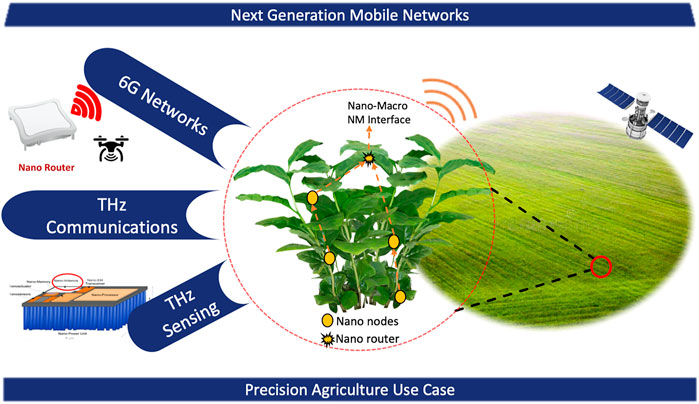
FIGURE 3. THz-based communications and sensing system architecture envisioned for 6G mobile networks.
4.1 Challenges and Future Research Directions
6G and its capabilities are slowly turning from a fantasy to a hypothetical potential. Nevertheless, with particular focus on precision agriculture, realising the envisaged architecture as depicted in Figure 3 has several challenges. Accordingly, we list in this subsection some of the challenges and future directions that would need investigative consideration when further exploiting the use case presented in this paper.
4.1.1 Energy Harvesting
It is of no doubt that there is a great potential in having sensing and communication systems that are based on wireless nano-sensors. Nevertheless, one of the greatest challenges is finding a constant and sufficient source of power to keep them running efficiently (Canovas-Carrasco et al., 2018; Phillips, 2021).
Power consumption expectations from nano-sensors is in the range of nW and μW which means they could be powered using several methods, including from the environment (Wang, 2008). Studies in the literature on Energy Harvesting (EH) for wireless sensors have proposed several options to consider when designing a system such as the one proposed in Figure 3. EH technologies include the utilisation of light energy from Photovoltaics (PV), electromagnetic energy from Radio Frequency (RF) communication, thermal energy from thermoelectric generators, kinetic energy from piezoelectric devices, and others (Tang et al., 2018; Phillips, 2021; Rong et al., 2021). The question that remains though is “What is the ideal way to power the sensors?”. We believe that this depends on several factors a few of which are identified here:
• Application - For a precision agriculture it is crucial to consider solutions that will cause no damage to the plants during the process of harvesting energy and/or during sensors’ maintenance or replacement.
• Sensor’s Power Requirement—Although power expectations are low, a decision towards the energy harvesting technique can not be taken unless a clear vision of what the nano-sensing node will look like.
• System’s Operation - Particulars of the system’s operation will play a role in deciding on the best Energy Harvesting (EH) technique. This includes real-time operation, size of the data, and others.
4.1.2 System Deployment and Sustainability
The deployment of THz infrastructure at different levels in 6G communications network will require a sustainable integration plan and the required equipment, given it will be deployed at nano and macro-scale, for both sensing and communications. Macro scale represents the use of THz to ensure high data rate communication for the implementation of contemporary applications, such as haptic communications, the transportation of high volume data collected from nano-sensors, three-dimensional (3D) calls and tele-operated driving. All of these high data rate applications require a sustainable system architecture that can efficiently integrate the deployed THz systems at macro and nano-levels.
To have this integrated system working, there will be a need of transceivers design at THz frequencies to enable simultaneous communication and sensing at macro and nano-scale. In this regard, THz antenna arrays may be needed, which will require a novel design for highly dense THz array to overcome the physical size of RF transistor and small wavelength as compared to the element spacing in the array. Secondly, the currently deployed medium access control (MAC) layer protocols may not work in such a complex network, where THz systems are deployed at macro and nano-scale for joint communications and sensing. The THz-band is very wide (approximately 10 THz) and the attributes of the carrier frequency significantly varies within this wide band, especially in terms of the transmission distance. Hence, to incorporate such a diverse system, an over hauling of the current MAC protocols deployed in the cellular systems is required.
4.1.3 Practicality of Sensors
An important challenge to consider is the practical aspects of the deployed nano-sensors. For instance, to collecting real time information from the sensors at nano-scale may become a challenge. It may be practically impossible to connect each and every nano-sensor to the network. A solution can be to connect nano-sensors to relatively larger nodes (such macro nodes in Figure 2), which stores the real-time information and send it to the server when needed. Since macro-nodes are also battery-powered devices so an option can be to connect them with the network using unmanned air vehicle (UAV), which may pass over the field once a day to collect all the data from the macro-nodes.
Another important aspect is to identify a suitable location for the sensors to be deployed. A one-to-one number of sensors to number of plants in the field is not practical. Hence, particular areas may be targeted to get an overall plants’ health status in the field. The choice of the location may depend on the types of sensing attributes in terms of sensing the plants, food, soil or the climate. Depending on the attributes and the desired locations, optimal number of required nano-sensors and macro nodes can be estimated to cover sufficiently larger areas with the smallest nodes possible.
4.1.4 Backhaul Agnosticism
The most important technical challenge for mobile networks is the backhaul connectivity to the Internet or required services. The precision agriculture use-case presented in this paper considers last few inches connectivity using THz. Flow of information from the nano-router to the external services that analyse, infer and predict for the stakeholder is the most important challenge and will be a defining moment for future 6G networks. For these networks to work at scale and provide efficient connectivity to the end sensors and users, the network needs to consider an agnostic backhaul.
Agricultural field that contain sensors is vast and can at times be miles long. Even though the terrestrial networks can cover large radius, considering the cost of power and installation, alternate methods are to be explored. If drones are to be used to collect data periodically as suggested in Subsection 4.1.3, some questions that require consideration include: How well a periodic flight of drone be able to capture this data? Will it be able to send this periodic information to satellites? How can this method be made more sustainable? There are still many backhaul related challenges for precise use-cases that we envisage research will address over the next few years.
4.1.5 THz Spectrum Management
Similar to the “Backhaul Agnosticism” challenge, if the network needs to adapt continuously, the transmission characteristics will vary over time. At the same time, in order to keep the network secure, free from interference and attacks, the spectrum in use needs to be regulated. Currently accessing the regulated spectrum takes weeks. We envisage with extensive AI, this spectrum access can be made autonomous and fairly quick. Hence, one of the most important future research direction is towards the optimization of autonomous spectrum management. Reducing this time from weeks to minutes to even seconds can make significant savings in terms of costs and energy.
Similar mechanisms for 5G mobile networks have been proposed and implemented in some countries where the spectrum is allocated autonomously and swiftly. Bringing this mechanism to nano-scale has challenges and further investigation to tackle.
4.2 Limitations of THz Communications
While providing a number of opportunities, THz communications posses some fundamental limitations that should be taken into consideration during the design of THz empowered architecture in future 6G networks. These limitations can be broadly divided into two main categories; 1) channel characteristics and path-loss, and 2) Hardware Limitations.
4.2.1 Channel Characteristics and Path-Loss
Due to the high frequency of THz band, channel characteristics and path-loss need further attention as compared to microwaves. Indeed, free-space path loss, which includes both spreading and atmospheric loss, is the most important propagation feature. High path-loss is one of the primary obstacles to realise THz wireless communication. Further, THz communication has extremely high molecular absorption, which further leads to high propagation path loss. Apart from molecular losses, other types of losses in THz wireless propagation are; spreading losses, reflection and scattering losses and scintillation effects. Considering all these kinds of losses, a 10-m path-loss in a THz link may reach upto 100-dB. This limitation of THz may be overwhelmed by exploiting a cascaded relay infrastructure (Guan et al., 2019).
4.2.2 Hardware Limitations
One of the most pressing concerns about using the THz spectrum is to realise hardware modules, such as transceivers and antennas that are suitable for THz band frequencies. Despite substantial research into Terahertz sensing since the 1990s, the lack of small high-power signal transmitters and high-sensitivity detectors that work at room temperature remains a key challenge. Various electronics and photonics technologies are proposed in the literature to work in THz bands, including silicon-germanium BiCMOS technology, and III-V semiconductor-based High Electron Mobility Transistor (HEMT), metamorphic HEMT, and Heterojunction Biopolar Transistor (HBT) in electronics, and photomixers or photoconductive antennas, uni-traveling carrier photodiodes (UTC) and quantum cascade lasers (QCLs) in photonic technologies. In contrast to the electronic or photonic technologies, the current use of nanomaterials has opened up a new avenue for developing unique plasmonic devices for THz communications, such as graphene. These devices are naturally tiny, work efficiently at THz frequencies, and can handle extremely wide communication bandwidths.
Due to these hardware and communication-distance limitations, the infrastructure cost will be higher in THz based communication networks.
5 Conclusion
The paper envisions the role of THz frequencies in future 6G and beyond networks. The applications of THz frequencies in future 6G networks are discussed in-detail to elaborate its potentials to realise joint communications and sensing. To this aim, the case of precision agriculture is discussed in-detail by presenting a THz aided 6G architecture, which highlights the capabilities of THz in joint communication and sensing. However, the feasibility of such an architecture will require addressing certain challenges that are critical to realise the discussed use-cases of THz in future 6G networks. This paper not only discusses those challenges but presents future research directions to address them.
Author Contributions
Conceptualisation: MU, SA, AT, AZ, QA, and MI. Abstract and Introduction: MU and AT. Section 2: AT, AZ, and SA. Section 3: MU. Section 4: MU, SA, and AT. Conclusions: MU. Review and editing: MU, SA, AT, AZ, QA, and MI. Supervision: QA and MI. All authors have read and agreed to the submitted version of the manuscript.
Conflict of Interest
The authors declare that the research was conducted in the absence of any commercial or financial relationships that could be construed as a potential conflict of interest.
Publisher’s Note
All claims expressed in this article are solely those of the authors and do not necessarily represent those of their affiliated organizations, or those of the publisher, the editors and the reviewers. Any product that may be evaluated in this article, or claim that may be made by its manufacturer, is not guaranteed or endorsed by the publisher.
References
Abbasi, Q. H., Nasir, A. A., Yang, K., Qaraqe, K. A., and Alomainy, A. (2017). Cooperative In-Vivo Nano-Network Communication at Terahertz Frequencies. IEEE Access 5, 8642–8647. doi:10.1109/ACCESS.2017.2677498
Abbasi, Q. H., Yang, K., Chopra, N., Jornet, J. M., Abuali, N. A., Qaraqe, K. A., et al. (2016). Nano-communication for Biomedical Applications: A Review on the State-Of-The-Art from Physical Layers to Novel Networking Concepts. IEEE Access 4, 3920–3935. doi:10.1109/ACCESS.2016.2593582
Abdullahi, S., and Al-Turjman, F. (2020). “6g Applications and Standards-An Overview,” in International Conference on Forthcoming Networks and Sustainability in the IoT Era, Virtual Event, October 1–2, 2020 (Springer), 46–56.
Alomainy, A., Yang, K., Imran, M., Yao, X.-W., and Abbasi, Q. H. (2019). Nano-Electromagnetic Communication at Terahertz and Optical Frequencies: Principles and Applications. Institution of Engineering and Technology.
Alsharif, M. H., Albreem, M. A. M., Solyman, A. A. A., and Kim, S. (2021). Toward 6g Communication Networks: Terahertz Frequency Challenges and Open Research Issues. Comput. Mater. Continua 66, 2831–2842. doi:10.32604/cmc.2021.013176
Barakat, B., Taha, A., Samson, R., Steponenaite, A., Ansari, S., Langdon, P. M., et al. (2021). 6g Opportunities Arising from Internet of Things Use Cases: A Review Paper. Future Internet 13, 159. doi:10.3390/fi13060159
Brown, E. R. (2003). Fundamentals of Terrestrial Millimeter-Wave and Thz Remote Sensing. Int. J. Hi. Spe. Ele. Syst. 13, 995–1097. doi:10.1142/s0129156403002125
Canovas-Carrasco, S., Garcia-Sanchez, A.-J., and Garcia-Haro, J. (2018). On the Nature of Energy-Feasible Wireless Nanosensor Networks. Sensors 18, 1356. doi:10.3390/S18051356
Chaccour, C., Soorki, M. N., Saad, W., Bennis, M., Popovski, P., and Debbah, M. (2021). Seven Defining Features of Terahertz (Thz) Wireless Systems: A Fellowship of Communication and Sensing. arXiv preprint arXiv:2102.07668
Dang, S., Amin, O., Shihada, B., and Alouini, M.-S. (2020). What Should 6G Be? Nat. Electron. 3, 20–29. doi:10.1038/s41928-019-0355-6
Guan, K., Peng, B., He, D., Eckhardt, J. M., Rey, S., Ai, B., et al. (2019). Measurement, Simulation, and Characterization of Train-To-Infrastructure Inside-Station Channel at the Terahertz Band. IEEE Trans. Thz Sci. Technol. 9, 291–306. doi:10.1109/TTHZ.2019.2909975
Khelifi, A., Aziz, O., Farooq, M. S., Abid, A., and Bukhari, F. (2021). Social and Economic Contribution of 5G and Blockchain with Green Computing: Taxonomy, Challenges, and Opportunities. IEEE Access 9, 69082–69099. doi:10.1109/access.2021.3075642
Li, O., He, J., Zeng, K., Yu, Z., Du, X., Liang, Y., et al. (2021). “Integrated Sensing and Communication in 6g a Prototype of High Resolution Thz Sensing on Portable Device,” in 2021 Joint European Conference on Networks and Communications 6G Summit (EuCNC/6G Summit), Porto, Portugal, October 08–11, 2021, 544–549. doi:10.1109/EuCNC/6GSummit51104.2021.9482537
Mukhopadhyay, S. S. (2014). Nanotechnology in Agriculture: Prospects and Constraints. Nsa 7, 63. doi:10.2147/nsa.s39409
Nations, U. (2015). World Population Prospects: The 2015 Revision—Key Findings and advance Tables. [Dataset]. New York: United Nations. doi:10.000/55555
Ojha, T., Misra, S., and Raghuwanshi, N. S. (2015). Wireless Sensor Networks for Agriculture: The State-Of-The-Art in Practice and Future Challenges. Comput. Electron. Agric. 118, 66–84. doi:10.1016/j.compag.2015.08.011
Phillips, J. D. (2021). Energy Harvesting in Nanosystems: Powering the Next Generation of the Internet of Things. Front. Nanotechnol. 3, 5. doi:10.3389/fnano.2021.633931
Rong, G., Zheng, Y., and Sawan, M. (2021). Energy Solutions for Wearable Sensors: A Review. Sensors (Basel) 21, 3806. doi:10.3390/s21113806
Rong, Z., Leeson, M. S., Higgins, M. D., and Lu, Y. (2017). Simultaneous Wireless Information and Power Transfer for Af Relaying Nanonetworks in the Terahertz Band. Nano Commun. Networks 14, 1–8. doi:10.1016/j.nancom.2017.08.003
Said Mohamed, E., Belal, A., Kotb Abd-Elmabod, S., El-Shirbeny, M. A., Gad, A., and Zahran, M. B. (2021). Smart Farming for Improving Agricultural Management. Egypt. J. Remote Sensing Space Sci. 24, 971–981. doi:10.1016/j.ejrs.2021.08.007
Schönfeld, M. v., Heil, R., and Bittner, L. (2018). “Big Data on a Farm—Smart Farming,” in Big Data in Context. Editors T. Hoerenr, and B. Kolany-Raiser (Springer, Cham: Springer Briefs in Law, 109–120. doi:10.1007/978-3-319-62461-7_12
Song, Z., Yan, S., Zang, Z., Fu, Y., Wei, D., Cui, H.-L., et al. (2018). Temporal and Spatial Variability of Water Status in Plant Leaves by Terahertz Imaging. IEEE Trans. Thz Sci. Technol. 8, 520–527. doi:10.1109/tthz.2018.2851922
Tagarakis, A. C., Kateris, D., Berruto, R., and Bochtis, D. (2021). Low-cost Wireless Sensing System for Precision Agriculture Applications in Orchards. Appl. Sci. 11, 5858. doi:10.3390/app11135858
Tang, X., Wang, X., Cattley, R., Gu, F., and Ball, A. (2018). Energy Harvesting Technologies for Achieving Self-Powered Wireless Sensor Networks in Machine Condition Monitoring: A Review. Sensors 18, 4113. doi:10.3390/s18124113
Tang, Y., Dananjayan, S., Hou, C., Guo, Q., Luo, S., and He, Y. (2021). A Survey on the 5g Network and its Impact on Agriculture: Challenges and Opportunities. Comput. Electron. Agric. 180, 105895. doi:10.1016/j.compag.2020.105895
Taylor, Z. D., Garritano, J., Sung, S., Bajwa, N., Bennett, D. B., Nowroozi, B., et al. (2015). Thz and Mm-Wave Sensing of Corneal Tissue Water Content: In Vivo Sensing and Imaging Results. IEEE Trans. Thz Sci. Technol. 5, 184–196. doi:10.1109/TTHZ.2015.2392628
Usman, M., Asghar, M. R., Ansari, I. S., and Qaraqe, M. (2018). Security in Wireless Body Area Networks: From In-Body to Off-Body Communications. IEEE Access 6, 58064–58074. doi:10.1109/ACCESS.2018.2873825
Wang, Z. L. (2008). Energy Harvesting for Self-Powered Nanosystems. Nano Res. 1, 1–8. doi:10.1007/s12274-008-8003-x
Wedage, L. T., Butler, B., Balasubramaniam, S., Koucheryavy, Y., and Jornet, J. M. (2021). Climate Change Sensing through Terahertz Communications: A Disruptive Application of 6g Networks. arXiv preprint arXiv:2110.03074.
Zahid, A., Abbas, H. T., Qaraqe, K. A., Alomainy, A., Cumming, D. R. S., Abbasi, Q. H., et al. (2019b). Characterization and Water Content Estimation Method of Living Plant Leaves Using Terahertz Waves. Appl. Sci. 9, 2781. doi:10.3390/app9142781
Zahid, A., Abbas, H. T., Heidari, H., Imran, M., Alomainy, A., and Abbasi, Q. H. (2019a). “Electromagnetic Properties of Plant Leaves at Terahertz Frequencies for Health Status Monitoring,” in 2019 IEEE MTT-S International Microwave Biomedical Conference (IMBioC), Nanjing, China, May 06–08, 2021, 1–4. doi:10.1109/IMBIOC.2019.8777782
Zahid, A., Abbas, H. T., Ren, A., Alomainy, A., Imran, M. A., and Abbasi, Q. H. (2020). “Application of Terahertz Sensing at Nano‐Scale for Precision Agriculture,” in Wireless Automation as an Enabler for the Next Industrial Revolution. Hoboken, NJ: Wiley, 241–257. doi:10.1002/9781119552635.ch11
Zahid, A., Yang, K., Heidari, H., Li, C., Imran, M. A., Alomainy, A., et al. (2018). “Terahertz Characterisation of Living Plant Leaves for Quality of Life Assessment Applications,” in 2018 Baltic URSI Symposium (URSI), Poznan, Poland, May 14–17, 2018, 117–120. doi:10.23919/URSI.2018.8406770
Keywords: terahertz (THz), agritech, 6G, nano scale, communication and sensing
Citation: Usman M, Ansari S, Taha A, Zahid A, Abbasi QH and Imran MA (2022) Terahertz-Based Joint Communication and Sensing for Precision Agriculture: A 6G Use-Case. Front. Comms. Net 3:836506. doi: 10.3389/frcmn.2022.836506
Received: 15 December 2021; Accepted: 17 January 2022;
Published: 11 March 2022.
Edited by:
Akram Alomainy, Queen Mary University of London, United KingdomReviewed by:
Syeda Fizzah Jilani, Aberystwyth University, United KingdomImene Mitiche, Glasgow Caledonian University, United Kingdom
Syed Aziz Shah, Coventry University, United Kingdom
Copyright © 2022 Usman, Ansari, Taha, Zahid, Abbasi and Imran. This is an open-access article distributed under the terms of the Creative Commons Attribution License (CC BY). The use, distribution or reproduction in other forums is permitted, provided the original author(s) and the copyright owner(s) are credited and that the original publication in this journal is cited, in accordance with accepted academic practice. No use, distribution or reproduction is permitted which does not comply with these terms.
*Correspondence: Muhammad Ali Imran, Muhammad.Imran@glasgow.ac.uk