- 1Department of Electronics and Communication Engineering, Istanbul Technical University, Istanbul, Turkey
- 2Communications Research and Innovation Laboratory (CoreLab), Department of Electrical and Electronics Engineering, Koç University, Istanbul, Turkey
Reconfigurable intelligent surface (RIS)-empowered communication is a revolutionary technology that enables to manipulate wireless propagation environment via smartly controllable low-cost reflecting surfaces. However, in order to outperform conventional communication systems, an RIS-aided system with solely passive reflection requires an extremely large surface. To meet this challenge, the concept of active RIS, which performs simultaneous amplification and reflection on the incident signal at the expense of additional power consumption, has been recently introduced. In this paper, deploying an active RIS, we propose a novel beamforming concept, over-the-air beamforming, for RIS-aided multi-user multiple-input single-output (MISO) transmission schemes without requiring any pre/post signal processing hardware designs at the transmitter and receiver sides. In the proposed over-the-air beamforming-based transmission scheme, the reflection coefficients of the active RIS elements are customized to maximize the sum-rate gain. To tackle this issue, first, a non-convex quadratically constrained quadratic programming (QCQP) problem is formulated. Then, using semidefinite relaxation (SDR) approach, this optimization problem is converted to a convex feasibility problem, which is efficiently solved using the CVX optimization toolbox. Moreover, taking inspiration from this beamforming technique, a novel high-rate receive index modulation (IM) scheme with a low-complexity sub-optimal detector is developed. Through comprehensive simulation results, the sum-rate and bit error rate (BER) performance of the proposed designs are investigated.
1 Introduction
Customizing propagation environment via reconfigurable intelligent surfaces (RISs) has been an appealing field for wireless communication and provides novel insights about future generation networks. These light-weight and cost-effective electronic elements have been regarded as a game changer technology for conventional communication systems with power-hungry and complex hardware designs (Basar et al., 2019). Particularly, RISs are programmable metasurfaces that are capable of configuring the propagation environment in a desired manner via performing reflection, amplification, absorption, refraction, etc. (Di Renzo et al., 2020). However, the most of the extant literature particularly focuses on the application of the RIS with passive reflection in various emerging systems (Basar et al., 2019; Di Renzo et al., 2020; Gong et al., 2020).
In the early studies, a passive RIS is deployed for enhancing transmit signal quality of single-antenna (Basar, 2019) and multiple-antenna systems (Yu et al., 2019; Yigit et al., 2020; Zhang et al., 2020). In subsequent studies, an RIS is facilitated for numerous objectives of single-user and multi-user systems, such as promoting energy-efficiency (Björnson et al., 2019; Huang et al., 2019), enhancing error performance (Ferreira et al., 2020; Ye et al., 2020) and improving achievable rate (Di et al., 2020; Zhang and Zhang, 2020; Perović et al., 2021). Further, novel deep learning-based solutions for passive RIS designs (Kundu and McKay, 2021; Taha et al., 2021) and security enhanced RIS-aided communication systems (Shen et al., 2019), (Almohamad et al., 2020; Dong and Wang, 2020) are proposed. On the other hand, index modulation (IM) principle, which is emerged as a promising energy-efficient solution to meet high data-rate demand of future wireless networks (Basar et al., 2017), is beneficially amalgamated into the RIS-empowered communication (Basar, 2020; Li et al., 2021). Considering more conventional IM designs (Basar, 2020; Li et al., 2021), put forward RIS-aided receive IM schemes, which maximize the signal powers of the target receive antennas. However, in (Guo et al., 2020; Lin et al., 2020), novel reflection modulation (RM) concepts, which innovatively utilize the RISs for delivering additional information, are proposed. Above all, main limitation of the aforementioned studies is the lack of comprehensive practical insights on considered system configurations. Towards this aim, a low-complexity joint beamforming optimization that considers the effect of hardware impairments on the performance of RIS-aided multi-antenna systems are investigated in (Shen et al., 2021), different RIS prototypes are introduced for real-time implementations in (Dai et al., 2020; Tang et al., 2020), and realistic physical channel models for millimeter-wave (mmWave) (Basar et al., 2021) and sub-6 GHz bands (Yigit et al., 2021a; Kilinc et al., 2021) are presented. Nevertheless, the abovementioned system designs suffer from the multiplicative path attenuation due to the inherent drawback of the RIS-aided designs, and achieve negligible performance gains over the conventional communication systems.
Recently, to tackle above challenges, the concept of active RIS, which performs simultaneous amplification and reflection on the incident wave, is introduced in (Long et al., 2021; Zhang et al., 2021). Accordingly, the magnitudes and the phases of the reflecting elements of the active RIS, which are equipped with additional power amplifiers, are properly tuned in a customized way (Basar and Poor, 2021). Therefore, at the cost of additional power consumption, active RIS-aided systems are capable of achieving enhanced capacity gains (Long et al., 2021). In a recent study on designing active RISs, via leveraging power amplifiers and radio frequency (RF) chains (Nguyen et al., 2022), dynamic and fixed hybrid RIS architectures are constructed. Further, for improving the data rate, a new RM design, which employs the sub-groups of a hybrid RIS as information transfer units, is presented in (Yigit et al., 2021b). In follow-up studies, the concept of the active RIS is deployed for beamforming optimization of the RIS-aided multi-user systems (Gao et al., 2022; Thanh Nguyen et al., 2022). Above all, the potential of the active RIS-aided systems for achieving enormous performance gains will enable to develop promising solutions for future research.
In this study, unlike the conventional precoding techniques that employ power-hungry and hardware-complex devices (Sohrabi and Yu, 2016), for RIS-aided multi-user downlink transmission systems, we propose a novel over-the-air beamforming technique with the aid of an active RIS to exploit its capability of manipulating the magnitude of the incident wave. In other words, the main motivation of the over-the-air beamforming scheme is to simplify the transmitter and receiver ends of the overall network while transferring inter-user interference elimination tasks completely to an active RIS. Therefore, this paper proposes two novel over-the-air beamforming schemes that mitigate the burden of signal processing on the transmitter and receiver sides. In the proposed over-the-air beamforming-based transmission scheme, it is assumed that a multi-antenna transmitter serves K single-antenna users through an active RIS without utilizing any other signal processing tasks at the transmitter and the receiver sides. Then, the reflection coefficients of the active RIS is properly adjusted to maximize the sum-rate of the overall system. Moreover, taking inspiration from this over-the-air beamforming concept, a new receive IM scheme that transmits additional information bits to specify the index of the effective received antenna is also proposed. Contrary to the traditional receive IM systems (Zhang et al., 2013; Luo et al., 2021), in the proposed system, since no precoding is applied at the transmitter, the reflection coefficients of the active RIS are rectified to steer the incident signal into the intended receive antenna. On the other hand, since the receive IM scheme benefits from the multi-antenna transmission at the user side and IM system design at the receiver side, it shows the favourable features of both, such as high spectral efficiency and improved performance. In these proposed over-the-air downlink beamforming and over-the-air uplink receive IM schemes, to optimize reflection coefficients of the active RISs, two distinct semidefinite relaxation (SDR)-based optimization problems are formulated, which can be effectively solved through the CVX convex optimization toolbox (Grant and Boyd, 2008). Furthermore, the achievable rate and bit error rate (BER) performance of the proposed over-the-air beamforming-based transmission schemes are investigated through extensive computer simulations.
The rest of the paper is organized as follows. In Section 2, after giving a short review of the conventional zero-forcing (ZF) precoding, we introduce the system model of the proposed over-the-air beamforming-based multi-user multi-antenna transmission scheme. In Section 3, the over-the-air beamforming-based receive IM scheme and its low-complexity receiver detection are introduced. Section 4 provides the achievable rate and BER results of the proposed over-the-air beamforming based transmission systems, and the conclusions are drawn in Section 5.
Notations: Throughout this paper, matrices and vectors are denoted by boldface upper-case and boldface lower-case letters, respectively (⋅)T represents transpose and (⋅)H denotes the Hermitian transpose operation.
2 Over-the-air beamforming with RIS
In this section, after a review of conventional transmit precoding, the over-the-air beamforming concept is introduced for multi-user multiple-input single-output (MISO) downlink transmission systems.
2.1 Conventional transmit precoding
Considering a typical multi-user downlink transmission system without an RIS, a base station (BS) transmitter (T) with Tx antennas is assumed to perform ZF precoding to alleviate interference between K single-antenna users (Spencer et al., 2004). Let
where xk being an M-ary phase shift keying (PSK) signal to be transmitted over the k-th transmit antenna. Here,
Moreover, the overall transmit ZF precoding matrix, exploiting the perfect CSI, can be obtained as (Spencer et al., 2004)
where
2.2 System model of over-the-air beamforming with RIS
In this subsection, after a brief introduction of the active RIS concept, the system model of the over-the-air beamforming-based multi-user transmission system is introduced.
2.2.1 Active RIS
The principal drawback of RIS-aided communication systems is the inherent multiplicative path attenuation along the RIS-aided indirect link, which is hardly compensated by the RIS with passive reflecting elements (Basar and Poor, 2021; Zhang et al., 2021). Therefore, to overcome this challenge, an RIS architecture with active reflecting elements that enable to configure both the magnitude and phase of the incident wave at the expense of an additional power consumption, is recently proposed (Khoshafa et al., 2021; Zhang et al., 2021). Therefore, unlike the passive RISs, the active RISs reflect incident signal with amplification via employing additional power circuitry. Although the active reflecting elements have a similar capability of amplifying the incident signal as in the full-duplex amplify-and-forward (AF) relays, their hardware constructions are completely different from each other. While the AF relays embody a circuitry for amplification in their hardware constructions and they are also externally equipped with high power-consuming RF chains to transmit and receive signals (Wu and Zhang, 2019), the active reflecting elements employ reflective-type power amplifiers to simultaneously rectify the magnitude and phase of the incident wave (Zhang et al., 2021).
2.2.2 System model
An overwhelming literature on passive RIS-aided multi-user transmission deploys the RIS as a passive beamformer after a preprocessing is conducted at the transmitter (Wu and Zhang, 2019; Yan et al., 2020). However, in the proposed over-the-air beamforming concept, to avoid power-hungry hardware constructions at the transmitter and the users, exploiting simultaneous amplification and reflection capabilities of the active reflecting elements, both active and passive beamforming are carried out at an active RIS. Accordingly, the reflection coefficients of the active RIS are optimized to maximize the achievable rate of the overall system.
As given in Figure 1, in the proposed scheme, the direct transmission links between T with Tx antennas and K single-antenna users are neglected due to obstacles, thus, the communication is established through an active RIS with N reflecting elements. In the proposed over-the-air beamforming-based multi-user transmission, it is assumed that T and the users have the perfect channel state information (CSI) about T-RIS and RIS-users channels, which is conveyed to a smart RIS controller via a feedback control link (Wu and Zhang, 2019). Moreover, at the transmitter side, without requiring any additional signal processing approaches for interference mitigation, the overall signal is conveyed to the users through the RIS. Hence, unlike the traditional beamforming techniques that employ complex and power-hungry signal processing hardware (El Ayach et al., 2014; Sohrabi and Yu, 2016), the RIS is designed as a beamformer to alleviate multi-user interference by adjusting the amplitude and phase of each reflecting element. Towards this aim, the RIS elements are assumed to be equipped with additional power circuitry to modify both the magnitude and the phase of the incident signal (Yigit et al., 2021b; Zhang et al., 2021; Nguyen et al., 2022). Furthermore, in the proposed system, since all transmit antennas simultaneously convey their own M-PSK modulated signals, a spectral efficiency of ηMU = Tx log2(M) [bits/s/Hz] is achieved.
Let us assume that the channels between T-RIS are presented by the matrix
In addition, the RIS architecture that is equipped with additional power circuitry to operate as an active RIS (Zhang et al., 2021), is represented in a diagonal matrix
where
where H = [H1, H2, … , HK] and
Accordingly, the sum-rate of the overall system becomes:
Then, to maximize this sum-rate, the reflection coefficients of the active RIS elements are optimized. In what follows, the corresponding problem formulation and the proposed solution are presented.
2.3 Problem formulation and proposed solution
In the over-the-air beamforming-based multi-user transmission scheme, interference cancellation is performed at the RIS without employing any additional integrated high-cost signal processing circuitry, such as multiple RF chains, either at T or user sides. For this purpose, the reflection coefficients of the RIS are adjusted to maximize the SINR of the intended Uk. Therefore, to deal with this problem, the following QCQP problem is formulated.
where Γk is the minimum SINR requirement of Uk, PBS = KPk, and PA is the maximum reflection power introduced by the active reflecting elements. Please note that for the over-the-air beamforming-based multi-user systems, the total power PT is the sum of power dissipated at the transmitter (PBS) and the RIS (PA), that is PT = PA + PBS, while for the conventional transmission without RIS, PT denotes to total power consumed at the transmitter. Then, using the Cauchy-Schwarz inequality, the constraint in (10) can be rewritten as
Therefore, since the problem (P1) is non-convex and it is difficult to obtain an optimal solution, we resort to the SDR technique and define new variables
where
Here, Z is a positive semidefine matrix and rank(Z) = 1. However, since the rank-one constraint is non-convex, we remove this constraint and reformulate (P2) as a convex feasibility problem as follows.
Finally, through the existing solvers of CVX toolbox (Grant and Boyd, 2008), a feasible solution of (P3) satisfying the inequality constraints in (17) and (18) is obtained. However, after the relaxation, the optimal solution of (P3) cannot always ensure the rank-one solution. Therefore, for
where
3 Over-the-air receive index modulation
In this section, the proposed over-the-air beamforming concept is adopted to a novel receive IM transmission scheme. Considering the over-the-air beamforming approach given in Section II, a single-user uplink transmission of an active RIS-aided IM transmission system is developed.
3.1 System model of over-the-air receive IM
As given in Figure 2, in the proposed IM system, due to presence of the obstacles over the direct links, a multi-antenna user communicates with an Rx-antenna receiver (R) through an RIS with N reflecting elements. Besides, an RIS controller is attached to the RIS that exchanges the information through a feedback control link. In the proposed system, considering the IM transmission principle (Basar, 2020), an over-the-air receive IM scheme is developed. Unlike traditional receive IM schemes (Stavridis et al., 2012; Zhang et al., 2013; Luo et al., 2021) that deploy transmit precoding techniques via high-cost hardware devices for preprocessing the transmit signal before its transmission, the proposed receive IM scheme employs the RIS as a signal processing unit and apply an over-the-air beamforming at the RIS. In the over-the-air receive IM scheme, at the user side, a conventional multi-antenna transmission is considered. Moreover, in order to attain higher data rates, extra information bits are conveyed via indicating the active receive antenna index. Therefore, the incoming information bits are used to determine the modulated M-PSK symbols for each of the available Tx transmit antennas, as well as to specify the active receive antenna index, one out of Rx receive antennas. Therefore, the spectral efficiency achieved by this novel receive IM scheme is calculated as
In this system, the information of the active receive antenna index and perfect channel knowledge of user-RIS and RIS-R links is shared by the user to the RIS through the smart controller. Then, the reflection coefficient of the RIS elements are adjusted to ensure that the target receive antenna has the strongest received signal power. In other words, by the means of active reflecting elements, the RIS acts as a kind of digital beamformer and steers the overall signal along the desired receive antenna direction.
Let the multi-path fading channels between user-RIS and RIS-R links are modeled as the independent Rayleigh fading channels, which are denoted by the channel matrices of
where
Therefore, to address this problem, for Θr = diag (gr)H and
Then, resorting to SDR, the problem (P4) is expressed as.
Here, for δr ≫ 1,
3.2 Low-complexity successive greedy detector
In the subsection that follows, a sub-optimal successive detection algorithm for the proposed receive IM scheme is proposed. In the proposed system, after the optimization of the reflection matrix Ψr for the specified r-th receive antenna, it is straightforward to exploit a maximum likelihood (ML) detector that jointly estimates the “spatial symbol” r and the overall transmit signal vector x as follows
However, in the proposed receive IM scheme, in order to save the computational complexity, instead of considering joint detection, the receiver reconstructs the transmit information via a low-complexity greedy detector that perform the successive detection in the following way. First, using amplitude detectors, the index of the active receive antenna is detected as
Then, exploiting the ML detector, the transmit signal vector x is estimated, by considering all possible x realizations, as follows
Moreover, from the computational complexity standpoint, we note that since the complexity of SDR problem (P5) is
4 Numerical results
In this section, the sum-rate and BER performance of the proposed over-the-air beamforming-based single-user and multi-user downlink transmission, and uplink receive IM schemes are presented through the Monte Carlo simulations. Moreover, comparing to the ZF-based conventional transmission (Spencer et al., 2004; Zhang et al., 2013) and the state-of-the-art RIS-aided joint beamforming schemes (Wu and Zhang, 2019), the improved performance of the over-the-air beamforming-based systems are illustrated.
In all computer simulations, the following system setups are considered: the reference path loss value is C0 = −30 dBm, the noise variances are
4.1 Downlink transmission
In this subsection, the numerical results of the proposed over-the-air beamforming and the benckmark schemes for single-user and multi-user downlink systems are demonstrated.
4.1.1 Single-user
The following computer simulation results are performed for single-user MISO transmission schemes.
In Figure 3, for a single-user downlink transmission (K = 1) with
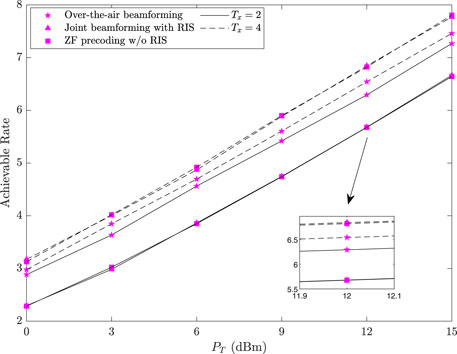
FIGURE 3. Comparison of the achievable rate performance of the proposed over-the-air beamforming with traditional ZF precoding (Spencer et al., 2004) and joint beamforming with RIS (Wu and Zhang, 2019) for single-user system configurations.
4.1.2 Multi-user
The following results are carried out for downlink multi-antenna transmission schemes, where a single transmit antenna is allocated to each user, i.e.,
In Figure 4, the sum rate of the downlink multi-antenna transmission scheme based on the conventional transmit ZF precoding (Spencer et al., 2004) and the novel over-the-air beamforming has been carried out for
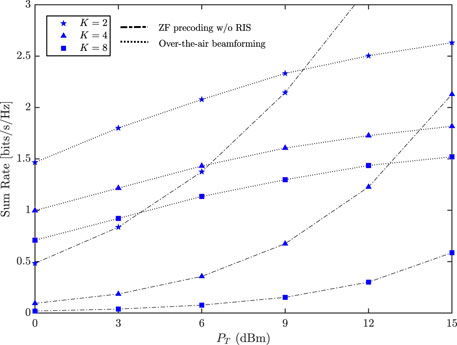
FIGURE 4. Sum-rate comparison of the proposed over-the-air beamforming and the classical transmit ZF precoding for
In Figure 5, the sum-rate of the proposed over-the-air beamforming-based downlink multi-user system is evaluated for different system configurations. In this case, for a constant PT, the performance of the over-the-air beamforming based systems are investigated for different number of the reflecting elements N, PBS = 0 dBm and QPSK signaling. It is observed that increasing RIS size has an adverse affect on the system performance. This results may be explained by the fact that in the over-the-air beamforming design, as given in (11), the power consumed by the reflecting elements is inversely proportional with the magnitude of the channel matrix H. Therefore, when a constant PA is considered for N = 16 and N = 64, it reveals that the proposed beamforming-based systems with the lower N values show considerably better performance than the ones with the higher N values.
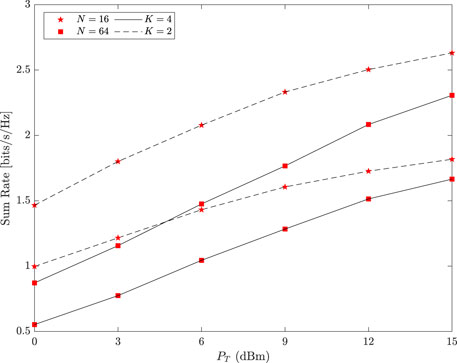
FIGURE 5. Sum-rate performance of the proposed over-the-air beamforming-based multi-user systems for different system configurations.
In Figure 6, the effect of increasing reflection power PA on the sum-rate of the proposed beamforming based systems with QPSK and PT = 30 dBm is investigated for N = 16. The results show that in all cases, the increasing PA improves the system performance up to a certain PA value, after which the performance begins to degrade. These results indicate the relation between the reflection power constraint PA and transmitter power PBS in (10). Indeed, in our system design, the overall consumed power PT is dissipated to the transmitter (PBS) and the RIS (PA), where PT = PBS + PA, and for a constant PT = 30 dBm, PBS decreases with increasing PA. However, it is clear from (6) that the minimizing PBS directly affects the SINR value. Surely, the investigation of this interesting trade-off points out the importance of the power allocation between the transmitter and the RIS, which is an open problem to be addressed in future studies.
4.2 Single-user uplink transmission
In this subsection, the BER performance of the proposed receive IM scheme is evaluated.
In Figure 7, the BER performance of the proposed receive IM scheme with sub-optimal greedy detector is investigated for different RIS-aided MIMO configurations with N = 16 and binary PSK (BPSK). Similar to the conventional receive IM schemes (Zhang et al., 2013; Wu et al., 2021), the performance results of the corresponding high-rate systems that employ a) Tx = 2 and b) Tx = 4 transmit antennas reveal a certain trade-off between system performance and data-rate.
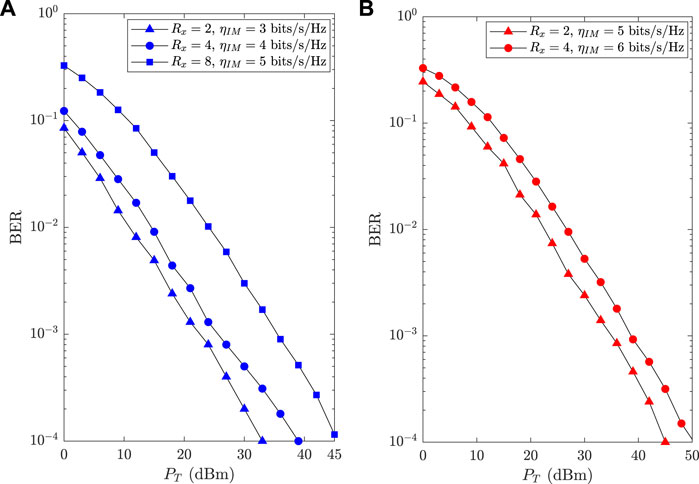
FIGURE 7. BER performance of the novel receive IM scheme with greedy detector for N = 16 and (A) Tx = 2, (B) Tx = 4.
In Figure 8, the BER performance of the transmit ZF precoded receive spatial modulation (RSM) (Zhang et al., 2013) and the proposed over-the-air receive IM schemes are compared. For Rx = Tx = 2, the receive IM and the RSM schemes respectively exploit BPSK and QPSK modulations to achieve ηIM = 3 bits/s/Hz. On the other hand, for Rx = Tx = 4 configuration, the receive IM with BPSK and the RSM with 16-PSK assess ηIM = 6 bits/s/Hz. The results demonstrate the significant performance improvement of the proposed receive IM scheme over the traditional ZF precoded RSM (Zhang et al., 2013).
5 Conclusion
In this paper, first, deploying an active RIS, a novel beamforming approach has been proposed for RIS-aided multi-user systems. In the proposed concept, without employing any other signal processing units at the transmitter and/or receiver sides, the reflection coefficients of the active RIS have been customized to mitigate the user interference. To meet this challenge, we have obtained SDR-based solutions via CVX software toolbox. Moreover, taking the proposed over-the-air beamforming concept one step further, a low-complexity receive IM scheme has been developed for single-user uplink transmission. Through computer simulations, the enhanced performance of the over-the-air beamforming-based systems over the traditional precoding-based systems have been indicated.
Data availability statement
The original contributions presented in the study are included in the article/supplementary material, further inquiries can be directed to the corresponding author.
Author contributions
ZY, EB, and IA contributed to the design and implementation of the research, to the analysis of the results and to the writing of the manuscript.
Funding
This work was supported by the Scientific and Technological Research Council of Turkey (TUBITAK)-COST project 120E401.
Conflict of interest
The authors declare that the research was conducted in the absence of any commercial or financial relationships that could be construed as a potential conflict of interest.
Publisher’s note
All claims expressed in this article are solely those of the authors and do not necessarily represent those of their affiliated organizations, or those of the publisher, the editors and the reviewers. Any product that may be evaluated in this article, or claim that may be made by its manufacturer, is not guaranteed or endorsed by the publisher.
References
Almohamad, A., Tahir, A. M., Al-Kababji, A., Furqan, H. M., Khattab, T., Hasna, M. O., et al. (2020). Smart and secure wireless communications via reflecting intelligent surfaces: A short survey. IEEE Open J. Commun. Soc. 1, 1442–1456. doi:10.1109/OJCOMS.2020.3023731
Basar, E., Di Renzo, M., De Rosny, J., Debbah, M., Alouini, M.-S., and Zhang, R. (2019). Wireless communications through reconfigurable intelligent surfaces. IEEE Access 7, 116753–116773. doi:10.1109/access.2019.2935192
Basar, E., and Poor, H. V. (2021). Present and future of reconfigurable intelligent surface-empowered communications [perspectives]. IEEE Signal Process. Mag. 38, 146–152. doi:10.1109/MSP.2021.3106230
Basar, E. (2020). Reconfigurable intelligent surface-based index modulation: A new beyond MIMO paradigm for 6G. IEEE Trans. Commun. 68, 3187–3196. doi:10.1109/TCOMM.2020.2971486
Basar, E. (2019). “Transmission through large intelligent surfaces: A new frontier in wireless communications,” in Proceeding of the 2019 European Conference on Networks and Communications (EuCNC), Valencia, Spain, June 2019 (IEEE), 112–117.
Basar, E., Wen, M., Mesleh, R., Di Renzo, M., Xiao, Y., and Haas, H. (2017). Index modulation techniques for next-generation wireless networks. IEEE Access 5, 16693–16746. doi:10.1109/ACCESS.2017.2737528
Basar, E., Yildirim, I., and Kilinc, F. (2021). Indoor and outdoor physical channel modeling and efficient positioning for reconfigurable intelligent surfaces in mmWave bands. IEEE Trans. Commun. 69, 8600–8611. doi:10.1109/TCOMM.2021.3113954
Björnson, E., Özdogan, Ö., and Larsson, E. G. (2019). Intelligent reflecting surface versus decode-and-forward: How large surfaces are needed to beat relaying? IEEE Wireless. Commun. Lett. 9, 244–248. doi:10.1109/LWC.2019.2950624
Dai, L., Wang, B., Wang, M., Yang, X., Tan, J., Bi, S., et al. (2020). Reconfigurable intelligent surface-based wireless communications: Antenna design, prototyping, and experimental results. IEEE Access 8, 45913–45923. doi:10.1109/ACCESS.2020.2977772
Di, B., Zhang, H., Song, L., Li, Y., Han, Z., and Poor, H. V. (2020). Hybrid beamforming for reconfigurable intelligent surface based multi-user communications: Achievable rates with limited discrete phase shifts. IEEE J. Sel. Areas Commun. 38, 1809–1822. doi:10.1109/JSAC.2020.3000813
Di Renzo, M., Zappone, A., Debbah, M., Alouini, M.-S., Yuen, C., De Rosny, J., et al. (2020). Smart radio environments empowered by reconfigurable intelligent surfaces: How it works, state of research, and the road ahead. IEEE J. Sel. Areas Commun. 38, 2450–2525. doi:10.1109/JSAC.2020.3007211
Dong, L., and Wang, H.-M. (2020). Secure MIMO transmission via intelligent reflecting surface. IEEE Wireless. Commun. Lett. 9, 787–790. doi:10.1109/LWC.2020.2969664
El Ayach, O., Rajagopal, S., Abu-Surra, S., Pi, Z., and Heath, R. W. (2014). Spatially sparse precoding in millimeter wave mimo systems. IEEE Trans. Wireless. Commun. 13, 1499–1513. doi:10.1109/TWC.2014.011714.130846
Ferreira, R. C., Facina, M. S., De Figueiredo, F. A., Fraidenraich, G., and De Lima, E. R. (2020). Bit error probability for large intelligent surfaces under double-Nakagami fading channels. IEEE Open J. Commun. Soc. 1, 750–759. doi:10.1109/OJCOMS.2020.2996797
Gao, Y., Wu, Q., Zhang, G., Chen, W., Ng, D. W. K., and Di Renzo, M. (2022). Beamforming optimization for active intelligent reflecting surface-aided SWIPT. arXiv preprint arXiv:2203.16093.
Gong, S., Lu, X., Hoang, D. T., Niyato, D., Shu, L., Kim, D. I., et al. (2020). Toward smart wireless communications via intelligent reflecting surfaces: A contemporary survey. IEEE Commun. Surv. Tutorials 22, 2283–2314. doi:10.1109/COMST.2020.3004197
Grant, M., and Boyd, S. (2008). “Graph implementations for nonsmooth convex programs,” in Recent advances in learning and control. Lecture notes in control and information sciences. Editors V. Blondel, S. Boyd, and H. Kimura (Springer-Verlag Limited), 95–110. Available at: http://stanford.edu/∼boyd/graph_dcp.html.
Guo, S., Lv, S., Zhang, H., Ye, J., and Zhang, P. (2020). Reflecting modulation. IEEE J. Sel. Areas Commun. 38, 2548–2561. doi:10.1109/JSAC.2020.3007060
Huang, C., Zappone, A., Alexandropoulos, G. C., Debbah, M., and Yuen, C. (2019). Reconfigurable intelligent surfaces for energy efficiency in wireless communication. IEEE Trans. Wireless. Commun. 18, 4157–4170. doi:10.1109/TWC.2019.2922609
Khoshafa, M. H., Ngatched, T. M., Ahmed, M. H., and Ndjiongue, A. R. (2021). Active reconfigurable intelligent surfaces-aided wireless communication system. IEEE Commun. Lett. 25, 3699–3703. doi:10.1109/LCOMM.2021.3110714
Kilinc, F., Yildirim, I., and Basar, E. (2021). “Physical channel modeling for RIS-empowered wireless networks in sub-6 GHz bands,” in Proceeding of the 2021 55th Asilomar Conference on Signals, Systems, and Computers (IEEE), 704–708.
Kundu, N. K., and McKay, M. R. (2021). Channel estimation for reconfigurable intelligent surface aided MISO communications: From LMMSE to deep learning solutions. IEEE Open J. Commun. Soc. 2, 471–487. doi:10.1109/OJCOMS.2021.3063171
Li, Q., Wen, M., and Di Renzo, M. (2021). Single-RF MIMO: From spatial modulation to metasurface-based modulation. IEEE Wireless. Commun. 28, 88–95. doi:10.1109/MWC.021.2000376
Lin, S., Zheng, B., Alexandropoulos, G. C., Wen, M., Di Renzo, M., and Chen, F. (2020). Reconfigurable intelligent surfaces with reflection pattern modulation: Beamforming design and performance analysis. IEEE Trans. Wireless. Commun. 20, 741–754. doi:10.1109/TWC.2020.3028198
Long, R., Liang, Y.-C., Pei, Y., and Larsson, E. G. (2021). Active reconfigurable intelligent surface-aided wireless communications. IEEE Trans. Wireless. Commun. 20, 4962–4975. doi:10.1109/TWC.2021.3064024
Luo, Z.-Q., Ma, W.-K., So, A. M.-C., Ye, Y., and Zhang, S. (2010). Semidefinite relaxation of quadratic optimization problems. IEEE Signal Process. Mag. 27, 20–34. doi:10.1109/MSP.2010.936019
Luo, S., Yang, P., Che, Y., Yang, K., Wu, K., Teh, K. C., et al. (2021). Spatial modulation for RIS-assisted uplink communication: Joint power allocation and passive beamforming design. IEEE Trans. Commun. 69, 7017–7031. doi:10.1109/TCOMM.2021.3096965
Nguyen, N. T., Vu, D., Lee, K., and Juntti, M. (2022). Hybrid relay-reflecting intelligent surface-assisted wireless communications. IEEE Trans. Veh. Technol. 71, 6228–6244. doi:10.1109/TVT.2022.3158686
Perović, N. S., Tran, L.-N., Di Renzo, M., and Flanagan, M. F. (2021). Achievable rate optimization for MIMO systems with reconfigurable intelligent surfaces. IEEE Trans. Wireless. Commun. 20, 3865–3882. doi:10.1109/TWC.2021.3054121
Shen, H., Xu, W., Gong, S., He, Z., and Zhao, C. (2019). Secrecy rate maximization for intelligent reflecting surface assisted multi-antenna communications. IEEE Commun. Lett. 23, 1488–1492. doi:10.1109/LCOMM.2019.2924214
Shen, H., Xu, W., Gong, S., Zhao, C., and Ng, D. W. K. (2021). Beamforming optimization for IRS-aided communications with transceiver hardware impairments. IEEE Trans. Commun. 69, 1214–1227. doi:10.1109/TCOMM.2020.3033575
Sohrabi, F., and Yu, W. (2016). Hybrid digital and analog beamforming design for large-scale antenna arrays. IEEE J. Sel. Top. Signal Process. 10, 501–513. doi:10.1109/JSTSP.2016.2520912
Spencer, Q. H., Swindlehurst, A. L., and Haardt, M. (2004). Zero-forcing methods for downlink spatial multiplexing in multiuser MIMO channels. IEEE Trans. Signal Process. 52, 461–471. doi:10.1109/TSP.2003.821107
Stavridis, A., Sinanovic, S., Di Renzo, M., and Haas, H. (2012). “Transmit precoding for receive spatial modulation using imperfect channel knowledge,” in Proceeding of the 2012 IEEE 75th Vehicular Technology Conference (VTC Spring), Yokohama, Japan, May 2012 (IEEE), 1–5.
Taha, A., Alrabeiah, M., and Alkhateeb, A. (2021). Enabling large intelligent surfaces with compressive sensing and deep learning. IEEE Access 9, 44304–44321. doi:10.1109/ACCESS.2021.3064073
Tang, W., Dai, J. Y., Chen, M. Z., Wong, K.-K., Li, X., Zhao, X., et al. (2020). MIMO transmission through reconfigurable intelligent surface: System design, analysis, and implementation. IEEE J. Sel. Areas Commun. 38, 2683–2699. doi:10.1109/JSAC.2020.3007055
Thanh Nguyen, N., Nguyen, V.-D., Wu, Q., Tolli, A., Chatzinotas, S., and Juntti, M. (2022). Hybrid active-passive reconfigurable intelligent surface-assisted multi-user MISO systems. in Proceeding of the IEEE 23rd International Workshop on Signal Processing Advances in Wireless Communication (SPAWC), Oulu, Finland, July 2022 (IEEE), 1–5.
Wu, M., Lei, X., Zhou, X., Xiao, Y., Tang, X., and Hu, R. Q. (2021). Reconfigurable intelligent surface assisted spatial modulation for symbiotic radio. IEEE Trans. Veh. Technol. 70, 12918–12931. doi:10.1109/TVT.2021.3121698
Wu, Q., and Zhang, R. (2019). Intelligent reflecting surface enhanced wireless network via joint active and passive beamforming. IEEE Trans. Wireless. Commun. 18, 5394–5409. doi:10.1109/TWC.2019.2936025
Yan, W., Yuan, X., He, Z.-Q., and Kuai, X. (2020). Passive beamforming and information transfer design for reconfigurable intelligent surfaces aided multiuser mimo systems. IEEE J. Sel. Areas Commun. 38, 1793–1808. doi:10.1109/JSAC.2020.3000811
Ye, J., Guo, S., and Alouini, M.-S. (2020). Joint reflecting and precoding designs for SER minimization in reconfigurable intelligent surfaces assisted MIMO systems. IEEE Trans. Wireless. Commun. 19, 5561–5574. doi:10.1109/TWC.2020.2994455
Yigit, Z., Basar, E., and Altunbas, I. (2020). Low complexity adaptation for reconfigurable intelligent surface-based MIMO systems. IEEE Commun. Lett. 24, 2946–2950. doi:10.1109/LCOMM.2020.3014820
Yigit, Z., Basar, E., and Altunbas, I. (2021a). “SimMBM channel simulator for media-based modulation systems,” in Proceeding of the 2021 IEEE 32nd Annual International Symposium on Personal, Indoor and Mobile Radio Communications (PIMRC), Helsinki, Finland, September 2021 (IEEE), 531–536.
Yigit, Z., Basar, E., Wen, M., and Altunbas, I. (2021b). Hybrid reflection modulation. IEEE Trans. Wireless. Commun. (Early Access). doi:10.1109/TWC.2022.3223132
Yu, X., Xu, D., and Schober, R. (2019). “MISO wireless communication systems via intelligent reflecting surfaces,” in Proceeding of the 2019 IEEE/CIC International Conference on Communications in China (ICCC), Changchun, China, August 2019 (IEEE), 735–740.
Zhang, S., and Zhang, R. (2020). Capacity characterization for intelligent reflecting surface aided MIMO communication. IEEE J. Sel. Areas Commun. 38, 1823–1838. doi:10.1109/JSAC.2020.3000814
Zhang, R., Yang, L.-L., and Hanzo, L. (2013). Generalised pre-coding aided spatial modulation. IEEE Trans. Wireless. Commun. 12, 5434–5443. doi:10.1109/TWC.2013.100213.130848
Zhang, J., Zhang, Y., Zhong, C., and Zhang, Z. (2020). Robust design for intelligent reflecting surfaces assisted MISO systems. IEEE Commun. Lett. 24, 2353–2357. doi:10.1109/LCOMM.2020.3002557
Keywords: reconfigurable intelligent surface (RIS), active RIS, over-the-air beamforming, multi-user (MU) transmission, index modulation (IM)
Citation: Yigit Z, Basar E and Altunbas I (2022) Over-the-air beamforming with reconfigurable intelligent surfaces. Front. Comms. Net 3:1016270. doi: 10.3389/frcmn.2022.1016270
Received: 10 August 2022; Accepted: 25 November 2022;
Published: 07 December 2022.
Edited by:
Jules M. Moualeu, University of the Witwatersrand, South AfricaCopyright © 2022 Yigit, Basar and Altunbas. This is an open-access article distributed under the terms of the Creative Commons Attribution License (CC BY). The use, distribution or reproduction in other forums is permitted, provided the original author(s) and the copyright owner(s) are credited and that the original publication in this journal is cited, in accordance with accepted academic practice. No use, distribution or reproduction is permitted which does not comply with these terms.
*Correspondence: Zehra Yigit, yigitz@itu.edu.tr